ABSTRACT
The recent discovery of browning of white adipose tissue (WAT) has raised great research interest because of its significant potential in counteracting obesity and type II diabetes. However, the mechanisms underlying browning are still poorly understood. Liver X receptors (LXRs) are one class of nuclear receptors, which play a vital role in regulating cholesterol, triglyceride and glucose metabolism. Following our previous finding that LXRs serve as repressors of UCP1 in classic brown adipose tissue in female mice, we found that LXRs, especially LXRβ, also repress the browning process of subcutaneous adipose tissue (SAT) in male rodents fed a normal diet. Depletion of LXRs activated thyrotropin releasing hormone positive neurons in the paraventricular area of the hypothalamus, and thus stimulated secretion of thyroid-stimulating hormone from the pituitary. Consequently production of thyroid hormones in the thyroid gland and circulating thyroid hormone level were increased. Moreover, the activity of thyroid signaling in SAT was markedly increased. One unexpected finding of our study is that LXRs are indispensable in the thyroid hormone negative feedback loop at the level of the hypothalamus. LXRs maintain expression of thyroid receptors in the brain and when they are inactivated there is no negative feedback of thyroid hormone in the hypothalamus. Together, our findings have uncovered the basis of increased energy expenditure in male LXR knock-out mice and provided support for targeting LXRs in treatment of obesity.
Liver X receptors (LXRs) α (NR1H3) and β (NR1H2) are two members of the nuclear receptor family involved in multiple metabolic pathways including insulin sensitivity, metabolism of glucose, lipid and cholesterol and energy expenditure.Citation1 Following ligand binding, they repress or activate transcription of target genes by binding to specific sites on DNA and interacting with co-repressors or co-activators. In addition to sharing similar ligands and binding sites on DNA, LXRα and LXRβ also share a high degree of sequence homology.Citation1 The differences in their biological functions seem, at least partially, to be due to their different tissue distribution. LXRα is mainly expressed in organs that are involved in lipid metabolism such as liver, intestine, macrophages and adipose tissue. LXRβ is more widely expressed in the immune system, in glial cells in the central nervous system, the gall bladder, islets of the pancreas, skeletal muscle and the prostate epithelium.Citation1 Both receptors exert important regulatory functions in atherosclerosis, inflammation, central nervous system development and metabolic syndrome.
The metabolic syndrome is a constellation of related disorders characterized by obesity, insulin resistance, dyslipidemia, fatty liver, hypertension and atherosclerosis.Citation2,3 Of note, obesity, which is due to the chronic imbalance between energy intake and energy expenditure, represents a leading event in the metabolic syndrome and a major challenge in counteracting this epidemic is to identify possible targets that either can decrease energy intake or increase energy expenditure.Citation4 As a consequence, there is now a great interest in the investigation of brown adipose tissue, which is specialized for the dissipation of chemical energy in the form of heat.Citation5 However, adult humans lack the thermogenic interscapular organ Citation6 which is found in newborns. Recently, studies have demonstrated that adult humans harbor a distinct cold-inducible depot of brown adipocytes that are interspersed among certain white adipose tissues in the supraclavicular, para-aortic and suprarenal regions.Citation7-9 These cells, called beige or brite fat cells, undergo a browning process upon cold exposure and share some molecular, histologic and functional characteristics with beige adipocytes found in the subcutaneous white adipose tissue (SAT) of mice.Citation10,11 This finding has raised a strong clinical interest in the possibility of therapeutically targeting beige fat for the treatment of obesity.
For the past ten years, our team has investigated the role of LXR in adipose tissue and has found that LXRs negatively regulate the activity of interscapular brown adipose tissue (BAT), specifically in female mice.Citation12,13 After gene expression profiling of BAT, we found that several energy-dissipation related genes are highly down-regulated after activation of LXR ligands.Citation14 When fed a normal chow diet, a four-fold induction of Uncoupling Protein 1 (UCP1) expression in BAT was observed in LXRα knock-out mice (LXRα KO), but not in LXRβ knockout mice (LXRβ KO).Citation12 In line with our observation, Wang et al. showed that LXRα is a direct transcriptional inhibitor of UCP1 expression in BAT.Citation15 Surprisingly, in male LXR KO mice, the opposite has been reported. By using two different lines of LXRαβ knock-out mice (LXRαβ KO), both the Kalaany et al. group as well as our group found that knockout of both LXRα and LXRβ increases energy expenditure in both male and female rodents.Citation12,16 However, the increased activity of BAT only occurs in female mice and the resistance to diet-induced obesity in LXRαβ KO male mice appears to be due to ectopic expression of UCP1 in gonadal white adipose tissues (gonadal WAT) and skeletal muscles.Citation16 Not long after, detailed histological examination of skeletal musclesCitation17 revealed that, rather than being expressed in myocytes, UCP1 is expressed in mitochondria of brown adipocytes interspersed between muscle bundles. Long before the concept of ‘browning’ or the discovery of beige cells, we and several other groups have reported the expression of UCP1 in white adipose tissue under certain circumstances.Citation14,16,18,19 The contribution to energy consumption by these cells was not thoroughly investigated. In fact, the role of LXRs in the browning of white adipocytes was not addressed until our recent discovery that LXRβ directly affects the recruitment of beige cells in the subcutaneous white adipose tissue in vivo.
Male LXRβ and LXRαβ KO mice, remained lean when fed a normal chow diet, but LXRα KO mice did not. Along with adipose organ weight changes, UCP1 expression and a series of beige-cell specific genes were markedly increased in SAT from LXRβ and LXRαβ KO mice. We observed that beige adipocytes are most abundant in the SAT not in gonadal fat in rodents This observation may explain why Kalaany et al. found an increase of UCP1 expression in gonadal WAT of LXRαβ KO mice only after they were being fed a western diet.
The thermogenic response of BAT or browning of WAT is controlled by adrenergic (central) and non-adrenergic (peripheral, mostly hormone-regulated) pathways. Heat production is stimulated by the sympathetic nervous system (SNS), but it has an absolute requirement for a crucial hormone, thyroid hormone (TH).Citation20 While T3 and norepinephrine (NE) each increase UCP1 expression by 2-fold separately, there is a 20-fold induction of UCP1 when both agents are combined.Citation21 TH is secreted from the thyroid gland under the regulation of the hypothalamic-pituitary axis (HPT axis). It is released into the circulatory system and delivered to target organs such as liver and adipose tissues, where specific transporters facilitate its movement into the cell and subsequently its transcription of target genesCitation22 With the knowledge that TH could contribute to the augmentation of energy expenditure, we tested its signaling activity in SAT in LXRαβ KO mice. As we expected, TH signaling, measured by induced expression of TRs, TH transporters and deiodinase 2 (DIO2), was significantly stimulated in SAT of LXRαβ KO mice. DIO2 is the enzyme responsible for rapid increase in intracellular T3 by conversion of T4. Having identified elevated action of TH in SAT, we sought to characterize the regulation of TH synthesis systemically. As demonstrated by ELISA tests, the concentration of T3 was increased in the circulatory system, and this was attributed to the increased expression of TH synthesis genes and TSHR, the receptor for thyroid stimulating hormone (TSH) that directly modulates TH. Although there is no direct evidence that LXR is able to regulate the activity of TH synthesis in the thyroid gland, it has been accepted that LXR is an important repressor of DIO2. Christoffolete et al. demonstrated that LXR/RXR signaling inhibits hepatic DIO2 expression and activity both in vivo and in vitro.Citation23
One of the most surprising and significant findings from this study was the over-active HPT axis identified in LXR KO mice, which suggested to us that the regulation of TH feedback by LXR in the hypothalamus significantly contributed to the increased TH synthesis in the LXR KO mice. Neurons that express thyrotropin releasing hormone (TRH) in PVN project to the portal system, through which they reach the thyrotropin producing cells of the anterior pituitary. TSH, which is subsequently released, binds to its receptor (TSHR) in the thyroid gland where TH is produced and released.Citation22 In line with the observation that LXRβ predominantly controlled the browning of SAT, it was also the predominant LXR expressed in the paraventricular nucleus (PVN) area of the hypothalamus. Inactivation of both receptors in the PVN of knockout mice, de-repressed TRH expression, subsequently promoting TSH expression in the anterior pituitary. The repressive function of LXR on TRH was also confirmed by Ghaddab-Zroud et al.Citation24 They showed that LXR is able to bind to the promoter region of TRH in the hypothalamus and transcriptionally repress its mRNA expression. Local conversion of T4 to T3, by DIO2, provides negative feedback through binding to TRs at the level of thyrotrophs in the pituitary and tanycytes in the hypothalamus. This results in reduction in TRH and TSH secretion in response to adequate tissue level of TH. Nevertheless, the negative feedback loop appeared to be broken in the hypothalamus of LXRαβ KO mice. Somehow, the depletion of LXRs caused loss of TRs, especially TRβ in the PVN area. Unlike what we have discovered in the cortex of the brain, where TRα is partially capable of compensating for LXRβ's function during postnatal brain development,Citation25 the existence of LXRs seemed indispensable for maintaining expression of TR and negative feedback in the PVN. In this way, TRH-positive neurons are no longer repressed by TH and continuously release stimulatory signaling. However, the underlying mechanism remains open for further investigation. Since no suppression of mRNA level of TRs has been observed (unpublished data), post-translational modification of TR mediated by LXR or their co-factors might provide a possible explanation.
Our study not only identifies LXR as a novel repressor of the browning response in SAT through promoting HPT axis, importantly, we also highlight its previously unrevealed role in mediating the classic thyroid hormone negative feedback loop (). We believe that this finding will provide a new understanding about the neuroendocrine regulation of thyroid hormone and an alternative way for the treatment of obesity.
Figure 1. Schematic diagram of actions of LXRβ in controlling of thyroid hormone feedback in the brain and browning of SAT. TRH expressed by the neurons in PVN of the hypothalamus stimulates release of TSH from the anterior pituitary, which in turn stimulates thyroid hormone synthesis at the thyroid gland. T4/T3 in the circulation enter their target organs such as SAT and activate the browning process. In reverse, the circulating thyroid hormones negatively regulate their own production through targeting both pituitary and hypothalamus. LXRβ is able to transcriptionally inhibit the expression of TRH in the PVN area. Genetic depletion of LXRs releases their transcriptional suppression on TRH and breaks the negative feedback loop due to the lack of TRs in the PVN area, thus promotes TSH secretion in the pituitary and activates the synthesis of thyroid hormone, which eventually increases the browning of SAT.
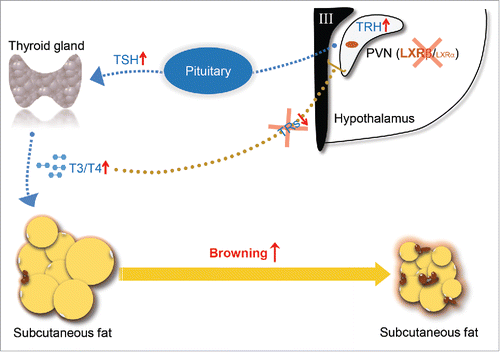
At present, the thought of targeting LXR to treat human obesity does not appear to be a viable pharmacological approach. In addition to causing diseases associated with high levels of thyroid hormone, there are many important physiological functions of LXR in the brain including anti-inflammatory actions, which protect the brain against neurodegeneration. Perhaps, in the future, LXR isoform-selective antagonists will be developed which will deliver the wanted effects and leave the beneficial effects of these receptors intact. Meanwhile, much work has been done on the study of the effects of thyroid hormone in human adipocytes Citation26 for review. Direct targeting of thyroid hormone receptors with the synthetic thyroid agonist (GC-1) appears to be a more promising approach to the treatment of obesity.
Abbreviations
WAT | = | white adipose tissue |
LXRs | = | liver X receptors |
SAT | = | subcutaneous adipose tissue |
BAT | = | brown adipose tissue |
LXRα KO | = | LXRα knockout mice |
LXRβ KO | = | LXRβ knock-out mice |
LXRαβ KO | = | LXRαβ knockout mice |
SNS | = | sympathetic nervous system |
TH | = | thyroid hormone |
NE | = | norepinephrine |
HPT | = | hypothalamic-pituitary-thyroid gland |
DIO2 | = | deiodinase 2 |
TSH | = | thyroid stimulating hormone |
TSHR | = | the receptor for TSH |
TRH | = | thyrotropin releasing hormone |
PVN | = | paraventricular nucleus |
ERs | = | estrogen receptors |
TRs | = | thyroid hormone receptors |
UCP1 | = | uncoupling protein 1 |
Disclosure of potential conflicts of interest
No potential conflicts of interest were disclosed.
References
- Korach-Andre M, Gustafsson JA. Liver X receptors as regulators of metabolism. Biomol Concepts 2015; 6:177-90; PMID:25945723; http://dx.doi.org/10.1515/bmc-2015-0007
- Barros RP, Gustafsson JA. Estrogen receptors and the metabolic network. Cell Metab 2011; 14:289-99; PMID:21907136; http://dx.doi.org/10.1016/j.cmet.2011.08.005
- Wang C, Chi Y, Li J, Miao Y, Li S, Su W, Jia S, Chen Z, Du S, Zhang X, et al. FAM3A activates PI3K p110alpha/Akt signaling to ameliorate hepatic gluconeogenesis and lipogenesis. Hepatology 2014; 59:1779-90; PMID:24806753; http://dx.doi.org/10.1002/hep.26945
- Harms M, Seale P. Brown and beige fat: development, function and therapeutic potential. Nature Med 2013; 19:1252-63; PMID:24100998; http://dx.doi.org/10.1038/nm.3361
- Enerback S. Human brown adipose tissue. Cell Metab 2010; 11:248-52; PMID:20374955; http://dx.doi.org/10.1016/j.cmet.2010.03.008
- Lidell ME, Betz MJ, Enerback S. Two types of brown adipose tissue in humans. Adipocyte 2014; 3:63-6; PMID:24575372; http://dx.doi.org/10.4161/adip.26896
- Cypess AM, Lehman S, Williams G, Tal I, Rodman D, Goldfine AB, Kuo FC, Palmer EL, Tseng YH, Doria A, et al. Identification and importance of brown adipose tissue in adult humans. New Eng J Med 2009; 360:1509-17; PMID:19357406; http://dx.doi.org/10.1056/NEJMoa0810780
- Saito M, Okamatsu-Ogura Y, Matsushita M, Watanabe K, Yoneshiro T, Nio-Kobayashi J, Iwanaga T, Miyagawa M, Kameya T, Nakada K, et al. High incidence of metabolically active brown adipose tissue in healthy adult humans: effects of cold exposure and adiposity. Diabetes 2009; 58:1526-31; PMID:19401428; http://dx.doi.org/10.2337/db09-0530
- van Marken Lichtenbelt WD, Vanhommerig JW, Smulders NM, Drossaerts JM, Kemerink GJ, Bouvy ND, Schrauwen P, Teule GJ. Cold-activated brown adipose tissue in healthy men. N Eng J Med 2009; 360:1500-8; http://dx.doi.org/10.1056/NEJMoa0808718
- Wu J, Bostrom P, Sparks LM, Ye L, Choi JH, Giang AH, Khandekar M, Virtanen KA, Nuutila P, Schaart G, et al. Beige adipocytes are a distinct type of thermogenic fat cell in mouse and human. Cell 2012; 150:366-76; PMID:22796012; http://dx.doi.org/10.1016/j.cell.2012.05.016
- Wu J, Cohen P, Spiegelman BM. Adaptive thermogenesis in adipocytes: is beige the new brown? Genes Dev 2013; 27:234-50; PMID:23388824; http://dx.doi.org/10.1101/gad.211649.112
- Korach-Andre M, Archer A, Barros RP, Parini P, Gustafsson JA. Both liver-X receptor (LXR) isoforms control energy expenditure by regulating brown adipose tissue activity. Proc Natl Acad Sci U S A 2011; 108:403-8; PMID:21173252; http://dx.doi.org/10.1073/pnas.1017884108
- Korach-Andre M, Parini P, Larsson L, Arner A, Steffensen KR, Gustafsson JA. Separate and overlapping metabolic functions of LXRalpha and LXRbeta in C57Bl/6 female mice. Am J Phy Endocrinol Metab 2010; 298:E167-78; http://dx.doi.org/10.1152/ajpendo.00184.2009
- Stulnig TM, Steffensen KR, Gao H, Reimers M, Dahlman-Wright K, Schuster GU, Gustafsson JA. Novel roles of liver X receptors exposed by gene expression profiling in liver and adipose tissue. Mol Pharmacol 2002; 62:1299-305; PMID:12435796; http://dx.doi.org/10.1124/mol.62.6.1299
- Wang H, Zhang Y, Yehuda-Shnaidman E, Medvedev AV, Kumar N, Daniel KW, Robidoux J, Czech MP, Mangelsdorf DJ, Collins S. Liver X receptor α is a transcriptional repressor of the uncoupling protein 1 gene and the brown fat phenotype. Mol Cell Biol 2008; 28:2187-200; PMID:18195045; http://dx.doi.org/10.1128/MCB.01479-07
- Kalaany NY, Gauthier KC, Zavacki AM, Mammen PP, Kitazume T, Peterson JA, Horton JD, Garry DJ, Bianco AC, Mangelsdorf DJ. LXRs regulate the balance between fat storage and oxidation. Cell Metab 2005; 1:231-44; PMID:16054068; http://dx.doi.org/10.1016/j.cmet.2005.03.001
- Almind K, Manieri M, Sivitz WI, Cinti S, Kahn CR. Ectopic brown adipose tissue in muscle provides a mechanism for differences in risk of metabolic syndrome in mice. Proc Natl Acad Sci U S A 2007; 104:2366-71; PMID:17283342; http://dx.doi.org/10.1073/pnas.0610416104
- Cousin B, Cinti S, Morroni M, Raimbault S, Ricquier D, Penicaud L, Casteilla L. Occurrence of brown adipocytes in rat white adipose tissue: molecular and morphological characterization. J Cell Sci 1992; 103(Pt 4):931-42; PMID:1362571
- Young P, Arch JR, Ashwell M. Brown adipose tissue in the parametrial fat pad of the mouse. FEBS Lett 1984; 167:10-4; PMID:6698197; http://dx.doi.org/10.1016/0014-5793(84)80822-4
- Silva JE, Rabelo R. Regulation of the uncoupling protein gene expression. Eur J Endocrinol Eur Feder Endocrine Societies 1997; 136:251-64; http://dx.doi.org/10.1530/eje.0.1360251
- Bianco AC, Sheng XY, Silva JE. Triiodothyronine amplifies norepinephrine stimulation of uncoupling protein gene transcription by a mechanism not requiring protein synthesis. J Biol Chem 1988; 263:18168-75; PMID:3192531
- Mullur R, Liu YY, Brent GA. Thyroid hormone regulation of metabolism. Physiol Rev 2014; 94:355-82; PMID:24692351; http://dx.doi.org/10.1152/physrev.00030.2013
- Christoffolete MA, Doleschall M, Egri P, Liposits Z, Zavacki AM, Bianco AC, Gereben B. Regulation of thyroid hormone activation via the liver X-receptor/retinoid X-receptor pathway. J Endocrinol 2010; 205:179-86; PMID:20176747; http://dx.doi.org/10.1677/JOE-09-0448
- Ghaddab-Zroud R, Seugnet I, Steffensen KR, Demeneix BA, Clerget-Froidevaux MS. Liver X receptor regulation of thyrotropin-releasing hormone transcription in mouse hypothalamus is dependent on thyroid status. PloS One 2014; 9:e106983; PMID:25229406; http://dx.doi.org/10.1371/journal.pone.0106983
- Fan X, Kim HJ, Bouton D, Warner M, Gustafsson JA. Expression of liver X receptor β is essential for formation of superficial cortical layers and migration of later-born neurons. Proc Natl Acad Sci U S A 2008; 105:13445-50; PMID:18768805; http://dx.doi.org/10.1073/pnas.0806974105
- Obregon MJ. Adipose tissues and thyroid hormones. Front Physiol 2014; 5:479-91; PMID:25566082; http://dx.doi.org/10.3389/fphys.2014.00479