ABSTRACT
The tumor microenvironment (TME) contains high levels of the Wnt family of ligands, and aberrant Wnt-signaling occurs in many tumors. Past studies have been directed toward how the Wnt signaling cascade regulates cancer development, progression and metastasis. However, its effects on host antitumor immunity remain unknown. In this report, we show that Wnts in the TME condition dendritic cells (DCs) to a regulatory state and suppress host antitumor immunity. DC-specific deletion of Wnt co-receptors low-density lipoprotein receptor-related protein 5 and 6 (LRP5/6) in mice markedly delayed tumor growth and enhanced host antitumor immunity. Mechanistically, loss of LRP5/6-mediated signaling in DCs resulted in enhanced effector T cell differentiation and decreased regulatory T cell differentiation. This was due to increased production of pro-inflammatory cytokines and decreased production of IL-10, TGF-β1 and retinoic acid (RA). Likewise, pharmacological inhibition of the Wnts' interaction with its cognate co-receptors LRP5/6 and Frizzled (Fzd) receptors had similar effects on tumor growth and effector T cell responses. Moreover, blocking Wnt-signaling in DCs resulted in enhanced capture of tumor-associated antigens and efficient cross-priming of CD8+ T cells. Hence, blocking the Wnt pathway represents a potential therapeutic to overcome tumor-mediated immune suppression and augment antitumor immunity.
Introduction
The Wnt signaling cascade plays an important role in the development and differentiation of immune cells Citation1,2 as well as in tissue homeostasis Citation3 Wnts bind to Fzd receptors and activate multiple signaling pathways, including the canonical pathway that regulates transcription of target genes through β-catenin/TCF signaling and the non-canonical pathway that is independent of β-catenin. Co-receptors LRP5 and LRP6 are critical for canonical Wnt-signaling.Citation1,3,4 Tumors actively suppress immune response through multiple mechanisms by creating tolerance to their own antigens and DCs play a key role in this process.Citation5-8 While DC-driven immune suppression has been extensively studied, signaling networks that program DCs to a regulatory state in TME are not fully understood. TME contains high levels of Wnts and Wnt/β-catenin pathway is dysregulated in many tumors.Citation9-12 However, how Wnts in TME regulate antitumor immunity and drive tumor-induced immune suppression remain poorly understood. In settings of inflammation, our recent studies have shown that the Wnt-signaling pathway in DCs acts as a counter regulatory mechanism to control inflammation and restores homeostasis.Citation13,14 Furthermore, other studies have shown that ex vivo conditioning of DCs with Wnts induces regulatory T cell differentiation and suppresses effector T cell differentiation.Citation14,15 Thus, these observations led us to hypothesize that increased Wnts in the TME could modulate effector functions of DCs to program them to a regulatory state, which effectively suppresses host effector T cell responses and induce tumor immune tolerance.
In the present study, we show that loss of co-receptors LRP5 and LRP6 in DCs results in reduced tumor growth with enhanced antitumor immune responses. Thus, deletion of LRP5 and LRP6 in DCs resulted in decreased expression of immune regulatory factors such as IL-10, TGF-β and RA with concomitant increase in inflammatory cytokine production. Interestingly, loss of LRP5/6 in DCs promoted efficient capture of tumor-associated antigens by DCs and cross-priming of effector CD8+ T cells, indicating a critical role for Wnt signaling in suppressing host antitumor immunity. Furthermore, our study demonstrates that pharmacological blocking of Wnt-signaling is an attractive means of attenuating tumor-induced immune tolerance via enhancement of effector T cell responses with concomitant suppression of regulatory T cell differentiation.
Results
Deletion of LRP5 and LRP6 in dendritic cells impairs tumor growth
To determine the role of Wnts in regulating host antitumor immunity, we first analyzed the expression of different Wnt ligands in TME and tumor-draining lymph nodes (TDLNs), using B16 melanoma cells expressing ovalbumin (B16-OVA) implanted subcutaneously (s.c) in syngenic C57BL/B6 (B6) mice. As shown in , we noted increased expression of multiple Wnts in the TDLN compared to the control lymph nodes (CLN). Similarly, implantation of EL4 thymoma cells expressing ovalbumin (EL4-OVA) or Lewis lung carcinoma (LLC) cells resulted in a significant increase in Wnts in the TDLN (). Moreover, we also observed a similar pattern of Wnt expression in B16-OVA, EL4-OVA and LLC tumors isolated from tumor-bearing mice (data not shown). Our recent study has shown that DCs express both the co-receptors LRP5 and LRP6 that are critical for efficient Wnt-mediated signaling.Citation14 Therefore, we reasoned that the Wnts in TME could activate canonical Wnt pathway in DCs and suppress antitumor immunity with increased tumor progression. As shown in , B16-OVA tumor growth was significantly reduced in the mice lacking LRP5/6 in DCs (LRP5/6ΔDC mice) compared to control-floxed (WT) mice. Similarly, EL4-OVA or LLC tumor growth is markedly reduced in the LPΠ5/6ΔDC mice compared to control-floxed (WT) mice (). Taken together, these data indicated that Wnts in the TME could promote tumor progression partly through activation of Wnt-LRP5/6 signaling in DCs.
Figure 1. Tumors induce Wnt expression and DC-specific deletion of Wnt co-receptors LRP5 and LRP6 delays tumor growth in mice. Real-time PCR analysis for mRNA levels of different Wnts in CLNs (control lymph nodes ) from tumor-free mice or TDLNs (tumor-draining lymph nodes) on day 9 after MO4 (A), EL4 (B) and LLC (C) tumor inoculation in B6 WT mice (n = 5). Data are represented as fold increase over CLN (mean ± SEM). B16-OVA (D), EL4-OVA (E) and LLC (F) tumor progression in LRP5/6ΔDC and Control-FL mice (n = 6–8). Data are representative of three independent experiments and show mean values ± SEM. *p < 0.05; **p < 0.01; ***p < 0.001.
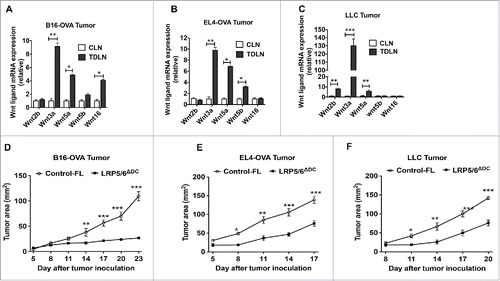
Wnt-LRP5/6-mediated signaling in DCs suppresses antitumor immunity
Next, we examined the effector phenotype of tumor-infiltrating lymphocytes (TILs) isolated from LRP5/6ΔDC mice. Analysis of T cells from B16 tumors and TDLN of LRP5/6ΔDC mice showed a significant increase in frequencies of IFNγ+ CD4+, IFNγ+ CD8+, Granzyme B+ CD8+ (GzmB+ CD8+) and TNF-α+ CD8+ T cells compared to control mice (; S1A). Interestingly, there was a significant decrease in the frequencies of Foxp3+CD4+ T cells, IL-10+CD4+ Tr1 cells and IL-10+CD8+ regulatory T cells in B16 tumor bearing LRP5/6ΔDC mice compared to the control group (). We observed identical trends in LRP5/6ΔDC mice bearing EL4 and LLC tumors (data not shown). Next, we analyzed tumor specific immune responses by co-culturing TDLN lymphocytes and DCs pulsed with a B16 tumor extract. As shown in , TDLN lymphocytes from LRP5/6ΔDC mice produced significantly higher levels of IFNγ and TNF-α and lower levels of IL-10 compared to TDLN lymphocytes from WT mice. Collectively, these results suggest that Wnt-LRP5/6 signaling in DCs promotes tumor immune tolerance through differential regulation of effector and regulatory T cell responses.
Figure 2. Wnt ligands in the TME activate the β-catenin/TCF pathway in DCs and DC-specific deletion of LRP5/6 enhances antitumor immunity in mice. (A–D) Representative dot plots and percentages of IFNγ+CD4+, IFNγ+CD8+, Granzyme B+CD8+, TNF-α+CD8+, IL-10+CD4+, Foxp3+CD4+ and IL-10+ CD8+ T cells isolated from MO4 tumors in Control-FL and LRP5/6ΔDC mice on day 14 post-inoculation (n = 6). (E) Cytokine concentrations in supernatants obtained after culture of TDLN lymphocytes with WT DCs loaded with B16 lysate for 48 h (n = 5). Data represents one of two experiments with similar results and shows mean values ±SEM. *p < 0.05; **p < 0.01; ***p < 0.001.
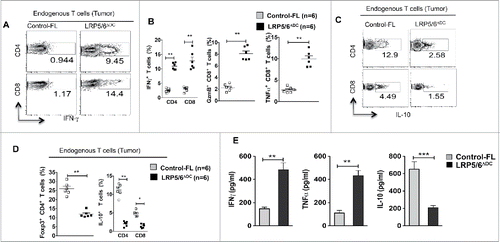
Wnt-LRP5/6-mediated signaling in DCs suppresses effector T cell differentiation but promotes regulatory T cell differentiation
Functional activation of T cells by DCs usually occurs in the TDLNs.Citation16 Tumors condition DCs to acquire regulatory properties that mostly induce regulatory T cell responses.Citation6,16,17 To determine whether canonical Wnt signaling limits tumor antigen-specific CD8+ T cell responses, we transferred naive OT-I T cells into control WT and LRP5/6ΔDC mice bearing B16-OVA tumors. As shown in , we noted a significant increase in the frequencies of IFNγ+, TNF-α+ and GzmB+ CD8+OT-IThy1.1+ T cells in B16-OVA tumors isolated from LRP5/6ΔDC mice compared to control mice. These results suggest that activation of Wnt-LRP5/6-mediated signaling in DCs suppresses the differentiation of effector CD8+ T cells in response to tumor. Past studies have shown that activation of β-catenin in DCs promotes regulatory T cell responses while suppressing effector T cell responses.Citation13,18,19 In parallel, other studies have shown tumors activate β-catenin in DCs.Citation20-23 Therefore, we hypothesized that that tumor-induced activation of the Wnt-LRP5/6 pathway imparts a regulatory phenotype on DCs that promotes regulatory T cell responses. To test this hypothesis, LRP5/6ΔDC and control mice bearing B16-OVA tumors were adoptively transferred with naive OT-IIThy1.1+ T cells on day 9 post tumor induction as described in our previous study.Citation20 As shown in , tumor bearing LRP5/6ΔDC mice showed a significant reduction in OVA-specific induced Foxp3+ CD4+ Treg cells in TDLN and tumors compared to control mice. Based on the these observations, we hypothesize that the reduced tumor growth in LRP5/6ΔDC mice is due to enhanced effector T cell responses and reduced regulatory T cell responses. To test this, we depleted CD4+ or CD8+ T cells in LRP5/6ΔDC mice using antibodies against CD4+ or CD8+ cells on the same day as B16-OVA (MO4) or EL4-OVA (EG7) tumor implantation and again on day 15 after tumor implantation. α-CD8+ or α-CD4+ treatment resulted in a significant increase in tumor size compared to untreated mice in LRP5/6ΔDC mice (), indicating that CD4+ and CD8+ cells are at least in part responsible for delayed tumor growth in mice lacking canonical Wnt signaling in DCs. Taken together, these data demonstrate that the activation of canonical Wnt signaling in DCs differentially regulates effector and regulatory T cell differentiation, and induces active immune tolerance to tumors.
Figure 3. Loss of LRP5/6 in DCs promotes effector T cell differentiation whereas limits regulatory T cell differentiation. (A, B) In vivo OT-I CD8+ T cell differentiation in Control-FL and LRP5/6ΔDC mice in response to B16-OVA (MO4) tumors. Representative dot plots and percentages percentage of OVA-specific IFNγ+CD8+, Granzyme B+CD8+ and TNF-α+CD8+ T cells in tumors isolated from Control-FL and LRP5/6ΔDC mice (n = 6). (C, D) In vivo OT-II Treg differentiation in Control-FL and LRP5/6ΔDC mice in response to B16-OVA (MO4) tumors (n = 6). (E) MO4 melanoma and (F) EG7 tumor growth in LRP5/6ΔDC in response to α-CD4+ antibody or α-CD8+ antibody treatment (n = 6). Data represents cumulative percentage of OVA-specific Foxp3+CD4+ T cells in TDLNs and tumors isolated from Control-FL and LRP5/6ΔDC mice (n = 5). *p < 0.05; **p < 0.01; ***p < 0.001.
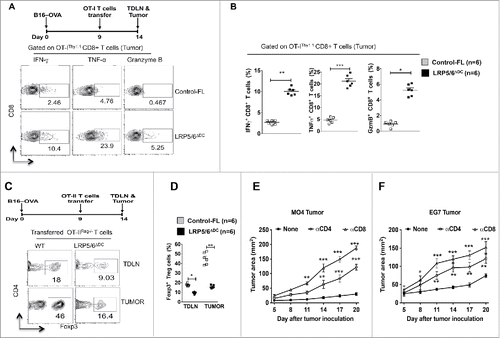
Canonical Wnt signaling in DCs induces the expression of immune regulatory factors while suppressing the expression of inflammatory cytokines
DCs control the fate of naive T cell differentiation through the secretion of various inflammatory or anti-inflammatory cytokines.Citation8,24 The above results prompted us to assess whether canonical Wnt signaling regulates DC function through the expression of inflammatory and anti-inflammatory cytokines in response to tumors. Accordingly, DCs isolated from the TDLN of LRP5/6ΔDC mice bearing B16 tumors showed a significant reduction in mRNA levels for immune regulatory genes such as IL-10, TGF-β1, and Aldh1a2 in LRP5/6ΔDC DCs compared to Control-FL DCs (). In contrast, TDLN DCs from LRP5/6ΔDC mice showed significant increases in the expression of inflammatory cytokines such as IL-6, TNF-α, IL-12p19 and IL-12p35 compared to the TDLN DCs from Control-FL mice (). Our previous studies have shown that induction of IL-10, TGF-β1, and Aldh1a2 in DCs is dependent on β-catenin pathway.Citation13,14,19,20 So, we analyzed whether lack of LRP5/6 in DCs during tumor progression affects activation of downstream signaling pathway, particularly activation of. β-catenin/TCF pathway. As shown in , deletion of LRP5/6 in DCs resulted in a marked decrease in β-catenin activation and expression of its target gene Axin2. Consistent with previous observations,Citation15,25,26 Wnts such as 3a, 5b and 16 that are present in the TME also activates β-catenin/TCF pathway in DCs (). Altogether, these data demonstrate that the canonical Wnt-signaling pathway in DCs promotes Treg cell differentiation by inducing immune regulatory factors but suppresses effector T cell differentiation by limiting the expression of inflammatory cytokines.
Figure 4. Loss of LRP5/6 in DCs differentially regulates pro- and anti-inflammatory molecules. (A–B) Quantitative real-time PCR analysis of Il-12p40, Il-12p35, Il-6, Il-12p19, Il10, Aldh1a1 and Aldh1a2 mRNA expression in CD11c+ DCs isolated from the TDLN of WTFL/FL and LRP5/6ΔDC mice on day 9 post-B16 tumor inoculation (n = 3). (C) Representative histograms of active β-catenin expression in CD11c+ cells from MO4 tumor TDLN of LRP5/6Δce and control-FL mice on day 9 after tumor inoculation. (D) Axin2 mRNA expression analyzed by qRT-PCR in TDLNs and CLNs of LRP5/6ΔAx and control-FL mice on day 9 after inoculation (n = 4). The result represents fold increase over the CLNs. (E) Representative histograms showing β-gal expression in CD11c+ cells from TCF/LEF-LacZ reporter mice after treatment with or without Wnt2b, Wnt3a, Wnt5a, Wnt5b, or Wnt16b (500 ng/mL) for 24 h in vitro. DCs were pooled from five to six mice, and the experiment was repeated at least two times. Data represents one of two experiments with similar results. *p < 0.05; **p < 0.01; ***p < 0.001.
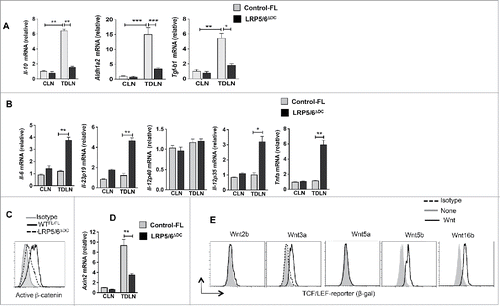
Deletion of LRP5/6 in DCs augments antigen uptake and cross-priming of CD8+ T cells
Effective priming of T cells by DCs involves efficient capture and cross-presentation of tumor-associated antigens.Citation7,27 However, tumors actively suppress capture and cross-presentation of tumor-associated antigen by DCs. Thus, we hypothesized that DCs deficient in LRP5/6 are more efficient in their capacity to capture and cross-present tumor-antigens to T cells. Given the enhanced antitumor immunity in LRP5/6ΔDC mice, we determined whether this is due to efficient capture of tumor antigens. So, we implanted B16-GFP tumor cells in LRP5/6ΔDC mice and control-FL mice followed by analysis of DCs in TDLN on day 7-post tumor implantation. Consistent with our previous data, we observed a significant increase in the frequencies of CD11c+ GFP+ DCs in the TLDN from LRP5/6ΔDC mice compared to WT mice (). Next, we determined whether activation of canonical Wnt-signaling in DCs limits cross-presentation of tumor antigens to CD8+ T cells. To test this, we transferred the CFSE-labeled naive OT-1Thy1.1 CD8+ T cells into LRP5/6ΔDC mice and WT-FL mice bearing B16-OVA tumors. We noted a significant increase in the proliferation of CFSE labeled OT-1Thy1.1 CD8+ T cells in LRP5/6ΔDC mice compared to WT-FL mice (). Likewise, deletion of LRP5/6ΔDC resulted in significant increase in the proliferation of CFSE labeled OT-IIThy1.1 CD4+ cells (). Next, we determined whether the enhanced T cell proliferation observed in LRP5/6ΔDC mice is due to increased migration of DCs to the TDLN. We noted a marked increase in the frequency of CD11c+ DCs in the TDLN of LRP5/6ΔDC mice compared to WT mice (). However, we observed similar levels of MHC class II expression in TDLN CD11c+ DCs isolated from WT and LRP5/6ΔDC mice (). This observation is consistent with previous studies suggesting that the LRP5/6-β-catenin pathway is not critical for DC maturation.Citation14,15,19,20 Collectively, these data suggest that activation of canonical Wnt-LRP5/6 signaling in TME DCs suppresses its migration, efficient capture of tumor-associated antigens and cross-priming of CD8+ T cells.
Figure 5. Loss of LRP5/6 in DCs promotes efficient capture of tumor-associated antigens and cross-priming of CD8+ T cells. (A, B) Control-FL and LRP5/6ΔDC mice were implanted s.c with B16-GFP tumor cells (n = 5). On day 7, TDLN and CLN were harvested and were tested using FACS for DC population. Data represents FACS plot and cumulative frequency showing percentage of CD11c+ GFP+ DCs (n = 8). (C–D) B16-OVA tumor-bearing Control-FL and LRP5/6ΔDC mice were adoptively transferred with CFSE-labeled naive OT-I T cells on day 9 (n = 6). On day 14, the TDLN was harvested and analyzed using FACS for OT-I T cell proliferation. Representative histograms showing cell division and percent proliferated OT-ICFSE cells. Data represents one of two experiments with similar results. ***p < 0.001. (E, F) B16-OVA tumor-bearing Control-FL and LRP5/6ΔDC mice were adoptively transferred with CFSE-labeled naive OT-II T cells on day 9 (n = 6). On day 14, the TDLN was harvested and analyzed using FACS for OT-II T cell proliferation. Representative histograms showing cell division and percent proliferated OT-IICFSE cells. (G) Representative FACS plot showing frequency of CD11c+ cells in the TDLN of B16-OVA tumor bearing Control-FL and LRP5/6ΔDC mice on day 14 after tumor inoculation. (H) Representative histogram shows the expression of MHC Class II by CD11c+ DCs in TDLN of TDLN of B16-OVA tumor-bearing Control-FL and LRP5/6ΔDC mice on day 14 after tumor inoculation.
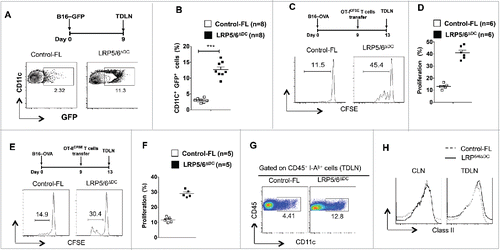
Pharmacological inhibition of the LRP5/6 signaling axis enhances antitumor immunity against tumors
To explore the above findings in a clinically relevant model, we examined the effects of pharmacological inhibition of binding of Wnt-ligands to cognate Fzd receptors using PORCN inhibitor III (IWP-L6).Citation28 Porcupine (POCRN) is a membrane-bound-O-acetyltransferase enzyme that palmitoylates Wnts, which is critical for its interactions with co-receptors LRP5/6 and Fzd receptors. Treatment of mice with established B16-OVA tumors or EL4-OVA tumors with IWP-L6 delayed tumor growth compared to untreated mice (). In addition, IWP-L6 treatment resulted in significant increases in the frequencies of TNFα+CD8+, GzmB+ CD8+, IFNγ+ CD8+ and IFNγ+CD4+ effector T cells infiltrating the tumor (). In contrast, blocking Wnt-signaling resulted in significant decrease in the frequencies of Foxp3+ Tregs and IL-10+ Tr1 cells within the tumors (). Furthermore, the ratio of effector T cells to Treg cells was markedly increased within the tumors of treated mice compared to the control mice (data not shown).
Figure 6. Pharmacological inhibition of Wnt signaling enhances antitumor immunity against established tumors. (A–B) B16F10 melanoma and EL4 tumor progression in WT mice treated with or without IWP-L6 (5 mg/kg) every 3 d from day 5 after tumor inoculation. Data are mean tumor size and are cumulative, representative of two independent experiments (n = 10–12 mice). (C–D) Representative dot plots and percentage of Granzyme B+CD8+, IFNγ+CD8+, and TNFα+CD8+ T cells isolated from MO4 melanoma on day 20 after inoculation (n = 6 mice). Mice were treated with or without IWP-L6 as described in A. (E) Representative percentage of Foxp3+CD4+, IFNγ+CD4+, and IL-10+CD4+ T cells isolated from MO4 tumors on day 20 after inoculation (n = 6 mice). Mice treated with or without IWP-L6 as described in A (F–G) Representative dot plots and percentage of GFP+CD11c+ DCs isolated from TDLNs on day 9 after B16-GFP tumor inoculation (n = 8 mice). Mice were treated with or without IWP-L6 on day 5 and 7. (H, I) MO4 bearing mice were adoptively transferred with CFSE-labeled naive OT-I T cells on day 9 (n = 6). Mice were treated with or without IWP-L6 on day 5, 8 and 11. On day 14, the TDLN was harvested and analyzed using FACS for OT-I T cell proliferation. Representative histograms showing cell division and percent proliferated OT-ICFSE cells. Data represents one of two experiments with similar results. (J) MO4-bearing mice were adoptively transferred with OT-I T cells from Rag−/− OT-I mice on day 9 after tumor inoculation. After 5 d, Granzyme B+CD8+, IFNγ+CD8+, and TNFα+CD8+ analysis was performed on CD45+Vα2+Vβ5.1/5.2+ T cells from TDLNs (n = 6–8 mice). Data are representative of two independent experiments and show mean values±SEM. (K) Cytokine concentrations in supernatants obtained after culture of TDLN lymphocytes with DCs loaded with B16 lysate for 48 h. Mice were treated with or without IWP-L6 as described in A (n = 5). Data are representative of two independent experiments and show mean values ± SEM. Statistical levels of significance were analyzed by the Student t test (unpaired). *p < 0.05; **p < 0.01; ***p < 0.001.
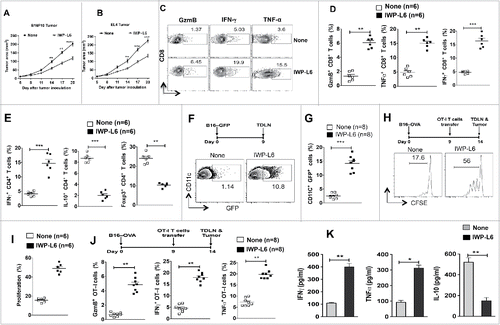
Next, we tested whether IWP-L6 treatment enhances DCs ability to capture and cross-present tumor antigens to CD8+ T cells. Like deletion of LRP5/6 in DCs, blocking Wnt-signaling resulted in significant increase in the frequency of CD11c+ GFP+ DCs in the TLDN of treated mice implanted with B16-GFP tumors (). Similarly, we observed robust proliferation of CFSE-labeled OT-1Thy1.1 CD8+ T cells in LRP5/6ΔDC mice compared to WT-FL mice (). Furthermore, DCs isolated form TDLN of treated mice expressed significantly higher levels of inflammatory cytokines IL-6, TNF-α, IL-12p19 and IL-12p35 and lower levels of IL-10, Aldh1a2 and TGF-β1 (data not shown). Consistent with this observation, IWP-L6 treatment of B16-OVA tumor-bearing mice that had received OT-IThy1.1 CD8+ T cells showed significant increases in the frequencies of TNFα+ CD8+, GzmB+ CD8+ and IFNγ+ CD8+ effector T cells within the tumors (). In contrast, IWP-L6 treatment of B16-OVA tumor-bearing mice that had received OT-II CD4+ T cells showed significant decrease in the frequencies of OT-II+ Foxp3+ Tregs and OT-II+ IL-10+ Tr1 cells within the tumors (data not shown). In line with these observations, TDLN lymphocytes from treated mice co-cultured with DCs pulsed with B16 tumor extract produced significantly higher levels of IFNγ and TNF-α and lower levels of IL-10 compared to TDLN lymphocytes from control mice (). We confirmed these findings using another POCRN inhibitor, C59.Citation9,29 Like IWP-L6 treatment, treatment of tumor-bearing mice with C59 delayed tumor growth (data not shown). Collectively, these data demonstrate that blocking the Wnt-LRP5/6 pathway promotes strong tumor-specific T cell responses by regulating DC function.
Discussion
Aberrant Wnt-signaling occurs in many tumors. However, little is known about the role of Wnts in antitumor immunity. The current study demonstrates for the first time that Wnts in the TME directly drive immune suppression by inducing regulatory DCs and limiting antitumor immunity. This is due to the role of canonical Wnt-signaling by regulating DCs ability to capture tumor-associated antigens and its migration to TDLN. Furthermore, Wnts also limit efficient cross priming of CD8+ T cells by suppressing the expression of inflammatory cytokines and promoting the induction of regulatory factors such as IL-10, RA and TGF-β. This resulted in enhanced regulatory T cell responses and decreased effector T cell responses to tumors. In addition, pharmacologically blocking the interaction of Wnts with LRP5/6 and FZD resulted in delayed tumor growth with enhanced antitumor immunity. Collectively, these findings support the hypothesis that Wnts in the TME induces regulatory DCs, which drives regulatory T cell responses and confines antitumor immunity. Hence, this pathway constitutes a new target for cancer immunotherapy.
The canonical Wnt-signaling that activates the β-catenin/TCF pathway is associated with many human tumors, but the focus of most research has been on the effects of this pathway on the tumor cells themselves.Citation10-12 Wnt ligands in the TME could also initiate paracrine signaling within the immune cells and regulate host antitumor immunity.Citation30 Our present study shows that deletion of LRP5/6 in DCs or blocking Wnt-signaling in mice results in delayed tumor growth with enhanced immune responses. Wnts activate both β-catenin-dependent and -independent signaling pathways in DCs.Citation4 We, as well as others, have shown that tumors activate β-catenin in DCs and promote immune suppression by inducing regulatory T cell response while suppressing T effector cell responses.Citation20-23 Ex vivo conditioning of DCs with Wnt ligands such as Wnt3a, Wnt5b and Wnt16 activates β-catenin pathway and program DCs to a regulatory state.Citation14,15,23,26 Similarly, DCs isolated from melanoma patients displayed a tolerogenic phenotype with increase in the expression and activation of β-catenin.Citation31 In addition to DC-intrinsic LRP5/6-signaling, active Wnt/β-catenin signaling within melanomas also promotes immune suppression.Citation32,33 Thus, our data indicates that signaling initiated by Wnts in the TME activates the β-catenin/TCF pathway in DCs, which suppresses host antitumor immune responses and promotes tumor growth.
The normal initiation and activation of a robust antitumor immune response is associated with migration, efficient capture and presentation of tumor-associated antigens by DCs to T cells.Citation8,34 It is well documented that immune regulatory factors in the TME suppress this process.Citation6,35 The present study shows that canonical Wnt-signaling in DCs plays an important role in limiting DC migration and its ability to the uptake of tumor antigens. Deletion of LRP5/6 in DCs enhanced its ability to capture and present tumor antigens to T cells. Likewise, our experiments also show that blocking Wnts' interaction with cognate receptors enhances the capture and cross-presentation of tumor antigens. In addition, deletion of LRP5/6 in DCs or blocking Wnt-signaling resulted in increased migration of DCs to TDLN. In line with this observation, a recent study has elegantly shown that active Wnt/β-catenin signaling in tumors suppresses the recruitment of DCs and T cells.Citation32 However, it remains to be determined how DC-intrinsic LRP5/6-signaling regulates its migration to TDLN and tumors. Thus, our study identifies that canonical Wnt-signaling regulates DC migration and its ability to capture tumor-associated antigens.
Lastly, the cytokines produced by DCs dictate the type of T helper cell differentiation and adaptive immune responses against tumors.Citation8,35 DCs in the TME are regulatory and they express immune regulatory molecules such as IDO, IL-10, TGF-β and RA that drive regulatory T cell responses and suppress T effector cell differentiation.Citation5,6 Our data shows that either deletion of LRP5/6 or blocking LRP5/6 signaling in DCs programmed them to an inflammatory state, resulting in increased antitumor immunity. This was due to high levels of inflammatory cytokines such as IL-6, TNF-α, IL-1β, IL-12p40 and IL-12p70 by DCs that are key in driving T effector cell differentiation and expansion. In contrast, DCs deficient in LRP5/6 expressed low levels IL-10, TGF-β1 and RA. In addition, parallel work has shown that Wnts in the TME induces IDO expression in DCs.Citation23 In settings of inflammation, Wnt-signaling in DCs acts as a counter regulatory mechanism to suppress pathological chronic inflammation and restores homeostasis.Citation13, 18, 25, 30, 35 However, we now show that in transplantable tumor settings, the same pathway in DCs drives pathological immune suppression and limits antitumor immunity. Thus, our findings show that canonical Wnt-signaling in DCs promotes immune suppression by regulating the expression of inflammatory and anti-inflammatory factors.
Our study also reveals a novel mechanism by which tumors promote immune suppression in the host. We have identified that the canonical Wnt pathway in DCs is a key regulator of immune tolerance to tumors. Disruption of this pathway altered DC function in the TME, resulting in enhanced host antitumor immune responses. These results represent an important advancement in our understanding of how Wnt-ligands in the TME can regulate host antitumor immunity and provide insight into potential immunotherapeutic strategies against tumors.
Materials and methods
Mice
C57BL/6 male mice of 6 to 12 weeks of age were purchased from The Jackson Laboratory (Bar Harbor, ME). OT-IThy1.1(Rag2−/−), OT-IIThy1.1 (Rag2−/−), LRP5 floxed,Citation36 LRP6 floxed,Citation36 LRP5/6 floxed mice,Citation14 DC-specific deletion of LRP5, LRP6 and LRP5/6 (LRP5/6ΔDC),Citation14 TCF/lymphoid enhancer factor (LEF)-reporter mice Citation37 were bred on-site. All the mice were housed under specific pathogen-free conditions in the Laboratory Animal Services of Georgia Regents University. Animal care protocols were approved by the Institutional Animal Care and Use Committee of Georgia Regents University.
Antibodies and reagents
Antibodies against mouse CD4+ (GK1.5), CD8a (53–6.7), CD45 (30-F11), Foxp3 (FJK-16s), IL-10 (JES5-16E3), CD11c (N418), CD90.1 (HIS51), Vα2 TCR (B20.1), Vβ5.1/5.2 TCR (MR9-4), IL-17 (TC11-18H10), IFNγ (XMG1.2), TNF-α (MP6-XT22), and Granzyme B (GB11) were purchased from eBioscience. Non-phospho active β-catenin antibody was obtained from Cell signaling technology. β-galactosidase (β-gal) antibody was purchased from Abcam. SKL2001, IWP-L6, C59 were obtained from Calbiochem and were dissolved in DMSO (10 mg/mL), and CFSE (Biolegend) was dissolved in DMSO (10 mM). Wnt2b, 3a, 5a, 5b, and 16b were purchased from R&D systems. OVA323–339 (ISQVHAAHAEINEAGR) peptide was purchased from Anaspec.
Tumor model and in-vivo treatment
Age-matched littermate controls were used for tumor studies. B16 melanoma cells expressing ovalbumin (B16-OVA), were provided from Dr David Munn (Georgia Regents University, Augusta, GA),Citation38 LLC cells were obtained from Dr Andrew L. Mellor (Georgia Regents University, Augusta, GA), EL4 thymoma cells expressing ovalbumin (EL4-OVA) was purchased from the American Type Culture Collection, and B16-Green Fluorescent Protein (GFP) tumor cells were established by transducing parental B16 cells with recombinant lentivector containing GFP and selection with blasticidin by Dr Yukai He's lab (Georgia Regents University, Augusta, GA). To establish tumor models, 5 × 105 MO4, EL4, LLC or B16-GFP cells were injected subcutaneously (s.c.) into mice in the shaved flank region. The tumor growth was monitored every 3 d by measuring the perpendicular diameters. The tumors were excised and the TDLNs were collected for various analyses. To study the effects of SKL2001 and IWP-L6 in vivo, tumor-transplanted C57BL/6 mice were injected intraperitoneally (i.p.) with IWP-L6 (5 mg/kg) or C59 (10 mg/kg) every 3 d on day 5, 8, 11 and 14 after tumor implantation. To investigate the functions of CD4+ and CD8+ T cells in vivo, tumor-bearing C57BL/6 mice were injected intraperitoneally (i.p.) with antibody against mouse CD4+ (500 µg/mouse) or antibody against mouse CD8+ (500 µg/mouse) on the same day of tumor inoculation and then on day 15 after tumor inoculation. In some experiments, tumor-bearing mice were administered with 4 × 106 CD8+ T cells from OT-I TCR transgenic mice or CD4+ T cells from OT-II TCR transgenic mice with or without CFSE (10 mM) labeling by intravenous (i.v.) route on day 9. Five days later, tumors and TDLNs were removed and stained with antibodies and analyzed by flow cytometry.
Cell cultures
CD11c+ splenic DCs (2 × 104) enriched from TCF/LEF-reporter mice were isolated by FACS sorting and treated with Wnt2b, Wnt3a, Wnt5a, Wnt5b, Wnt16b (500 ng/mL) for 18 h, and then the cells were collected and analyzed by flow cytometry. In some experiments, purified CD11c+ DCs (2 × 104) from LRP5/6ΔDC mice and control-FL mice were treated with Wnt2b, Wnt3a, Wnt5a, Wnt5b, Wnt16b (500ng/mL) for 10 h and washed three times with medium, followed by co-culture with naive CD25−CD4+ OT-II T cells (1 × 105) in 200 μL RPMI complete medium containing OVA peptide (2 µg/mL) and TGFβ (1 ng/mL) in 96-well round-bottomed plates. After 5 d, cells were collected and analyzed by flow cytometry.
Flow cytometry analysis
To measure cytokines, single-cell suspensions from the TDLN or tumor were ex vivo stimulated with phorbol 12-myristate 13-acetate (PMA)/Ionomycin and Brefeldin A/monensin (eBioscience) for 6 h at 37°C, and intracellular staining of IFNγ, TNFα and IL10 was performed. To measure FoxP3, Granzyme B, active β-catenin, or β-gal expression, corresponding Abs were added after permeabilization and fixation of cells.Citation19 Flow cytometric analysis was performed using a FACS LSRII system (BD Biosciences), and the data were analyzed using FlowJo software.
Real-time PCR
CD11c+ DCs purified from pooled TDLNs or tumors and Total RNA was isolated using the Omega Total RNA Kit according to the manufacturer's protocol. cDNA was generated using the RNA to cDNA Ecodry Premix Kit (Clontech) according to the manufacturer's protocol. cDNA was used as a template for quantitative real-time PCR using SYBR Green Master Mix (Roche) and gene-specific primers.Citation13,25,39 PCR analysis was performed using a MyiQ5 ICycler (BioRad). Gene expression was calculated relative to Gapdh.
Statistical analyses
Statistical analyses were performed using GraphPad Prism software. An unpaired one-tailed Student's t test was used to determine statistical significance for tumor areas, mRNA expression levels, Treg percentages, Granzyme B expression and cytokines released by various cell types between different groups. A p value less than 0.05 (*) was considered to be significant, a p value less than 0.01 (**) was considered to be very significant, and a p value less than 0.001 (***) was considered to be extremely significant.
Disclosure of potential conflicts of interest
No potential conflicts of interest were disclosed.
KONI_A_1115941_supplementary_figure_1.pdf
Download PDF (82.5 KB)Acknowledgments
We thank Dr Brat O Williams (Van Andel Research Institute, MI) for kindly providing LRP5 and LRP6 floxed mice; Jeane Silvia, Jeanene Pihkala and William King for technical help with FACS sorting and analysis; Janice Randall for technical assistance with mice used in this study, as well as our colleagues in the Georgia Regents University Cancer Immunology, Inflammation and Tolerance program for constructive comments on various aspects of this study.
Funding
This work was supported by grant to S.M. by National Institutes of Health Grant DK097271.
References
- Staal FJ, Luis TC, Tiemessen MM. WNT signalling in the immune system: WNT is spreading its wings. Nat Rev Immunol 2008; 8:581-93; PMID:18617885; http://dx.doi.org/10.1038/nri2360
- Van den Bossche J, Malissen B, Mantovani A, De Baetselier P, Van Ginderachter JA. Regulation and function of the E-cadherin/catenin complex in cells of the monocyte-macrophage lineage and DCs. Blood 2012; 119:1623-33; PMID:22174153; http://dx.doi.org/10.1182/blood-2011-10-384289
- Clevers H. Wnt/beta-catenin signaling in development and disease. Cell 2006; 127:469-80; PMID:17081971; http://dx.doi.org/10.1016/j.cell.2006.10.018
- Reya T, Clevers H. Wnt signalling in stem cells and cancer. Nature 2005; 434:843-50; PMID:15829953; http://dx.doi.org/10.1038/nature03319
- Munn DH, Mellor AL. Indoleamine 2,3-dioxygenase and tumor-induced tolerance. J Clin Invest 2007; 117:1147-54; PMID:17476344; http://dx.doi.org/10.1172/JCI31178
- Rabinovich GA, Gabrilovich D, Sotomayor EM. Immunosuppressive strategies that are mediated by tumor cells. Annu Rev Immunol 2007; 25:267-96; PMID:17134371; http://dx.doi.org/10.1146/annurev.immunol.25.022106.141609
- Palucka K, Banchereau J. Cancer immunotherapy via dendritic cells. Nat Rev Cancer 2012; 12:265-77; PMID:22437871; http://dx.doi.org/10.1038/nrc3258
- Steinman RM, Hawiger D, Nussenzweig MC. Tolerogenic dendritic cells. Annu Rev Immunol 2003; 21:685-711; PMID:12615891; http://dx.doi.org/10.1146/annurev.immunol.21.120601.141040
- Anastas JN, Moon RT. WNT signalling pathways as therapeutic targets in cancer. Nat Rev Cancer 2013; 13:11-26; PMID:23258168; http://dx.doi.org/10.1038/nrc3419
- Huang D, Du X. Crosstalk between tumor cells and microenvironment via Wnt pathway in colorectal cancer dissemination. World J Gastroenterol 2008; 14:1823-7; PMID:18350618; http://dx.doi.org/10.3748/wjg.14.1823
- Macheda ML, Stacker SA. Importance of Wnt signaling in the tumor stroma microenvironment. Curr Cancer Drug Targets 2008; 8:454-65; PMID:18781892; http://dx.doi.org/10.2174/156800908785699324
- Larue L, Delmas V. The WNT/Beta-catenin pathway in melanoma. Front Biosci 2006; 11:733-42; PMID:16146765; http://dx.doi.org/10.2741/1831
- Manicassamy S, Reizis B, Ravindran R, Nakaya H, Salazar-Gonzalez RM, Wang YC, Pulendran B. Activation of beta-catenin in dendritic cells regulates immunity versus tolerance in the intestine. Science 2010; 329:849-53; PMID:20705860; http://dx.doi.org/10.1126/science.1188510
- Suryawanshi A, Manoharan I, Hong Y, Swafford D, Majumdar T, Taketo MM, Manicassamy B, Koni PA, Thangaraju M, Sun Z et al. Canonical wnt signaling in dendritic cells regulates Th1/Th17 responses and suppresses autoimmune neuroinflammation. J Immunol 2015; 194:3295-304; PMID:25710911; http://dx.doi.org/10.4049/jimmunol.1402691
- Oderup C, LaJevic M, Butcher EC. Canonical and noncanonical Wnt proteins program dendritic cell responses for tolerance. J Immunol 2013; 190:6126-34; PMID:23677472; http://dx.doi.org/10.4049/jimmunol.1203002
- Munn DH, Sharma MD, Hou D, Baban B, Lee JR, Antonia SJ, Messina JL, Chandler P, Koni PA, Mellor AL. Expression of indoleamine 2,3-dioxygenase by plasmacytoid dendritic cells in tumor-draining lymph nodes. J Clin Invest 2004; 114:280-90; PMID:15254595; http://dx.doi.org/10.1172/JCI200421583
- Flavell RA, Sanjabi S, Wrzesinski SH, Licona-Limon P. The polarization of immune cells in the tumour environment by TGFbeta. Nat Rev Immunol 2010; 10:554-67; PMID:20616810; http://dx.doi.org/10.1038/nri2808
- Jiang A, Bloom O, Ono S, Cui W, Unternaehrer J, Jiang S, Whitney JA, Connolly J, Banchereau J, Mellman I. Disruption of E-cadherin-mediated adhesion induces a functionally distinct pathway of dendritic cell maturation. Immunity 2007; 27:610-24; PMID:17936032; http://dx.doi.org/10.1016/j.immuni.2007.08.015
- Manoharan I, Hong Y, Suryawanshi A, Angus-Hill ML, Sun Z, Mellor AL, Munn DH, Manicassamy S. TLR2-Dependent Activation of beta-Catenin Pathway in Dendritic Cells Induces Regulatory Responses and Attenuates Autoimmune Inflammation. J Immunol 2014; 193:4203-13; PMID:25210120; http://dx.doi.org/10.4049/jimmunol.1400614
- Hong Y, Manoharan I, Suryawanshi A, Majumdar T, Angus-Hill ML, Koni PA, Manicassamy B, Mellor AL, Munn DH, Manicassamy S. beta-Catenin Promotes Regulatory T-cell Responses in Tumors by Inducing Vitamin A Metabolism in Dendritic Cells. Cancer Res 2015; 75:656-65; PMID:25568183; http://dx.doi.org/10.1158/0008-5472.CAN-14-2377
- Fu C, Liang X, Cui W, Ober-Blobaum JL, Vazzana J, Shrikant PA, Lee KP, Clausen BE, Mellman I, Jiang A. beta-Catenin in dendritic cells exerts opposite functions in cross-priming and maintenance of CD8+ T cells through regulation of IL-10. Proc Natl Acad Sci U S A 2015; 112:2823-8; PMID:25730849; http://dx.doi.org/10.1073/pnas.1414167112
- Liang X, Fu C, Cui W, Ober-Blobaum JL, Zahner SP, Shrikant PA, Clausen BE, Flavell RA, Mellman I, Jiang A. beta-catenin mediates tumor-induced immunosuppression by inhibiting cross-priming of CD8(+) T cells. J Leukocyte Biol 2014; 95:179-90; PMID:24023259; http://dx.doi.org/10.1189/jlb.0613330
- Holtzhausen A, Zhao F, Evans KS, Tsutsui M, Orabona C, Tyler DS, Hanks BA. Melanoma-Derived Wnt5a Promotes Local Dendritic-Cell Expression of IDO and Immunotolerance: Opportunities for Pharmacologic Enhancement of Immunotherapy. Cancer Immunol Res 2015; 3:1082-95; PMID:26041736; http://dx.doi.org/10.1158/2326-6066.CIR-14-0167
- Manicassamy S, Pulendran B. Modulation of adaptive immunity with Toll-like receptors. Semin Immunol 2009; 21:185-93; PMID:19502082; http://dx.doi.org/10.1016/j.smim.2009.05.005
- Suryawanshi A, Manoharan I, Hong Y, Swafford D, Majumdar T, Taketo MM, Manicassamy B, Koni PA, Thangaraju M, Sun Z et al. Canonical Wnt signaling in dendritic cells regulates Th1/Th17 responses and suppresses autoimmune neuroinflammation. J Immunol 2015; 194:3295-304; PMID:25710911; http://dx.doi.org/10.3390/ijms150712928
- Shen CC, Kang YH, Zhao M, He Y, Cui DD, Fu YY, Yang LL, Gou LT. WNT16B from ovarian fibroblasts induces differentiation of regulatory T cells through beta-catenin signal in dendritic cells. Inter J Mol Sci 2014; 15:12928-39; PMID:25050785; http://dx.doi.org/10.3390/ijms150712928
- Chen DS, Mellman I. Oncology meets immunology: the cancer-immunity cycle. Immunity 2013; 39:1-10; PMID:23890059; http://dx.doi.org/10.1016/j.immuni.2013.07.012
- Huang SM, Mishina YM, Liu S, Cheung A, Stegmeier F, Michaud GA, Charlat O, Wiellette E, Zhang Y, Wiessner S et al. Tankyrase inhibition stabilizes axin and antagonizes Wnt signalling. Nature 2009; 461:614-20; PMID:19759537; http://dx.doi.org/10.1038/nature08356
- Waaler J, Machon O, Tumova L, Dinh H, Korinek V, Wilson SR, Paulsen JE, Pedersen NM, Eide TJ, Machonova O et al. A novel tankyrase inhibitor decreases canonical Wnt signaling in colon carcinoma cells and reduces tumor growth in conditional APC mutant mice. Cancer Res 2012; 72:2822-32; PMID:22440753; http://dx.doi.org/10.1158/0008-5472.CAN-11-3336
- Swafford D, Manicassamy S. Wnt signaling in dendritic cells: its role in regulation of immunity and tolerance. Discov Med 2015; 19:303-10; PMID:25977193; http://dx.doi.org/10.4049/jimmunol.1402691
- Baur AS, Lutz MB, Schierer S, Beltrame L, Theiner G, Zinser E, Ostalecki C, Heidkamp G, Haendle I, Erdmann M et al. Denileukin diftitox (ONTAK) induces a tolerogenic phenotype in dendritic cells and stimulates survival of resting Treg. Blood 2013; 122:2185-94; PMID:23958949; http://dx.doi.org/10.1182/blood-2012-09-456988
- Spranger S, Bao R, Gajewski TF. Melanoma-intrinsic beta-catenin signalling prevents anti-tumour immunity. Nature 2015; 523:231-5; PMID:25970248; http://dx.doi.org/10.1038/nature14404
- Yaguchi T, Goto Y, Kido K, Mochimaru H, Sakurai T, Tsukamoto N, Kudo-Saito C, Fujita T, Sumimoto H, Kawakami Y. Immune suppression and resistance mediated by constitutive activation of Wnt/beta-catenin signaling in human melanoma cells. J Immunol 2012; 189:2110-7; PMID:22815287; http://dx.doi.org/10.4049/jimmunol.1102282
- Joffre OP, Segura E, Savina A, Amigorena S. Cross-presentation by dendritic cells. Nat Rev Immunol 2012; 12:557-69; PMID:22790179; http://dx.doi.org/10.1038/nri3254
- Manicassamy S, Pulendran B. Dendritic cell control of tolerogenic responses. Immunol Rev 2011; 241:206-27; PMID:21488899; http://dx.doi.org/10.1111/j.1600-065X.2011.01015.x
- Zhong Z, Baker JJ, Zylstra-Diegel CR, Williams BO. Lrp5 and Lrp6 play compensatory roles in mouse intestinal development. J Cell Biochem 2012; 113:31-8; PMID:21866564; http://dx.doi.org/10.1002/jcb.23324
- Maretto S, Cordenonsi M, Dupont S, Braghetta P, Broccoli V, Hassan AB, Volpin D, Bressan GM, Piccolo S. Mapping Wnt/beta-catenin signaling during mouse development and in colorectal tumors. Proc Natl Acad Sci U S A 2003; 100:3299-304; PMID:12626757; http://dx.doi.org/10.1073/pnas.0434590100
- Sharma MD, Baban B, Chandler P, Hou DY, Singh N, Yagita H, Azuma M, Blazar BR, Mellor AL, Munn DH. Plasmacytoid dendritic cells from mouse tumor-draining lymph nodes directly activate mature Tregs via indoleamine 2,3-dioxygenase. J Clin Invest 2007; 117:2570-82; PMID:17710230; http://dx.doi.org/10.1172/JCI31911
- Manicassamy S, Ravindran R, Deng J, Oluoch H, Denning TL, Kasturi SP, Rosenthal KM, Evavold BD, Pulendran B. Toll-like receptor 2-dependent induction of vitamin A-metabolizing enzymes in dendritic cells promotes T regulatory responses and inhibits autoimmunity. Nat Med 2009; 15:401-9; PMID:19252500; http://dx.doi.org/10.1038/nm.1925