ABSTRACT
Background: Anticancer vaccines could represent a valuable complementary strategy to established therapies, especially in settings of early stage and minimal residual disease. HER-2 is an important target for immunotherapy and addressed by the monoclonal antibody trastuzumab. We have previously generated HER-2 mimotope peptides from phage display libraries. The synthesized peptides were coupled to carriers and applied for epitope-specific induction of trastuzumab-like IgG. For simplification and to avoid methodological limitations of synthesis and coupling chemistry, we herewith present a novel and optimized approach by using adeno-associated viruses (AAV) as effective and high-density mimotope-display system, which can be directly used for vaccination. Methods: An AAV capsid display library was constructed by genetically incorporating random peptides in a plasmid encoding the wild-type AAV2 capsid protein. AAV clones, expressing peptides specifically reactive to trastuzumab, were employed to immunize BALB/c mice. Antibody titers against human HER-2 were determined, and the isotype composition and functional properties of these were tested. Finally, prophylactically immunized mice were challenged with human HER-2 transfected mouse D2F2/E2 cells. Results: HER-2 mimotope AAV-vaccines induced antibodies specific to human HER-2. Two clones were selected for immunization of mice, which were subsequently grafted D2F2/E2 cells. Both mimotope AAV clones delayed the growth of tumors significantly, as compared to controls. Conclusion: In this study, a novel mimotope AAV-based platform was created allowing the isolation of mimotopes, which can be directly used as anticancer vaccines. The example of trastuzumab AAV-mimotopes demonstrates that this vaccine strategy could help to establish active immunotherapy for breast-cancer patients.
Introduction
Ever since the first promising vaccination experiments against cancer by the New York surgeon William B. Coley (1862–1936), who injected inoperable sarcoma patients with bacteria and thereby accomplished a cure rate of around 10%,Citation1 cancer research has been aiming to develop active immunotherapies against cancer. The “Coley's toxin” vaccine was evidently unspecific and clinical effects were likely due to the response of macrophages with the release of pro-inflammatory cytokines such as IL-12.Citation2 Nevertheless, such adjuvant therapies may certainly help to overcome tumor-induced immunosuppression and may initiate an active immune reaction against the malignant cells.Citation3
Current targeted anticancer immunotherapies are directed against tumor-associated antigens, which are highly overexpressed on malignant cells, but scarcely expressed in normal tissue.Citation4 Candidate targets comprise growth factor receptorsCitation5 that are important during embryonic developmentCitation6 and silenced in adults, but overexpressed again in some cancers.Citation7 The human epidermal growth factor receptor-2 (HER-2) is a ligand-less receptor tyrosine kinase typically amplified in breast, gastric and esophageal cancer.Citation8 Via homo- or hetero-dimerization with related molecules, HER-2 mediates proliferative and anti-apoptotic signals, ultimately leading to unfavorable courses of the disease.Citation9 Therefore, HER-2 belongs to the most prominent targets for specific anticancer therapies, proven many times by the clinically used anti-HER-2 monoclonal antibodies trastuzumab (Herceptin®, Roche) or pertuzumab (Perjeta®, Roche).Citation10-11 As the serum half-life of these monoclonal antibodies is around 2–4 weeks, depending on the dosageCitation12-13 (t1/2= 16,4 d for trastuzumabCitation14 and 18 d for pertuzumabCitation15), repetitive applications in tri-weekly intervals are necessary.Citation16 Even after being chimerized or humanized, these antibodies are recognized as foreign proteins by the immune system and severe side effects may occur during treatments due to hypersensitivity reactions.Citation17-20 Hence, these therapies have to be applied under strict medical supervision and often under cortisol or anti-histamine premedication,Citation21 making them laborious for patients as well as oncologists. Last but not the least, they are expensive for the health-care system and may encourage development of class-based treatment. Consequently, turning passive immunotherapies with monoclonal antibodies into active vaccines triggering the patient's own immune system to produce antibodies of the same specificity, would be highly desirable and could overcome many of the aforementioned obstacles.Citation22
However, HER-2, due to its pivotal role in ductal morphogenesis of the human mammary glandCitation23 and its involvement in repair mechanisms of myocardial cells,Citation24-25 is a self-antigen. Therefore, immunization strategies against HER-2 have to vanquish the patient's self-tolerance. Small B-cell epitope mimicking peptides, so called “mimotopes,” could be an interesting option to break tolerance, as they do not share consensus sequence with the mimicked epitopes, but possess the same structure due to similar amino acid charges.Citation26 Thus, immunization with mimotopes induces antibodies that recognize the natural antigen only via molecular mimicry. In previous studies, our group selected mimotopes from phage display libraries and demonstrated their high specificity and immunogenicity in mice.Citation26-32 As it is not possible to use filamentous phages for vaccinating patients, the mimotope peptides had to be produced synthetically and to reconstitute their immunogenicity, chemical coupling to antigenic carriers such as keyhole limpet hemocyaninCitation28 or tetanus toxoidCitation33 was necessary. During this process substantial loss of the mimicry potential of the epitope was observed.Citation26 Therefore, suitable vehicles are called for, which guarantee safe and stable display while maintaining immunogenicity. We propose in this study AAV as novel mimotope display systems, as they could be proven to induce strong humoralCitation34 and cellularCitation35 immune responses.Citation36
AAV are small (25 nm), non-enveloped single-stranded DNA virusesCitation37-38 which belong to the genus Dependovirus—they need a helper virus to facilitate their replication. Appropriate helper viruses for AAV are adenoviruses or herpes simplex virus.Citation38 As AAV are non-pathogenic, they were long-neglected in medical research,Citation38 a fact that suddenly reversed when Hermonat and Muzyczka used an AAV-based vector to express foreign genes in mammalian tissue culture cells in 1984.Citation39 Within the last 30 y, AAV were established as vectors for gene therapy and their experimental use ranges from the cardiovascular systemCitation40 over neurodegenerative disordersCitation41 to bone defects, cartilage lesions or rheumatoid arthritisCitation42 as well as infectious diseases.Citation36 In parallel, AAV or their self-assembled capsid proteins alone as virus-like particles (AAVLP) were employed as a display system for vaccines,Citation36 because the virus serotype 2 (AAV2) tolerates the insertion of small peptides into its VP3 capsid protein.Citation43
With regards to oncology, promising results for AAVLP-based vaccines were obtained especially against the tumorigenic virus Human Papilloma Virus (HPV). Nieto et al. could demonstrate that upon prophylactic vaccination with AAVLP displaying the L2 epitope of HPV, high titers of antibodies were induced in mice and rabbits, resulting in neutralization of infections with several HPV types in a pseudovirion infection assay.Citation44
Especially intriguing, however, would be a combination of the high-density display AAV system with a library allowing the selection of mimotopes from a repertoire of peptides with an antibody of interest. We addressed here whether in this way AAV mimotope vaccines could be created that at the same time would be useful as immunogen, using HER-2 as a model target.
Results
Selection of HER-2 mimotopes from an AAV library using trastuzumab
AAV-mimotope clones (schematic representation in , amino acid sequences depicted in ) were selected based on their ability to bind to the monoclonal anti-HER-2 antibody trastuzumab. Extensive description of the selection algorithm and the results can be found in the Supplementary section.
Figure 1. HER-2 mimotope AAV model and immunogenicity evaluation. (A) Model of the AAV vector displaying HER-2 specific mimotopes fused to its capsid protein VP3. Mimotopes are inserted between amino acid positions 587 and 588, which resemble a peak in the capsid, for optimal display. (B) AAV vectors displaying HER-2 mimotopes are able to induce HER-2 specific antibodies. Screening of sera from mice, immunized with AAV-displaying HER-2 mimotopes and Al(OH)3 as adjuvant, against the extracellular domain of human HER-2. Depicted are means (n = 2/mouse serum) & SD; values of MIS4 were statistically analyzed (One-way ANOVA, Tukey's post-test). (C) Antibodies induced by AAV clone DMD4 recognize HER-2 on the surface of cancer cells.Immunofluorescence staining of D2F2/E2 cells, overexpressing human HER-2 with sera of mice immunized with AAV clone DMD4 (PIS = Pre-Immune Serum, MIS 3 = MIS after three immunizations). After immunization with the HER-2 specific mimotope, membrane specific staining can be seen. Pre-Immune sera and serum of mice immunized with wtAAV alone do not show any specific staining on D2F2/E2 cells.
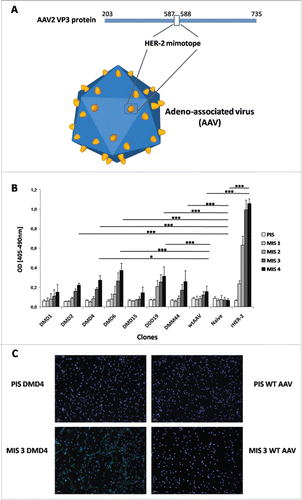
Table 1. Amino acid sequences of tested mimotopes.
Immunization with HER-2 mimotope AAV clones
AAV-mimotope clones were next selected based on their ability to induce HER-2 specific antibodies. After four rounds of immunization without any observed local or systemic side effects, HER-2 specific antibodies were detectable in sera of immunized mice, with significantly higher levels compared to naïve mice in 5 out of 7 tested mimotope groups. When compared to wtAAV immunized animals, mice from 3 mimotope groups, DMD4 (p < 0 .05), DMD6 (p < 0 .001) and DDD19 (p < 0 .001), displayed significantly higher levels of HER-2 specific IgG antibodies (). To prove the specificity of the induced antibodies, immunohistochemical stainings were performed with HER2-overexpressing and non-expressing tumor cells. As depicted in , staining with IgG from sera of immunized mice showed a membrane specific pattern in HER-2 transfected D2F2/E2 cells only, whereas the parental cell line D2F2, negative for human HER-2, remained unstained. Specificity was tested using purified IgG antibodies in ELISA against rHER-2, but also against two other known tumor-associated antigens, EGFR and CEA, or against BSA for control purposes. The HER-2 mimotope clones DMD4 and DMD6 induced specific anti-HER-2 antibodies (), which reacted significantly higher compared to antibodies purified from naive mice (p < 0 .001), or antibodies purified from the DMD1 or DMD2 groups (p < 0 .001 for both clones). Only background reactivity against control proteins sEGFR, sCEA or BSA was measured for all treatment groups ().
Figure 2. Specificity and functionality testing of antibodies purified from sera of immunized mice. (A) AAV-mimotope induced antibodies recognize HER-2, but not tumor-associated antigens EGFR, CEA or control protein BSA. Antibodies (c = 1 µg/mL) purified from sera of naïve mice and mice immunized with candidate particles were screened for their reactivity toward known tumor antigens HER-2, EGFR, CEA as well as the control protein BSA in ELISA. Antibodies induced by mimotopes DMD4 and DMD6 show significant higher IgG reactivity toward rHER-2 than naive mice (p < 0.001) and mice immunized with candidates DMD1 (p < 0 .001) and DMD2 (p < 0 .001). Toward all other antigens only background reactivity is visible. Bars represent mean values & SD (n = 2); One-way ANOVA, Tukey's post-test. (B) Purified antibodies of immunized mice to different degrees inhibit growth of human HER-2 overexpressing mammary carcinoma cells. Tetrazolium-based proliferation assays with HER-2 overexpressing BT474 cells are depicted upon incubation for 72 h with purified antibodies (c = 1 µg/mL) of sera from mice immunized with candidate particles. Bars displaying mean values & SD, analyzed by means of Kruskal–Wallis test plus Dunns post-test.
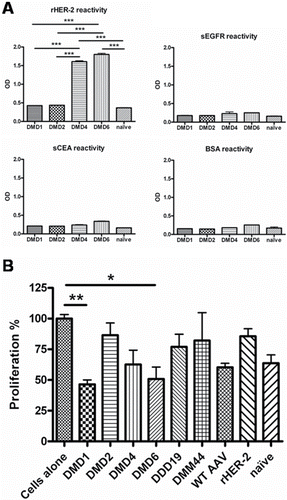
Epitope specificity is particularly essential for cancer immunotherapy, because antibodies against HER-2 can act either tumor-promoting or -inhibiting, even when directed against the same molecule.Citation26,45 Thus, the second line of screening was done by means of a tetrazolium-based cell proliferation assay to exclude mimotopes that induce antibodies either with insufficient tumoricidic effects or favoring tumor growth. Here, purified antibodies from sera of immunized mice were employed for incubation of HER-2 overexpressing BT474 cells. After 72 h, cell viability was measured (). Clones DMD1, DMD4 and DMD6 mediated growth inhibition; DMD2, DDD19 and DMM44 had only minor effects on tumor growth, but also antibodies purified from wtAAV immunized or naive mice showed tumor growth inhibition to some extent. Also antibodies induced by rHER-2, which are not restricted to the trastuzumab epitope and thus a mix of tumor-promoting and -inhibiting ones, were not able to mediate significant growth inhibition. Upon statistical evaluation, only antibodies induced by clones DMD1 and DMD6 were able to reach significance when compared to untreated cells (p < 0 .01 for DMD1 and p < 0 .05 for DMD6).
Finally, antibodies induced by clone DMD15 were tumor-promoting (). This effect, however, was drastic as BT474 cells showed 4-fold faster proliferation compared to untreated cells (p <0 .01) and underlines the importance of tumor cell proliferation inhibition assays as part of the screening procedure of novel anticancer vaccines.
Analyzing the subclass immune responses after vaccination of BALB/c mice
Clones DMD4 and DMD6 had performed best overall throughout the screening steps and were thus chosen for monitoring the IgA, IgG1, IgG2a, IgG2b and IgE responses during a further immunization experiment in BALB/c mice.
Both DMD4 and DMD6 induced negligible levels of HER-2 specific IgA (), whereas DMD4, less DMD6, induced IgG1 against HER-2 (p < 0 .05 for DMD4 compared to the naive group, ). Neither DMD4 nor DMD6 induced large extents of HER-2 specific IgG2a and IgG2b (). However, IgG2a levels in DMD4 immunized mice were significantly elevated after four rounds of immunization compared to the animals of the naive group (p < 0 .001; , right panel). Interestingly, this effect was not observed, when the DMD4 group was compared to the group immunized with wtAAV, as this group also showed elevated levels of IgG2a antibodies.
Figure 3. Subclass analysis of HER-2 specific antibodies induced by selected mimotope AAV clones DMD4 and DMD6 by ELISA. Left Panel: a-e: IgA, IgG1, IgG2a, IgG2b or IgE antibody determination before (PIS) and after each immunization (MIS 1-4). Right panel: Antibody levels after four immunization rounds (MIS 4); Box and whiskers plot, displaying minimum and maximum values. Each serum was measured in duplicate, eight mice per group ± SD; Kruskal–Wallis test, Dunns post-test.
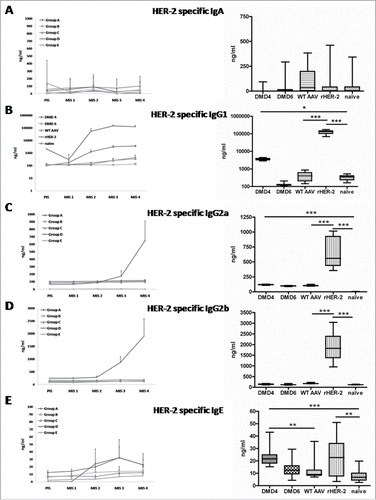
As for IgE, again DMD4 induced significant levels of HER-2 specific IgE, as compared to naive (p < 0 .001) and wtAAV immunized animals (p < 0 .01), but vaccination with DMD6 did not lead to a significant IgE anti HER-2 immune response ().
The positive control group, being vaccinated four times with the extracellular domain of HER-2, showed significant antibody levels of HER-2 specific IgG1, IgG2a, IgG2b and IgE isotypes, but not IgA ().
To determine the effect of different adjuvant agents on the elicited immune response, a cohort of BALB/c mice was immunized with the HER-2 mimotope AAV clone DMD2, alongside several different adjuvants, being either in clinical use or in clinical trials. DMD2 plus aluminum-hydroxide (Alum), monophosphoryl-Lipid A (MPL), or a combination of both, induced higher levels of IgG specific for HER-2 as compared to ODN-1826 or Alum plus ODN-1826 (Fig. S2A). This IgG response seems to be constituted predominantly by IgG1 antibodies (Fig. S2B). As for all other investigated isotypes (IgG2a, IgG2b and IgM), no differences were observable (Figs. S2C–E).
The memory effects of the mimotope-AAV vaccine were also investigated: the immune response in immunized mice remained stable until day 137 and was not dependent on the type of adjuvant used (Fig. S3).
Tumor graft trial
Immunized mice were grafted with 2 × 106 D2F2/E2 cells, overexpressing human HER-2. In all mice, tumors could be detected after 5 d. In control groups (naive mice and mice immunized with wtAAV), tumors grew exponentially. In clear contrast, the tumors in mice vaccinated with either rHER-2, or HER-2 mimotope AAV clones DMD4 or DMD6 grew significantly slower (p < 0 .01 for DMD4 compared to naive mice; p < 0 .05 compared to wtAAV treated animals; p < 0 .05 for DMD6 compared to naive as well as wtAAV immunized mice; ). The mean tumor volumes at day 12 of the trial and standard errors of the mean were as follows: DMD4 = 139, 27 mm3 ± 40, 45; DMD6 = 156, 13 mm3 ± 54, 99; wtAAV = 289, 61 mm3 ± 67, 91; rHER-2 = 124, 11 mm3 ± 51, 11 and naive mice = 309, 09 mm3 ± 128, 59, respectively.
Figure 4. Readout of tumor graft trial. (A) Mice immunized with either rHER-2, DMD4 or DMD6 particles display significantly slower and smaller tumor growth compared to naive mice and mice immunized with wtAAV only. Tumor growth curve of grafted HER-2 overexpressing D2F2/E2 cells; at day 12, DMD4 immunized mice had significantly smaller tumors compared to those of the naive (p < 0.01) and of the wtAAV group (p < 0.05). DMD6 treated mice also developed significantly smaller tumors (p < 0.05 compared to naive group, p < 0.05 compared to wtAAV). Mice immunized with rHER-2 also developed significantly smaller tumors compared to both wtAAV treated (p < 0.01) or naive mice (p < 0.01). Tumor size at day 12 was analyzed by One-way ANOVA alongside Tukey's post-test; displayed are mean tumor volume values ± standard error of the mean (SEM). (B) Representative macroscopic pictures of explanted tumors. (C) Tumors of mice immunized with mimotope DMD6 or rHER-2 had significantly lower weight compared to tumors of animals receiving wtAAV. Diagram displaying weight of explanted tumors in grams; Box and whiskers plot, whiskers displaying minimum and maximum values (eight mice/group). Analysis by means of Kruskal–Wallis test and Dunns post-test.
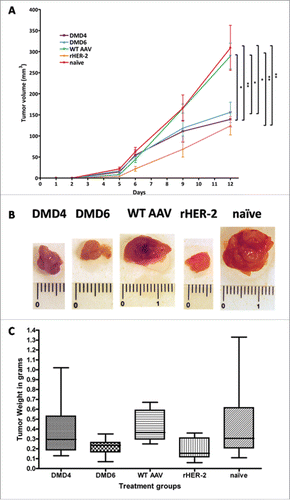
When the first mouse reached a tumor volume larger than 300 mm3, the experiment was terminated and all mice were euthanized. shows representative pictures of explanted tumors and displays a graph of the tumor weights in grams, where mice of the rHER-2 and DMD6 immunized groups presented the lowest tumor weights.
Thus, vaccination with both tested vaccines, DMD4 and DMD6, distinctly showed a protective effect in BALB/c mice, comparable to vaccination with human HER-2.
Discussion
Although HER-2 is highly successfully targeted by passive immunotherapies in clinical oncology, no active anticancer vaccine against HER-2 is in clinical use yet. However, an active anti-HER-2 vaccine could be complementary to passive immunotherapy and especially relevant in a prophylactic setting in high-risk families, in early stage and in minimal residual disease, or even to protect the next generation.Citation46 Anticancer-vaccines that lead to apoptosis of cancer cells may besides the antibody-mediated effectsCitation47 also activate the T-cellular branch via antigen crosspresentation and recruition of CD8+Citation48,49 depending on the induced isotype.Citation50
Moreover, we propose that anticancer vaccines, especially in combination with immune checkpoint-inhibitors may be another breakthrough in oncology. Overcoming the strong immunosuppressive tumor environment may also be supported by using a similar, but not identical antigen for immunization, such as a mimotope.
Mimotopes are small epitope-mimicking peptides that via molecular mimicry are able to induce epitope-specific antibodies. Hence, mimotopes for clinically approved antibodies could be effective tools to induce the desired epitope specificity in an active vaccination approach.Citation26-32,51 However, the small mimotope peptides need to be fixed to an immunogenic carrier to elicit an immune response.Citation28,33
Up to now this has been a methodological problem in mimotope vaccine production when translating phage-displayed mimotopes to a vaccine, involving peptide synthesis and chemical coupling to an immunogenic carrier. Our study presents an AAV library that enables selection of mimotope AAV clones, which can be used without further modifications as a high-density display system for vaccination (). Selections of the AAV library with trastuzumab (Herceptin®) rendered HER-2 mimotopes, which triggered antibody formation against the same epitope as trastuzumab. Seven mimotopes were used for immunizing mice and optimal candidates classified with respect to (i) antibody induction capacity (), (ii) specificity of the induced antibodies () and (iii) growth inhibitory potential when applied to HER-2 overexpressing cancer cells (). In spite of the fact that we used trastuzumab for mimotope selections, clone DMD15 induced antibodies which acted tumor-promoting (Fig. S1), underlining the importance of our screening algorithm. Based on their good performance, two candidate molecules, DMD4 and DMD6, were chosen for prophylactic vaccination in mice prior to subcutaneous tumor grafting with HER-2 overexpressing syngeneic D2F2/E2 cells. Both immunized groups showed significantly slower tumor growth compared to control groups ().
In order to elucidate the type of the induced humoral immune response, sera of mice were taken after each immunization round and screened in ELISA for HER-2 specific antibodies. As can be seen in , the immune response is mainly mediated via IgG1 antibodies and to some extent via IgE, whereas IgA and IgG2b levels were not significantly elevated. This might be explained by the fact, that BALB/c mice show a genetic bias toward Th2 immunity.Citation52-53 Independent of the employed adjuvant, ranging from the typical Th2 adjuvant Al(OH3) (Alum)Citation54 to Th1 adjuvants such as MPL Citation55 or ODN,Citation56 the immune response was stable and all adjuvants triggered mostly IgG1 antibodies (Fig. S2). However, groups receiving AAV alongside Alum or MPL (or in combination) had higher IgG levels of HER-2 specific antibodies. Based on these results, Alum, the adjuvant most used in clinical practice, was selected for all further immunizations.
Taken together, these results indicate that from our AAV library platform potent HER-2 mimotope AAV vaccines can be generated and directly used for vaccination. These HER-2 vaccines did not cause any local or systemic side effects in the immunized mice. Our observations are in line with the clinical experience from the use of AAV for gene therapy (e.g., in inherited retinal disorders and hemophilia B), where those vectors displayed favorable safety profiles (reviewed in Citation57,58). However, vaccination approaches using viral vectors are often considered dangerous due to their content of viral DNA. In this regard, AAV exhibit significant advantages, as they are non-pathogenic and inherently replication defective,Citation59 as they need concomitant helper viruses to cause an infection.Citation38 Therefore, usage of adeno-associated virus-like particles (AAVLP), consisting only of the virus capsid as display system instead of the whole virus, will enhance safety. In a previous study, AAVLP had no anaphylactogenic potency even when employed in an allergy mouse model where high levels of antibodies including IgE and IgG1 were induced.Citation60
Thus, the concept of AAVLP-based vaccines is of high interest in the field of cancer vaccines, with a great potential for prophylactic vaccination of high-risk patients, or those with minimal residual disease.
Materials and methods
Cell lines
The human BT474 cell line is a human ductal mammary carcinoma cell line, first described by E. Lasfargues and W.G. Coutinho in 1978,Citation61 distributed by ATCC (American Type Culture Collection; Cat-No: BT474 or HTB-20™, respectively), and was a kind gift of Prof Thomas Grunt from the Institute of Cancer Research of the Medical University of Vienna. BT474 cells were authenticated by short tandem repeat profiling (PowerPlex® 16 Loci Service, LGC Standards).
Mouse mammary carcinoma cells D2F2/E2 are derived from the D2F2 cell lineCitation62 by stable transfection of human HER-2Citation63 and were kindly provided by Prof Wei-Zen Wei (Karmanos Cancer Institute, Wayne State University School of Medicine, Detroit, Michigan, USA).
AAV particle production and candidate selection
Methods for production of the AAV library and selection of trastuzumab specific AAV clones are described in detail within the supplementary information. In short, an AAV capsid library was produced by transfection of HEK293T cells with a plasmid library encoding for AAV and containing a random peptide insertion of 15 amino acids with a diversity of 109 between amino acid position 587 and 588 of the capsid protein VP3 (see schematic ). Resulting AAV capsids with different peptide insertions were selected for this study based on their reactivity toward trastuzumab (for detailed information see Supplemental Materials and Results).
Animals
For immunization and tumor graft experiments, 6–8 weeks old female BALB/c mice were obtained from Charles river and kept based on authorization of the Animal Ethics Committee of the Medical University according to the Austrian, European Union and FELASA guidelines for animal care and protection (GZ: BWMF-66.009/0003-II/3b/2011).
Immunization trials
Mice were immunized in immunization experiments according to the scheme in Fig. S4A; respective groups received subcutaneously 10 µg of either AAV displaying HER-2 mimotopes or wild type AAV (wtAAV) adsorbed to 50 μL AlOH3 (Imject→ Alum Adjuvant, Pierce Protein Biology Products, Thermo Scientific; Cat-No: 77161) + 50 μL PBS. Mice were immunized four times in two-week intervals. Serum samples were taken prior to the first immunization (PIS = pre immune serum) and 10 d after each immunization round (MIS1-4 = mouse immune serum 1-4).
For evaluation of the effects of different adjuvants, only one candidate clone, DMD2, was used; the time points of immunization and serum sampling were scheduled as depicted in Fig. S4B. In this trial, the long-term effects of the AAV based mimotope vaccine were also evaluated. Hence, multiple blood samples were taken after the last round of immunization (MIS3-7) and the immune response was monitored until day 137 (Fig. S4B).
Tumor graft experiments
2 × 106 D2F2/E2 cells were grafted subcutaneously (Day 0) in immunized mice (see scheme in Fig. S4C). Mice were observed regularly for tumor growth as well as tumor-related side effects such as ceasing to ingest food or weight loss, which did not occur in a single mouse during the whole study. Tumor size was monitored by caliper measurement and tumor volume was calculated by means of the following formula: V (mm3) = d2 (mm2) × D (mm)/2, where d stands for the smallest and D for the largest diameter of the tumor. Endpoint of the tumor graft trial was defined as when the tumor of the first mouse reached 300 mm3. Then, all mice were sacrificed and tumors were taken for further analysis.
Data handling and statistics
ELISAs measuring antibody responses () and specificity of induced antibodies () were done in duplicates and data of MIS4 were analyzed by means of one-way ANOVA with Tukey's post-test. EZ4U® cell viability assays () were calculated using Kruskal–Wallis test due to the non-Gaussian distribution of the values in connection with Dunns post-test. Cell viability evaluation in Fig. S3 was analyzed using the Mann–Whitney test. Subclass analysis of induced anti-HER-2 antibodies () in MIS4 was analyzed again by Kruskal–Wallis test in connection with Dunns post-test. In the tumor graft trial, size of mouse tumors was measured by caliper () and values of day 12 were analyzed by means of one-way ANOVA with Tukey's post-test. Tumor weight () was analyzed using Kruskal–Wallis test in connection with Dunns post-test. For all experiments significance was accepted at p < 0.05 (*), p < 0.01 (**) and p < 0.001 (***). All statistic calculations were performed using GraphPad Prism 4 Software (GraphPad).
Disclosure of potential conflicts of interest
No potential conflicts of interest were disclosed.
KONI_A_1171446_s02.zip
Download Zip (1 MB)Acknowledgments
The authors would like to express their gratitude to all members of the Jensen-Jarolim lab for critical and fruitful discussions, as well as to Anna Willensdorfer and Erika Bajna for excellent technical support. Moreover, we thank Amelie Wein for proofreading our manuscript.
Funding
This work was supported by MediGene AG (Martinsried, Germany) and Biomed Int. R + D GmbH (Vienna, Austria), and in part by the Austrian Science Fund (FWF) grants P23398-B11 and W1205-B09. Erika Jensen-Jarolim is shareholder of Biomed Int. R+D GmbH. The study was funded by grants from Medigene AG to Erika Jensen-Jarolim.
References
- Wiemann B, Starnes CO. Coley's toxins, tumor necrosis factor and cancer research: a historical perspective. Pharmacol Ther 1994; 64:529-64; PMID:7724661; http://dx.doi.org/10.1016/0163-7258(94)90023-X
- Tsung K, Norton JA. Lessons from Coley's toxin. Surg Oncol 2006; 15:25-8; PMID:16814541; http://dx.doi.org/10.1016/j.suronc.2006.05.002
- Smyth MJ, Teng MW. Targeting the IL-12/IL-23 axis: An alternative approach to removing tumor induced immune suppression. Onco Immunol 2014; 3:e28964; PMID:25083324
- Scott AM, Wolchok JD, Old LJ. Antibody therapy of cancer. Nat Rev Cancer 2012; 12:278-87; PMID:22437872; http://dx.doi.org/10.1038/nrc3236
- Nguyen DM, Schrump DS. Growth factor receptors as targets for lung cancer therapy. Semin Thorac Cardiovasc Surg 2004; 16:3-12; PMID:15366682; http://dx.doi.org/10.1053/j.semtcvs.2003.12.002
- Negro A, Brar BK, Lee KF. Essential roles of Her2/erbB2 in cardiac development and function. Recent Prog Horm Res 2004; 59:1-12; PMID:14749494; http://dx.doi.org/10.1210/rp.59.1.1
- Mortus JR, Zhang Y, Hughes DP. Developmental pathways hijacked by osteosarcoma. Adv Exp Med Biol 2014; 804:93-118; PMID:24924170; http://dx.doi.org/10.1007/978-3-319-04843-7_5
- Arteaga CL, Engelman JA. ERBB receptors: from oncogene discovery to basic science to mechanism-based cancer therapeutics. Cancer Cell 2014; 25:282-303; PMID:24651011; http://dx.doi.org/10.1016/j.ccr.2014.02.025
- Bianchini G, Gianni L. The immune system and response to HER2-targeted treatment in breast cancer. Lancet Oncol 2014; 15:e58-68; PMID:24480556; http://dx.doi.org/10.1016/S1470-2045(13)70477-7
- Hudis CA. Trastuzumab–mechanism of action and use in clinical practice. N Engl J Med 2007; 357:39-51; PMID:17611206; http://dx.doi.org/10.1056/NEJMra043186
- Sabatier R, Goncalves A. Pertuzumab (Perjeta) approval in HER2-positive metastatic breast cancers. Bull Cancer 2014; 101:765-71; PMID:25091659; http://dx.doi.org/10.1684/bdc.2014.1940
- Leveque D, Gigou L, Bergerat JP. Clinical pharmacology of trastuzumab. Curr Clin Pharmacol 2008; 3:51-5; PMID:18690878; http://dx.doi.org/10.2174/157488408783329931
- Tabrizi MA, Tseng CM, Roskos LK. Elimination mechanisms of therapeutic monoclonal antibodies. Drug Discov Today 2006; 11:81-8; PMID:16478695; http://dx.doi.org/10.1016/S1359-6446(05)03638-X
- Baselga J, Carbonell X, Castaneda-Soto NJ, Clemens M, Green M, Harvey V, Morales S, Barton C, Ghahramani P. Phase II study of efficacy, safety, and pharmacokinetics of trastuzumab monotherapy administered on a 3-weekly schedule. J Clin Oncol 2005; 23:2162-71; PMID:15800309; http://dx.doi.org/10.1200/JCO.2005.01.014
- Garg A, Quartino A, Li J, Jin J, Wada DR, Li H, Cortés J, McNally V, Ross G, Visich J, Lum B. Population pharmacokinetic and covariate analysis of pertuzumab, a HER2-targeted monoclonal antibody, and evaluation of a fixed, non-weight-based dose in patients with a variety of solid tumors. Cancer Chemother Pharmacol 2014; 74:819-29; PMID:25119184; http://dx.doi.org/10.1007/s00280-014-2560-3
- Leyland-Jones B. Dose scheduling–Herceptin. Oncology 2001; 61 Suppl 2:31-6; PMID:11694785; http://dx.doi.org/10.1159/000055399
- Chung CH, Mirakhur B, Chan E, Le QT, Berlin J, Morse M, Murphy BA, Satinover SM, Hosen J, Mauro D et al. Cetuximab-induced anaphylaxis and IgE specific for galactose-α-1,3-galactose. N Engl J Med 2008; 358:1109-17; PMID:18337601; http://dx.doi.org/10.1056/NEJMoa074943
- Maier S, Chung CH, Morse M, Platts-Mills T, Townes L, Mukhopadhyay P, Bhagavatheeswaran P, Racenberg J, Trifan OC. A retrospective analysis of cross-reacting cetuximab IgE antibody and its association with severe infusion reactions. Cancer Med 2014; 4(1):36-42; PMID:25296628; http://dx.doi.org/10.1002/cam4.333. Epub 2014 Oct 9
- Hsu Blatman KS, Castells MC. Desensitizations for chemotherapy and monoclonal antibodies: indications and outcomes. Curr Allergy Asthma Rep 2014; 14:453; PMID:24994467; http://dx.doi.org/10.1007/s11882-014-0453-5
- Castells M, Sancho-Serra Mdel C, Simarro M. Hypersensitivity to antineoplastic agents: mechanisms and treatment with rapid desensitization. Cancer Immunol Immunother 2012; 61:1575-84; PMID:22576054; http://dx.doi.org/10.1007/s00262-012-1273-x
- Vultaggio A, Castells MC. Hypersensitivity reactions to biologic agents. Immunol Allergy Clin North Am 2014; 34:615-32, ix; PMID:25017680; http://dx.doi.org/10.1016/j.iac.2014.04.008
- Jensen-Jarolim E, Singer J. Cancer vaccines inducing antibody production: more pros than cons. Expert Rev Vaccines 2011; 10:1281-9; PMID:21919618; http://dx.doi.org/10.1586/erv.11.105
- Sternlicht MD. Key stages in mammary gland development: the cues that regulate ductal branching morphogenesis. Breast Cancer Res 2006; 8:201; PMID:16524451; http://dx.doi.org/10.1186/bcr1368
- Kuramochi Y, Guo X, Sawyer DB. Neuregulin activates erbB2-dependent src/FAK signaling and cytoskeletal remodeling in isolated adult rat cardiac myocytes. J Mol Cell Cardiol 2006; 41:228-35; PMID:16769082; http://dx.doi.org/10.1016/j.yjmcc.2006.04.007
- Wadugu B, Kuhn B. The role of neuregulin/ErbB2/ErbB4 signaling in the heart with special focus on effects on cardiomyocyte proliferation. Am J Physiol Heart Circ Physiol 2012; 302:H2139-47; PMID:22427524; http://dx.doi.org/10.1152/ajpheart.00063.2012
- Knittelfelder R, Riemer AB, Jensen-Jarolim E. Mimotope vaccination–from allergy to cancer. Expert Opin Biol Ther 2009; 9:493-506; PMID:19344285; http://dx.doi.org/10.1517/14712590902870386
- Riemer AB, Hantusch B, Sponer B, Kraml G, Hafner C, Zielinski CC, Scheiner O, Pehamberger H, Jensen-Jarolim E. High-molecular-weight melanoma-associated antigen mimotope immunizations induce antibodies recognizing melanoma cells. Cancer Immunol Immunother 2005; 54:677-84; PMID:15565329; http://dx.doi.org/10.1007/s00262-004-0632-7
- Riemer AB, Kurz H, Klinger M, Scheiner O, Zielinski CC, Jensen-Jarolim E. Vaccination with cetuximab mimotopes and biological properties of induced anti-epidermal growth factor receptor antibodies. J Natl Cancer Inst 2005; 97:1663-70; PMID:16288119; http://dx.doi.org/10.1093/jnci/dji373
- Riemer AB, Forster-Waldl E, Bramswig KH, Pollak A, Zielinski CC, Pehamberger H, Lode HN, Scheiner O, Jensen-Jarolim E. Induction of IgG antibodies against the GD2 carbohydrate tumor antigen by vaccination with peptide mimotopes. Eur J Immunol 2006; 36:1267-74; PMID:16568495; http://dx.doi.org/10.1002/eji.200535279
- Bramswig KH, Knittelfelder R, Gruber S, Untersmayr E, Riemer AB, Szalai K, Horvat R, Kammerer R, Zimmermann W, Zielinski CC et al. Immunization with mimotopes prevents growth of carcinoembryonic antigen positive tumors in BALB/c mice. Clin Cancer Res 2007; 13:6501-8; PMID:17975163; http://dx.doi.org/10.1158/1078-0432.CCR-07-0692
- Riemer AB, Jensen-Jarolim E. Mimotope vaccines: epitope mimics induce anti-cancer antibodies. Immunol Lett 2007; 113:1-5; PMID:17825923; http://dx.doi.org/10.1016/j.imlet.2007.07.008
- Riemer AB, Untersmayr E, Knittelfelder R, Duschl A, Pehamberger H, Zielinski CC, Scheiner O, Jensen-Jarolim E. Active induction of tumor-specific IgE antibodies by oral mimotope vaccination. Cancer Res 2007; 67:3406-11; PMID:17409451; http://dx.doi.org/10.1158/0008-5472.CAN-06-3758
- Riemer AB, Klinger M, Wagner S, Bernhaus A, Mazzucchelli L, Pehamberger H, Scheiner O, Zielinski CC, Jensen-Jarolim E. Generation of Peptide mimics of the epitope recognized by trastuzumab on the oncogenic protein Her-2/neu. J Immunol 2004; 173:394-401; PMID:15210798; http://dx.doi.org/10.4049/jimmunol.173.1.394
- Li X, Cao H, Wang Q, Di B, Wang M, Lu J, Pan L, Yang L, Mei M, Pan X et al. Novel AAV-based genetic vaccines encoding truncated dengue virus envelope proteins elicit humoral immune responses in mice. Microbes Infect 2012; 14:1000-7; PMID:22626929; http://dx.doi.org/10.1016/j.micinf.2012.05.002
- Sipo I, Knauf M, Fechner H, Poller W, Planz O, Kurth R, Norley S. Vaccine protection against lethal homologous and heterologous challenge using recombinant AAV vectors expressing codon-optimized genes from pandemic swine origin influenza virus (SOIV). Vaccine 2011; 29:1690-9; PMID:21195079; http://dx.doi.org/10.1016/j.vaccine.2010.12.037
- Nieto K, Salvetti A. AAV Vectors Vaccines Against Infectious Diseases. Front Immunol 2014; 5:5; PMID:24478774; http://dx.doi.org/10.3389/fimmu.2014.00005
- McCarty DM. Self-complementary AAV vectors; advances and applications. Mol Ther 2008; 16:1648-56; PMID:18682697; http://dx.doi.org/10.1038/mt.2008.171
- Daya S, Berns KI. Gene therapy using adeno-associated virus vectors. Clin Microbiol Rev 2008; 21:583-93; PMID:18854481; http://dx.doi.org/10.1128/CMR.00008-08
- Hermonat PL, Muzyczka N. Use of adeno-associated virus as a mammalian DNA cloning vector: transduction of neomycin resistance into mammalian tissue culture cells. Proc Natl Acad Sci USA 1984; 81:6466-70; PMID:6093102; http://dx.doi.org/10.1073/pnas.81.20.6466
- Zacchigna S, Zentilin L, Giacca M. Adeno-associated virus vectors as therapeutic and investigational tools in the cardiovascular system. Circ Res 2014; 114:1827-46; PMID:24855205; http://dx.doi.org/10.1161/CIRCRESAHA.114.302331
- Bourdenx M, Dutheil N, Bezard E, Dehay B. Systemic gene delivery to the central nervous system using Adeno-associated virus. Front Mol Neurosci 2014; 7:50; PMID:24917785; http://dx.doi.org/10.3389/fnmol.2014.00050
- Dai J, Rabie AB. The use of recombinant adeno-associated virus for skeletal gene therapy. Orthod Craniofac Res 2007; 10:1-14; PMID:17284242; http://dx.doi.org/10.1111/j.1601-6343.2007.00381.x
- Muzyczka N, Warrington KH, Jr. Custom adeno-associated virus capsids: the next generation of recombinant vectors with novel tropism. Hum Gene Ther 2005; 16:408-16; PMID:15871672; http://dx.doi.org/10.1089/hum.2005.16.408
- Nieto K, Weghofer M, Sehr P, Ritter M, Sedlmeier S, Karanam B, Seitz H, Müller M, Kellner M, Hörer M et al. Development of AAVLP(HPV16/31L2) particles as broadly protective HPV vaccine candidate. PLoS One 2012; 7:e39741; PMID:22761884; http://dx.doi.org/10.1371/journal.pone.0039741
- Chang C, Takayanagi A, Yoshida T, Shimizu N. Recombinant human IgG antibodies recognizing distinct extracellular domains of EGF receptor exhibit different degrees of growth inhibitory effects on human A431 cancer cells. Exp Cell Res 2013; 319:1146-55; PMID:23499740; http://dx.doi.org/10.1016/j.yexcr.2013.03.002
- Barutello G, Curcio C, Spadaro M, Arigoni M, Trovato R, Bolli E, Zheng Y, Ria F, Quaglino E, Iezzi M et al. Antitumor immunization of mothers delays tumor development in cancer-prone offspring. Onco Immunol 2015; 4:e1005500; PMID:26155401; http://dx.doi.org/10.1080/2162402X.2015.1005500
- Michaud HA, Eliaou JF, Lafont V, Bonnefoy N, Gros L. Tumor antigen-targeting monoclonal antibody-based immunotherapy: Orchestrating combined strategies for the development of long-term antitumor immunity. Onco Immunol 2014; 3:e955684
- Spel L, Boelens JJ, Nierkens S, Boes M. Antitumor immune responses mediated by dendritic cells: How signals derived from dying cancer cells drive antigen cross-presentation. Onco Immunol 2013; 2:e26403; PMID:24482744; http://dx.doi.org/10.4161/onci.26403
- Buonaguro L, Tagliamonte M, Visciano ML, Tornesello ML, Buonaguro FM. Developments in virus-like particle-based vaccines for HIV. Expert Rev Vaccines 2013; 12:119-27; PMID:23414404; http://dx.doi.org/10.1586/erv.12.152
- Platzer B, Elpek KG, Cremasco V, Baker K, Stout MM, Schultz C, Dehlink E, Shade KT, Anthony RM, Blumberg RS et al. IgE/FcepsilonRI-mediated antigen cross-presentation by dendritic cells enhances anti-tumor immune responses. Cell Rep 2015; S2211-1247(15):00143-6; PMID:25753415; http://dx.doi.org/10.1016/j.celrep.2015.02.015
- Hafner C, Wagner S, Allwardt D, Riemer AB, Scheiner O, Pehamberger H, Breiteneder H. Cross-reactivity of mimotopes with a monoclonal antibody against the high molecular weight melanoma-associated antigen (HMW-MAA) does not predict cross-reactive immunogenicity. Melanoma Res 2005; 15:111-7; PMID:15846144; http://dx.doi.org/10.1097/00008390-200504000-00005
- Schulte S, Sukhova GK, Libby P. Genetically programmed biases in Th1 and Th2 immune responses modulate atherogenesis. Am J Pathol 2008; 172:1500-8; PMID:18467709; http://dx.doi.org/10.2353/ajpath.2008.070776
- Fukushima A, Yamaguchi T, Ishida W, Fukata K, Taniguchi T, Liu FT, Ueno H. Genetic background determines susceptibility to experimental immune-mediated blepharoconjunctivitis: comparison of Balb/c and C57BL/6 mice. Exp Eye Res 2006; 82:210-8; PMID:16102751; http://dx.doi.org/10.1016/j.exer.2005.06.010
- Petrovsky N, Aguilar JC. Vaccine adjuvants: current state and future trends. Immunol Cell Biol 2004; 82:488-96; PMID:15479434; http://dx.doi.org/10.1111/j.0818-9641.2004.01272.x
- Wheeler AW, Marshall JS, Ulrich JT. A Th1-inducing adjuvant, MPL, enhances antibody profiles in experimental animals suggesting it has the potential to improve the efficacy of allergy vaccines. Int Arch Allergy Immunol 2001; 126:135-9; PMID:11729351; http://dx.doi.org/10.1159/000049504
- Zimmermann S, Egeter O, Hausmann S, Lipford GB, Rocken M, Wagner H, Heeg K. CpG oligodeoxynucleotides trigger protective and curative Th1 responses in lethal murine leishmaniasis. J Immunol 1998; 160:3627-30; PMID:9558060
- Mingozzi F, High KA. Therapeutic in vivo gene transfer for genetic disease using AAV: progress and challenges. Nat Rev Genet 2011; 12:341-55; PMID:21499295; http://dx.doi.org/10.1038/nrg2988
- Dismuke DJ, Tenenbaum L, Samulski RJ. Biosafety of recombinant adeno-associated virus vectors. Curr Gene Ther 2013; 13:434-52; PMID:24195602; http://dx.doi.org/10.2174/15665232113136660007
- Flotte TR, Berns KI. Adeno-associated virus: a ubiquitous commensal of mammals. Hum Gene Ther 2005; 16:401-7; PMID:15871671; http://dx.doi.org/10.1089/hum.2005.16.401
- Manzano-Szalai K, Thell K, Willensdorfer A, Weghofer M, Pfanzagl B, Singer J, Ritter M, Stremnitzer C, Flaschberger I, Michaelis U et al. Adeno-associated virus-like particles as new carriers for B-cell vaccines: testing immunogenicity and safety in BALB/c mice. Viral Immunol 2014; 27:438-48; PMID:25247267; http://dx.doi.org/10.1089/vim.2014.0059
- Lasfargues EY, Coutinho WG, Redfield ES. Isolation of two human tumor epithelial cell lines from solid breast carcinomas. J Natl Cancer Inst 1978; 61:967-78; PMID:212572
- Mahoney KH, Miller BE, Heppner GH. FACS quantitation of leucine aminopeptidase and acid phosphatase on tumor-associated macrophages from metastatic and nonmetastatic mouse mammary tumors. J Leukoc Biol 1985; 38:573-85; PMID:2413152
- Wei WZ, Shi WP, Galy A, Lichlyter D, Hernandez S, Groner B, Heilbrun L, Jones RF. Protection against mammary tumor growth by vaccination with full-length, modified human ErbB-2 DNA. Int J Cancer 1999; 81:748-54; PMID:10328228; http://dx.doi.org/10.1002/(SICI)1097-0215(19990531)81:5<748::AID-IJC14>3.0.CO;2-6
- Cho HS, Mason K, Ramyar KX, Stanley AM, Gabelli SB, Denney DW, Jr, Leahy DJ. Structure of the extracellular region of HER2 alone and in complex with the Herceptin Fab. Nature 2003; 421:756-60; PMID:12610629; http://dx.doi.org/10.1038/nature01392
- Veldwijk MR, Topaly J, Laufs S, Hengge UR, Wenz F, Zeller WJ, Fruehauf S. Development and optimization of a real-time quantitative PCR-based method for the titration of AAV-2 vector stocks. Mol Ther 2002; 6:272-8; PMID:12349826; http://dx.doi.org/10.1006/mthe.2002.0659
- Wu P, Xiao W, Conlon T, Hughes J, Agbandje-McKenna M, Ferkol T, Flotte T, Muzyczka N. Mutational analysis of the adeno-associated virus type 2 (AAV2) capsid gene and construction of AAV2 vectors with altered tropism. J Virol 2000; 74:8635-47; PMID:10954565; http://dx.doi.org/10.1128/JVI.74.18.8635-8647.2000
- Kneitz RH, Schubert J, Tollmann F, Zens W, Hedman K, Weissbrich B. A new method for determination of varicella-zoster virus immunoglobulin G avidity in serum and cerebrospinal fluid. BMC Infect Dis 2004; 4:33; PMID:15355548; http://dx.doi.org/10.1186/1471-2334-4-33
- Horer M, Hallek M. Method for producing a recombinant adeno-associated virus, suitable means therefor and use for producing a medicament. Google Patents 2005.
- Buening H, Nieland J, Perabo L, Goldnau D, Lux K, Hallek M et al. Mutated parvovirus structural proteins as vaccines. Google Patents 2008.
- Corpet F. Multiple sequence alignment with hierarchical clustering. Nucleic Acids Res 1988; 16:10881-90; PMID:2849754; http://dx.doi.org/10.1093/nar/16.22.10881