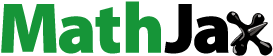
ABSTRACT
Immunotherapy is currently investigated as treatment option in many types of cancer. So far, results from clinical trials have demonstrated that significant benefit from immunomodulatory therapies is restricted to patients with select histologies. To broaden the potential use of these therapies, a deeper understanding for mechanisms of immunosuppression in patients with cancer is needed. Soft-tissue sarcoma (STS) presents a medical challenge with significant mortality even after multimodal treatment. We investigated function and immunophenotype of peripheral natural killer (NK) cells from chemotherapy-naive STS patients (1st line) and STS patients with progression or relapse after previous chemotherapeutic treatment (2nd line). We found NK cells from peripheral blood of both STS patient cohorts to be dysfunctional, being unable to lyse K562 target cells while NK cells from renal cell cancer (RCC) patients did not display attenuated lytic activity. Ex vivo stimulation of NK cells from STS patients with interleukin-2 plus TKD restored cytotoxic function. Furthermore, altered NK cell subset composition with reduced proportions of CD56dim cells could be demonstrated, increasing from 1st- to 2nd-line patients. 2nd-line patients additionally displayed significantly reduced expression of receptors (NKG2D), mediators (CD3ζ), and effectors (perforin) of NK cell activation. In these patients, we also detected fewer NK cells with CD57 expression, a marker for terminally differentiated cytotoxic NK cells. Our results elucidate mechanisms of NK cell dysfunction in STS patients with advanced disease. Markers like NKG2D, CD3ζ, and perforin are candidates to characterize NK cells with effective antitumor function for immunotherapeutic interventions.
Abbreviations
7-AAD | = | 7-Aminoactinomycin |
CLL | = | chronic lymphatic leukemia |
GIST | = | gastrointestinal stromal tumor |
HD | = | healthy donor |
HLA | = | human leukocyte antigen |
Hsp70 | = | heat shock protein 70 |
IL-2 | = | interleukin-2 |
KIR | = | killer cell immunoglobulin-like receptor |
MCA | = | 3-methylcholanthrene |
MHC | = | major histocompatibility complex |
NK cell | = | natural killer cell |
NKG2D | = | natural-killer group 2, member D receptor |
PBMC | = | peripheral blood mononuclear cells |
RCC | = | renal cell carcinoma |
STS | = | soft-tissue sarcoma |
TKD | = | 14 amino acid derivative of heat shock protein 70 |
TKI | = | tyrosine-kinase inhibitor |
Introduction
Natural killer (NK) cells are lymphocytes with the ability to lyse virus-infected and neoplastic cells.Citation1 To distinguish infected or transformed cells from healthy tissues, NK cells utilize a multitude of receptors to establish a complex balance of activating and inhibitory signals.Citation2 Stressed or transformed cells express antigens that are usually not found on healthy tissues. Activating NK cell receptors like NKG2D bind these antigens and subsequently shift the balance into the activating direction.Citation3 In contrast, killer cell immunoglobulin-like receptors (KIRs) bind human leukocyte antigen (HLA) molecules, expressed on all healthy cells, and mediate inhibitory signals.Citation4 With their ability to recognize and lyse transformed cells, NK cells can play an important role in the immunosurveillance of cancer, and are currently investigated for use in immunotherapy.Citation5 Subjects with reduced NK cell-specific cytotoxicity have been shown to have a higher incidence of malignant diseases in a prospective longitudinal study,Citation6 and familial cancer has been associated with reduced NK cell cytotoxicity.Citation7 Moreover, patients with newly diagnosed cancer commonly display alterations of the cytotoxic capacity of NK cells.Citation8-11 The underlying mechanisms of NK cell dysfunction are still incompletely understood, but downregulation of activating NK cell receptors can often be found on NK cells of patients suffering from neoplastic disease.Citation12-14 So far, it has not been possible to differentiate if the cancer itself causes these alterations, or if the neoplastic disease is the result of an impaired NK cell cytotoxicity. In established human tumors, NK cell infiltrates often are non-existent, or NK cells isolated from tumor tissue show signs of NK cell dysfunction.Citation15,16 Numerous reports have shown that insufficient recognition of tumors by NK cells, e.g., by disrupting the function of activating NK cell receptors, enables tumor growth and dissemination.Citation17 Re-establishing sufficient lytic activity of NK cells might be a promising therapeutic approach for patients suffering from cancer.
Soft-tissue sarcomas (STS) are a group of heterogeneous and rare malignant tumors that arise in any of the mesodermal tissues of the body. Currently, more than 50 different histologic subtypes of STS have been described. Standard of care for high-risk patients (with large tumors and deep location) is a multidisciplinary approach including surgery with sufficient resection margins and—when possible—radiation.Citation18 Chemotherapy can be applied in a neoadjuvant or adjuvant setting for locally advanced disease as part of a multimodal therapy, and is the mainstay of therapy for patients with metastatic disease.Citation19-21 Despite comprehensive efforts to identify new agents that are efficient in the treatment of STS patients,Citation22 however, relapse of disease even after wide resection of the tumor is common. Ultimately, approximately 40% of the patients still die from STS,Citation23 illustrating the urgent medical need for new therapeutic options. Mouse models have demonstrated that NK cells are involved in the elimination of 3-methylcholanthrene (MCA)-induced STS.Citation24,25 Augmentation of NK cell cytotoxicity might, therefore, be beneficial for STS patients. However, NK cell function of patients with STS has not been characterized yet.
Here, we report a significant decrease in NK cell-specific cytotoxicity for PBMCs of chemotherapy-naive STS patients, referred to as 1st-line patients and STS patients with progression or relapse after previous chemotherapeutic treatment, referred to as 2nd-line patients. To characterize the underlying mechanisms of NK cell dysfunction, we analyzed NK cell subsets and the expression of activating NK cell receptors important for the initiation of cytotoxicity. We found significant alterations in NK cell subset proportions and in the expression of activating receptors and differentiation markers of 2nd-line STS patients. Apparently, the observed deviations were specific for STS patients since NK cells of patients with renal cell carcinoma (RCC) did not show these alterations and displayed features similar to NK cells from healthy donors (HD). Incubation with interleukin-2 plus TKD (a 14 amino acid derivative of heat shock protein 70) ex vivo was able to reverse NK cell dysfunction and might increase the efficacy of immunotherapeutic regimens in STS patients.
Results
Cytotoxicity of peripheral NK cells is impaired in STS patients but can be restored by ex vivo stimulation with IL-2
NK cells can recognize and kill tumor cells, and impaired NK cell cytotoxicity may be a means of immune escape. We evaluated the NK cell-specific cytotoxicity of PBMCs from patients with STS and RCC by chromium release assay using K562 cells as NK cell-specific targets.Citation26 We observed a significantly lower NK cell-specific cytotoxic capacity for PBMCs from STS patients (), reduced to approximately 1/5 of the activity of PBMCs of HD, whereas PBMCs from RCC patients showed NK cell-specific lysis of target cells comparable with PBMCs of HD.
Figure 1. Peripheral NK cells of patients with STS are less cytotoxic than NK cells of RCC patients and HD, but regain cytotoxicity after incubation with IL-2/TKD. NK-specific cytotoxicity against radiolabeled K562 target cells was assessed by 4 h 51Cr release assay. Displayed are the specific lysis values of PBMC/K562 at a ratio of 20:1. (A) NK-specific cytotoxicity of HD (n = 32), STS (n = 24), and RCC patients (n = 4). (B) NK-specific cytotoxicity of STS patients without previous chemotherapy (1st-line, n = 13) compared with STS patients with prior chemotherapy (2nd-line, n = 11). (C) Left panel: NK-specific cytotoxicity of HD (n = 10) and STS patients (1st-line n = 6, 2nd-line n = 6), assessed before and after 96 h of incubation in medium containing interleukin-2 (IL-2) and 14-mer heat shock protein 70 (hsp70) peptide TKD. Only paired samples are shown. Right panel: Normalized data of left panel; relative increase in cytotoxicity for HD, 1st- and 2nd-line STS patients after 96 h of incubation with IL-2 and TKD. For A, B, and C (left panel), box plots represent the median, .75 and .25 percentiles, with whiskers showing minimum and maximum values. Each symbol corresponds to one sample. For C (right panel), mean values with standard errors are shown. For statistical analyses, Kruskal–Wallis tests with Dunn's post hoc tests (A), Mann–Whitney U test (B) and two-way ANOVA with Bonferroni's post hoc test (C) were used.
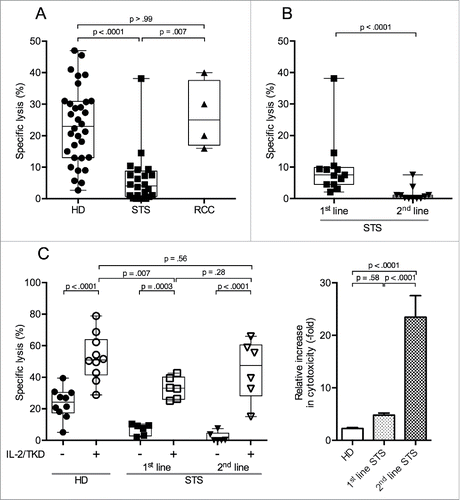
Our cohort of STS patients consisted of two different subgroups. The first subgroup encompassed patients who had been diagnosed with STS within weeks before inclusion in our analyses (n = 13). All patients in this group had locally advanced disease but no documented metastases. None of them had received any tumor-specific medication (chemotherapy) before blood withdrawal. Based on these criteria, patients in this STS subgroup were termed 1st-line patients ().
Table 1. Characteristics of STS patients. Median age of 1st-line patients was 44 y, of 2nd-line patients was 34 y. All 2nd-line patients had been treated with anthracyclines before. Months since initial diagnosis indicates the time interval to blood sample withdrawal. TNM stage indicates tumor stage, assessed by CT/MRI imaging, at time of blood withdrawal, with resection status in brackets. Months since last cytostatic treatment indicates the time interval from last application of chemotherapeutic agents to blood sample withdrawal.
The second subgroup, termed 2nd-line patients (n = 11), included STS patients who had received chemotherapy before inclusion in our analyses. All had progressive disease after cytostatic treatment, whereby progression did not necessarily occur during chemotherapeutic treatment, but might have also emerged after the completion of (e.g., adjuvant) chemotherapeutic treatment. Thus, time intervals since last cytostatic drug treatment varied between patients. Two thirds of the 2nd-line patients had metastatic disease at the time of blood withdrawal, and one third had local progression without documented metastases ().
A comparison of the cytotoxicity of PBMCs of 1st- and 2nd-line STS patients revealed that 2nd-line patients had almost undetectable lytic activity (median 0.6%), whereas PBMCs of 1st-line patients displayed a significantly higher NK cell-specific cytotoxicity (median 7.5%, ), which, however, still was significantly below the NK cell-specific cytotoxic capacity of PBMCs of HD and RCC (p = .001 and .03, respectively; data not shown).
For 2nd-line patients, median time interval since last chemotherapeutic treatment was 5 mo, with a minimum of 4 weeks. There was neither a significant correlation between the time since last cytotoxic treatment and NK cell-specific cytotoxicity (Pearson's r = .31; p = .34, data not shown) for 2nd-line patients, nor was the NK cell-specific cytotoxicity significantly different between patients who had their blood withdrawn within 5 mo of last chemotherapy (median time interval to last treatment) and patients with longer time intervals to last chemotherapeutic treatment (Fig. S1A). 2nd-line patients with metastatic disease had a lower NK cell-specific cytotoxicity than 2nd-line patients with non-metastatic, e.g., locally relapsed or progressive disease (Fig. S1B). However, the lytic activity of PBMCs from non-metastatic 2nd-line patients still was lower than that of PBMCs of (all non-metastatic) 1st-line patients (Fig. S1C).
Interleukin-2 (IL-2) is a strong activator of NK cell cytotoxicity. TKD can further augment the stimulatory capacity of IL-2.Citation27 We evaluated whether the suppressed NK cell function of STS patients could be restored by stimulation with IL-2/TKD. This experiment employed select samples obtained from STS patients and HD analyzed above. Levels of NK cell-specific cytotoxicity from unstimulated PBMCs of these samples were representative for the respective group. We found that cultivation of PBMCs with IL-2/TKD significantly increased NK cell-specific cytotoxicity in all three groups, with PBMCs of 2nd-line STS patients reaching lytic activities comparable to activated PBMCs of HD (median 47.5% and 51.0%, respectively). PBMCs of 1st-line STS patients also responded to IL-2/TKD stimulation, but the increase in NK cell-specific cytotoxicity remained below that of IL-2/TKD-stimulated PBMCs of HD (median 33.0%, , left panel). When cytotoxicity after stimulation was normalized to values of the uncultured samples, the relative increase upon IL-2/TKD stimulation was 2-, 5-, and 23-fold for PBMCs from HD, 1st-line and 2nd-line STS patients, respectively. Thus, 2nd-line patients showed a significantly higher increase compared with both HD and 1st-line STS patients (, right panel), and stimulation with IL-2/TKD could reinstate cytotoxic capacity in PBMCs from patients with STS, even with severely suppressed cytotoxicity in 2nd-line patients.
NK cell frequencies and NK cell subset distributions are altered in STS patients
Polychromatic flow cytometry was performed to identify mechanisms that might explain the observed impairment in NK cell-mediated cytotoxicity.
NK cells were identified as CD3−CD56+ cells among live single PBMCs (Fig. S2A), and the two main NK cell subsets were distinguished based on the intensity of their CD56 expression ( and Fig. S2B). As commonly observed for PBMCs of HD, the frequency of NK cells (CD3−CD56+) in our HD collective ranged from 4.6% to 32.0% (mean 13.7%) with 13.0% belonging to the CD56dim subset and 0.7% being CD56bright. Regarding the RCC samples, the overall frequency and the subset distribution was not significantly different from the HD samples. However, in PBMCs of STS patients, the NK cell frequency was lower compared with HD and RCC reaching significance in 2nd-line PBMCs. Notably, it was the CD56dim NK cell subset that was significantly reduced in 2nd-line STS patients (mean 7.1% and 2.1% in 1st- and 2nd-line STS patients, respectively, vs. 13.0% in HD and 10.1% in RCC patients) while the fraction of CD56bright NK cells was comparable between all patient and donor groups. The CD56bright and CD56dim NK cell subsets were further subgrouped with respect to their expression level of CD16, distinguishing three groups, CD16+, CD16low, and CD16− ( and Fig. S2B). Among the CD56dim NK cells of HD, CD16+ cells were the most frequent subset while CD16− were sparse. A comparison of the CD16 distribution in CD56dim NK cells of HD, 1st-line and 2nd-line STS patients revealed that the fraction of CD16+ cells within the CD56dim NK cells was lower in STS patients than in HD with a progressive decrease from 1st-line to 2nd-line STS patients (median of 82.9% in HD, 80.8% in 1st line and 31.6% in 2nd-line STS) (). In accordance with the loss of CD16+ NK cells in the CD56dim subset there was a gradual gain in the CD16low (median 15.3% in HD, 17.5% in 1st line and 65.2% in 2nd-line STS) and CD16− (median 3.0% in HD, 3.0% in 1st line and 4.6% in 2nd-line STS) populations of CD56dim NK cells. Within the CD56bright NK cells, no significant differences were observed between 1st- and 2nd-line STS patients and HD ().
Figure 2. Percentage of CD56dim NK cells among PBMCs is reduced and CD16 expression on CD56dim NK cells is diminished in 2nd-line STS patients. (A) Relative distributions of NK cells and NK cell subsets (CD56dim and CD56bright) among PBMCs were assessed by polychromatic flow cytometry. Mean values with standard errors of indicated PBMC subtype cells among live, single, small lymphocytes (FSC/SSC) are shown. Asterisks indicate p values resulting from comparisons of total NK cells (CD3−CD56+ cells) and the CD56dim NK cell subset. Comparison of the percentages of CD56bright NK cells revealed no statistically significant difference. (B, C) show the frequencies of CD16+, CD16low, and CD16− cells among CD56dim and CD56bright NK cells, respectively. Relative distributions are depicted as percentages of the respective NK cell subset, defined as CD3−CD56bright or CD3−CD56dim cells among live, single, small PBMCs (FSC/SSC), as assessed by polychromatic flow cytometry. Box plots represent the median, .75 and .25 percentiles, with whiskers showing minimum and maximum values. Each symbol corresponds to one sample, and comparisons of 1st-line STS (n = 8), 2nd-line STS (n = 5), RCC patients (n = 11) with HD (n = 21) are depicted. For statistical analysis, Kruskal–Wallis test with Dunn's post hoc test was used. *p < .05, ***p < .001.
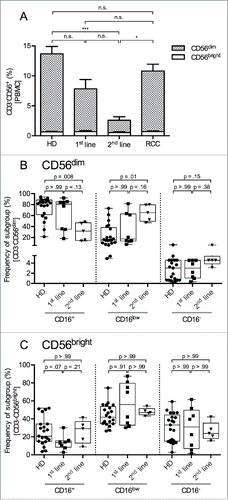
Since the frequency of NK cells was reduced in PBMCs of STS patients, the diminished NK cell-mediated cytotoxicity, as observed, might be caused by reduced numbers of these cells. However, differences between HD and STS patients remained after normalizing the cytotoxicity values to the frequency of NK cells or CD56dim NK cells among PBMCs, indicating that other mechanisms limit the NK cell cytotoxicity in STS (Figs. S3A and B).
Percentages of NKG2D- and CD3ζ-expressing NK cells are reduced in 2nd-line STS patients
As the reduced NK cell-specific lytic capacity of PBMCs from 1st-line patients cannot be fully explained by reduced proportions of NK cells within PBMCs, we analyzed the expression of NKG2D and NKp46, two of the main activating NK cell receptors that trigger NK cell cytotoxicity.Citation28,29 Additionally, expression of CD3ζ, a signaling adaptor protein for NKp46, and the expression of the inhibitory receptor CD94 were assessed.
NKG2D is frequently downregulated on peripheral NK cells of cancer patients.Citation13,30 This downregulation correlates with decreased cytotoxic activity.Citation31 Percentages of NKG2D+ NK cells were similar for 1st-line STS patients, RCC patients and HD (range 41–86%). In contrast, significantly lower percentages were observed for 2nd-line STS patients (median 37%, range 28–69%) (, left panels).
Figure 3. 2nd-line STS patients show reduced percentages of cells expressing the activating NK cell receptor NKG2D and the CD3ζ signaling adaptor protein in peripheral NK cells. (A) Expression of NKG2D (left panel), NKp46 (middle panel), and CD3ζ (right panel) of HD (n = 4), 1st-line STS patients (n = 6), and RCC patients (n = 4) analyzed by polychromatic flow cytometry of uncultured PBMCs. (B) Marker expression in HD (n = 13), 2nd-line STS patients (n = 5) and RCC patients (n = 7). (A–B) Percentages of marker-positive cells among NK cells (CD3−CD56+ cells within live, single, small (FSC/SSC) PBMCs) are depicted. Box plots represent the median, .75 and .25 percentiles, with whiskers showing minimum and maximum values. Each symbol corresponds to one sample. For statistical analyses, Kruskal–Wallis test with Dunn's post hoc tests was used.
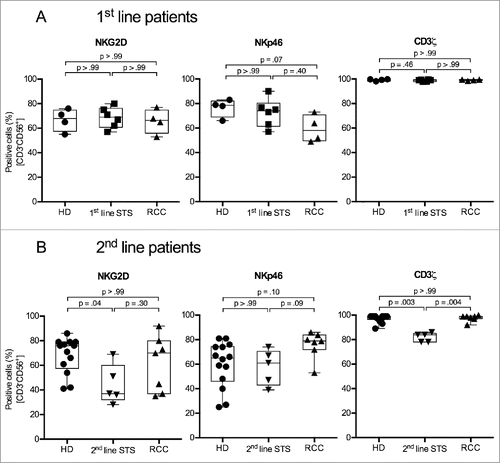
NKp46 has been found to show reduced expression in patients with cancer, e.g., melanoma and acute myeloid leukemia.Citation14,32 However, we did not observe significant differences in the frequency of NKp46+ NK cells in all analyzed patient cohorts (, middle panels).
CD3ζ-deficiency is often seen in NK cells of cancer patients and is associated with impaired cytotoxicity.Citation33 Proportions of CD3ζ+ NK cells were comparable for 1st-line STS patients, RCC patients and HD (median 99% for all groups), however, the frequency of CD3ζ+ NK cells was significantly reduced in 2nd-line STS patients (median 84%) (, right panels).
CD94, when dimerized with NKG2A, is an inhibitory NK cell receptor.Citation34 No significant differences in the percentage of CD94+ NK cells were seen between patient groups and HD (data not shown).
Percentages of perforin-positive NK cells and NK cells expressing CD57 are reduced in 2nd-line STS patients
Cytotoxic activity of NK cells depends on their ability to secrete lytic effector proteins, i.e., perforin and granzyme B.Citation35 Intracellular staining of PBMCs showed that percentages of perforin+ NK cells were not different between 1st-line STS patients, RCC patients and HD. In contrast, 2nd-line STS patients had significantly lower proportions of perforin+ NK cells (median 48%) compared with HD (97%). (, left panels). For granzyme B, patient and donor groups showed comparable proportions of positive cells, and NK cells from RCC patients showed a trend toward higher percentages of granzyme B+ cells compared to HD (median 83% vs. 63%; , middle panels).
Figure 4. 2nd-line STS patients show reduced percentages of perforin+ and CD57+ cells in peripheral NK cells. (A) Expression of perforin (left panel), granzyme B (middle panel) and CD57 (right panel) of HD (n = 4), 1st-line STS patients (n = 6) and RCC patients (n = 4) analyzed by polychromatic flow cytometry of uncultured PBMCs. (B) Marker expression in HD (n = 13), 2nd-line STS patients (n = 5) and RCC patients (n = 7). (A–B) Percentages of marker-positive cells among NK cells (CD3−CD56+ cells within live, single, small (FSC/SSC) PBMCs) are depicted. Box plots represent the median, .75 and .25 percentiles, with whiskers showing minimum and maximum values. Each symbol corresponds to one sample. For statistical analyses, Kruskal–Wallis test with Dunn's post hoc tests was used.
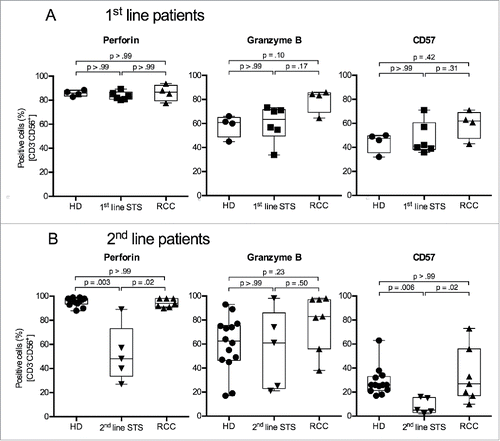
CD57 is a marker for terminally differentiated, cytotoxic NK cells.Citation36 CD57+ NK cells contain high amounts of granzyme B and perforin.Citation37 High frequencies of CD57+ tumor-infiltrating NK cells have been associated with improved outcomes for different kinds of cancer.Citation38 Frequencies of CD57+ NK cells were comparable between HD (median 48%), 1st-line STS (41%) and RCC patients (62%). On the other hand, in line with observed lower frequencies of CD56dim and perforin+ NK cells, 2nd-line STS patients showed a significantly reduced percentage of CD57+ NK cells (median 5%) compared with NK cells of RCC patients (27%) and HD (26%) (, right panels).
Discussion
NK cells and CD8+ T lymphocytes can act as major players in antitumor responses, and inhibition of their function has been associated with tumor immune escape.Citation39,40 Here, we examined the NK cell-specific cytotoxicity of PBMCs of patients with STS and RCC in comparison with healthy controls, and aimed to identify differences between patient groups and the underlying mechanisms of NK cell activation and inhibition. RCC patients were chosen as a second reference group due to the documented prognostic importance of tumor-infiltrating NK cells for this histology.Citation41-44
We observed that the cytotoxic function of NK cells from STS patients was profoundly impaired, while, notably, NK cells of RCC patients exhibited a cytotoxic ability similar to HD. To our knowledge, this is the first description of functional deficits of NK cells from patients with intermediate and high-grade STS. While suppressed NK cell activity has been demonstrated for a variety of epidermal and mesenchymal neoplasias,Citation8,10,11,30,45,46 functional impairment of NK cells from peripheral blood does not seem to be a universal phenomenon in cancer patients, as NK cell-mediated cytotoxicity was normal in patients with pancreatic cancer and RCC.Citation47,48 Interestingly, patients with gastrointestinal stromal tumors (GIST), a STS sub-entity with distinct clinical and pathophysiological features separating it from other STS, have also been shown to display unaltered NK cell-specific cytotoxicity compared with HD.Citation49
We attempted to gain insight into the mechanisms that might cause the NK cells' functional deficit, as this might reveal means of intervention that could help prevent the development of dysfunction or restore activity. Surgery has been described to cause NK cell dysfunction,Citation50 but these effects were of limited duration, with cytotoxic capacity returning to pre-surgery values after approximately 30 d.Citation47 In our cohort of STS patients, blood samples were taken at least 4 weeks after surgery. Therefore, post-surgical effects seem unlikely as explanation of impaired NK cell-specific cytotoxicity. Additionally, there was no difference between patients who had undergone tumor resection and patients who had been diagnosed by tumor biopsy without surgery (data not shown). Age can also be excluded as an influential factorCitation51 since RCC patients, who were older (median age 65 y) than STS patients (median 44 and 34 y for 1st- and 2nd-line STS patients, respectively), showed even higher levels of NK cell cytotoxicity in comparison with STS patients.
Administration of cytostatic agents needs to be considered as one factor with impact on NK cell-specific cytotoxicity, in particular since the analyzed STS cases include samples from patients who had or had not received chemotherapy before blood withdrawal. Reports on the effects of cytostatic treatment on NK cell-specific cytotoxicity in vivo are scarce, and results vary depending on the treated cancer and the used cytostatic agents, documenting inhibition as well as augmentation of NK cell-specific cytotoxicity.Citation52-56 In vitro assays show distinct effects of different chemotherapeutic agents on NK cell cytotoxicity. Doxorubicin and epirubicine, anthracyclines frequently used in the treatment of STS patients, are among those agents that have been shown to only marginally influence NK cell lytic activity.Citation57 However, these results have to be interpreted with caution, as in vitro assays cannot consider drug metabolism and interactions relevant for NK cell activation. Moreover, detectable effects are restricted to short-term outcomes and thus cannot reveal effects that are associated with or depend on NK cell proliferation.
In our study, all 2nd-line patients had been treated with anthracyclines and usually ifosfamide, but not with any tyrosine-kinase inhibitors (TKI), and were included following relapse or progression of disease. The NK cells of the 2nd-line patient cohort showed strikingly poor cytotoxicity with levels significantly below those of chemotherapy-naive 1st-line STS patients. This might suggest negative effects of systemic chemotherapy on the function of peripheral NK cells. However, when 2nd-line STS patients were divided into two groups according to the median time interval between last chemotherapeutic treatment and blood withdrawal (5 mo), patients who had not received cytotoxic medication for years did not show higher NK cell-specific cytotoxicity compared with patients who had received their last chemotherapy within 5 mo before blood withdrawal. Interestingly, one of the 2nd-line patients (red triangle in Fig. S1A) had a NK cell-specific lytic activity that was comparable to 1st-line STS patients. This patient had very late relapse of clear cell sarcoma almost 18 y after initial treatment. The observed preserved NK cell cytotoxicity supports the assumption that this late reoccurrence might rather be a secondary de novo transformation than an actual relapse of the original disease.
Since NK cell dysfunction still persists in 2nd-line STS patients with very long chemotherapy-free periods, the detrimental effects of chemotherapy on NK cell activity would have had to be very long-lasting if they were to explain the observed NK cell dysfunction. However, such very long-lasting effects seem unlikely considering that NK cell numbers quickly recover after chemotherapyCitation58 and cytotoxicity is restored quickly even after maximally invasive procedures like allogeneic transplantation.Citation59
Therefore, in addition—or alternatively—to chemotherapy, disease burden and dissemination might explain the difference between 1st-line and 2nd-line STS patients. For different neoplasias decrease in NK cell cytotoxicity has been described to be stage-dependent. For patients with lymphoma, melanoma, and head and neck cancer, a more advanced, e.g., metastatic/disseminated disease was associated with lower NK cell lytic activity compared to patients with localized, early-stage disease.Citation9,10,60 Of the 11 2nd-line STS patients in our study, seven had metastatic disease, while none of the patients in the 1st-line cohort had evidence of metastasis. The differences in NK cell cytotoxicity between 1st- and 2nd-line patients might therefore be associated with the more advanced disease status of the 2nd-line patients. Accordingly, subgroup analyses revealed that PBMCs of 2nd-line patients with metastatic disease had lower NK cell-mediated cytotoxicity than those of 2nd-line patients without metastasis. However, in addition to disease status, and contradictory to the observation that NK cell dysfunction did not recover in a time-dependent manner after chemotherapy, chemotherapeutic treatment possibly had an impact on the cytotoxic capacity of NK cells, since NK cell cytotoxicity of non-metastatic 2nd-line STS patients was significantly lower compared with the cytotoxicity of PBMCs of (all non-metastatic) 1st-line patients. We observed no obvious correlation between NK cell-specific cytotoxicity and tumor volume, and 1st-line STS patients with resected tumors (hence absent/minimal tumor burden) did not show significantly higher NK cell cytotoxicity than patients who had not received surgery (data not shown). Taken together, disease dissemination can be seen as an important factor that influences NK cell cytotoxicity in STS patients. However, other factors like chemotherapeutic treatment also seem to affect the cytotoxic capacity of NK cells. Yet, these interpretations have to be taken with caution due to the low number of patients available for the analyses.
Finally, we tested a scenario that patients with low NK cell-specific cytotoxicity might have a higher probability of relapse after 1st-line therapy. This would enrich the group of 2nd-line STS patients with individuals showing low NK cell cytotoxicity. However, in our cohort, patients with progressive disease or relapse after 1st-line therapy had comparable NK cell-specific cytotoxicity with patients that did not experience relapse (data not shown). While this refutes such consideration, caution is indicated, as only 13 patients were assessed. Prospective serial measurements of NK cell function over the course of disease and therapy are warranted in order to substantiate the rejection of this hypothesis. Interestingly, new small molecule agents used for targeted therapy, e.g., BRAF inhibitors or TKIs like imatinib have been shown to positively affect NK cell proliferationCitation61 and to increase NK cell cytotoxicity.Citation49 Furthermore, imatinib therapy leads to increased tumor infiltration of immune cells, including NK cells, in GIST.Citation15 As TKIs like pazopanib become important in the treatment of non-GIST STS, it will be exciting to see how these relatively new agents influence NK cell-specific cytotoxicity of STS patients, and if the effects of TKIs are different from those of “classic” cytostatic agents. The possible promotion of tumor infiltration with NK cells by these agents will be of special interest, as STS have been shown to display only minor infiltration by NK cells.Citation15
To define the cellular basis of the poor NK cell function, we analyzed the NK cell population by flow cytometry. We observed drastic changes in the composition of peripheral NK cell populations of patients that intensified from 1st- to 2nd-line STS patients. Alterations included reduced NK cell frequencies and altered distributions of NK cell subsets. Notably, mainly CD56dim NK cell subsets were affected. As this subset is considered the main cytotoxic subset of NK cells,Citation62 the pronounced reduction of this NK subset could be an explanation for the poor cytotoxicity of PBMCs of STS patients. Yet, other factors still contribute since NK cell cytotoxicity values of PBMCs of STS patients remained below those of HD and RCC patients after normalization to the percentage of NK cells. One contributing factor could be the reduction of CD16 expression on CD56dim NK cells, as CD16 in synergy with other activating receptors like NKG2D or NKp46 is required to activate cytotoxicity.Citation63
PBMCs of 1st-line STS also exhibited a notable reduction of CD56dim NK cell frequencies compared with HD and RCC. Values of CD56dimCD16+ subgroup proportions of 1st-line STS patients were between those of HD and 2nd-line patients. As 1st-line STS patients did not receive systemic cytostatic therapy, these changes may be disease-associated. Notably, not all cancer types seem to exert effects on peripheral NK cells as NK cells from RCC patients did not differ from those of HD. Disturbances in NK cell subsets seem to follow disease progression, as they worsened from 1st- line to 2nd-line STS patients. Besides increasing tumor burden, a contribution of chemotherapy to the development of these alterations cannot be excluded. However, such long lasting effects are to date unprecedented as discussed before.
The drastic alterations of NK cell subset composition in PBMCs of 2nd-line STS patients compared to HD and RCC patients provided a plausible explanation for the impaired NK cell reactivity. Yet, alterations in 1st-line STS patients were moderate and did not reach statistical significance, thus they cannot convincingly explain the poor cytotoxicity of NK cells of 1st-line STS patients. Subsequent analyses included expression of activating NK receptors (NKG2D, NKp46) as well as inhibitory CD94 receptor or signaling adaptor molecule CD3ζ and cytotoxic proteins (perforin, granzyme B), all of which contribute to the level of NK cell activity and are frequently downregulated in cancer patient NK cells.Citation14,45 Again, our observations failed to provide an explanation for the deficient NK cell activity of 1st-line STS patients as none of the markers showed any difference between 1st-line STS patients and HD or RCC patients.
On the other hand, NK cells of 2nd-line STS patients showed deficits in several of these markers, including reduced proportions of NK cells expressing NKG2D, CD3ζ, perforin, and CD57. Interestingly, percentages of NK cells expressing granzyme B, NKp46 or CD94 were not significantly altered.
CD57 is a marker for terminally differentiated and cytotoxic NK cells.Citation36,37 The reduced percentage of CD57+ NK cells in 2nd-line STS patients might hint at a relative predominance of immature NK cells in the peripheral blood of these patients, and higher proportions of immature NK cells might be seen as an effect of recent cytostatic treatment, as chemotherapy temporarily suppresses bone marrow function. Arguing against this interpretation, however, is the observation that the expression of NKp46, which is only found on NK cells at later maturation stages,Citation1 was comparable between HD, RCC, and sarcoma patients in our study.
The observation that deficits in marker expression of NK cells were only seen in 2nd-line STS might indicate that chemotherapy negatively affects the quality of NK cells. Yet, as discussed above, all 2nd-line patients had month-long treatment-free periods before blood withdrawal. Moreover, published results do not suggest that strong effects of cytostatic treatment are to be expected. For example, neoadjuvant chemotherapy had no effect on NKG2D expression on NK cells of breast cancer patients,Citation54 and doxorubicin did not alter NKG2D expression in a murine system in vivo.Citation64 For NKp46, chemotherapy increased expression in melanoma patients.Citation65 Influence of chemotherapeutic treatment on CD3ζ expression in NK cells has not been addressed yet. In T cells, treatment of CLL patients with fludarabin lead to an decrease in the percentage of CD3ζ+ cells.Citation66 Taken together, there is no convincing evidence that the observed changes in NK cell marker expression might be linked to previous cytostatic treatment. Thus, disease-specific effects, e.g., mediated by cytokine release or receptor shedding,Citation3,67,68 might be an explanation for the observed changes.
In summary, we could demonstrate profound alterations of NK cell characteristics in the peripheral blood of STS patients, including reduction in NK cell frequency and deviations in subset distribution, as well as changes in the expression of activating receptors, signaling and cytotoxic molecules, and differentiation markers. Interestingly, none of these changes were seen in NK cells of RCC patients. 1st- and 2nd-line STS patient cohorts differed regarding the degree of alterations, which was considerably more pronounced in 2nd-line patients. The observed changes in subset composition and expression of molecules involved in the activation of NK cells suffice to explain the lack of cytotoxicity of NK cells in 2nd-line STS patients. Although attempts to elucidate the reduced NK cell cytotoxicity of 1st-line STS patients remain inconclusive at present, reduced NK cell frequencies, particularly in the cytotoxic subset of CD56dimCD16+ NK cells were discernable and hint at possible explanations for this phenomenon. Further analyses to better characterize the NK cells of 1st-line STS patients are warranted.
Our study provides important information for potential immunotherapeutic approaches in patients with STS. STS negatively affects the NK cell population concerning frequency but also, and most importantly, concerning cytotoxic function. This is apparently specific for soft-tissue sarcoma, as NK cells of RCC patients were not affected. Yet, despite severe suppression, NK cells of STS patients can recover activity when stimulated with IL-2/TKD reaching levels of cytotoxicity comparable with those of similarly activated PBMCs of HD. This indicates that STS patients may benefit from immunotherapeutic approaches tailored to activate NK cells. We did not perform flow cytometry analyses of the activated cells, so the reversion of alterations observed in 2nd-line STS patients after stimulation still need to be addressed. This knowledge might, however, be of interest for future immunotherapeutic interventions for STS patients, as protocols using ex vivo expanded and activated NK cells as adoptive immunotherapy have provided disappointing outcomes for different tumor entities.Citation69-73 To improve the outcome of these therapies, NK cell markers and effector molecules that describe functional NK cells have to be defined. Receptors and effector molecules we observed to be aberrantly expressed might be suitable as objectives to optimize NK cells used for immunotherapeutic approaches in STS patients.
Patients, materials, and methods
Patients and healthy donors
Blood samples were taken from patients and donors after they gave written informed consent. The study was approved by the Institutional Review Board on Medical Ethics, and the Declaration of Helsinki was observed.
STS patients with intermediate and high-grade tumors were enrolled between 2008 and 2014 (Klinikum Großhadern Medical Center, Munich, Germany and Schön Klinik Starnberger See, Berg, Germany). Of the 24 STS patients, 13 (median age 44 y, range 31–75 y) had not received previous chemotherapy (1st-line patients), whereas 11 patients (median age 34 y, range 24–77 y) had been treated with anthracycline-based chemotherapy before enrollment (2nd-line patients). Patients with GIST were excluded. All 1st-line patients had macroscopic tumor burden or had had tumor resection within 4–16 weeks before inclusion. All 2nd-line patients showed progression or relapse of disease at the time of enrollment, whereby progression did not necessarily occur during previous chemotherapeutic treatment, but could also emerge after the end of (e.g., adjuvant) chemotherapeutic treatment. Time intervals since last cytostatic drug treatment, therefore, varied between patients. Detailed patients' characteristics are shown in . Blood samples of 1st-line patients were taken after definite diagnosis (by biopsy or resection) 4–8 weeks after the diagnostic procedure (surgery in 8 of 11 patients), but always before the initiation of cytostatic treatment. For 2nd-line patients, blood samples were taken directly before the initiation of the non-1st-line chemotherapy. The time interval between last cytostatic treatment and blood withdrawal was always 4 weeks or longer. Longitudinal analyses of single individuals were not possible, as no patient was recruited consecutively in both the 1st- and the 2nd-line patient group.
All RCC patients (n = 11, median age 65 y, range 42–80 y) had not received prior systemic treatment. Blood was taken before nephrectomy. Detailed patients' characteristics are shown in .
Table 2. Characteristics of RCC patients. Median age of all patients was 65 y. TNM stage indicates tumor stage, assessed by CT/MRI imaging and pathologic diagnosis, at time of blood withdrawal. None of the patients had received systemic treatment or had tumor-related surgery before blood was withdrawn. Patients 1–4 were assessed as comparative group for cytotoxicity assays and flow cytometry assays for 1st-line STS patients, patients 5–11 were assessed as comparative group for flow cytometry assays for 2nd-line STS patients.
32 HD were recruited at HelmholtzZentrum Munich. Initially, HD were stratified into two age groups (24–59 y and 60–68 years, respectively) to match them to younger STS and older RCC patient groups in our cohort. Since we did not observe significant differences in all analyzed data between the two HD groups (data not shown), experiments were pooled for graphical presentation.
Isolation of PBMC and stimulation of NK cell cytotoxicity
PBMCs were isolated from venous blood using density gradient separation with Pancoll (PAN-Biotech, no. P04-60500). Cells from the interphase were either cryo-preserved or used immediately.
Where indicated, PBMCs were cultured in RPMI 1640 (Sigma-Aldrich, no. R0883), supplemented with 10% fetal calf serum, L-glutamine (both from PAN-Biotech, no. P30-1302 and P04-80100, respectively) and penicillin/streptomycin (Gibco/Thermo Fisher Scientific, no. 15140-122) and NK cells were stimulated with interleukin-2 (IL-2, 1000 IU/mL, Gibco/ Thermo Fisher Scientific, no. PHC0021) and TKD (14 amino acid derivative of Hsp70, 2 µg/mL, gift from G. Multhoff, Munich)Citation27 for 96 h at 37°C and 5 % CO2.
Chromium release assay
The MHC class I-deficient cell line K562 (ATCC no. CCL-243) was cultured in RPMI 1640 (Sigma-Aldrich, no. R0883), supplemented with 10% fetal calf serum, L-glutamine, amino acids (BME amino acids solution, all from PAN-Biotech, no. P30-1302, P04-80100, and P08-2000, respectively) and penicillin/streptomycin (Gibco/ Thermo Fisher Scientific, no. 15140-122). NK cytotoxicity was determined in a standard 4 h chromium release assay.Citation74 Briefly, 3 × 105 K562 cells were incubated with 3.7 × 106 Bq Na251CrO4 (Hartmann Analytic, no. Cr-RA-8) for 90 min and then washed twice. 1.2 × 105 PBMC in 100 µL RPMI 1640 were added as triplicates to a 96-well U bottom plate. 1:1 dilutions of the PBMC were performed to create serial dilutions. 3 × 103 K562 in 100 µL medium were added to achieve 40:1, 20:1, 10:1, and 5:1 effector to target ratios. After 4 h the supernatants were collected in solid scintillator coated microplates (LumaPlate, Perkin Elmer, no. 6006633). Activity of the dried plates was measured in a gamma counter. To determine the spontaneous release, supernatants from wells with K562 targets without added effector PBMC were collected. Maximum release was assessed from wells with K562 cells collected directly after incubation with Na251CrO4. The lytic activity was calculated as
PBMCs from all RCC patients were cryo-conserved and used directly after thawing, with no cytokines added to the medium. PBMCs of HD or STS patients were either cryo-conserved and used directly after thawing with no cytokines added to the medium, or were used freshly within 48 h after isolation. IL-2 was added only for stimulation assays. All assays included PBMCs from HD as positive control. Spontaneous release was always < 20% of maximum release.
Multiparameter flow cytometry
Cells were stained and analyzed as previously described.Citation75 Antibodies are listed in Table S1. Dead cells were excluded with propidium iodide (0.4 µg/mL, Invitrogen, no. P3566) or 7-AAD (10 µg/mL, Sigma, no. A9400). NK cells were gated as CD3−CD56+ cells within live, single leukocytes (selected based on forward scatter/side scatter characteristics). The gating strategy is exemplified in Fig. S2A. The percentage of marker-positive cells within the gated CD3−CD56+ NK cells was determined using an internal population negative for the analyzed marker or isotype controls as reference, respectively. Identification of NK cell subsets is exemplified in Fig. S2B. PBMCs of STS and RCC patients were always analyzed in parallel with samples from HD. As 1st- and 2nd-line STS patients were enrolled at different time points, they were analyzed in independent experiments. Moreover, HD and RCC patients employed as controls were different for 1st- and 2nd-line STS patient analyses. Therefore, results of HD and RCC measurements were not pooled, but displayed in separate graphs. Age matching of HD samples was later omitted since initial analyses did not show significant differences in the examined markers between younger and older HD (see above).
Statistical analyses
Comparisons of multiple subgroups (e.g., expression levels of NK cell antigens) were performed by non-parametrical Kruskal–Wallis test with Dunn's post hoc tests or two-way ANOVA with Bonferroni's post hoc test (for paired values, e.g., cytotoxicity before and after stimulation). For comparisons of two subgroups (e.g., percentages of leukocyte subgroups of patients and HD), non-parametrical Mann–Whitney U tests were performed. Correlation analyses were done with Pearson's correlation and linear regression tests. Graphpad Prism (Version 6, Graphpad software) was used for all statistical analyses.
Disclosure of potential conflicts of interest
No potential conflicts of interest were disclosed.
KONI_A_1178421_s02.zip
Download Zip (1.9 MB)Funding
AM, JCS, and EN received financial support through Deutsche Forschungsgemeinschaft (SFB-TR36) and Deutsche Krebshilfe.
References
- Caligiuri MA. Human natural killer cells. Blood 2008; 112:461-9; PMID:18650461; http://dx.doi.org/10.1182/blood-2007-09-077438
- Holmes TD, El-Sherbiny YM, Davison A, Clough SL, Blair GE, Cook GP. A human NK cell activation/inhibition threshold allows small changes in the target cell surface phenotype to dramatically alter susceptibility to NK cells. J Immunol 2011; 186:1538-45; PMID:21191066; http://dx.doi.org/10.4049/jimmunol.1000951
- Nausch N, Cerwenka A. NKG2D ligands in tumor immunity. Oncogene 2008; 27:5944-58; PMID:18836475; http://dx.doi.org/10.1038/onc.2008.272
- Lanier LL. NK cell recognition. Annu Rev Immunol 2005; 23:225-74; PMID:15771571; http://dx.doi.org/10.1146/annurev.immunol.23.021704.115526
- Waldhauer I, Steinle A. NK cells and cancer immunosurveillance. Oncogene 2008; 27:5932-43; PMID:18836474; http://dx.doi.org/10.1038/onc.2008.267
- Imai K, Matsuyama S, Miyake S, Suga K, Nakachi K. Natural cytotoxic activity of peripheral-blood lymphocytes and cancer incidence: an 11-year follow-up study of a general population. Lancet 2000; 356:1795-9; PMID:11117911; http://dx.doi.org/; http://dx.doi.org/10.1016/S0140-6736(00)03231-1
- Strayer DR, Carter WA, Mayberry SD, Pequignot E, Brodsky I. Low natural cytotoxicity of peripheral blood mononuclear cells in individuals with high familial incidences of cancer. Cancer Res 1984; 44:370-4; PMID:6690050
- Szkaradkiewicz A, Karpiński TM, Drews M, Borejsza-Wysocki M, Majewski P, Andrzejewska E. Natural killer cell cytotoxicity and immunosuppressive cytokines (IL-10, TGF-beta1) in patients with gastric cancer. J Biomed Biotechnol 2010; 2010:901564-7; PMID:20445748; http://dx.doi.org/10.1155/2010/901564
- Mirjačić Martinović K, Konjević G, Babović N, Inić M. The stage dependent changes in NK cell activity and the expression of activating and inhibitory NK cell receptors in melanoma patients. J Surg Res 2011; 171:637-49; PMID:20828749; http://dx.doi.org/10.1016/j.jss.2010.05.012
- Konjević G, Jurisić V, Banićevic B, Spuzić I. The difference in NK-cell activity between patients with non-Hodgkin“s lymphomas and Hodgkin”s disease. Br J Haematol 1999; 104:144-51; PMID:10027727; http://dx.doi.org/10.1046/j.1365-2141.1999.01129.x
- Verhoeven DHJ, de Hooge ASK, Mooiman ECK, Santos SJ, Dam ten MM, Gelderblom H, Melief CJM, Hogendoorn PCW, Egeler RM, van Tol MJD et al. NK cells recognize and lyse Ewing sarcoma cells through NKG2D and DNAM-1 receptor dependent pathways. Mol Immunol 2008; 45:3917-25; PMID:18657862; http://dx.doi.org/10.1016/j.molimm.2008.06.016
- Viel S, Charrier E, Marçais A, Rouzaire P, Bienvenu J, Karlin L, Salles G, Walzer T. Monitoring NK cell activity in patients with hematological malignancies. Oncoimmunology 2013; 2:e26011; PMID:24327939; http://dx.doi.org/10.4161/onci.26011
- Peng Y-P, Zhu Y, Zhang J-J, Xu Z-K, Qian Z-Y, Dai C-C, Jiang K-R, Wu J-L, Gao W-T, Li Q et al. Comprehensive analysis of the percentage of surface receptors and cytotoxic granules positive natural killer cells in patients with pancreatic cancer, gastric cancer, and colorectal cancer. J Transl Med 2013; 11:262; PMID:24138752; http://dx.doi.org/10.1186/1479-5876-11-262
- Sanchez-Correa B, Morgado S, Gayoso I, Bergua JM, Casado JG, Arcos MJ, Bengochea ML, Duran E, Solana R, Tarazona R. Human NK cells in acute myeloid leukaemia patients: analysis of NK cell-activating receptors and their ligands. Cancer Immunol Immunother 2011; 60:1195-205; PMID:21644031; http://dx.doi.org/10.1007/s00262-011-1050-2
- Rusakiewicz S, Semeraro M, Sarabi M, Desbois M, Locher C, Mendez R, Vimond N, Concha A, Garrido F, Isambert N et al. Immune infiltrates are prognostic factors in localized gastrointestinal stromal tumors. Cancer Res 2013; 73:3499-510; PMID:23592754; http://dx.doi.org/10.1158/0008-5472.CAN-13-0371
- Schleypen JS, Geldern Von M, Weiss EH, Kotzias N, Rohrmann K, Schendel DJ, Falk CS, Pohla H. Renal cell carcinoma-infiltrating natural killer cells express differential repertoires of activating and inhibitory receptors and are inhibited by specific HLA class I allotypes. Int J Cancer 2003; 106:905-12; PMID:12918068; http://dx.doi.org/10.1002/ijc.11321
- Desbois M, Rusakiewicz S, Locher C, Zitvogel L, Chaput N. Natural killer cells in non-hematopoietic malignancies. Front Immunol 2012; 3:395; PMID:23269924; http://dx.doi.org/10.3389/fimmu.2012.00395
- Clark MA, Fisher C, Judson I, Thomas JM. Soft-tissue sarcomas in adults. N Engl J Med 2005; 353:701-11; PMID:16107623; http://dx.doi.org/10.1056/NEJMra041866
- Casali PG, Blay J-Y, ESMO/CONTICANET/EUROBONET Consensus Panel of experts. Soft tissue sarcomas: ESMO Clinical Practice Guidelines for diagnosis, treatment and follow-up. Ann Oncol 2010; 21(Suppl 5):v198-203; PMID:20555081; http://dx.doi.org/10.1093/annonc/mdq209
- Italiano A, Delva F, Mathoulin-Pelissier S, Le Cesne A, Bonvalot S, Terrier P, Trassard M, Michels J-J, Blay J-Y, Coindre JM et al. Effect of adjuvant chemotherapy on survival in FNCLCC grade 3 soft tissue sarcomas: a multivariate analysis of the French Sarcoma Group Database. Ann Oncol 2010; 21:2436-41; PMID:20439343; http://dx.doi.org/10.1093/annonc/mdq238
- Issels RD, Lindner LH, Verweij J, Wust P, Reichardt P, Schem B-C, Abdel-Rahman S, Daugaard S, Salat C, Wendtner C-M et al. Neo-adjuvant chemotherapy alone or with regional hyperthermia for localised high-risk soft-tissue sarcoma: a randomised phase 3 multicentre study. Lancet Oncol 2010; 11:561-70; PMID:20434400; http://dx.doi.org/10.1016/S1470-2045(10)70071-1
- Penel N, Van Glabbeke M, Marreaud S, Ouali M, Blay J-Y, Hohenberger P. Testing new regimens in patients with advanced soft tissue sarcoma: analysis of publications from the last 10 years. Ann Oncol 2011; 22:1266-72; PMID:21183581; http://dx.doi.org/10.1093/annonc/mdq608
- Sinha S, Peach AHS. Diagnosis and management of soft tissue sarcoma. BMJ 2010; 341:c7170; PMID:21190968; http://dx.doi.org/10.1136/bmj.c7170
- Smyth MJ, Crowe NY, Godfrey DI. NK cells and NKT cells collaborate in host protection from methylcholanthrene-induced fibrosarcoma. Int Immunol 2001; 13:459-63; PMID:11282985; http://dx.doi.org/10.1093/intimm/13.4.459
- O'Sullivan T, Saddawi-Konefka R, Vermi W, Koebel CM, Arthur C, White JM, Uppaluri R, Andrews DM, Ngiow SF, Teng MWL et al. Cancer immunoediting by the innate immune system in the absence of adaptive immunity. J Exp Med 2012; 209:1869-82; PMID:22927549; http://dx.doi.org/10.1084/jem.20112738
- Moser C, Schmidbauer C, Gürtler U, Gross C, Gehrmann M, Thonigs G, Pfister K, Multhoff G. Inhibition of tumor growth in mice with severe combined immunodeficiency is mediated by heat shock protein 70 (Hsp70)-peptide-activated, CD94 positive natural killer cells. Cell Stress Chaperones 2002; 7:365-73; PMID:12653481; http://dx.doi.org/10.1379/1466-1268(2002)007<0365:IOTGIM>2.0.CO;2
- Multhoff G, Pfister K, Gehrmann M, Hantschel M, Gross C, Hafner M, Hiddemann W. A 14-mer Hsp70 peptide stimulates natural killer (NK) cell activity. Cell Stress Chaperones 2001; 6:337-44; PMID:11795470; http://dx.doi.org/10.1379/1466-1268(2001)006<0337:AMHPSN>2.0.CO;2
- Moretta L, Bottino C, Pende D, Vitale M, Mingari MC, Moretta A. Human natural killer cells: Molecular mechanisms controlling NK cell activation and tumor cell lysis. Immunol Lett 2005; 100:7-13; PMID:16109445; http://dx.doi.org/10.1016/j.imlet.2005.07.004
- Chen X, Trivedi PP, Ge B, Krzewski K, Strominger JL. Many NK cell receptors activate ERK2 and JNK1 to trigger microtubule organizing center and granule polarization and cytotoxicity. Proc Natl Acad Sci USA 2007; 104:6329-34; PMID:17395718; http://dx.doi.org/10.1073/pnas.0611655104
- Garcia-Iglesias T, Del Toro-Arreola A, Albarran-Somoza B, Del Toro-Arreola S, Sanchez-Hernandez PE, Ramirez-Dueñas MG, Balderas-Peña LMA, Bravo-Cuellar A, Ortiz-Lazareno PC, Daneri-Navarro A. Low NKp30, NKp46 and NKG2D expression and reduced cytotoxic activity on NK cells in cervical cancer and precursor lesions. BMC Cancer 2009; 9:186; PMID:19531227; http://dx.doi.org/10.1186/1471-2407-9-186
- Lee J-C, Lee K-M, Kim D-W, Heo DS. Elevated TGF-beta1 secretion and down-modulation of NKG2D underlies impaired NK cytotoxicity in cancer patients. J Immunol 2004; 172:7335-40; PMID:15187109; http://dx.doi.org/; http://dx.doi.org/10.4049/jimmunol.172.12.7335
- Fregni G, Messaoudene M, Fourmentraux-Neves E, Mazouz-Dorval S, Chanal J, Maubec E, Marinho E, Scheer-Senyarich I, Cremer I, Avril M-F et al. Phenotypic and functional characteristics of blood natural killer cells from melanoma patients at different clinical stages. PLoS One 2013; 8:e76928; PMID:24204708; http://dx.doi.org/10.1371/journal.pone.0076928
- Hanaoka N, Jabri B, Dai Z, Ciszewski C, Stevens AM, Yee C, Nakakuma H, Spies T, Groh V. NKG2D initiates caspase-mediated CD3zeta degradation and lymphocyte receptor impairments associated with human cancer and autoimmune disease. J Immunol 2010; 185:5732-42; PMID:20926796; http://dx.doi.org/10.4049/jimmunol.1002092
- Uhrberg M, Valiante NM, Shum BP, Shilling HG, Lienert-Weidenbach K, Corliss B, Tyan D, Lanier LL, Parham P. Human diversity in killer cell inhibitory receptor genes. Immunity 1997; 7:753-63; PMID:9430221; http://dx.doi.org/10.1016/S1074-7613(00)80394-5
- Smyth MJ, Cretney E, Kelly JM, Westwood JA, Street SEA, Yagita H, Takeda K, van Dommelen SLH, Degli-Esposti MA, Hayakawa Y. Activation of NK cell cytotoxicity. Mol Immunol 2005; 42:501-10; PMID:15607806; http://dx.doi.org/10.1016/j.molimm.2004.07.034
- Lopez-Vergès S, Milush JM, Pandey S, York VA, Arakawa-Hoyt J, Pircher H, Norris PJ, Nixon DF, Lanier LL. CD57 defines a functionally distinct population of mature NK cells in the human CD56dimCD16+ NK-cell subset. Blood 2010; 116:3865-74; PMID:20733159; http://dx.doi.org/10.1182/blood-2010-04-282301
- Chattopadhyay PK, Betts MR, Price DA, Gostick E, Horton H, Roederer M, De Rosa SC. The cytolytic enzymes granyzme A, granzyme B, and perforin: expression patterns, cell distribution, and their relationship to cell maturity and bright CD57 expression. J Leukocyte Biol 2009; 85:88-97; PMID:18820174; http://dx.doi.org/10.1189/jlb.0208107
- Nielsen CM, White MJ, Goodier MR, Riley EM. Functional Significance of CD57 Expression on Human NK Cells and Relevance to Disease. Front Immunol 2013; 4:422; PMID:24367364; http://dx.doi.org/10.3389/fimmu.2013.00422
- Dunn GP, Bruce AT, Ikeda H, Old LJ, Schreiber RD. Cancer immunoediting: from immunosurveillance to tumor escape. Nat Immunol 2002; 3:991-8; PMID:12407406; http://dx.doi.org/10.1038/ni1102-991
- Zitvogel L, Tesniere A, Kroemer G. Cancer despite immunosurveillance: immunoselection and immunosubversion. Nat Rev Immunol 2006; 6:715-27; PMID:16977338; http://dx.doi.org/10.1038/nri1936
- Eckl J, Buchner A, Prinz PU, Riesenberg R, Siegert SI, Kammerer R, Nelson PJ, Noessner E. Transcript signature predicts tissue NK cell content and defines renal cell carcinoma subgroups independent of TNM staging. J Mol Med 2011; 90:55-66; PMID:21870102; http://dx.doi.org/10.1007/s00109-011-0806-7
- Geissler K, Fornara P, Lautenschläger C, Holzhausen H-J, Seliger B, Riemann D. Immune signature of tumor infiltrating immune cells in renal cancer. Oncoimmunology 2015; 4:e985082; PMID:25949868; http://dx.doi.org/10.4161/2162402X.2014.985082
- Remark R, Alifano M, Cremer I, Lupo A, Dieu-Nosjean M-C, Riquet M, Crozet L, Ouakrim H, Goc J, Cazes A et al. Characteristics and clinical impacts of the immune environments in colorectal and renal cell carcinoma lung metastases: influence of tumor origin. Clin Cancer Res 2013; 19:4079-91; PMID:23785047; http://dx.doi.org/10.1158/1078-0432.CCR-12-3847
- Prinz PU, Mendler AN, Brech D, Masouris I, Oberneder R, Noessner E. NK-cell dysfunction in human renal carcinoma reveals diacylglycerol kinase as key regulator and target for therapeutic intervention. Int J Cancer 2014; 135:1832-41; PMID:24615391; http://dx.doi.org/10.1002/ijc.28837
- Mamessier E, Sylvain A, Thibult M-L, Houvenaeghel G, Jacquemier J, Castellano R, Gonçalves A, André P, Romagné F, Thibault G et al. Human breast cancer cells enhance self-tolerance by promoting evasion from NK cell antitumor immunity. J Clin Invest 2011; 121:3609-22; PMID:21841316; http://dx.doi.org/10.1172/JCI45816
- Sanchez CJ, Treut T, Boehrer A, Knoblauch B, Imbert J, Olive D, Costello RT. Natural killer cells and malignant haemopathies: a model for the interaction of cancer with innate immunity. Cancer Immunol Immunother 2010; 60:1-13; PMID:20697893; http://dx.doi.org/10.1007/s00262-010-0898-x
- Iannone F, Porzia A, Peruzzi G, Birarelli P, Milana B, Sacco L, Dinatale G, Peparini N, Prezioso G, Battella S et al. Effect of surgery on pancreatic tumor-dependent lymphocyte asset: modulation of natural killer cell frequency and cytotoxic function. Pancreas 2015; 44:386-93; PMID:25621568; http://dx.doi.org/10.1097/MPA.0000000000000288
- Schleypen JS, Baur N, Kammerer R, Nelson PJ, Rohrmann K, Gröne EF, Hohenfellner M, Haferkamp A, Pohla H, Schendel DJ et al. Cytotoxic markers and frequency predict functional capacity of natural killer cells infiltrating renal cell carcinoma. Clin Cancer Res 2006; 12:718-25; PMID:16467081; http://dx.doi.org/10.1158/1078-0432.CCR-05-0857
- Menard C, Blay J-Y, Borg C, Michiels S, Ghiringhelli F, Robert C, Nonn C, Chaput N, Taieb J, Delahaye NF et al. Natural killer cell IFN- levels predict long-term survival with imatinib mesylate therapy in gastrointestinal stromal tumor-bearing patients. Cancer Res 2009; 69:3563-9; PMID:19351841; http://dx.doi.org/10.1158/0008-5472.CAN-08-3807
- Ramirez MF, Ai D, Bauer M, Vauthey J-N, Gottumukkala V, Kee S, Shon D, Truty M, Kuerer HM, Kurz A et al. Innate immune function after breast, lung, and colorectal cancer surgery. J Surg Res 2015; 194:185-93; PMID:25475022; http://dx.doi.org/10.1016/j.jss.2014.10.030
- Hazeldine J, Lord JM. The impact of ageing on natural killer cell function and potential consequences for health in older adults. Ageing Res Rev 2013; 12:1069-78; PMID:23660515; http://dx.doi.org/10.1016/j.arr.2013.04.003
- Cox MC, Battella S, La Scaleia R, Pelliccia S, Di Napoli A, Porzia A, Cecere F, Alma E, Zingoni A, Mainiero F et al. Tumor-associated and immunochemotherapy-dependent long-term alterations of the peripheral blood NK cell compartment in DLBCL patients. Oncoimmunology 2015; 4:e990773; PMID:25949906; http://dx.doi.org/10.4161/2162402X.2014.990773
- Kikuchi Y, Oomori K, Kizawa I, Kato K. The effect of cimetidine on natural killer activity of peripheral blood lymphocytes of patients with ovarian carcinoma. Jpn J Clin Oncol 1985; 15:377-83; PMID:3839542
- Verma C, Kaewkangsadan V, Eremin JM, Cowley GP, Ilyas M, El-Sheemy MA, Eremin O. Natural killer (NK) cell profiles in blood and tumour in women with large and locally advanced breast cancer (LLABC) and their contribution to a pathological complete response (PCR) in the tumour following neoadjuvant chemotherapy (NAC): differential restoration of blood profiles by NAC and surgery. J Transl Med 2015; 13:180; PMID:26040463; http://dx.doi.org/10.1186/s12967-015-0535-8
- Robertson LE, Denny AW, Huh YO, Plunkett W, Keating MJ, Nelson JA. Natural killer cell activity in chronic lymphocytic leukemia patients treated with fludarabine. Cancer Chemother Pharmacol 1996; 37:445-50; PMID:8599867; http://dx.doi.org/10.1007/s002800050410
- Kiyohara T, Taniguchi K, Kubota S, Koga S, Sakuragi T, Saitoh Y. Induction of lymphokine-activated killer-like cells by cancer chemotherapy. J Exp Med 1988; 168:2355-60; PMID:3199072; http://dx.doi.org/10.1084/jem.168.6.2355
- Markasz L, Stuber G, Vanherberghen B, Flaberg E, Olah E, Carbone E, Eksborg S, Klein E, Skribek H, Szekely L. Effect of frequently used chemotherapeutic drugs on the cytotoxic activity of human natural killer cells. Mol Cancer Ther 2007; 6:644-54; PMID:17308061; http://dx.doi.org/10.1158/1535-7163.MCT-06-0358
- Verma R, Foster RE, Horgan K, Mounsey K, Nixon H, Smalle N, Hughes TA, Carter CR. Lymphocyte depletion and repopulation after chemotherapy for primary breast cancer. Breast Cancer Res 2016; 18:10; PMID:26810608; http://dx.doi.org/10.1186/s13058-015-0669-x
- Eissens DN, Schaap NPM, Preijers FWMB, Dolstra H, van Cranenbroek B, Schattenberg AVM, Joosten I, van der Meer A. CD3+/CD19+-depleted grafts in HLA-matched allogeneic peripheral blood stem cell transplantation lead to early NK cell cytolytic responses and reduced inhibitory activity of NKG2A. Leukemia 2010; 24:583-91; PMID:20033055; http://dx.doi.org/10.1038/leu.2009.269
- Vinzenz K, Micksche M. Systemic and regional natural cytotoxicity in patients with head and neck cancer. J Maxillofac Surg 1986; 14:270-5; PMID:3464682; http://dx.doi.org/10.1016/S0301-0503(86)80303-4
- Ferrari de Andrade L, Ngiow SF, Stannard K, Rusakiewicz S, Kalimutho M, Khanna KK, Tey S-K, Takeda K, Zitvogel L, Martinet L et al. Natural killer cells are essential for the ability of BRAF inhibitors to control BRAFV600E-mutant metastatic melanoma. Cancer Res 2014; 74:7298-308; PMID:25351955; http://dx.doi.org/10.1158/0008-5472.CAN-14-1339
- Cooper MA, Fehniger TA, Caligiuri MA. The biology of human natural killer-cell subsets. Trends Immunol 2001; 22:633-40; PMID:11698225; http://dx.doi.org/10.1016/S1471-4906(01)02060-9
- Bryceson YT, March ME, Ljunggren H-G, Long EO. Synergy among receptors on resting NK cells for the activation of natural cytotoxicity and cytokine secretion. Blood 2006; 107:159-66; PMID:16150947; http://dx.doi.org/10.1182/blood-2005-04-1351
- Hu J, Zhu S, Xia X, Zhang L, Kleinerman ES, Li S. CD8+T cell-specific induction of NKG2D receptor by doxorubicin plus interleukin-12 and its contribution to CD8+T cell accumulation in tumors. Mol Cancer 2014; 13:34; PMID:24565056; http://dx.doi.org/10.1186/1476-4598-13-34
- Fregni G, Perier A, Pittari G, Jacobelli S, Sastre X, Gervois N, Allard M, Bercovici N, Avril M-F, Caignard A. Unique functional status of natural killer cells in metastatic stage IV melanoma patients and its modulation by chemotherapy. Clin Cancer Res 2011; 17:2628-37; PMID:21224372; http://dx.doi.org/10.1158/1078-0432.CCR-10-2084
- Kiaii S, Choudhury A, Mozaffari F, Rezvany R, Lundin J, Mellstedt H, Osterborg A. Signaling molecules and cytokine production in T cells of patients with B-cell chronic lymphocytic leukemia: long-term effects of fludarabine and alemtuzumab treatment. Leuk Lymphoma 2006; 47:1229-38; PMID:16923551; http://dx.doi.org/10.1080/10428190600565503
- Song H, Kim J, Cosman D, Choi I. Soluble ULBP suppresses natural killer cell activity via down-regulating NKG2D expression. Cell Immunol 2006; 239:22-30; PMID:16630603; http://dx.doi.org/10.1016/j.cellimm.2006.03.002
- Raffaghello L, Prigione I, Airoldi I, Camoriano M, Levreri I, Gambini C, Pende D, Steinle A, Ferrone S, Pistoia V. Downregulation and/or release of NKG2D ligands as immune evasion strategy of human neuroblastoma. Neoplasia 2004; 6:558-68; PMID:15548365; http://dx.doi.org/10.1593/neo.04316
- Parkhurst MR, Riley JP, Dudley ME, Rosenberg SA. Adoptive transfer of autologous natural killer cells leads to high levels of circulating natural killer cells but does not mediate tumor regression. Clin Cancer Res 2011; 17:6287-97; PMID:21844012; http://dx.doi.org/10.1158/1078-0432.CCR-11-1347
- Burns LJ, Weisdorf DJ, DeFor TE, Repka TL, Ogle KM, Hummer C, Miller JS. Enhancement of the anti-tumor activity of a peripheral blood progenitor cell graft by mobilization with interleukin 2 plus granulocyte colony-stimulating factor in patients with advanced breast cancer. Exp Hematol 2000; 28:96-103; PMID:10658681; http://dx.doi.org/10.1016/S0301-472X(99)00129-0
- Escudier B, Farace F, Angevin E, Charpentier F, Nitenberg G, Triebel F, Hercend T. Immunotherapy with interleukin-2 (IL2) and lymphokine-activated natural killer cells: improvement of clinical responses in metastatic renal cell carcinoma patients previously treated with IL2. Eur J Cancer 1994; 30A:1078-83; PMID:7654433; http://dx.doi.org/10.1016/0959-8049(94)90460-X
- Lister J, Rybka WB, Donnenberg AD, deMagalhaes-Silverman M, Pincus SM, Bloom EJ, Elder EM, Ball ED, Whiteside TL. Autologous peripheral blood stem cell transplantation and adoptive immunotherapy with activated natural killer cells in the immediate posttransplant period. Clin Cancer Res 1995; 1:607-14; PMID:9816022
- Ishikawa E, Tsuboi K, Saijo K, Harada H, Takano S, Nose T, Ohno T. Autologous natural killer cell therapy for human recurrent malignant glioma. Anticancer Res 2004; 24:1861-71; PMID:15274367
- Macdonald HR, Engers HD, Cerottini JC, Brunner KT. Generation of cytotoxic T lymphocytes in vitro. II. Effect of repeated exposure to alloantigens on the cytotoxic activity of long-term mixed leukocyte cultures. J Exp Med 1974; 140:718-30; PMID:4278109; http://dx.doi.org/10.1084/jem.140.3.718
- Prinz PU, Mendler AN, Masouris I, Durner L, Oberneder R, Noessner E. High DGK-α and disabled MAPK pathways cause dysfunction of human tumor-infiltrating CD8+ T cells that is reversible by pharmacologic intervention. J Immunol 2012; 188:5990-6000; PMID:22573804; http://dx.doi.org/10.4049/jimmunol.1103028