ABSTRACT
Realizing the basis for generating long-lasting clinical responses in cancer patients after therapeutic vaccinations provides the means to further ameliorate clinical efficacy. Peptide cancer vaccines stimulating CD4+ T helper cells are often promising for inducing immunological memory and persistent CD8+ cytotoxic T cell responses. Recent reports from our clinical trial with the AE37 vaccine, which is a HER2 hybrid polypeptide, documented its efficacy to induce CD4+ T cell immunity, which was associated with clinical improvements preferentially among HLA-DRB1*11+ prostate cancer patients. Here, we performed in-depth investigation of the CD4+ T cell response against the AE37 vaccine. We used the DR11/AE37 tetramer in combination with multicolor flow cytometry to identify and characterize AE37-specific CD4+ T cells regarding memory and Tregs phenotype in HLA-DRB1*11+ vaccinated patients. To verify vaccine-specific immunological memory in vivo, we also assessed AE37-specific CD4+ T cells in defined CD4+ memory subsets by cell sorting. Finally, vaccine-induced AE37-specific CD4+ T cells were assessed regarding their functional profile. AE37-specific memory CD4+ T cells could be detected in peptide-stimulated cultures from prostate cancer patients following vaccination even 4 y post-vaccination. The vast majority of vaccine-induced AE37-specific CD4+ T cells exhibited a multifunctional, mostly Th1 cytokine signature, with the potential of granzyme B production. In contrast, we found relatively low frequencies of Tregs among AE37-specific CD4+ T cells. This is the first report on the identification of vaccine-induced HER2-specific multifunctional long-lasting CD4+ T cells in vaccinated prostate cancer patients.
Abbreviations
CM | = | central memory |
DC | = | dendritic cell |
EM | = | effector memory |
HER2 | = | human epidermal growth factor receptor 2 or HER2/neu |
IVS | = | in vitro stimulation |
LAP | = | latency-associated peptide |
LT | = | long term |
LTI | = | long-term immunity |
MHC | = | major histocompatibility complex |
PBMCs | = | peripheral blood mononuclear cells |
PVS | = | primary vaccination series |
TCR | = | T cell receptor |
Tregs | = | regulatory T cells |
Tscm | = | stem cell memory T cells |
Introduction
Complementation of conventional cancer therapies through active immunization has been explored as a modality to prevent recurrences leading to disease stabilization.Citation1,2 Initially, there was preferential attention to vaccine-induced antitumor responses by CD8+ cytotoxic T lymphocytes (CTL). However, accumulating evidence later on suggested that CD4+ helper T cells (Th) represent an essential component of the antitumor immune response.Citation3 Therefore, improved peptide vaccines focused on stimulating Th aiming at generating tumor-specific immune memory and durable stimulation of CTL.Citation2
On this basis, significant improvement was achieved using therapeutic vaccines encompassing in their sequence naturally occurring and overlapping immunogenic epitopes, recognized by both Th and CTL.Citation4,5 Arguably, most direct data on the relevance of Th, specifically recognizing MHC-class II restricted peptides in human tumors, comes from a rather restricted number of clinical studies on vaccines consisting of recombinant proteins, polypeptides, or mixtures of peptides.Citation6 Combined with data demonstrating that intratumoral accumulation of effector CD8+ as well as memory CD4+ T cells predicts survival in various cancer types Citation7,8 and that T cell-based pre-existent immunity in cancer patients is directed against MHC-class I and class II tumor-associated antigens, including neo-antigens,Citation9 it is conceivable that Th have a central role in antitumor immunity. Therefore, targeting MHC class II-restricted antigens may significantly improve the therapeutic efficacy of cancer vaccines.Citation10
Stimulating CD4+ T cells is considered to be essential for long-lasting immunity. However, there have been concerns implicating subsets of these cells with active suppression.Citation11 Such T regulatory cells (Tregs) are clinically relevant due to their ability to suppress antitumor immune responses.Citation12 Moreover, their presence, either systemic or at the tumor sites, is often associated with poor prognosis.Citation13,14 It is possible that Tregs may be active in cancer patients particularly upon recognition of tumor peptides included in a vaccine formulation. Consequently, expanded populations of Tregs may have potent negative feedback by suppressing vaccine-induced T cell-based antitumor immunity. Therefore, vaccine evaluation requires monitoring not only effector CD4+ T cells but also tumor antigen-specific Tregs. Such immunomonitoring should constitute a key part of any immunotherapeutic approach aiming at generating tumor antigen-specific CD4+ T cells. In this context, workshops on cancer immunotherapy trials have concluded that immunomonitoring should include functional assays, such as cytokine intracellular staining flow cytometry in combination with multimer staining assessing antigen-specificity.Citation15 Fluorescent MHC-peptide multimers (i.e., tetramers, dextramers) offer the advantage of direct visualization of antigen-specific T cells, further contributing to vaccine evaluation and thus to the improvement of vaccine development.Citation16 So far, the specificities of human effector CD4+ T cells and Tregs have been investigated in a restricted number of immunotherapeutic approaches targeting NY-ESO-1,Citation17 MAGE-A3,Citation18 mammaglobin,Citation19 and MELAN-A,Citation20 whereas there are limited studies reporting results on HER2-specific CD4+ T cells in the context of vaccine evaluation.Citation19,21
Our group has evaluated the generation of CD4+ T cells in prostate cancer patients vaccinated with an MHC class II peptide vaccine consisting of a HER2/neu peptide (776–790) (the native peptide or AE36) hybridized to the Ii-Key tetrapeptide (LRMK) of the HLA class II-associated invariant chain.Citation22-24 The Ii-Key modification enhances antigen presentation by facilitating epitope interaction with the class II molecule (the hybrid peptide Ii-key/AE36, or AE37).Citation24 Results from our phase I study showed that AE37 vaccination was safe and could induce HER2-specific immune responses, by stimulating both CD4+ and CD8+ T cells, in vaccinated prostate cancer patients.Citation21 Our findings from the follow-up immunological assessment showed that the AE37 vaccine could generate vaccine-specific responses (assessed by IFNγ ELISPOT, proliferation and DTH), which could be detected even more than 3 y after initiation of inoculations, in vaccinated prostate cancer patients.Citation22 In addition to this, results from our recent retrospective analysis showed that patients expressing HLA-DRB1*11 responded immunologically, with increased CD4+ T cell-derived IFNγ secretion, and clinically to AE37 vaccination.Citation25 Herein, we assessed the ability of the AE37 vaccine to specifically induce functional memory CD4+ T cells in vaccinated patients using an MHC class II/peptide tetramer in combination with multiparameter flow cytometry. In this context, we also investigated the ability of AE37 to induce immunosuppressive Tregs.
Results
Analysis of AE37-stimulated cultures from vaccinated patients using the DR11/AE37 tetramer
We initially evaluated vaccine-induced CD4+ T cells in immunized HLA-DRB1*11+ patients, by staining post-vaccination samples previously stimulated in vitro with AE37 peptide for 10 d, with the DR11/AE37 tetramer. As illustrated in , AE37-stimulation for 10 d resulted in expansion of CD4+ T cells in culture reaching levels >90%. The same figure describes a representative example of the gating strategy followed for the identification of DR11/AE37 CD4+ T cells. In this particular case of patient no.10 (PR10) (also a high responder to the vaccine), PBMCs collected 2 mo after initiation of vaccinations, just before the 2nd injection (see also Fig. S1), were stimulated with the AE37 peptide. At day 10 of stimulation, expanded CD4+ T cells were analyzed using DR11/AE37 tetramer and a high proportion of DR11/AE37+CD4+ T cells (74.7%) was detected (). presents the levels of AE37-specific CD4+ T cells detected in peptide-stimulated cultures using the DR11/AE37 tetramer, from all patients analyzed, at pre-vaccination and various timepoints during and post-vaccinations. This figure clearly shows that only marginal levels of DR11/AE37+ CD4+ T cells could be detected before the beginning of vaccinations, with two patients actually displaying measurable frequencies (patients PR14 and PR15; see also , upper panel). During vaccinations, we could detect significant proportions of DR11/AE37+ CD4+ T cells in cultures from all patients (in patients PR10 and PR12 at considerably increased frequencies as compared to patients PR14 and PR16). , also shows that DR11/AE37+ CD4+ T cells were long-lived since these could be detected in fairly significant proportions even longer than 3 y (LTI) post-AE37 booster (patients PR14 and PR15). A striking example of this is provided by the analyses performed with patient's PR14 CD4+ T cells, which had varying proportions of DR11/AE37+ CD4+ T cells ranging from 8% (R5) to 20% (R3) during vaccinations while at LTI (i.e., almost 4 y after the booster inoculation) a significant proportion (7%) of such vaccine-specific CD4+ T cells was still detectable. Patient PR15 had also increased levels of DR11/AE37+ CD4+ T cells at LTI () but no comparisons could be made with earlier timepoints due to restricted availability of blood samples. , shows detailed analyses of patients PR14 during (R2) and PR15 post-vaccinations (LTI) respectively, as well as, at pre-vaccination (R0), where the AE37-specific CD4+ T cells in IVS cultures were at barely detectable levels.
Figure 1. Detection of DR11/AE37 CD4+ T cells in peptide-stimulated cultures from PBMCs of DRB1*11+ prostate cancer patients following vaccination. (A) PBMCs from patient no.10 (PR10), isolated from a blood sample collected during vaccinations (after 1st vaccine inoculation; R2), were assessed with DR11/AE37 tetramer following in vitro AE37-stimulation and analyzed by flow cytometry. According to the representative example of the gating strategy depicted, DR11/AE37 tetramer+ cells were identified in CD4+ T lymphocytes, after exclusion of doublets, dead, and generally non-specifically binding cells. An FMO (fluorescence minus one) control was used to determine tetramer positivity. Final dot plot presents DR11/A37+ T cells gated on total alive CD4+ T cells (after exclusion of CD14+CD16+CD19+cells). (B) Pre-vaccination, during and post-vaccination PBMCs' samples from HLA-DRB1*11+ patients, were stimulated in vitro with AE37 peptide, followed by DR11/AE37 tetramer and surface mAbs staining and analyzed by flow cytometry. Graph presents proportions of DR11/AE37+CD4+ T cells detected in all patients analyzed at pre-vaccination, during and post-vaccination, at the timepoints indicated. (C) Dot plots and proportions of DR11/AE37+ CD4+ T cells, from patients PR14 and PR15, detected in AE37-stimulated cultures from blood samples collected at the indicated timepoints.
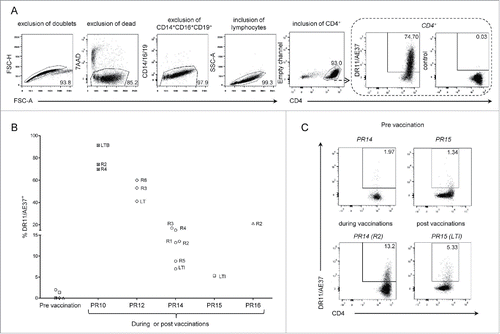
DR11/AE37+ CD4+ T cells belong to the memory repertoire
We have recently reported that our AE37-vaccinated prostate cancer patients demonstrated significant IFNγ responses, as well as, DTH reactions upon re-challenge with AE37, even 3 y post-AE37 booster injection.Citation23 These data attest to the ability of the AE37 vaccine to induce durable immunological memory. Based on these findings, we, herein, analyzed AE37-expanded CD4+ T cells regarding their memory status along with their antigen specificity using DR11/AE37 tetramer. As illustrated in , AE37 in vitro stimulation favored the expansion of effector memory (EM) CD4+T cells. As depicted in , the vast majority of CD4+ T cells from patient PR14 (at LTI) after the 10-d stimulation period, displayed central memory (CM) and EM phenotypes, of which a substantial proportion could be stained positive with DR11/AE37 tetramer. shows DR11/AE37+ cells as percentages of CM and EM CD4+ T cells from all patients tested. Tetramer positive CD4+ T cells were detected in the specific memory subsets in all patients analyzed at various proportions, ranging from 14–88% in CM and from 9–66% in EM. It is also noteworthy that we could still detect vaccine-specific CM and EM CD4+ T cells, albeit at low frequencies, in two of our patients (PR14 and PR15) who were tested 4 or 3 y after booster injection (see also ), respectively, further supporting the concept of giving booster injections at regular timepoints for maintaining immune memory.
Figure 2. Detection of vaccine-specific memory CD4+ T cells. (A) Assessment of total number of Central memory-CM (CD45RA−CCR7+CD28+) and Effector memory-EM (CD45RA−CCR7−CD28+) CD4+ T cells before (ex vivo) and after AE37 peptide in vitro stimulation, from DRB1*11+ vaccinated patients. (B) Gating strategy for identification of CM and EM CD4+ T cells in AE37 in vitro stimulated samples after vaccination. Alive CD4+ T cells, after exclusion of CD14+CD16+CD19+ cells were gated according to CD45RA and CCR7 expression. Subsequent gating based on CD28 expression identified CM and EM cell subsets, which were analyzed for vaccine specificity using DR11/AE37 tetramer. Dot plots of PR14 with samples collected 4 y post-vaccinations are presented. (C) Shown are percentages of CM and EM DR11/AE37+ CD4+ T cells for all patients analyzed.
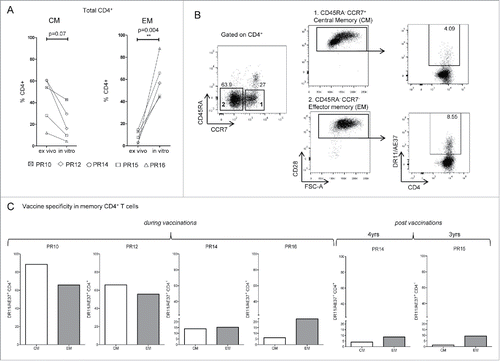
Assessment of AE37-specific CD4+ T cells in populations of defined phenotype confirms vaccine-induced immunological memory in vivo
Based on the data previously reported, AE37-specific CD4+ T cells were induced by vaccinations and share a memory phenotype. However, since AE37-specific CD4+ T cells were detected and characterized in post-vaccination samples after peptide-stimulation, the possibility of in vitro priming is not negligible. Thus, in order to confirm the vaccine-induced immunological memory in vivo, we decided to assess AE37-specific CD4+ T cells in sorted CD4+ T cell memory subpopulations. To this purpose, PBMCs from the vaccinated patient PR14 at LTI(see also ) were stained with specific mAbs including also CD45RA, CCR7, and CD28, whose expression defines certain circulating CD4+ T cell subsets, of diverse in vivo differentiation stages.Citation26,27 After gating on alive CD3+CD4+ T cells, we isolated naive cells (CD45RA+CCR7+CD28+) and antigen-experienced subpopulations, including central (CD45RA−CCR7+CD28+) and effector (CD45RA−CCR7−CD28+) memory cells, using flow cytometric cell sorting (). Sorted subpopulations were stimulated with AE37 peptide loaded DCs for 8 d and antigen specificity was assessed using DR11/AE37 tetramer, as described above. As illustrated in , vaccine-induced AE37-specific CD4+ T cells could be detected among central and effector memory cells, at 6.76% and 11.2% respectively. At relatively lower levels (4.01%), AE37-specific CD4+ T cells were identified in naive cells following in vitro stimulation. Further phenotypic analysis showed that a significant proportion of stem cell memory T cells (Tscm) (CD45RA+CCR7+CD28+CD95+) was present after the AE37 stimulation of naive cells, but not of the sorted central and effector memory subsets (Fig. S2).
Figure 3. Assessment of DR11/AE37 CD4+ T cells in naive and memory subsets. (A) Purified naive and memory CD4+ T cell populations were isolated from circulating peripheral lymphocytes, from patient PR14, 4 y post-vaccinations by FACS cell sorting. Sorting strategy included separation of three defined CD4+ T cells subsets: (1) naive (CD45RA+CCR7+CD28+) and memory subsets (2) CM (CD45RA−CCR7+CD28+) and (3) EM (CD45RA−CCR7−CD28+). (B) Sorted populations were stimulated in vitro with the AE37 peptide and assessed at day 8 with DR11/AE37 tetramer as in .
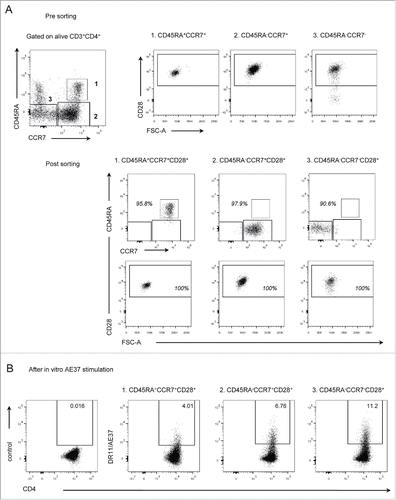
Functional assessment of AE37-specific CD4+ T cells by ICS
AE37-responsive CD4+ T cells were further assessed based on their capacity to specifically produce cytokines in response to AE37. For this, after IVS, CD4+ T cells were tested by multiparameter ICS for cytokine production upon 16 h stimulation with AE37-pulsed DCs. We could detect a significant proportion of CD4+ T cells from patient PR16 producing TNFα, IFNγ, and IL2, but only few producing IL10, IL17, or IL4 (), supporting the notion that the vast majority of AE37-specific CD4+ T cells are Th1 effectors. GzB production was tested as a surrogate for cytotoxic capacity. Interestingly, a substantial proportion of AE37-responsive CD4+ T cells showed expression of GzB (). , shows a data summary from all patients analyzed. Apparently, TNFα was predominantly produced (median: 35.50%, range: 25.17–63.8% of AE37-stimulated CD4+ T cells) followed by IFNγ (25.30%, range: 8.31–61.8%) and IL2 (23.69%, range: 3.80–34.35%). Only patient PR15 had a substantial proportion of CD4+ T cells producing IL4 (26.5%) whereas both IL17 and IL10 were produced at marginal levels by all patients. For patients PR14 and 15, GzB+ cells were detected among AE37-stimulated CD4+ T cells at a frequency of 16% and 22%, respectively; for the rest of the patients, frequencies of GzB+ CD4+ T cells ranged from 3.6–6.7% (). The graph in summarizes the percentages (median values) of AE37-stimulated CD4+ T cells producing the various cytokines and GzB from all patients analyzed.
Figure 4. Functional profile of AE37-responsive CD4+ T cells. (A) After 10 d of in vitro stimulation, AE37-expanded CD4+ T cells were restimulated for 16 h with AE37 peptide-loaded DCs and cytokine/GzB production was assessed with intracellular staining. Unloaded DCs were used for determination of background cytokine and GzB production. An example of dot plots from PR16 presents the percentages of cytokine or GzB producing CD4+ T cells. (B) Data summary from for all patients analyzed. Blood samples were collected during (PR10, PR12, PR16) and post (PR14, PR15) vaccinations. (C) Cumulative results (%) (Median values) of CD4+ T cells producing the indicated cytokines, from all patients analyzed, are shown.
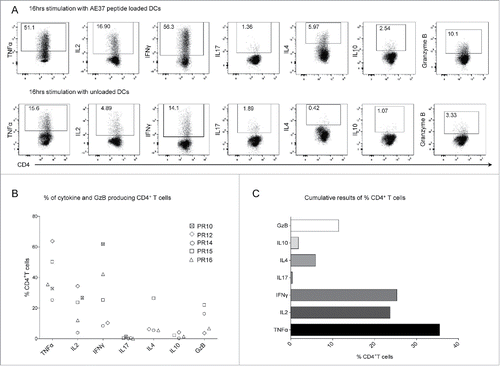
To assess CD4+ CM and EM subsets functionally, AE37-stimulated CD4+ T cell cultures were stained for memory markers in combination with ICS followed by flow cytometry analysis. Our results (Fig. S3) allowed us to draw some clear correlates: first, the main body of IFNγ, TNFα, and IL2 were produced by CM (CD45RA−CCR7+CD28+) and EM (CD45RA−CCR7−CD28+) cells; second, there was only negligible production of IL10 and IL17 by these subsets, whereas production of IL4 was only prominent in PR15 and produced mainly by EM cells; third, GzB was produced at higher levels by EM than CM cells.
Multifunctional CD4+ T cells are induced by the AE37 vaccine
Multiparameter flow cytometry led to a better understanding of the functional capacity of effector and memory T cell responses, thereby contributing to improvements in therapeutic vaccine strategies for cancer.Citation28 Moreover, the expansion of multifunctional T cells is associated with protective vaccine-induced antitumor immunity.Citation29 We, therefore, examined the multifunctionality of AE37-stimulated CD4+ T cells from our vaccinated patients focusing on the production of IL2, IFNγ, TNFα, and IL17, which were included in the same ICS panel. , shows the proportions of CD4+ T cells which were stained positive for between one to four cytokines after stimulation with AE37. Statistic pie charts clearly demonstrate that there was an increase in the proportion of cells positive for two cytokines. This stimulated CD4+ T population was further enriched for cells producing three cytokines (). , shows that in all four patients analyzed, the most prominent proportion of cells scored positive for TNFα and either IL2 or IFNγ. An increased proportion of cells producing three cytokines (i.e., TNFα, IL2, and IFNγ) was evident in three of four patients, whereas in single cytokine analyses, higher proportions of IFNγ-producing CD4+ T cells could be detected in two patients (PR10 and PR16). Prominent levels of single IL2 or TNFα production could be detected in patients PR15 and PR14, respectively (). Finally, only marginal IL17 production was noticed in CD4+ T cells scoring positive for one to three cytokines. Four-cytokine producing cells could be detected at negligible proportions (PR10 exhibited the highest percentage of 1.8%) (), and therefore no further analysis was performed regarding four cytokine combination.
Figure 5. Multifunctional profile of AE37-responsive CD4+ T cells. (A) Pie charts show percentages of AE37-stimulated CD4+ T cells (10-d stimulation cultures) producing 1, 2, 3, or 4 cytokines among the total CD4+ T cell population. (B) Graph presents the proportions (%) of cytokine combinations that were evaluated in AE37-responsive CD4+ T cells. Multifunctional cytokine analysis was performed after stringent gating of each cytokine positive population and subsequent Boolean gating using FlowJo software.
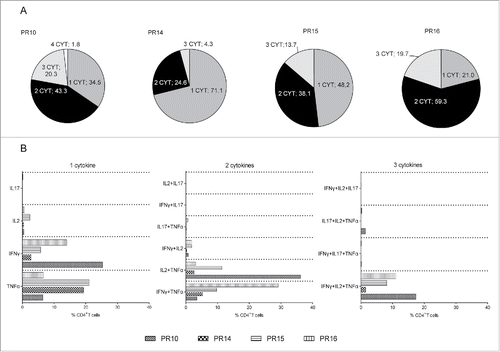
Assessment of CD4+ Tregs among vaccine-induced DR11/AE37+ CD4+cells
There is always a concern that extended exposure to an antigen through repeated administration of peptide injections can be associated with an increase of Tregs capable of suppressing antitumor activity, which would suggest that vaccination approaches could have the potential to induce tolerance instead of effector immunity against the tumor. The concept that Tregs may in fact possess TCRs-recognizing tumor antigens Citation12 implies that tumor-induced Tregs can expand specifically after immunization with anticancer vaccines. Thus, the potential for induction of Tregs by cancer vaccines is a significant issue, indicating the assessment of vaccine-induced Tregs as part of the vaccine evaluation process. In this context, we investigated the population of LAP+Tregs among AE37-stimulated and expanded CD4+ T cells in vitro from our vaccinated patients. Peptide-stimulated cultures, established from post-vaccination PBMCs samples after 10-d stimulation with AE37 peptide, were stained with the DR11/AE37 tetramer followed by staining with mAbs specific for CD25, CD127, and the LAP (latency-associated peptide) molecule (). Phenotypic characterization of Tregs using the combination CD4+CD25+CD127low/neg Citation30-32 does not apply in case of activated Tregs, since in vitro activated T cells acquire CD25 and lose CD127 expression. This was also verified in our stimulated samples, where almost >99% of CD4+ T cells were CD25+CD127low/neg, thereby making it difficult to distinguish Tregs. Hence, we identified a population of Tregs using LAP, a molecule that binds to the surface TGFβ and has been used for the assessment of activated Τregs in previous studies.Citation31,33 Tregs were assessed as CD25+LAP+ in DR11/AE37+CD4+ T cells. As illustrated in the representative example in , the analysis using LAP molecule identified DR11/AE37+CD4+CD25+LAP+ cells that comprised approximately 5% of DR11/AE37+CD4+ T cells. By applying this gating strategy, we could identify LAP+ Tregs among our vaccinated patients, which ranged from 0.19% to 8.22% of DR11/AE37+ CD4+ T cells ().
Figure 6. Assessment of Tregs in vaccine-specific CD4+ T cells. (A) Gating strategy: DR11/AE37+ cells were initially gated on live CD4+ T cells, after exclusion of CD14+CD16+CD19+ cells. Subsequent gating on tetramer+ cells, identified CD25+CD127low/neg cells and DR11/AE37+CD25+LAP+ T cells (Tregs). Dot plots from patient PR10 are presented as an example. (B) Percentages of DR11/AE37+CD4+CD25+LAP+ T cells in 10-d AE37-stimulated CD4+ T cells in all patients analyzed. Representative data at one timepoint during vaccinations with the highest Treg frequency for each patient are shown (PR10_R2, PR12_R6, PR14_R2, PR16_R2, and PR15_LTI).
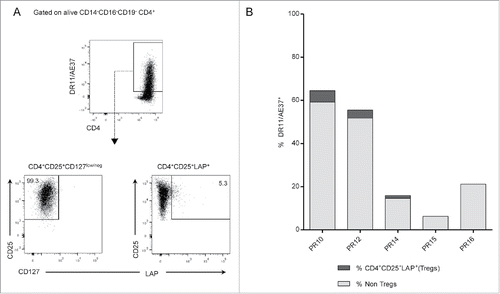
Discussion
Given the fact that detection of antigen-specific T cells may be indicative for the immunological potency of a vaccine, our data provide strong evidence for the capacity of AE37 vaccine to induce HER2-specific CD4+ T cells in vaccinated prostate cancer patients. The analyses performed from the available samples clearly demonstrated that AE37 vaccination could efficiently result in vaccine-specific CD4+ T cells persisting even 4 y post-AE37 booster inoculation. In fact, we detected DR11/AE37-specific CD4+ T cells in peptide-stimulated cultures from vaccinated patients, with various clinicopathological characteristics. It is noteworthy that, pre-vaccination samples, stimulated under the same conditions, did not expand or resulted in barely detectable levels of specific CD4+ T cells (patients PR14 and PR15), further supporting that DR11/AE37 tetramer+ cells, detected mainly during inoculations, had actually been induced by AE37 vaccinations.
We have previously reported that AE37 vaccination can induce specific immune responses, that can be sustained even 3 y post-booster inoculation,Citation22 demonstrating the ability of the AE37 vaccine to induce durable immunological memory. In line with this, assessment of the memory status and antigen specificity of AE37 responsive CD4+ T cells, showed various levels of vaccine-induced specific CD4+ T cells, among CM and EM CD4+ T cells. Thus, we hypothesize that vaccinated patients have developed vaccine-specific memory T cells that upon re-exposure to the specific antigen can be differentiated into effectors and EM cells.Citation34,35 In support to this, we detected AE37-specific T cells among CD4+ T cell populations of defined memory phenotype in the same vaccinated patient PR14, 4 y after booster inoculation (same timepoint as the one depicted in ). Our results clearly demonstrate that AE37 vaccine can actually induce in vivo specific immunological memory in vaccinated patients. Lower levels of DR11/AE37 tetramer+ CD4+ T cells were also detected among naive cells. Although the applied culture conditions do not favor the in vitro priming, we could speculate that the Ii-key modification might be possible to compensate for that, since it allows direct charging and long-lasting binding of the linked peptide to the MHC molecule.Citation36
The presence of antigen-specific memory T cells may be indicative for the establishment of long-lasting and protective antitumor immunological memory. Based on that, a newly described antigen-experienced T cell population seems to play an important role in the maintenance of the memory repertoire.Citation34 According to previous reports, Tscm is a self-renewing cell population with higher proliferative capacity than CM T cells and capable of reconstituting the full diversity of memory and effector T cells.Citation37,38 These qualities along with the fact that Tscm have also exhibited persistent antitumor immunity in vivo have encouraged current therapeutic strategies to employ Tscm in adoptive immunotherapies for cancer.Citation37,39 Interestingly, we were able to identify a substantial population of Tscm after in vitro stimulation with AE37 of sorted naive cells, not detectable in the memory subset cultures. Thus, with respect to the long-lasting immunity, it would be interesting to investigate whether vaccinated patients have generated AE37-specific Tscm and if these could correlate with the clinical outcome.
According to our results, we detected various levels of DR11/AE37-specific CD4+ T cells restricted to DRB1*11 allele among vaccinated patients analyzed. One reason that could account for this is the differential responses of these patients to the vaccine based on their genetic backgrounds. Given that, although the HLA-DRB1*11 allele has been identified by us as a responder allele,Citation25 it is quite possible that the ability of each patient to respond immunologically to the vaccine is not restricted by just one single HLA allele. Indeed, there have been reports that show associations between clinical benefits with vaccine treatment and expression of more than two HLA-alleles.Citation40-42 Another possibility could be that the various therapies which have been applied to those patients may have quantitatively affected these cells. Finally, differential sequestration to lymphoid organs at the time of blood drawing might also be considered as an additional possibility. Regarding the AE37 vaccine, it is also possible that patients with lower levels of DR11/AE37 CD4+ T cells might have developed additional AE37-specific T cell clones of lower binding affinity that could actually expand owing to the Ii-key modification.Citation43 Furthermore, the range of variation in the patterns of expression of memory phenotypes may be due to the fact that samples were taken at different vaccination cycles. We should also mention that the patients assessed in the present study were at different disease stages (Supplementary Material and Methods Section), which may also explain, at least to a certain degree, the variations in their memory subsets.
Our findings regarding functional assessment, showed that AE37 responsive CD4+ T cells share mainly a Th1 cytokine profile, as they secreted TNFα, IFNγ, and IL2, and low to negligible (even undetectable) levels of IL10 or IL17, while IL4 was evident in only one of the patients tested. It is worth noting that besides Th1 cytokine immune response, AE37 can additionally induce GzB producing CD4+ T cells. CD4+ T cells with cytolytic potential (CD4+ CTLs) have been previously described,Citation44,45 however, there are limited reports on therapeutic cancer vaccine-elicited memory CD4+ CTLs. Direct cytolytic activity of CD4+ T cell clones was noted in a patient with lung cancer who responded clinically upon therapeutic vaccination with a telomerase targeting long peptide vaccine.Citation46 In a previous report, low number of CD4+ CTLs correlated with poor survival in HCC patients, suggesting a potential prognostic value.Citation47 Currently, vaccination strategies against infectious diseases and cancer also aim at inducing CD4+ CTLs.Citation48
Based on the multifunctional analysis, our data provide additional information on the capacity of AE37 to promote the development of multifunctional CD4+ T cells producing more than one inflammatory cytokines that may contribute to strengthen effector antitumor functions. It is noteworthy that, TNFα features among AE37-responsive CD4+ T cells either producing one or more cytokines. This is also in agreement with a recent study, where in patients with solid cancers peptide vaccination-induced specific CD4+ T cells responses constitute of mainly TNFα producing subsets.Citation49 In line with this, a previous study in lung cancer patients, reports the presence of Th1 type vaccine-induced MAGE-3-specific CD4+ T cells, capable of secreting mainly TNFα.Citation50 In support to the important role of TNFα, it has been reported by Reissfelder et al.Citation51 that this particular cytokine might serve as an indicator for intratumoral active tumor-specific T cells and might be a better prognostic biomarker in patients with CRC than the infiltration of effector T cells.
Peptide vaccination against tumor antigens raises concern that tumor-specific Tregs may be also activated, promoting suppression of antitumor immunity. Indeed, several studies have reported induction of Tregs upon peptide vaccination against common cancer antigens.Citation52-54 In line with this, investigating the induction of Tregs upon peptide vaccination is an essential aspect of the vaccine evaluation process. To this aim, we used LAP molecule for the identification of a particular Tregs subpopulation, among vaccine-induced CD4+ T cells expanded after in vitro stimulation with the cognate peptide. In addition to the upregulation of CD25 expression, it is important to mention that expression of FoxP3, a common marker for the identification of Tregs, can also be induced after stimulation of T cells; hence, it is not suitable to distinguish Tregs from activated effector T cells.Citation52,55 On the contrary, LAP molecule permits identification of Tregs after in vitro activation. Based on previous results, LAP can define a population of functional Tregs (CD4+CD25+LAP+) exhibiting suppression of immune responses in vitro.Citation31 In line with this and given the diversity of Tregs subsets, LAP expression correlates with a functional Treg population in the setting of in vitro activation, rendering it suitable in the context of immunomonitoring in patients treated with immunotherapy. Regarding our data, the percentages of LAP+ Tregs identified among vaccine-induced CD4+ T cells were at relatively low levels and did not differ from the levels of Tregs measured in the circulation during phase I clinical trial.Citation21 Therefore, our data of negligible IL-10 and IL-17 secretion by in vitro expanded DR11/AE37-specific T cells, along with the low frequencies of LAP+ Tregs detected in AE37-stimulated cultures, provide evidence that AE37 vaccine, albeit a CD4+ T cell-stimulating vaccine, does not induce the generation of vaccine-specific Tregs.
To conclude, assessment of AE37-specific CD4+ T cells with the DR11/AE37 tetramer confirmed the ability of the AE37 vaccine to induce long-lasting and polyfunctional HER2-specific CD4+ T cells, producing mainly Th1 cytokines and Granzyme B. Further assessment revealed relatively low numbers of regulatory Tregs. Given the limited number of reports, our findings should be considered as supportive for the use of MHC class II/peptide tetramers, in combination with multicolor flow cytometry, for assessment of tumor-specific CD4+ T cells as part of the HER2 peptide vaccine evaluation process. Our data provide the first assessment of long-lasting vaccine-induced CD4+ T cells specific for HER2 oncoprotein in prostate cancer patients vaccinated with the AE37 peptide vaccine.
Materials and methods
Patient samples, cells, and in vitro stimulation (IVS) cultures
Peripheral blood mononuclear cells (PBMCs) were collected from prostate cancer patients enrolled in the phase I clinical trial of vaccination with the AE37 vaccine (Ii-Key/HER2/neu(776–790):Ac-LRMKGVGSPYVSRLLGICL-NH2), EudraCT2006-003299-37.Citation21 Following approval of St Savas Institutional Review Board and written informed consent, patients received 6 monthly inoculations (primary vaccination series; PVS) followed by a single booster AE37 inoculation 6 mo after completion of PVS (Fig. S1).Citation21 A total of 32 prostate cancer patients were enrolled in the study, 29 of them completed PVS, and 23 received one booster inoculation. HLA typing was performed by PCR-SSP (polymerase chain reaction-sequence specific primers).Citation25 IVS was carried out as follows: frozen aliquots of PBMCs from HLA-DRB1*11+ patients were thawed in pre-warmed RPMI 1640 culture medium (Gibco® by Life Technologies, Europe) supplemented with 20% FCS, 0.5 mM L-Glutamine (Gibco® by Life Technologies, Europe), and antibiotic-antimycotic (Gibco® by Life Technologies, Europe, ref:15240-062)], rested overnight and stimulated in CTL-test ™ medium (serum-free culture media C.T.L. Europe GmbH, catalog#CTLT-010) with AE37 peptide (NeoMPS, Inc., USA) (10 μg/mL) in the presence of IL2 (Proleukin, Novartis Hellas) (20 IU/mL), for 10–15 d. We used cryopreserved samples from HLA-DRB1*11+ patients which had been collected at pre-vaccination and at different timepoints during and after vaccinations, based on availability. In the current study, five HLA-DRB1*11+ patients with different clinical characteristics at enrollment, were analyzed (Table S1t).
Identification and assessment of AE37-specific CD4+ T cells
Assessment of AE37-specific CD4+ T cells was performed following IVS. Peptide-stimulated PBMCs cultures were incubated with the tetramer HLA-DRB1*11/LMRK-HER2(776-790)-PE (DR11/AE37) (10 μg/mL) (provided by TC Metrix, Switzerland) for 1 h at 37°C, washed and additional phenotypic staining was performed. Cells were stained for 20 min at 4°C with 7AAD dye (BD Biosciences-Europe) for the exclusion of dead cells and the specific monoclonal antibodies (mAbs) CD14/16/19-FITC (Biolegend-Europe) for the exclusion of monocytes, NK cells, and B cells, respectively, and CD4+-PECy7, CD28-Alexa Fluor700, CCR7-APC, CD45RO-BV605, LAP-APC, CD127-BV605, CD25-BV421, CD45RA-AlexaFluor700 (all from Biolegend, Europe). Cells were washed twice and analyzed by flow cytometry (FACSAria III, BD Biosciences).
Assessment of AE37-specific CD4+ T cells in naive and memory subpopulations
For the cell sorting experiment, PBMCs from a vaccinated patient (PR14, 4 y post-vaccination) were stained with mAbs anti-CD4+-PECy7, anti-CD3-APCCy7, anti-CD45RA-AlexFluor700, anti-CD45RO-PE, anti-CCR7-APC, anti-CD28-PECy5 (7AAD viability dye also included). After gating on alive CD3+CD4+ T lymphocytes, cells were gated in three populations according to CD45RA and CCR7 expression (CD45RA+CCR7+, CD45RA−CCR7+, and CD45RA−CCR7−). Each population was gated according to CD28 positive expression and using flow cytometry (FACSAria III, BD Biosciences) the following subsets were sorted: CD45RA+CCR7+CD28+, CD45RA−CCR7+CD28+, CD45RA−CCR7−CD28+. Sorted populations were stimulated with AE37-loaded dendritic cells (DCs) (5:1) in the presence of IL2(10 IU/mL) (Proleukin, Novartis Hellas) and IL7(10 ng/mL) (R&D Systems, Europe) for 8 d. AE37-specific CD4+ T cells were identified following DR11/AE37 tetramer staining as detailed above.
Functional profile analysis of AE37-responsive CD4+ T cells
After IVS, CD4+ T cells were stimulated with AE37 peptide loaded-DCs for 16 hours in the presence of Brefeldin A (5μg/ml, Applichem, Bioline Scientific, Europe) and cytokine production was assessed by intracellular cytokine staining (ICS) assay. Functional profile was based on the detection of six cytokines (IL2, IL17A, IFNγ, TNFα, IL4, and IL10) along with the cytolytic mediator Granzyme B (GzB). After restimulation, cells were washed and stained with surface specific mAbs: CD4-APCCy7, CD28-PECy5, CCR7-BV510, and CD45RA-AlexaFluor700 (all from Biolegend, Europe). Staining with CD14/16/19-PerCP (Biolegend, Europe) and 7AAD dye (BD biosciences, Europe) were used for excluding unwanted as well as dead cells (see above also). After surface staining, cells were fixed and permeabilized with Cytofix/CytoPerm (BD biosciences, Europe) and stained with mAbs specific for IL2-PECy7, IL17A-BV421, IFNγ-APC, TNFα-FITC (panel 1), and for IL10-BV421, IL4-PECy7 (all from Biolegend, Europe), and GzB-FITC (BD Biosciences, Europe) (panel 2). Cells were analyzed by flow cytometry (FACSAria III, BD Biosciences). Data analysis was performed with FlowJo software.
Disclosure of potential conflicts of interest
No potential conflicts of interest were disclosed.
KONI_A_1178439_supplementary_materials.zip
Download Zip (231.7 KB)Acknowledgments
We thank Panagiotis Tzonis, MD for the clinical support and Joanne Kalogeropoulou for excellent technical assistance.
References
- Melero I, Gaudernack G, Gerritsen W, Huber C, Parmiani G, Scholl S, Thatcher N, Wagstaff J, Zielinski C, Faulkner I et al. Therapeutic vaccines for cancer: an overview of clinical trials. Nat Rev Clin Oncol 2014; 11:509-24; PMID:25001465; http://dx.doi.org/10.1038/nrclinonc.2014.111
- Rosenberg SA, Yang JC, Restifo NP. Cancer immunotherapy: moving beyond current vaccines. Nat Med 2004; 10:909-15; PMID:15340416; http://dx.doi.org/10.1038/nm1100
- Galaine J, Borg C, Godet Y, Adotevi O. Interest of Tumor-Specific CD4 T Helper 1 Cells for Therapeutic Anticancer Vaccine. Vaccines (Basel) 2015; 3:490-502; PMID:26350591; http://dx.doi.org/10.3390/vaccines3030490
- Butterfield LH. Cancer vaccines. BMJ 2015; 350:h988; PMID:25904595; http://dx.doi.org/10.1136/bmj.h988
- Melief CJ, van der Burg SH. Immunotherapy of established (pre)malignant disease by synthetic long peptide vaccines. Nat Rev Cancer 2008; 8:351-60; PMID:18418403; http://dx.doi.org/10.1038/nrc2373
- Slingluff CL, Jr. The present and future of peptide vaccines for cancer: single or multiple, long or short, alone or in combination? Cancer J 2011; 17:343-50; PMID:21952285; http://dx.doi.org/10.1097/PPO.0b013e318233e5b2
- Fridman WH, Pages F, Sautes-Fridman C, Galon J. The immune contexture in human tumours: impact on clinical outcome. Nat Rev Cancer 2012; 12:298-306; PMID:22419253; http://dx.doi.org/10.1038/nrc3245
- Galon J, Mlecnik B, Bindea G, Angell HK, Berger A, Lagorce C, Lugli A, Zlobec I, Hartmann A, Bifulco C et al. Towards the introduction of the ‘Immunoscore’ in the classification of malignant tumours. J Pathol 2014; 232:199-209; PMID:24122236; http://dx.doi.org/10.1002/path.4287
- Mueller KL. Cancer immunology and immunotherapy. Realizing the promise. Introduction. Science 2015; 348:54-5; PMID:25838372; http://dx.doi.org/10.1126/science.348.6230.54
- Baxevanis CN, Papamichail M, Perez SA. Prostate cancer vaccines: the long road to clinical application. Cancer Immunol Immunother 2015; 64:401-8; PMID:25690791; http://dx.doi.org/10.1007/s00262-015-1667-7
- Ebert LM, MacRaild SE, Zanker D, Davis ID, Cebon J, Chen W. A cancer vaccine induces expansion of NY-ESO-1-specific regulatory T cells in patients with advanced melanoma. PloS One 2012; 7:e48424; PMID:23110239; http://dx.doi.org/10.1371/journal.pone.0048424
- Oleinika K, Nibbs RJ, Graham GJ, Fraser AR. Suppression, subversion and escape: the role of regulatory T cells in cancer progression. Clin Exp Immunol 2013; 171:36-45; PMID:23199321; http://dx.doi.org/10.1111/j.1365-2249.2012.04657.x
- deLeeuw RJ, Kost SE, Kakal JA, Nelson BH. The prognostic value of FoxP3+ tumor-infiltrating lymphocytes in cancer: a critical review of the literature. Clin Cancer Res 2012; 18:3022-9; PMID:22510350; http://dx.doi.org/10.1158/1078-0432.CCR-11-3216
- Idorn M, Kollgaard T, Kongsted P, Sengelov L, Thor SP. Correlation between frequencies of blood monocytic myeloid-derived suppressor cells, regulatory T cells and negative prognostic markers in patients with castration-resistant metastatic prostate cancer. Cancer Immunol Immunother 2014; 63:1177-87; PMID:25085000; http://dx.doi.org/10.1007/s00262-014-1591-2
- Keilholz U, Weber J, Finke JH, Gabrilovich DI, Kast WM, Disis ML, Kirkwood JM, Scheibenbogen C, Schlom J, Maino VC et al. Immunologic monitoring of cancer vaccine therapy: results of a workshop sponsored by the Society for Biological Therapy. J Immunother 2002; 25:97-138; PMID:12074049; http://dx.doi.org/10.1097/00002371-200203000-00001
- Altman JD, Davis MM. MHC-peptide tetramers to visualize antigen-specific T cells. In Current protocols in immunology. eds. John E Coligan et al 2003; Chapter 17:Unit 17.3; Page 1-33
- Ayyoub M, Dojcinovic D, Pignon P, Raimbaud I, Schmidt J, Luescher I, Valmori D. Monitoring of NY-ESO-1 specific CD4+ T cells using molecularly defined MHC class II/His-tag-peptide tetramers. Proc Natl Acad Sci U S A 2010; 107:7437-42; PMID:20368442; http://dx.doi.org/10.1073/pnas.1001322107
- Francois V, Ottaviani S, Renkvist N, Stockis J, Schuler G, Thielemans K, Colau D, Marchand M, Boon T, Lucas S et al. The CD4(+) T-cell response of melanoma patients to a MAGE-A3 peptide vaccine involves potential regulatory T cells. Cancer Res 2009; 69:4335-45; PMID:19435913; http://dx.doi.org/10.1158/0008-5472.CAN-08-3726
- Schmidt HH, Ge Y, Hartmann FJ, Conrad H, Klug F, Nittel S, Bernhard H, Domschke C, Schuetz F, Sohn C et al. HLA Class II tetramers reveal tissue-specific regulatory T cells that suppress T-cell responses in breast carcinoma patients. Onco immunol 2013; 2:e24962; PMID:23894725; http://dx.doi.org/10.4161/onci.24962
- Gajewski TF. Monitoring Specific T-Cell Responses to Melanoma Vaccines: ELISPOT, Tetramers, and Beyond. Clinical and Diagnostic Laboratory Immunology 2000; 7(2):141-144; PMID:10702484
- Perez SA, Kallinteris NL, Bisias S, Tzonis PK, Georgakopoulou K, Varla-Leftherioti M, Papamichail M, Thanos A, von Hofe E, Baxevanis CN. Results from a phase I clinical study of the novel Ii-Key/HER-2/neu(776-790) hybrid peptide vaccine in patients with prostate cancer. Clin Cancer Res 2010; 16:3495-506; PMID:20466887; http://dx.doi.org/10.1158/1078-0432.CCR-10-0085
- Perez SA, Anastasopoulou EA, Papamichail M, Baxevanis CN. AE37 peptide vaccination in prostate cancer: identification of biomarkers in the context of prognosis and prediction. Cancer Immunol Immunother 2014; 63:1141-50; PMID:25052849; http://dx.doi.org/10.1007/s00262-014-1582-3
- Perez SA, Anastasopoulou EA, Tzonis P, Gouttefangeas C, Kalbacher H, Papamichail M, Baxevanis CN. AE37 peptide vaccination in prostate cancer: a 4-year immunological assessment updates on a phase I trial. Cancer Immunol Immunother 2013; 62:1599-608; PMID:23934022; http://dx.doi.org/10.1007/s00262-013-1461-3
- Perez SA, Peoples GE, Papamichail M, Baxevanis CN. Invariant chain-peptide fusion vaccine using HER-2/neu. Methods Mol Biol 2014; 1139:321-36; PMID:24619690; http://dx.doi.org/10.1007/978-1-4939-0345-0_26
- Anastasopoulou EA, Voutsas IF, Keramitsoglou T, Gouttefangeas C, Kalbacher H, Thanos A, Papamichail M, Perez SA, Baxevanis CN. A pilot study in prostate cancer patients treated with the AE37 Ii-key-HER-2/neu polypeptide vaccine suggests that HLA-A*24 and HLA-DRB1*11 alleles may be prognostic and predictive biomarkers for clinical benefit. Cancer Immunol Immunother 2015; 64:1123-36; PMID:26026288; http://dx.doi.org/10.1007/s00262-015-1717-1
- Amyes E, McMichael AJ, Callan MF. Human CD4+ T cells are predominantly distributed among six phenotypically and functionally distinct subsets. J Immunol 2005; 175:5765-73; PMID:16237068; http://dx.doi.org/10.4049/jimmunol.175.9.5765
- Sallusto F, Geginat J, Lanzavecchia A. Central memory and effector memory T cell subsets: function, generation, and maintenance. Annu Rev Immunol 2004; 22:745-63; PMID:15032595; http://dx.doi.org/10.1146/annurev.immunol.22.012703.104702
- Attig S, Hennenlotter J, Pawelec G, Klein G, Koch SD, Pircher H, Feyerabend S, Wernet D, Stenzl A, Rammensee HG et al. Simultaneous infiltration of polyfunctional effector and suppressor T cells into renal cell carcinomas. Cancer Res 2009; 69:8412-9; PMID:19843860; http://dx.doi.org/10.1158/0008-5472.CAN-09-0852
- Seder RA, Darrah PA, Roederer M. T-cell quality in memory and protection: implications for vaccine design. Nat Rev Immunol 2008; 8:247-58; PMID:18323851; http://dx.doi.org/10.1038/nri2274
- Liu W, Putnam AL, Xu-Yu Z, Szot GL, Lee MR, Zhu S, Gottlieb PA, Kapranov P, Gingeras TR, Fazekas de St GB et al. CD127 expression inversely correlates with FoxP3 and suppressive function of human CD4+ T reg cells. J Exp Med 2006; 203:1701-11; PMID:16818678; http://dx.doi.org/10.1084/jem.20060772
- Sun J, Tang DN, Fu T, Sharma P. Identification of human regulatory T cells in the setting of T-cell activation and anti-CTLA-4 immunotherapy on the basis of expression of latency-associated peptide. Cancer Discov 2012; 2:122-30; PMID:22585857; http://dx.doi.org/10.1158/2159-8290.CD-11-0236
- Yu N, Li X, Song W, Li D, Yu D, Zeng X, Li M, Leng X, Li X. CD4(+)CD25 (+)CD127 (low/-) T cells: a more specific Treg population in human peripheral blood. Inflammation 2012; 35:1773-80; PMID:22752562; http://dx.doi.org/10.1007/s10753-012-9496-8
- Mahalingam J, Lin YC, Chiang JM, Su PJ, Fang JH, Chu YY, Huang CT, Chiu CT, Lin CY. LAP+CD4+ T cells are suppressors accumulated in the tumor sites and associated with the progression of colorectal cancer. Clin Cancer Res 2012; 18:5224-33; PMID:22879386; http://dx.doi.org/10.1158/1078-0432.CCR-12-0211
- Gattinoni L, Lugli E, Ji Y, Pos Z, Paulos CM, Quigley MF, Almeida JR, Gostick E, Yu Z, Carpenito C et al. A human memory T cell subset with stem cell-like properties. Nat Med 2011; 17:1290-7; PMID:21926977; http://dx.doi.org/10.1038/nm.2446
- Restifo NP. Big bang theory of stem-like T cells confirmed. Blood 2014; 124:476-7; PMID:25061170; http://dx.doi.org/10.1182/blood-2014-06-578989
- Humphreys RE, Adams S, Koldzic G, Nedelescu B, von HE, Xu M. Increasing the potency of MHC class II-presented epitopes by linkage to Ii-Key peptide. Vaccine 2000; 18:2693-7; PMID:10781856; http://dx.doi.org/10.1016/S0264-410X(00)00067-0
- Gattinoni L, Klebanoff CA, Restifo NP. Paths to stemness: building the ultimate antitumour T cell. Nat Rev Cancer 2012; 12:671-84; PMID:22996603; http://dx.doi.org/10.1038/nrc3322
- Lugli E, Dominguez MH, Gattinoni L, Chattopadhyay PK, Bolton DL, Song K, Klatt NR, Brenchley JM, Vaccari M, Gostick E et al. Superior T memory stem cell persistence supports long-lived T cell memory. J Clin Invest 2013; 123:594-9; PMID:23281401; http://dx.doi.org/10.1172/JCI66327
- Gattinoni L, Restifo NP. Moving T memory stem cells to the clinic. Blood 2013; 121:567-8; PMID:23349370; http://dx.doi.org/10.1182/blood-2012-11-468660
- Mitchell MS, Harel W, Groshen S. Association of HLA phenotype with response to active specific immunotherapy of melanoma. J Clin Oncol 1992; 10:1158-64; PMID:1607920
- Sonpavde G, Wang M, Peterson LE, Wang HY, Joe T, Mims MP, Kadmon D, Ittmann MM, Wheeler TM, Gee AP et al. HLA-restricted NY-ESO-1 peptide immunotherapy for metastatic castration resistant prostate cancer. Invest New Drugs 2014; 32:235-42; PMID:23609828; http://dx.doi.org/10.1007/s10637-013-9960-9
- Sosman JA, Unger JM, Liu PY, Flaherty LE, Park MS, Kempf RA, Thompson JA, Terasaki PI, Sondak VK. Adjuvant immunotherapy of resected, intermediate-thickness, node-negative melanoma with an allogeneic tumor vaccine: impact of HLA class I antigen expression on outcome. J Clin Oncol 2002; 20:2067-75; PMID:11956267; http://dx.doi.org/10.1200/JCO.2002.08.072
- Xu M, Kallinteris NL, Von HE. CD4+ T-cell activation for immunotherapy of malignancies using Ii-Key/MHC class II epitope hybrid vaccines. Vaccine 2012; 30:2805-10; PMID:22386748; http://dx.doi.org/10.1016/j.vaccine.2012.02.031
- Brown DM. Cytolytic CD4 cells: Direct mediators in infectious disease and malignancy. Cell Immunol 2010; 262:89-95; PMID:20236628; http://dx.doi.org/10.1016/j.cellimm.2010.02.008
- van de Berg PJ, van Leeuwen EM, ten Berge IJ, van LR. Cytotoxic human CD4(+) T cells. Curr Opin Immunol 2008; 20:339-43; PMID:18440213; http://dx.doi.org/10.1016/j.coi.2008.03.007
- Inderberg-Suso EM, Trachsel S, Lislerud K, Rasmussen AM, Gaudernack G. Widespread CD4+ T-cell reactivity to novel hTERT epitopes following vaccination of cancer patients with a single hTERT peptide GV1001. Onco Immunol 2012; 1:670-86; PMID:22934259; http://dx.doi.org/10.4161/onci.20426
- Fu J, Zhang Z, Zhou L, Qi Z, Xing S, Lv J, Shi J, Fu B, Liu Z, Zhang JY et al. Impairment of CD4+ cytotoxic T cells predicts poor survival and high recurrence rates in patients with hepatocellular carcinoma. Hepatol 2013; 58:139-49; PMID:22961630; http://dx.doi.org/10.1002/hep.26054
- Coler R, Hudson T, Hughes S, Huang P, Beebe E, Orr M. Vaccination Produces CD4 T Cells with a Novel CD154-CD40-Dependent Cytolytic Mechanism. J Immunol 2015; 195:3190-7; PMID:26297758; http://dx.doi.org/10.4049/jimmunol.1501118
- Gross S, Lennerz V, Gallerani E, Mach N, Bohm S, Hess D, von BL, Knuth A, Ochsenbein A, Gnad-Vogt U et al. Short Peptide Vaccine Induces CD4+ T Helper Cells in Patients with Different Solid Cancers. Cancer Immunol Res 2016; 4:18-25; PMID:26563311; http://dx.doi.org/10.1158/2326-6066.CIR-15-0105
- Atanackovic D, Altorki NK, Stockert E, Williamson B, Jungbluth AA, Ritter E, Santiago D, Ferrara CA, Matsuo M, Selvakumar A et al. Vaccine-induced CD4+ T cell responses to MAGE-3 protein in lung cancer patients. J Immunol 2004; 172:3289-96; PMID:14978137; http://dx.doi.org/10.4049/jimmunol.172.5.3289
- Reissfelder C, Stamova S, Gossmann C, Braun M, Bonertz A, Walliczek U, Grimm M, Rahbari NN, Koch M, Saadati M et al. Tumor-specific cytotoxic T lymphocyte activity determines colorectal cancer patient prognosis. J Clin Invest 2015; 125:739-51; PMID:25562322; http://dx.doi.org/10.1172/JCI74894
- Bonertz A, Weitz J, Pietsch DH, Rahbari NN, Schlude C, Ge Y, Juenger S, Vlodavsky I, Khazaie K, Jaeger D et al. Antigen-specific Tregs control T cell responses against a limited repertoire of tumor antigens in patients with colorectal carcinoma. J Clin Invest 2009; 119:3311-21; PMID:19809157; http://dx.doi.org/10.1172/JCI39608
- Wang J, Ioan-Facsinay A, van der Voort EI, Huizinga TW, Toes RE. Transient expression of FOXP3 in human activated nonregulatory CD4+ T cells. Eur J Immunol 2007; 37:129-38; PMID:17154262; http://dx.doi.org/10.1002/eji.200636435
- Welters MJ, Kenter GG, Piersma SJ, Vloon AP, Lowik MJ, Berends-van der Meer DM, Drijfhout JW, Valentijn AR, Wafelman AR, Oostendorp J et al. Induction of tumor-specific CD4+ and CD8+ T-cell immunity in cervical cancer patients by a human papillomavirus type 16 E6 and E7 long peptides vaccine. Clin Cancer Res 2008; 14:178-87; PMID:18172269; http://dx.doi.org/10.1158/1078-0432.CCR-07-1880
- Walker MR, Kasprowicz DJ, Gersuk VH, Benard A, Van LM, Buckner JH, Ziegler SF. Induction of FoxP3 and acquisition of T regulatory activity by stimulated human CD4+. J Clin Invest 2003; 112:1437-43; PMID:14597769; http://dx.doi.org/10.1172/JCI19441