ABSTRACT
Recent studies have shown that tumor cells can release autophagosomes, which transport a broad array of biologically active molecules with potential modulatory effects on immune cell functions. In this study, we aimed to investigate the role of tumor cells-released autophagosomes (i.e. TRAP) in regulating B cell differentiation and function. TRAPs from murine tumor cell lines were found to induce splenic B cells to differentiate into IL-10-producing regulatory B cells (Bregs) with a distinct phenotype of CD1d+ CD5+, which could potently inhibit CD8+ and CD4+ T cell responses in IL-10-depedent manner both in vitro and in vivo. Notably, adoptive transfer of TRAP-induced Bregs abrogated the immune response and antitumor effect induced by OVA-loaded DC vaccinations in E.G7-OVA-bearing mouse model. Mechanistic studies revealed that membrane-bound high-mobility group B1 (HMGB1) on the intact TRAPs was crucial for inducing Breg differentiation via the activation of TLR2-MyD88-NF-κB signal pathway in B cells. Moreover, TRAPs enriched from malignant effusions of cancer patients could induce human B cells to differentiate into IL-10-producing B cells with immunoregulatory functions, the frequency of which were positively correlated with the HMGB1 levels on TRAPs. Together, our findings have demonstrated that TRAPs promote the generation of IL-10+ Bregs, which may contribute to the suppression of antitumor immunity.
Introduction
Autophagy represents a major lysosomal pathway for recycling intracellular proteins, lipids, and organelles, which has emerged as a key cellular event in tumorigenesis, and cancer therapy.Citation1 Despite its low basal level in normal cells, autophagy is dramatically induced in tumor cells under stress conditions such as starvation, hypoxia, and growth factor deprivation.Citation2 Upon the induction of autophagy, a broad spectrum of tumor antigens including long-lived proteins, short-lived proteins, and damage-associated molecules are sequestered by double-membrane vesicles termed autophagosomes.Citation3 Subsequently, autophagosomes fuse with lysosomes, where the sequestered contents are degraded and recycled.Citation4 However, recent studies on yeast and tumor cells have suggested that autophagosomes fail to fuse with lysosomes and instead are released into the extracellular milieu by the cells under stress conditions.Citation5,6 Abundant autophagosomes have been detected in gastrointestinal tumors and invasive melanomas. These autophagosomes are associated with tumor cell proliferation, metastasis, and worse prognosis, implying a potential role of autophagosomes in tumor progression and systemic paraneoplastic syndromes.Citation7 Numerous studies over the past decade have focused on the intracellular recycling function of autophagy in cancer,Citation1,6 but little is known about the mechanistic aspects of tumor cell-released autophagosomes (i.e., TRAP) in the modulation of immune cell function and tumor progression.
Interactions between the immune cells and malignant cells play an important role in tumorigenesis. Failure of the immune system to detect and delete transformed cells may lead to cancer development. Tumor cells display multiple immunosuppressive mechanisms to facilitate their escape from immunosurveillance, including activation of negative regulatory pathways,Citation8 specific recruitment of regulatory cell populations, and secretion of immunosuppressive and proapoptotic factors.Citation9,10 Among these secreted factors, membrane vesicles shed from tumor cells have been characterized in recent years for their pleiotropic immunosuppressive effects that are protective and supportive of the tumor progression.Citation11 These membranous organelles, particularly exosomes purified from tumor cell culture supernatants or from cancer patient sera, can expand and promote the suppressive activities of human regulatory T cells (Treg) or myeloid-derived suppressor cells (MDSCs) by upregulating the expression of FasL, interleukin (IL)-10, thus impairing the ability of effector lymphocytes to exert their cytolytic activity on tumor cells.Citation12 Membrane-bounded HSPs and transforming growth factor β1 (TGF-β1) have been identified as critical components in tumor-derived exosomes for the generation of immunosuppressive cells.Citation13,14 Considering the broad array of bioactive molecules incorporated in tumor-released autophagosomes, such as HMGB1, HSP60, HSP90, and S100A8,Citation15 these vacuoles, similar to the functions of exosomes or other microvesicles, might be implicated in the development of immunosuppression associated with advanced cancers.
Bregs, a distinct subset of B cells with immunoregulatory functions, are involved in the pathogenesis of autoimmune diseases and infections.Citation16,17 In SLE patients, Bregs can inhibit both proliferation and production of IFN-γ and TNF-α by CD4+ T cells.Citation18 The suppressive effects of Bregs are mediated by IL-10, the key immunosuppressive cytokine. Besides their regulatory function in autoimmune diseases, Bregs serve as a critical modulator of the antitumor immune response.Citation19 Recent studies on chronic lymphocytic leukemia (CLL) have revealed that malignant IL-10-competent CLL cells resemble Bregs in their phenotype.Citation20 Biragyn and his colleagues have shown that soluble factors secreted by tumor cells can promote the generation of Bregs, which facilitate 4T1 tumor metastasis.Citation21 However, the identity of these factors responsible for promoting the generation of Bregs during cancer progression has remained unclear. Recently, we have shown that the autophagosomes isolated from tumor cells can be up-taken by B cells, resulting in B cell activation, antibody secretion, and cytokine production, especially the high levels of IL-10 production.Citation22
In this study, we confirm the existence of TRAP and find that TRAPs could induce B cells to differentiate into IL-10-producing B cells, which exert regulatory functions both in vitro and in vivo. Mechanistically, we find that HMGB1 on TRAPs surface induces the differentiation of IL-10-producing B cells in a TLR2-MyD88-NF-κB dependent manner. Moreover, adoptive transfer of TRAP-induced Bregs effectively inhibits OVA-loaded DCs-induced antitumor immune responses. These findings have demonstrated a novel function of TRAP in the induction of immunosuppression associated with cancer development.
Results
TRAPs induce IL-10-producing B cell differentiation in vitro and in vivo
To verify the structural features of TRAPs, we first examined TRAPs collected from the culture supernatant of tumor cells by biochemical analysis and electron microscopy (Figs. S1A–C). To examine the potential effect of TRAP on B cell differentiation, murine splenic CD19+ B cells, or splenocytes were cultured with various doses of TRAP for 72 h. ELISA assay showed that TRAP treatment could induce B cells to produce high level of IL-10 in a dose-dependent manner, with a maximal effect detected at a concentration of 3 μg/mL (Fig. S2A). FACS analysis revealed that TRAPs stimulation significantly increased the frequency of IL-10+ CD19+ B cells in the splenocytes (), the elevated levels of IL-10 in culture supernatant were further confirmed using a standard ELISA assay (). Interestingly, an equivalent amount of tumor cell lysate did not induce IL-10 secretion at significant levels (Fig. S2B). More importantly, depletion of TRAPs via ultracentrifugation impaired the ability of tumor cell culture supernatant to induce IL-10 production B cells (Fig. S2C). We also found that IL-10-producing B cells induced by E.G7-OVA-derived TRAPs did not appear to be an isolated phenomenon, as TRAPs derived from a number of murine tumor cells had similar effects on B cell differentiation (Fig. S2D). IL-10 has been considered as a key trait of Breg cells, so we gated IL-10+ CD19+ B cells to analyze cell surface markers in order to characterize Bregs.Citation23 The results showed that TRAP-induced IL-10+ CD19+ B cells were positively stained for CD5 with high levels of CD1d expression, and with slightly upregulated MHC class II ().
Figure 1. TRAPs induce IL-10-producing B cell differentiation in vitro and in vivo. (A) Frequencies of IL-10-producing B cells determined by flow cytometry. Splenocytes were incubated with TRAPs (3 μg/mL) or LPS (10 μg/mL) for 72 h. PIM stimulation was performed for 5 h before IL-10 staining. (B) ELISA determinations of IL-10 secretion in culture supernatants were also shown. (C) Phenotypic analysis of IL-10+ (red line) or IL-10− (green line) B cells from TRAP-treated B cell cultures for 72 h by flow cytometry. Gray shaded histograms indicate the isotype staining. (D and E) Mice were i.v. injected with TRAPs (30 μg per mouse) three times with 1 d of intervals and frequencies of splenic IL-10+ B cells were determined 4 d after last treatment (n = 3; as shown in D), CD1d and CD5 expression was assessed by flow cytometry as previously shown. Representative contour plot showing CD1d and CD5 expression on IL-10+ and IL-10− B cells, as shown in E. (F and G), Mice bearing established Atg5 KD 4T1 tumor or control 4T1 tumor were sacrificed (n = 6) when solid tumor volumes reached approximately 150 mm2, and the frequency of IL-10+ B cells in the tumor tissue (as shown in F) and the draining lymph node (as shown in G) was detected. Data (mean ± s.e.m) are representative of three independent experiments. NS: p > 0.05, * p < 0.05, ** p < 0.01, *** p < 0.001 by unpaired t-test (A, B, D, F and G).
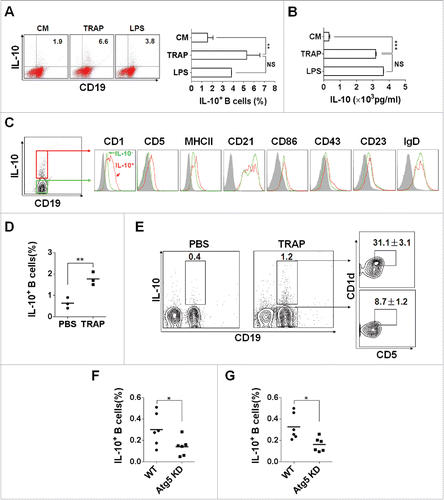
To detect whether TRAPs exerts their effects on B cell differentiation in vivo, TRAPs were i.v. injected into C57BL/6 mice three times with 1 d of intervals and the frequencies and characterizations of IL-10+ CD19+ B cells in the spleen collected at day 7 after last injection were evaluated by flow cytometry. The results showed a ∼2.7-fold increase in the frequencies of IL-10+ CD19+ B cells in the TRAP-injected mice (). Notably, frequencies of CD5+CD1dhigh B cells in TRAP-induced IL-10+ CD19+ B cells were significantly increased compared with IL-10− CD19+ B cells (36.7% vs. 11%) (). Together, these results demonstrated that TRAPs could induce B cells to differentiate into IL-10-producing B cells (Bregs) in vitro and in vivo.
To further examine the role of TRAP in inducing IL-10+ B cells in murine tumor microenvironment, Atg5 knockdown-4T1 cells were established by using lentivirus-mediated siRNA targeting Atg5, a gene essential for autophagosome formation. We found that Atg5 expression was significantly downregulated by siRNA targeting Atg5 compared with matched controls (Fig. S3A). Western-blot analysis for detection of proteins LC3 I and II used as a marker of autophagosomes or TRAPs showed that TRAPs secretion from culture supernatant of the Atg5 KD 4T1 cells were markedly repressed compared with same number of the control 4T1 cells (Fig. S3B). Meanwhile, TRAPs isolated from the centrifugal supernatant of digested tumor tissue established by Atg5 KD 4T1 cells were significantly reduced when compared with control 4T1 cells (Fig. S3C). Further, we found that the frequency of IL-10+ B cells, in the tumor tissue and draining lymph node of Atg5 KD 4T1 tumor model, were reduced when compared to that of the respective control tumor model (). These results suggested that the generation of IL-10+ B cells (Bregs) in murine tumor model was partially associated with the secretory autophagosomes released by tumor cells.
TRAP-induced Bregs exhibit regulatory functions in vitro
To verify whether TRAP-induced Bregs possess a regulatory function, CD4+ T and CD8+ T cells were isolated and stimulated with activating anti-CD3 and anti-CD28 mAb for 4 d in the presence of TRAP-induced Bregs or control B cells. The results showed that the proliferation of CD4+ T and CD8+ T cells were strongly suppressed in the presence of TRAP-induced Bregs but not control B cells (). More importantly, we found that TRAP-induced Bregs, even after separation from T cells by a porous membrane, still had an intact suppressive activity on CD4+ T and CD8+ T cell proliferation. While B cells from IL-10−/− mice, even pre-stimulated by TRAPs, failed to suppress the proliferation of CD4+ T and CD8+ T cells (). Thus, these data suggested that TRAP-induced Bregs could inhibit T cell proliferation via IL-10 but not direct cell–cell contact.
Figure 2. TRAP-induced IL-10-producing B cells suppress T-cell proliferation. (A–D) CFSE-labeled purified CD4+ T cells (A and B) and CD8+ T cells (C and D) were stimulated with plate-bound anti-CD3/anti-CD28 mAb and were either cultured alone (T cells only) or co-cultured with TRAP-induced B cells (3 μg/mL) from WT mice or IL-10−/− mice at ratio of 1:1 for 4 d, un-stimulated T cells were used as control. In the transwell experiment, B cells and T cells were added in the upper and lower chambers, respectively. CFSE dilution was determined by flow cytometry. (E–F) CFSE-labeled splenocytes from naive OT-1 mice were stimulated with OVA257–264 (1 µg/mL) and co-cultured with purified B cells, OVA+ TRAP-induced B cells, or OVA− TRAP-induced B cells at ratio of 1:1. Cells were collected after 4 d, stained with anti-CD8+ mAb and anti-Vβ 5.1/5.2. CFSE dilution was determined by flow cytometry. Results are representative of three independent experiments. NS: p > 0.05, *** p < 0.001 by unpaired t-test (B, D and F).
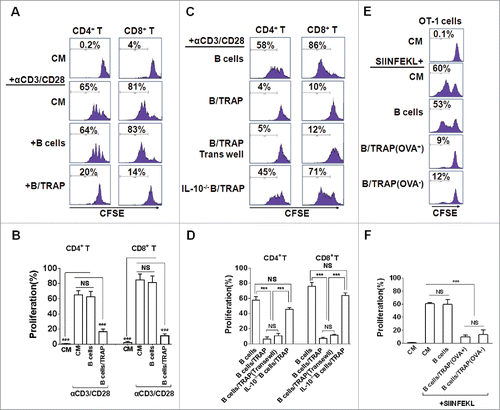
To further determine whether the immunoregulatory function of TRAP-induced Bregs was antigen specific, splenocytes from OT-1 transgenic mice were isolated and then activated using a specific antigen OVA257–264 (SIINFEKL) in the presence of OVA+-TRAP or OVA−-TRAP-induced Bregs. Results showed that OVA−-TRAP-induced Bregs had similar OVA–TRAP-inhibitory effect on T-cell proliferation with the OVA+-TRAP-induced Bregs (), suggesting that TRAP-induced Bregs exhibit their suppressive effect in a non-antigen-specific manner.
TRAP-induced Bregs inhibit DCOVA-induced T cell response and antitumor effect
Next, the potential effects of TRAP-induced Bregs on T cell response and their antitumor effect were assessed. Mice bearing 6-d established E.G7-OVA tumors (s.c.) were vaccinated with OVA-pulsed DCs, and then adoptively transferred (i.v.) with TRAP-induced Bregs. At day 7 after last vaccination, splenocytes harvested from each groups were re-stimulated with OVA protein, the IFN-γ+ T cells in splenocytes were enumerated after intracellular staining. As expected, adoptive transfer of TRAP-induced Bregs increased the frequencies of IL-10+ CD19+ cells in the splenocytes (). It was found that OVA-loaded DC vaccinations induced high frequencies of IFN-γ+ CD8+ and CD4+ T cells, whereas adoptive transfer of TRAP-induced Bregs, but not control B cells, resulted in a significant decrease in the frequencies of both IFN-γ+ CD8+ and CD4+ T cells (). Consistently, the decreased frequencies of IFN-γ+ T cells led to reduce IFN-γ production of the splenocytes from TRAP-induced B cells transferred mice (). Furthermore, we found that adoptive transfer of TRAP-induced Bregs, but not control B cells, obviously reduced the OVA-pulsed DC vaccination-induced antitumor effects and abrogated the improved survival (), suggesting that TRAP-induced B cells could remarkably suppress T cell responses and their antitumor efficacy.
Figure 3. TRAP-induced IL-10-producing B cells inhibit T cell immune response and their antitumor efficacy in a non-antigen specific manner. Tumor-bearing mice (n = 8) were s.c. vaccinated with DCOVA (1 × 106), and then adoptively transferred (i.v.) with E.G7-OVA or B16-F10 cells-derived TRAP-activated B cells (1 × 107). (A-F) At day 7 after last vaccination, the frequencies of the IL-10+ CD19+ B cells in the spleens from mice of each group (n = 3) (A and B) and the IFN-γ+ CD8+ T cells (C and D) and IFN-γ+ CD4+ T cells (E and F) cells in the spleen were determined by flow cytometry. (G) The secretion of IFN-γ by splenocytes when re-stimulated in vitro with OVA was measured by ELISA. (H and I) remaining five mice were continuously monitored and measured tumor volume (H) and percentage of survival (I). Data (mean ± s.e.m) are representative of three independent experiments. NS: p > 0.05, * p < 0.05, ** p < 0.01, *** p < 0.001 by unpaired t-test (B, D, F and G), two-way analysis of variance (H), and log-rank test (I).
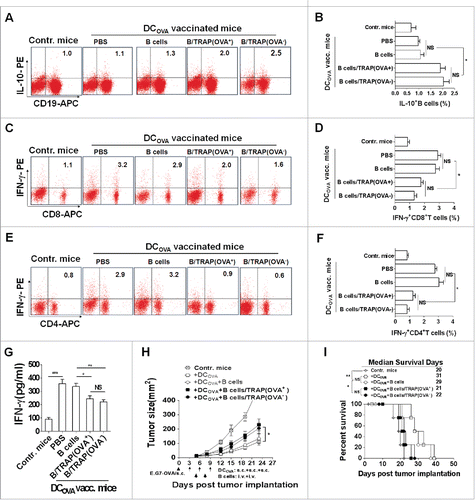
To further investigate whether or not the inhibitory effects of TRAP-induced Bregs on DCOVA-induced T cell response and their antitumor effect were antigen-specific, B16-F10 cells-derived TRAPs were used as non-OVA-specific TRAPs (OVA−-TRAPs) and the above experiment in the E.G7-OVA-bearing mouse model was repeated. Similar with OVA+ TRAP-induced Bregs, adoptive transfer of OVA− TRAP-induced Bregs reduced the frequencies of IFN-γ+ CD8+ and CD4+ T cells in splenocytes of DCOVA-vaccinated mice, consistent with a decreased level of IFN-γ in the culture supernatant (). Furthermore, adoptive administration of OVA−-TRAP-induced Bregs displayed a similar effect with OVA+-TRAP-induced Bregs in abrogating antitumor efficacy of OVA-loaded DC vaccination-induced T cell response (), indicating that the inhibitory effects of TRAP-induced Bregs was non-antigen specific.
The activation of TLR2-MyD88-NF-κB signal pathway is critical for TRAP-induced B cell differentiation
Host-endogenous molecules associated with damaged cells and tissues have been shown to activate TLRs,Citation24 raising the possibility that these receptors play a role in response to danger signals released from tumor cells.Citation25 It has been shown that TLRs signals are crucial for the induction of IL-10 production by antigen-presenting cells (APCs). Thus, we examined whether the TLRs signals were involved in sensing TRAPs. Splenic B cells from WT mice and mice deficient for TLR2, TLR4, or their adaptor proteins, MyD88, were examined for IL-10 production after stimulation with TRAPs. We found that TLR2- and MyD88-deficient B cells, but not TLR4-deficient B cells, failed to produce IL-10 in response to TRAPs (). Because TLRs activate the conserved MyD88-dependent pathway that leads to nuclear factor-κB (NF-κB) activation, we hypothesized that IL-10 production by TRAP-induced B cells may be related to TLR2-mediated NF-κB activation. It was found that pre-treatment of WT B cells with Bay11–7082, an IκB-α phosphorylation inhibitor, substantially attenuated TRAP-induced IL-10 production (). Moreover, intracellular staining analysis showed that TRAPs stimulation induced the phosphorylation of NF-κB p65, a known transcription factor leading to the activation of the IL-10 gene,Citation26 in B cells from WT mice and TLR4-deficient mice, but not TLR2- and MyD88-deficient mice (). Furthermore, we found that after pre-stimulation with TRAPs, B cells from TLR2 or MyD88-deficient mice were incapable of inhibiting CD8+ and CD4+ T cell proliferation when compared with WT controls, while B cells from TLR4-deficient mice still retained the intact capacity to suppress T cell proliferation (). Together, these results demonstrated that TRAPs could induce IL-10 production in B cells via activation of TLR2 and its adaptor MyD88, leading to NF-κB phosphorylation.
Figure 4. TRAPs induce IL-10-producing B cell via TLR2-MyD88-NF-κB dependent signaling pathway. (A) B cells purified from WT, TLR2-, TLR4-, or MyD88 deficient mice were stimulated with TRAPs (3 μg/mL) for 72 h. Frequency of IL-10-producing B cells was determined by flow cytometry. (B and C) B cells purified from WT mice were pre-treated with NF-κB inhibitor Bay11–7082 at different concentrations for 60 min, and then stimulated with TRAPs (3 μg/mL) for 72 h. Frequency of IL-10+ B cells was assessed by flow cytometry (B). IL-10 levels in supernatants were measured by ELISA (C). (D) B cells purified from WT, TLR2-, TLR4-, or MyD88 deficient mice were stimulated with TRAPs (3 μg/mL) for 1 h, and the expression of pNF-κB p65 was determined by flow cytometry. (E) CFSE-labeled CD4+ T or CD8+ T cells purified from WT mice were stimulated with plate-bound anti-CD3/anti-CD28 mAb and were either cultured alone (T cells only) or were co-cultured with TRAP-induced B cells (3 μg/mL) from WT, TLR2-, TLR4-, and MyD88 deficient mice at ratio of 1:1 for 4 d. T cell proliferation was evaluated by flow cytometry. Data (mean ± s.e.m) are representative of three independent experiments. ** p < 0.01, *** p < 0.001 by unpaired t-test.
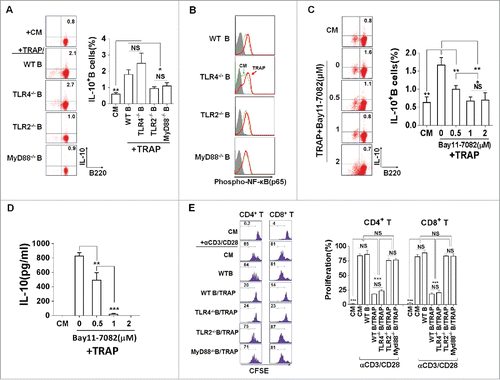
TRAP-derived HMGB1 is involved in promoting IL-10 production of B cells
To identify the factor present on TRAPs that could induce IL-10 production, we first screened endogenous TLR2 ligands in autophagosomes.Citation27,28 HMGB1, functions as a damage-associated molecular pattern (DAMP), is a known endogenous TLR2 ligands.Citation29 Previous studies showed that dying cancer cells could secrete HMGB1 in an autophagy-dependent manner, indicating a role for autophagosomes as ‘carriers’ of HMGB1.Citation30 Based on these findings, we hypothesized that HMGB1 on TRAPs might play an important role in TRAP-induced IL-10 production of B cells. As expected, more than 90% of LC3B+ TRAPs carried abundant levels of HMGB1 (). Subsequently, we found that pre-treatment of TRAPs with a functional anti-HMGB1 antibody almost completely abolished the effect of TRAPs on inducing IL-10-producing B cells compared pre-treatment with isotype antibody (). To further confirm a specific effect of HMGB1 in TRAPs, the tumor cells were stably transfected with HMGB1 shRNA. After transfection, the expression of HMGB1 was found to be significantly decreased both in the cells and purified TRAPs (Figs. S4A and B). ELISA detection showed that TRAPs derived from HMGB1-shRNA-transfected cells had a reduced capacity to induce IL-10 production in B cells when compared with TRAPs from WT cells or cells transfected with negative control shRNA (). Interestingly, the sonicated TRAPs significantly reduced their potential effects on inducing IL-10 production of B cells compared with the intact TRAPs (). These findings suggested that HMGB1 carried on the intact TRAPs was critical for TRAP-induced IL-10 production of B cells.
Figure 5. The expression of HMGB1 on TRAPs is essential for the induction of IL-10-producing B cells. (A) TRAPs derived from E.G7-OVA cells were labeled with anti-LC3 and anti-HMGB1 mAb, or control isotype. FACS analysis was then performed to determine HMGB1 level on the surface of LC3+ TRAPs. (B) TRAPs were pretreated with functional anti-HMGB1 mAb or control isotype overnight at 4°C and then used to stimulate purified B cells for 72 h. Frequency of IL-10+ B cells was assessed by flow cytometry. (C) The production of IL-10 by B cells was determined at 72 h after stimulated with TRAPs isolated from B16-F10 transfected with HMGB1-shRNA or Control-shRNA Plasmid. (D) TRAPs derived from WT B16-F10 cells were subjected to 20 cycles of sonication (30 sec sonication, 30 sec ice) and then used to stimulate purified B cells for 72 h. IL-10 production by B cells were determined by ELISA. Data (mean ± s.e.m) represent a typical result from three independent experiments. ** p < 0.01, *** p < 0.001 by unpaired t-test (C and D).
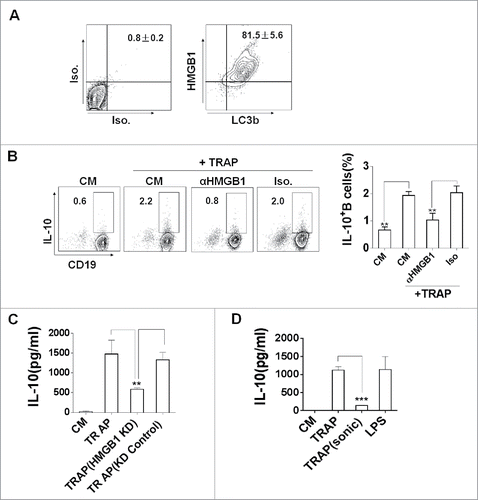
Autophagosomes isolated from malignant effusions or ascites induce human Breg differentiation
To further examine whether autophagosomes from cancer patients could induce human B cells to differentiate into Bregs, we collected the malignant effusions or ascites from 14 cancer patients with their clinical characteristics summarized in Fig. S5. FACS analyses showed that 96% of the membrane vesicles in effusions from cancer patients were positively stained with the typical autophagosomal marker LC3 (Fig. S6A). Western-blotting analysis revealed that LC3-II, the lipidated form of LC3-I that is specifically linked with autophagosomes membranes, was also expressed at high levels in fractions (Fig. S6B). Further TEM examination indicated that high-speed pelleted fractions from malignant pleural effusions contained abundant double-membrane vesicles with the structural features of autophagosomes (Fig. S6C). These results, together with their morphological characteristics and typical autophagosome markers, confirmed that these vesicles isolated from malignant effusions of cancer patients were extracellular autophagosomes (i.e. human TRAPs).
To test whether human TRAPs from cancer patients have similar biologic activities as murine TRAPs, PBMCs from four healthy blood donors were stimulated with human TRAPs prepared from malignant effusions or ascites of 14 cancer patients or from human hepatocarcinoma cell line (HepG2 cells). Notably, both human TRAPs from cancer patients and HepG2 cells efficiently induced the differentiation of IL-10-producing B cells, as well as the secretion of IL-10 (). Interestingly, all of human TRAPs from cancer patients expressed HMGB1, albeit with variable expression levels (). Moreover, a significant positive correlation was observed between the expression levels of HMGB1 and frequency of human TRAPs-induced IL-10-producing B cells (). Further, the potential suppressive activity of these human TRAPs-induced human Bregs was assessed. Consistent with the murine TRAP-induced Bregs, human TRAPs-induced Bregs suppressed the proliferation of autologous CD4+ and CD8+ T cells (). These findings suggested that autophagosomes isolated from the malignant effusions or ascites of cancer patients could induce regulatory IL-10-producing B cells. The induction was positively correlated with the expression level of HMGB1 on autophagosomes.
Figure 6. TRAPs from cancer patients induce human regulatory IL-10-producing B cells. (A and B) PBMC from four healthy donors was stimulated with TRAPs (1 μg/mL) from malignant effusions of 14 cancer patients or HepG2 cells for 72 h. The frequency of IL-10+ B cells was assessed by flow cytometry (A). ELISA determinations of IL-10 secretion in culture supernatants were also shown (B). (C) The expression of HMGB1 on human TRAPs was analyzed by flow cytometry, hTRAP1-3 were representative samples out of 14 cancer patients' TRAPs. hTRAP1-3 derived from patient 6, 7, and 2. (D) The frequencies of IL-10+ B cells induced by hTRAP1-3 were shown. (E) The graphs showed the correlation between intensities of HMGB1 expression in 14 cancer patients' TRAP and frequency of induced IL-10+ B cells. (F) TRAP-induced human B cells inhibit proliferation of T cells stimulated with anti-CD3/CD28 mAbs. Data (mean ± s.e.m) represent a typical result from three independent experiments. *p < 0.05, **p < 0.01, ***p < 0.001 by unpaired t-test (A and B) and Spearman's rank correlation (E).
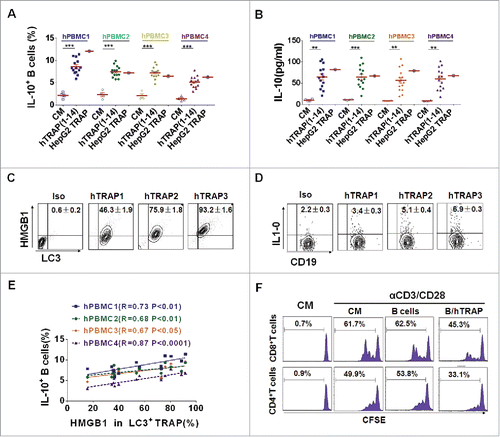
Discussion
As a homeostatic and evolutionarily conserved process, autophagy begins with the formation of autophagosomes that encapsulate damaged organelles or cellular debris, and then ends with fusion with lysosomes to degrade their contents.Citation31 However, our previous studies on tumor autophagy suggested an alternative fate of the autophagosomes after formation, that is, its release into the extracellular fluid upon stress induction with either proteasome inhibition or Vitamin E analog to accumulation of misfolded proteins or increase protein oxidation.Citation32,33 In this study, we found the presence of double-membrane structure vesicles, which expressed the typical autophagosome marker LC3-II, in murine tumor cells culture supernatant without exogenous stress induction, indicating that murine tumor cells could release autophagosomes into extracellular milieu (Fig. S1). More importantly, an abundance of these unconventional secreted autophagosomes was found in the microenvironment of human tumors, as shown in Fig. S6. These vesicles would constitute an entity phenotypically different from exosomes, which are single-membrane vesicles originating from endosome and are not pelleted at the low speed centrifugation (12000 × g), as they are only 50–100 nm in diameter. Numerous studies over the past decade have shed light on the importance of autophagy in cancer, but the functions of these secreted autophagosomes from tumor cells are still unclear.
Since the discovery of exosomes, growing evidence supports the concept that tumors-derived exosomes have a dual role in tumor progression.Citation34,35 They could induce MDSC expansionCitation14 or inhibit T cell function or dendritic cell differentiation.Citation36,37 The immune-activating functions of tumor-derived exosomes have also been reported, but they mainly occur when the tumor cells are exposed to stress conditions.Citation38,39 Tumor-released autophagosomes are a source of shared tumor rejection antigens. Our previous studies have found that autophagosomes, which are enriched from tumor cells after inhibition of proteosome- and lysosome-mediated degradation, can serve as a vessel to ferry a broad spectrum of tumor antigens, including intact proteins, short-lived proteins (SLiP), and defective ribosomal products (DRiP), to APCs for efficient cross-presentation. We refer these autophagosome-enriched artificially, DRiPs-containing blebs as to DRibbles (DRiPs in blebs). Our previous studies have demonstrated that DRibbles are efficient carriers of tumor antigens for effective cross-presentation by dendritic cells, thus facilitate the generation of tumor specific T cell response.Citation6,15,40 These artificial autophagosomes are efficient carriers of tumor antigens for effective cross-presentation by dendritic cells, thus facilitate the generation of tumor specific T cell response.Citation15,41,42
Subsequently, we found that DRibbles can be up-taken by B cells, resulting in B cell activation, antibody secretion and cytokine production, especially the high levels of IL-10 production.Citation22 It is generally believed that IL-10, as an inhibitory cytokine, is a key trait of regulatory B cells (Bregs), we wonder whether autophagosomes released naturally from tumor cells could induce B cells differentiation into IL-10-producing Bregs. In this report, we collected autophagosomes from the supernatant of tumor cells under normal culture conditions without proteasome inhibitor and evaluated their effect on inducing Bregs. Indeed, we found that these natural autophagosomes could induce B cells to differentiate into Bregs, exerting inhibitory activity on T cell proliferation and functions through IL-10 secretion, and ultimately lead to tumor growth (). We termed these autophagosomes released naturally by tumor cells as TRAP to show the difference between DRibbles (DRiPs-containing autophagosome) and TRAP (tumor cells-released autophagosomes, natural autophagosomes).
Figure 7. A proposed mode for the mechanisms and functions of IL-10-producing B cells induced by TRAPs. Membrane-bound HMGB1 on the intact TRAPs activate TLR2 on B cells, leading to TLR-mediated MyD88/NF-κB activation and secretion of IL-10 that may impair antitumor T cell response and ultimately lead to tumor growth.
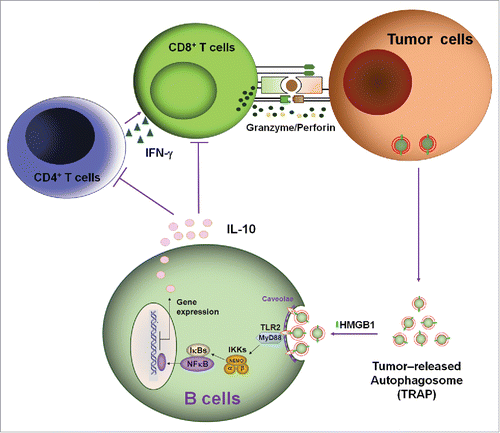
Bregs, a newly described subset of B cells, have been proved to play a suppressive role in immune system. Considering the immune regulating function of the Bregs, recent studies have focused on the role of Bregs in cancer.Citation19 In a study based on a mouse mammary adenocarcinoma 4T1 model, CD25+ B cells induced by cancer conditional media are able to inhibit T-cell proliferation and induce the conversion of CD4+ T cells into Tregs, thus favoring metastasis.Citation21 A recent study even revealed that human GrB-expressing B cells and IL-10-expressing B cells indeed infiltrate solid tumor tissue, where they may contribute to the suppression of antitumor immune responses.Citation43 Here, we found that TRAP induced B cells could inhibit T-cell proliferation in response to nonspecific stimuli (anti-CD3/CD28 antibody; ) and specific stimuli (OVA257–264; ). IL-10 and TGF-β were the most prominent immunosuppressive factors secreted by Bregs.Citation19 While in our study, the immune modulatory function of TRAP-induced B cells was mediated by their production of IL-10, as TGF-β was undetectable. Adoptive transfer of TRAP induced B cells to the DCOVA vaccinated tumor-bearing mice leads to suppression of IFN-γ production by effector CD4+ and CD8+ T cells and thus favors tumor progression (). However, whether effector T cells were the primary targets of suppression by IL-10 producing B cells in vivo needs to be demonstrated. Although a high level of IL-10 was secreted by B cells in our experiments, IL-10 did not promote generation of CD4+ CD25+ Foxp3 Treg cells (data not shown). The exact phenotype of Bregs was not clearly defined and multiple discrepancies existed in different experimental conditions. Markers on mouse Bregs resembled those on CD1dhi CD5+ B10 cells, CD1dhi CD21hi CD23− CD24hi IgDlo marginal-zone B cells, and CD19+ CD21hi CD23hi CD24hi IgDhi IgMhi T2-MZP cells.Citation23,44 Here, our data showed that TRAP-induced IL-10-producing B cells were hybrid Bregs subsets which contained B10 cells, but not splenic marginal-zone B cells (). Also, CD25 and GrB were not expressed on the TRAP-induced B cells.
Mounting evidence suggested that TLR stimulation was required in the immune regulatory function of B cells. B cells activated with Helicobacter felis, which signals via TLR2 and MyD88, constrain the development of severe gastric pathology by reducing excessive Th1 responses.Citation45 Similarly, in mice whose B cells are unable to respond to TLR2/4 stimulation, EAE is exacerbated due to an inability of Bregs to produce IL-10 and suppress T cell activation.Citation46 Consistent with previous reports, TLR2 and its adaptor MyD88 were involved in the induction of IL-10-producing B cells by TRAPs, thus might be responsible for the immunosuppressive functions of induced B cells. Next, we identified the factors present on TRAPs which could induce IL-10-producing B cells. By screening the expression of known endogenous TLR2 ligands in TRAPs, we focused on HMGB1. Initially identified as the prototypical DAMP, HMGB1 is overexpressed in several solid tumors, and linked with tumor progression, invasion, and metastasis.Citation47 HMGB1, as an immune-stimulatory molecule, has been reported to play paradoxical dual effect in tumors. HMGB1 can facilitate dendritic cell maturationCitation48 and enhances DC-mediated antigen presentation during chemotherapy and radiotherapy,Citation25 thus facilitates the activation of tumor-reactive T cells. However, HMGB1 is also known to facilitate tumor progression by co-opting other immune cells and by directly affecting tumor cell growth.Citation29,49,50 Parker and his colleague found that ubiquitous HMGB1 in the tumor microenvironment promoted the development of MDSCs and increased MDSC-mediated production of IL-10 via activating the NF-kB signal transduction pathway in MDSCs.Citation51 By using blocking antibody or lipidosome-delivered shRNA, we demonstrated that membrane-associated HMGB1 expressed on TRAPs was critical in inducing IL-10 producing B cells, which was detrimental for the antitumor response (). HMGB1 presented in TRAPs is essential, but not the only factor, in inducing Bregs. Caveolae-mediated endocytosis of TRAP is also necessary as the capacity of TRAPs in inducing Bregs is significantly reduced when B cells were pretreated with either chlorpromazine, which inhibits clathrin-mediated endocytosis by perturbing clathrin processing, or filipin III, which complexes with membrane cholesterol and blocks caveolae-mediated endocytosis (unpublished data). The membrane integrity of TRAP might be important in caveolae-mediated endocytosis, this might explain why sonicated TRAP lost their stimulatory effect despite the presence of equal amount of HMGB1 ().
Malignant effusions and ascites represent tumors microenvironment and contain various cell types, including malignant cells that shed from the tumor and soluble growth factors.Citation52 In addition, mounting evidence showed that membrane vesicles including exosomes are present in large numbers in malignant effusions from a number of malignancies, and may stimulate the growth and invasive behavior of cancer cells.Citation34 Here, our results suggest that cancerous malignant effusions or ascites contained large numbers of LC3B positive autophagosomes which had abundant expression of HMGB1. Beside tumor cells, most of the other cells in the effusion of our 14 patients were lymphocytes and monocytes.Citation53 Although our current study did not determine whether the tumor cells are the exclusive source of secreted autophgosomes, we demonstrated that IL-10-producing B cells could be induced when PBMC from healthy individuals were pulsed with these autophagosomes. Of note, the frequency of induced IL-10-producing B cells was positively correlated with the amount of HMGB1 found in TRAPs. Future studies will be focused to determine the requirement and relative contribution of TRAP or immune-stimulatory molecules on the TRAP to the differentiation of Bregs in clinical cancer patients.
Collectively, these findings indicate that as long as cancer persists, autophagosomes that express a large array of bioactive molecules could be released from tumor cells into the extracellular space. Through HMGB1, these vesicles could induce the differentiation of IL-10-producing Bregs, exerting inhibitory activity on T cell proliferation and functions through IL-10 secretion, and ultimately lead to tumor growth (). In addition to IL-10-producing B cells, our recent research showed that MDSCs and M2-like macrophage also could be induced by TRAPs in vitro and in vivo (unpublished data), thus, further confirmed the tumor-supportive role of TRAPs.
Materials and methods
Mice, cells, and reagents
C57BL/6 female mice were purchased from the Comparative Medicine Center, Yangzhou University (Jiangsu, China). B6.IL-10-deficient mice (B6.129P2-Il10tm1Cgn/JNju), TLR4-deficient mice (C57BL/10ScNJNju), TLR2-deficient mice (B6.129-Tlr2tmIkir/JNju), and MyD88 Knockout mice were purchased from the National Resource Center for Mutant Mice Model Animal Research Center, Nanjing University (Nanjing, China). OT-1 T-cell receptor transgenic mice (recognize the H-2Kb-restricted OVA257–264 peptide) were gifts from Dr Sheng Xia (Medical School of Jiangsu University, Zhenjiang, China). All animal experiments were approved by the animal care and use committee of Southeast University. Hepa1–6, B16-F10, 4T1, EL4, and E.G7-OVA cells were cultured in complete medium made of RPMI 1640 (Invitrogen). E.G7-OVA cells are mouse thymoma EL4 cells stably transfected with the cDNA of chicken OVA and thus express OVA epitopes as a unique antigen. Unless otherwise indicated, all antibodies (Abs) were obtained from BD Pharmingen.
Patients
The patients were included if they presented with malignant effusions or ascites and had tumor cells in the biological fluid. All procedures were carried out ethically with pre-approval from the Ethics Committee for Human Studies, Southeast University. Clinical characteristics of the patients (n = 14) are summarized in Fig. S5. The malignant effusions or ascites were removed either at the first debulking operation or at examination under anesthetic, or under local anesthesia because of symptoms.
TRAPs purification and characterization
TRAPs were prepared as described previously.Citation41 Briefly, the tumor cell culture supernatant was harvested and spun at 480 × g to remove dead cells and debris, as described by Stromhaug and colleagues.Citation54 The supernatant was then spun at 12000 × g to harvest the autophagosome-containing pellet. Using a Percoll gradient and high-speed centrifugation, we further fractionated the vesicles from the pellet according to their density. Autophagosomes are light vesicles and band at lower density than mitochondria and lysosomes. We used a similar process to purify autophagosomes from malignant effusion or ascites samples accumulated over 3–5 passages. For the autophagosomes purification in tumor tissue, 4T1 tumor tissues were minced with scissors into 1-mm cubes, which were digested with 0.5% Collagenase IV (Sigma-Aldrich) and DNase IV (200 Units/mL, Sigma-Aldrich) at 37°C for 1 h on a platform rocker. After digestion, the TRAPs were isolated from the centrifugal supernatant of digested tumor tissue as described above. Protein concentrations of the autophagosomes were determined using a BCA protein assay Kit according to the manufacturer's protocol (Beyotime, P0010). The isolated pellet fraction was then stained with anti-LC3 (Novus Biological, NB600-1384AF488) and anti-HMGB1 (Novus Biological, NB100-2322AF647) mAb, and analyzed using flow cytometry. A sensitive photo multiplier tube was added on the Forward Side Scatter parameter of a FACS Aria II cell sorter, which allows detection of small-size particles to 200 nm or greater. Western blot was conducted as previously described.Citation41 The primary antibodies included rabbit anti-LC3 (1:1000; Novus Biological, NB100-2322) and rabbit anti-HMGB1 (1:1000; Novus Biological, NB100-2322). The secondary antibodies were goat anti-rabbit horseradish peroxidase (1:10000, Jackson Immuno Research, 111-035-003). The endotoxin levels of these autophagosomes were below the limit of detection of the LAL QCL-1000 kit (Lonza, E50-640).
Electron microscopy
For electron microscopy, samples were prepared as described.Citation15 Briefly, the samples were fixed in 100 mmol/L sodium cacodylate, 2.5% glutaraldehyde, 1.6% paraformaldehyde, 0.064% picric acid, 0.1% ruthenium red, post-fixed with osmium tetroxide plus potassium ferricyanide and embedded in Epon according to routine techniques. The tissue sections were stained with uranyl acetate and lead citrate. Electron microscopic photos were taken at 60 kV on a JEM-1011 transmission electron microscope (TEM).
Atg5 or HMGB1 knockdown by shRNA
For knockdown of Atg5, shRNA expression plasmid pLKO.1-puromycin shRNA scramble (5′-TCCTAAGGTTAAGTCGCCCTCG-3′) and shRNA ATG5 (5′-CCTGAACAGA ATCATCCTTAA-3′) were separately constructed (Hanbio, Shanghai, China). For lentivirus transfection, the 4T1 cells at a density of 30% confluence were transfected by pLKO.1-shRNA-Atg5 or control-shRNA-lentivirus particles using MOIs of 20 each other. Forty-eight hours later, shRNA expressing cells were selected in media containing 5 μg/mL puromycin for 3 d followed by a recovery period in regular media. For knockdown of HMGB1, tumor cells were transfected with shRNA Plasmid (pGPU6; GenePharma) using Lipofectamine® 3000 (Invitrogen, L3000015) and selected with Geneticin (Invitrogen, 11811023). Knockdown of ATG5 or HMGB1 in tumor cells was verified by Western-blot or quantitative real-time PCR (Q-PCR). The expression of HMGB1 in TRAPs derived from selected B16-F10 shRNA HMGB1 (HMGB1 knockdown [KD]) was detected using flow cytometry.
Cell culture and flow cytometric analysis
Murine splenic B cells (Invitrogen, 11422D), CD4+ T cells (Invitrogen, 11415D), and CD8+ T cells (Invitrogen, 11417D) were isolated by magnetic bead purification. Flow cytometric analysis of isolated B and T cell subpopulations consistently showed purity of > 95%. For TRAP-induced B cells generation, purified B cells were incubated with TRAPs (3 μg/mL) for 72 h in complete RPMI. Control B cells were treated with PBS or 10 μg/mL LPS. In some experiments, B cells were pretreated with the inhibitors Bay11-7082 (Calbiochem, 196870) for 60 min, washed with PBS for three times, and subsequently stimulated with TRAPs for 72 h. PBMCs prepared from healthy donor blood were isolated by Ficoll-Paque Plus (GE Healthcare, 17-1440-03). CD3+ T cells (Invitrogen, 11365D) and autologous CD19+ B cells (Invitrogen, 11351D) were isolated from PBMCs by magnetic bead purification. IL-10 secretion was quantified by ELISA following manufacturer's protocol (eBioscience, 88-7104-86). For intracellular staining, PMA (50 ng/mL; Sigma-Aldrich, P8139), ionomycin (500 ng/mL; Sigma-Aldrich, I3909), and monensin (2 mM; Abcam, ab120499) were added to the culture 5 h before flow cytometric analysis.Citation55 For detection of phosphorylation, Lyse/Fix Buffer (BD Phosflow, 558049) and Perm Buffer III (BD Phosflow, 558050) were used. Dead cells were detected by using LIVE/DEAD Fixable Green (Invitrogen, L23101) before cell-surface staining.
T cell suppression assay in vitro
Purified CD4+ or CD8+ T cells from wild-type C57BL/6 mice were labeled with CFSE (5 μM; Invitrogen, C1157), and then cultured in a 24-well plate pre-coated anti-CD3 (2 µg/mL; BD Pharmingen, 550275) and anti-CD28 mAb (2 µg/mL; BD Pharmingen, 553294). TRAP-induced B cells from wild-type C57BL/6 mice, MyD88−/− mice, IL-10−/− mice, TLR2-deficient mice, or TLR4-deficient mice and control B cells were co-cultured with those T cells at a ratio of 1:1. Four days later, proliferation of CD4+ or CD8+ T cells was evaluated with CFSE dilution by flow cytometry. In transwell culture experiment, TRAP-induced B cells were added in the upper chamber whereas T cells in the lower chamber. To assess whether the inhibitory effect of induced B cells was antigen specific, CFSE labeled OT-1 splenocytes were stimulated with OVA257–264 (1 µg/mL), followed by culturing with TRAP-induced B cells. To test the suppressive effects of human TRAP-induced B cells, purified B cells were pre-stimulated with cancer patients-derived autophagosomes for 3 d, and then cultured with CFSE-labeled autologous CD3+ T cells at a ratio of 1:1 in a 96-well plate pre-coated anti-CD3 (2 µg/mL; BD Pharmingen, 550367) for 4 d.
In vitro generation of bone marrow derived DC
BMDC were generated according to an established protocol.Citation56 Briefly, BM cells were prepared from female C57BL/6 mice, then cultured with GM-CSF (10 ng/mL; PeproTech, 315–03-20) and IL-4 (1 ng/mL; PeproTech, 214–14-20). Cells were fed on day 3 and day 5 by replacing half of the medium with fresh IL-4 and GM-CSF. At day 7, cells were harvested, and the purity of BMDC was identified by FACS. For immunizations, DCs were stimulated with LPS (1 µg/mL) for 24 h before using.
Tumor challenge and detection of immune responses
C57BL/6 mice were challenged through s.c. injection of 1 × 106 E.G7-OVA tumor cells on day 0. Mice were s.c. vaccinated with 1 × 106 OVA-loaded DCs at days 3, 6, and 9 after tumor challenge. To evaluate the role of IL-10-producing B cells in DCOVA mediated antitumor responses, mice were i.v. injected with 1 × 107 induced B cells, generated from C57BL/6 B cells treated for 3 d with E.G7-OVA- or B16-F10-derived TRAPs, at days 5, and 7 after tumor challenge. One week after last vaccination, three mice from each group were killed and the frequency of IFN-γ+ CD4+ T and IFN-γ+ CD8+ T cells in spleens was determined by intracellular cytokine staining after in vitro stimulation with the OVA protein for 24 h. The growth of E.G7-OVA tumors in the remaining five mice were continuously monitored and measured. For 4T1 tumor model establishment, C57BL/6 mice were s.c. injected with 2 × 106 Atg5 KD 4T1 tumor cells or control 4T1 tumor cells. Tumor-bearing mice were sacrificed when solid tumor volumes reached approximately 150 mm2, the frequencies of IL-10+ B cells in tumor-draining lymph node and tumor tissue were analyzed by FACS as described above.
Statistical analysis
Data were analyzed for significance in GraphPad Prism by unpaired Student's t tests, one-way ANOVA, or two-way ANOVA. Survival was assessed according to Kaplan and Meier including log-rank test. Correlation coefficients and their significance were calculated by two-tailed Spearman's rank correlation. Differences in values were considered significant if p values were < 0.05.
Disclosure of potential conflicts of interest
No potential conflicts of interest were disclosed.
Author contributions
M.Z. and L.X.W. designed the study. M.Z., F.C., Z.W., W.L., H.R., Y.S., and L.X.W. performed the experiments and collected the data. M.Z. and L.X.W. prepared the figures. All authors analyzed and discussed the data. M.Z. and L.X.W. wrote the manuscript. L.L. and H.M.H. contributed in writing the manuscript.
KONI_A_1180485_supplementary_materials.zip
Download Zip (2.5 MB)Acknowledgments
The authors thank Dr Bernard A. Fox (Earle A. Chiles Research Institute, Portland, OR) for his advice; Dr Suyu Shu (Cleveland Clinic, USA, Ret.) and Dr Pengju George Luo (Sherman College of Chiropractic, USA) for their critical review of this manuscript. The authors also thank Dr Yunlang Cai (Zhongda Hospital, Medical School of Southeast University) for providing human samples and Dr Guangming Gan (Electron microscope laboratory, Medical School of Southeast University) for electron microscopy sample preparation and imaging.
Funding
This work received grant support from the National Natural Science Foundation of China (Grant # 31370895, 31170857, 30972783 to L.X.W.).
References
- Maes H, Rubio N, Garg AD, Agostinis P. Autophagy: shaping the tumor microenvironment and therapeutic response. Trends Mol Med 2013; 19:428-46; PMID:23714574; http://dx.doi.org/10.1016/j.molmed.2013.04.005
- White E. Deconvoluting the context-dependent role for autophagy in cancer. Nat Rev Cancer 2012; 12:401-10; PMID:22534666; http://dx.doi.org/10.1038/nrc3262
- Komatsu M, Waguri S, Chiba T, Murata S, Iwata J, Tanida I, Ueno T, Koike M, Uchiyama Y, Kominami E et al. Loss of autophagy in the central nervous system causes neurodegeneration in mice. Nature 2006; 441:880-4; PMID:16625205; http://dx.doi.org/10.1038/nature04723
- Klionsky DJ, Abdalla FC, Abeliovich H, Abraham RT, Acevedo-Arozena A, Adeli K, Agholme L, Agnello M, Agostinis P, Aguirre-Ghiso JA et al. Guidelines for the use and interpretation of assays for monitoring autophagy. Autophagy 2012; 8:445-544; PMID:22966490; http://dx.doi.org/10.4161/auto.19496
- Duran JM, Anjard C, Stefan C, Loomis WF, Malhotra V. Unconventional secretion of Acb1 is mediated by autophagosomes. J Cell Biol 2010; 188:527-36; PMID:20156967; http://dx.doi.org/10.1083/jcb.200911154
- Yi Y, Zhou Z, Shu S, Fang Y, Twitty C, Hilton TL, Aung S, Urba WJ, Fox BA, Hu HM et al. Autophagy-assisted antigen cross-presentation: Autophagosome as the argo of shared tumor-specific antigens and DAMPs. Onco Immunol 2012; 1:976-8; PMID:23162777; http://dx.doi.org/10.4161/onci.20059
- Lazova R, Camp RL, Klump V, Siddiqui SF, Amaravadi RK, Pawelek JM. Punctate LC3B expression is a common feature of solid tumors and associated with proliferation, metastasis, and poor outcome. Clin Cancer Res 2012; 18:370-9; PMID:22080440; http://dx.doi.org/10.1158/1078-0432.CCR-11-1282
- Rabinovich GA, Gabrilovich D, Sotomayor EM. Immunosuppressive strategies that are mediated by tumor cells. Annual Rev Immunol 2007; 25:267-96; PMID:17134371; http://dx.doi.org/10.1146/annurev.immunol.25.022106.141609
- Curiel TJ, Coukos G, Zou L, Alvarez X, Cheng P, Mottram P, Evdemon-Hogan M, Conejo-Garcia JR, Zhang L, Burow M et al. Specific recruitment of regulatory T cells in ovarian carcinoma fosters immune privilege and predicts reduced survival. Nat Med 2004; 10:942-9; PMID:15322536; http://dx.doi.org/10.1038/nm1093
- Valenti R, Huber V, Filipazzi P, Pilla L, Sovena G, Villa A, Corbelli A, Fais S, Parmiani G, Rivoltini L. Human tumor-released microvesicles promote the differentiation of myeloid cells with transforming growth factor-β-mediated suppressive activity on T lymphocytes. Cancer Res 2006; 66:9290-8; PMID:16982774; http://dx.doi.org/10.1158/0008-5472.CAN-06-1819
- Kim JW, Wieckowski E, Taylor DD, Reichert TE, Watkins S, Whiteside TL. Fas ligand-positive membranous vesicles isolated from sera of patients with oral cancer induce apoptosis of activated T lymphocytes. Clin Cancer Res 2005; 11:1010-20; PMID:15709166
- Taylor DD, Gercel-Taylor C. Exosomes/microvesicles: mediators of cancer-associated immunosuppressive microenvironments. Seminars Immunopathol 2011; 33:441-54; PMID:21688197; http://dx.doi.org/10.1007/s00281-010-0234-8
- Xie Y, Bai O, Yuan J, Chibbar R, Slattery K, Wei Y, Deng Y, Xiang J. Tumor apoptotic bodies inhibit CTL responses and antitumor immunity via membrane-bound transforming growth factor-beta1 inducing CD8+ T-cell anergy and CD4+ Tr1 cell responses. Cancer Res 2009; 69:7756-66; PMID:19789353; http://dx.doi.org/10.1158/0008-5472.CAN-09-0496
- Chalmin F, Ladoire S, Mignot G, Vincent J, Bruchard M, Remy-Martin JP, Boireau W, Rouleau A, Simon B, Lanneau D et al. Membrane-associated Hsp72 from tumor-derived exosomes mediates STAT3-dependent immunosuppressive function of mouse and human myeloid-derived suppressor cells. J Clin Invest 2010; 120:457-71; PMID:20093776; http://dx.doi.org/10.1172/JCI40483
- Li Y, Wang LX, Pang P, Cui Z, Aung S, Haley D, Fox BA, Urba WJ, Hu HM. Tumor-derived autophagosome vaccine: mechanism of cross-presentation and therapeutic efficacy. Clin Cancer Res 2011; 17:7047-57; PMID:22068657; http://dx.doi.org/10.1158/1078-0432.CCR-11-0951
- Mizoguchi A, Mizoguchi E, Takedatsu H, Blumberg RS, Bhan AK. Chronic intestinal inflammatory condition generates IL-10-producing regulatory B cell subset characterized by CD1d upregulation. Immunity 2002; 16:219-30; PMID:11869683; http://dx.doi.org/10.1016/S1074-7613(02)00274-1
- Fillatreau S, Sweenie CH, McGeachy MJ, Gray D, Anderton SM. B cells regulate autoimmunity by provision of IL-10. Nat Immunol 2002; 3:944-50; PMID:12244307; http://dx.doi.org/10.1038/ni833
- Blair PA, Norena LY, Flores-Borja F, Rawlings DJ, Isenberg DA, Ehrenstein MR, Mauri C. CD19(+)CD24(hi)CD38(hi) B cells exhibit regulatory capacity in healthy individuals but are functionally impaired in systemic Lupus Erythematosus patients. Immunity 2010; 32:129-40; PMID:20079667; http://dx.doi.org/10.1016/j.immuni.2009.11.009
- Balkwill F, Montfort A, Capasso M. B regulatory cells in cancer. Trends Immunol 2013; 34:169-73; PMID:23206438; http://dx.doi.org/10.1016/j.it.2012.10.007
- DiLillo DJ, Weinberg JB, Yoshizaki A, Horikawa M, Bryant JM, Iwata Y, Matsushita T, Matta KM, Chen Y, Venturi GM et al. Chronic lymphocytic leukemia and regulatory B cells share IL-10 competence and immunosuppressive function. Leukemia 2013; 27:170-82; PMID:22713648; http://dx.doi.org/10.1038/leu.2012.165
- Olkhanud PB, Damdinsuren B, Bodogai M, Gress RE, Sen R, Wejksza K, Malchinkhuu E, Wersto RP, Biragyn A. Tumor-evoked regulatory B cells promote breast cancer metastasis by converting resting CD4(+) T cells to T-regulatory cells. Cancer Res 2011; 71:3505-15; PMID:21444674; http://dx.doi.org/10.1158/0008-5472.CAN-10-4316
- Li W, Zhou M, Ren H, Hu HM, Lu L, Cao M, Wang LX. Tumor-derived autophagosomes (DRibbles) induce B cell activation in a TLR2-MyD88 dependent manner. PloS One 2013; 8:e53564; PMID:23326458; http://dx.doi.org/10.1371/journal.pone.0053564
- DiLillo DJ, Matsushita T, Tedder TF. B10 cells and regulatory B cells balance immune responses during inflammation, autoimmunity, and cancer. Annals N York Acad Sci 2010; 1183:38-57; PMID:20146707; http://dx.doi.org/10.1111/j.1749-6632.2009.05137.x
- Marsh BJ, Williams-Karnesky RL, Stenzel-Poore MP. Toll-like receptor signaling in endogenous neuroprotection and stroke. Neurosci 2009; 158:1007-20; PMID:18809468; http://dx.doi.org/10.1016/j.neuroscience.2008.07.067
- Apetoh L, Ghiringhelli F, Tesniere A, Obeid M, Ortiz C, Criollo A, Mignot G, Maiuri MC, Ullrich E, Saulnier P et al. Toll-like receptor 4-dependent contribution of the immune system to anticancer chemotherapy and radiotherapy. Nat Med 2007; 13:1050-9; PMID:17704786; http://dx.doi.org/10.1038/nm1622
- Saraiva M, O'Garra A. The regulation of IL-10 production by immune cells. Nat Rev Immunol 2010; 10:170-81; PMID:20154735; http://dx.doi.org/10.1038/nri2711
- Dengjel J, Hoyer-Hansen M, Nielsen MO, Eisenberg T, Harder LM, Schandorff S, Farkas T, Kirkegaard T, Becker AC, Schroeder S et al. Identification of autophagosome-associated proteins and regulators by quantitative proteomic analysis and genetic screens. Mol Cell Proteomics 2012; 11:M111.014035; PMID:22311637; http://dx.doi.org/10.1074/mcp.M111.014035
- Pallet N, Sirois I, Bell C, Hanafi LA, Hamelin K, Dieude M, Rondeau C, Thibault P, Desjardins M, Hebert MJ. A comprehensive characterization of membrane vesicles released by autophagic human endothelial cells. Proteomics 2013; 13:1108-20; PMID:23436686; http://dx.doi.org/10.1002/pmic.201200531
- Sims GP, Rowe DC, Rietdijk ST, Herbst R, Coyle AJ. HMGB1 and RAGE in inflammation and cancer. Ann Rev Immunol 2010; 28:367-88; PMID:20192808; http://dx.doi.org/10.1146/annurev.immunol.021908.132603
- Thorburn J, Horita H, Redzic J, Hansen K, Frankel AE, Thorburn A. Autophagy regulates selective HMGB1 release in tumor cells that are destined to die. Cell Death Differentiation 2009; 16:175-83; PMID:18846108; http://dx.doi.org/10.1038/cdd.2008.143
- Ma Y, Galluzzi L, Zitvogel L, Kroemer G. Autophagy and cellular Immune Responses. Immunity 2013; 39:211-27; PMID:23973220; http://dx.doi.org/10.1016/j.immuni.2013.07.017
- Li Y, Hahn T, Garrison K, Cui ZH, Thorburn A, Thorburn J, Hu HM, Akporiaye ET. The vitamin E analogue α-TEA stimulates tumor autophagy and enhances antigen cross-presentation. Cancer Res 2012; 72:3535-45; PMID:22745370; http://dx.doi.org/10.1158/0008-5472.CAN-11-3103
- Li Y, Wang LX, Pang P, Twitty C, Fox BA, Aung S, Urba WJ, Hu HM. Cross-presentation of tumor associated antigens through tumor-derived autophagosomes. Autophagy 2009; 5:576-7; PMID:19333005; http://dx.doi.org/10.4161/auto.5.4.8366
- Andre F, Schartz NEC, Movassagh M, Flament C, Pautier P, Morice P, Pomel C, Lhomme C, Escudier B, Le Chevalier T et al. Malignant effusions and immunogenic tumour-derived exosomes. Lancet 2002; 360:295-305; PMID:12147373; http://dx.doi.org/10.1016/S0140-6736(02)09552-1
- Skog J, Wurdinger T, van Rijn S, Meijer DH, Gainche L, Sena-Esteves M, Curry WT, Jr, Carter BS, Krichevsky AM, Breakefield XO. Glioblastoma microvesicles transport RNA and proteins that promote tumour growth and provide diagnostic biomarkers. Nat Cell Biol 2008; 10:1470-6; PMID:19011622; http://dx.doi.org/10.1038/ncb1800
- Taylor DD, Gercel-Taylor C, Lyons KS, Stanson J, Whiteside TL. T-cell apoptosis and suppression of T-cell receptor/CD3-zeta by Fas ligand-containing membrane vesicles shed from ovarian tumors. Clin Cancer Res 2003; 9:5113-9; PMID:14613988
- Temme S, Eis-Hubinger AM, McLellan AD, Koch N. The herpes simplex virus-1 encoded glycoprotein B diverts HLA-DR into the exosome pathway. J Immunol (Baltimore, Md : 1950) 2010; 184:236-43; PMID:19949097; http://dx.doi.org/10.4049/jimmunol.0902192
- Dai S, Wan T, Wang B, Zhou X, Xiu F, Chen T, Wu Y, Cao X. More efficient induction of HLA-A*0201-restricted and carcinoembryonic antigen (CEA)-specific CTL response by immunization with exosomes prepared from heat-stressed CEA-positive tumor cells. Clin Cancer Res 2005; 11:7554-63; PMID:16243831; http://dx.doi.org/10.1158/1078-0432.CCR-05-0810
- Gastpar R, Gehrmann M, Bausero MA, Asea A, Gross C, Schroeder JA, Multhoff G. Heat shock protein 70 surface-positive tumor exosomes stimulate migratory and cytolytic activity of natural killer cells. Cancer Res 2005; 65:5238-47; PMID:15958569; http://dx.doi.org/10.1158/0008-5472.CAN-04-3804
- Twitty CG, Jensen SM, Hu HM, Fox BA. Tumor-derived autophagosome vaccine: induction of cross-protective immune responses against short-lived proteins through a p62-dependent mechanism. Clin Cancer Res 2011; 17:6467-81; PMID:21810919; http://dx.doi.org/10.1158/1078-0432.CCR-11-0812
- Li Y, Wang LX, Yang G, Hao F, Urba WJ, Hu HM. Efficient cross-presentation depends on autophagy in tumor cells. Cancer Res 2008; 68:6889-95; PMID:18757401; http://dx.doi.org/10.1158/0008-5472.CAN-08-0161
- Li H, Li Y, Jiao J, Hu HM. Alpha-alumina nanoparticles induce efficient autophagy-dependent cross-presentation and potent antitumour response. Nat Nanotechnol 2011; 6:645-50; PMID:21926980; http://dx.doi.org/10.1038/nnano.2011.153
- Lindner S, Dahlke K, Sontheimer K, Hagn M, Kaltenmeier C, Barth TF, Beyer T, Reister F, Fabricius D, Lotfi R et al. Interleukin 21-induced granzyme B-expressing B cells infiltrate tumors and regulate T cells. Cancer Res 2013; 73:2468-79; PMID:23384943; http://dx.doi.org/10.1158/0008-5472.CAN-12-3450
- Kalampokis I, Yoshizaki A, Tedder TF. IL-10-producing regulatory B cells (B10 cells) in autoimmune disease. Arthritis Res Therapy 2013; 15 Suppl 1:S1; PMID:23566714; http://dx.doi.org/10.1186/ar3907
- Sayi A, Kohler E, Toller IM, Flavell RA, Muller W, Roers A, Müller A. TLR-2-activated B cells suppress Helicobacter-induced preneoplastic gastric immunopathology by inducing T regulatory-1 cells. J Immunol (Baltimore, Md : 1950) 2011; 186:878-90; PMID:21149607; http://dx.doi.org/10.4049/jimmunol.1002269
- Lampropoulou V, Hoehlig K, Roch T, Neves P, Calderon Gomez E, Sweenie CH, Hao Y, Freitas AA, Steinhoff U, Anderton SM et al. TLR-activated B cells suppress T cell-mediated autoimmunity. J Immunol (Baltimore, Md : 1950) 2008; 180:4763-73; PMID:18354200; http://dx.doi.org/10.4049/jimmunol.180.7.4763
- Kang R, Zhang Q, Zeh HJ, 3rd, Lotze MT, Tang D. HMGB1 in cancer: good, bad, or both? Clin Cancer Res 2013; 19:4046-57; PMID:23723299; http://dx.doi.org/10.1158/1078-0432.CCR-13-0495
- Messmer D, Yang H, Telusma G, Knoll F, Li J, Messmer B, Tracey KJ, Chiorazzi N. High mobility group box protein 1: an endogenous signal for dendritic cell maturation and Th1 polarization. J Immunol (Baltimore, Md : 1950) 2004; 173:307-13; PMID:15210788; http://dx.doi.org/10.4049/jimmunol.173.1.307
- Lotze MT, Tracey KJ. High-mobility group box 1 protein (HMGB1): nuclear weapon in the immune arsenal. Nat Rev Immunol 2005; 5:331-42; PMID:15803152; http://dx.doi.org/10.1038/nri1594
- Bianchi ME, Manfredi AA. High-mobility group box 1 (HMGB1) protein at the crossroads between innate and adaptive immunity. Immunol Rev 2007; 220:35-46; PMID:17979838; http://dx.doi.org/10.1111/j.1600-065X.2007.00574.x
- Parker KH, Sinha P, Horn LA, Clements VK, Yang H, Li J, Tracey KJ, Ostrand-Rosenberg S. HMGB1 enhances immune suppression by facilitating the differentiation and suppressive activity of myeloid-derived suppressor cells. Cancer Res 2014; 74:5723-33; PMID:25164013; http://dx.doi.org/10.1158/0008-5472.CAN-13-2347
- Gortzak-Uzan L, Ignatchenko A, Evangelou AI, Agochiya M, Brown KA, St Onge P, Kireeva I, Schmitt-Ulms G, Brown TJ, Murphy J et al. A proteome resource of ovarian cancer ascites: integrated proteomic and bioinformatic analyses to identify putative biomarkers. J Proteome Res 2008; 7:339-51; PMID:18076136; http://dx.doi.org/10.1021/pr0703223
- Clayton A, Al-Taei S, Webber J, Mason MD, Tabi Z. Cancer exosomes express CD39 and CD73, which suppress T cells through adenosine production. J Immunol (Baltimore, Md : 1950) 2011; 187:676-83; PMID:21677139; http://dx.doi.org/10.4049/jimmunol.1003884
- Stromhaug PE, Berg TO, Fengsrud M, Seglen PO. Purification and characterization of autophagosomes from rat hepatocytes. Biochem J 1998; 335 (Pt 2):217-24; PMID:9761717; http://dx.doi.org/10.1042/bj3350217
- Zhou M, Li W, Wen Z, Sheng Y, Ren H, Dong H, Cao M, Hu HM, Wang LX. Macrophages enhance tumor-derived autophagosomes (DRibbles)-induced B cells activation by TLR4/MyD88 and CD40/CD40L. Exp Cell Res 2014; 331:320-30; PMID:25447440; http://dx.doi.org/10.1016/j.yexcr.2014.10.015
- Ryan SO, Turner MS, Gariepy J, Finn OJ. Tumor antigen epitopes interpreted by the immune system as self or abnormal-self differentially affect cancer vaccine responses. Cancer Res 2010; 70:5788-96; PMID:20587526; http://dx.doi.org/10.1158/0008-5472.CAN-09-4519