ABSTRACT
Myelodysplastic syndromes (MDS) represent a heterogeneous group of clonal stem cell disorders characterized by ineffective hematopoiesis frequently progressing into acute myeloid leukemia (AML), with emerging evidence implicating aberrant bone marrow (BM) microenvironment and inflammation-related changes. 5-azacytidine (5-AC) represents standard MDS treatment. Besides inhibiting DNA/RNA methylation, 5-AC has been shown to induce DNA damage and apoptosis in vitro. To provide insights into in vivo effects, we assessed the proinflammatory cytokines alterations during MDS progression, cytokine changes after 5-AC, and contribution of inflammatory comorbidities to the cytokine changes in MDS patients. We found that IL8, IP10/CXCL10, MCP1/CCL2 and IL27 were significantly elevated and IL12p70 decreased in BM of MDS low-risk, high-risk and AML patients compared to healthy donors. Repeated sampling of the high-risk MDS patients undergoing 5-AC therapy revealed that the levels of IL8, IL27 and MCP1 in BM plasma were progressively increasing in agreement with in vitro experiments using several cancer cell lines. Moreover, the presence of inflammatory diseases correlated with higher levels of IL8 and MCP1 in low-risk but not in high-risk MDS. Overall, all forms of MDS feature a deregulated proinflammatory cytokine landscape in the BM and such alterations are further augmented by therapy of MDS patients with 5-AC.
Abbreviations
5-AC | = | 5-azacytidine |
AML | = | acute myeloid leukemia |
BM | = | bone marrow |
BM-MNC, bone marrow mononucleated cells; CMML | = | chronic myelomonocytic leukemia |
CR | = | complete remission |
DDR | = | DNA damage response |
FAB | = | French, American, British classification |
IPSS | = | International Prognostic Scoring System |
IWG | = | International Working Group |
HSC | = | hematopoietic stem cell |
MB | = | myeloblasts |
MDS | = | myelodysplastic syndrome |
MDS 5q | = | myelodysplastic syndrome associated with isolated del(5q) |
MDS/MPN-u | = | myelodysplastic syndrome/myeloproliferative neoplasm unclassified |
MRSA, methicilin resistant Staphylococus aureus infection; OS, overall survival; PB | = | peripheral blood |
PD | = | progressive disease |
PR | = | partial remission |
RA | = | refractory anemia |
RAEB | = | refractory anemia with excess blasts |
RAEB-T | = | refractory anemia with excess blasts in transformation |
RARS | = | refractory anemia with ringed sideroblasts |
RCMD | = | refractory anemia with multilineage dysplasia |
RN | = | refractory neutropenia |
SA-β-Gal | = | senescence-associated β-galactosidase |
SCF, stem cell factor; SD with HI | = | stable disease with hematologic improvement |
SD without HI | = | stable disease without hematologic improvement |
Introduction
Myelodysplastic syndromes (MDS) represent a heterogeneous group of clonal stem cell disorders characterized by ineffective hematopoiesis, peripheral cytopenia, morphological dysplasia and the risk of transformation to acute myeloid leukemia (AML). The pathogenesis of MDS is highly complex. Besides cytogenetic, molecular and genetic abnormalities in hematopoietic stem cells (HSC), extrinsic factors such as interaction with immune system and bone marrow (BM) microenvironment alterations are involved not only in the BM failure, but also in clonal expansion of the aberrant HSC and impaired cellular differentiation.Citation1-4 There is growing evidence implicating inflammation-related changes, inhibitory cytokines and increased intramedullary apoptosis as contributors to ineffective hematopoiesis, specifically in the early stages of MDS.Citation5-7 Moreover, several recent studies implicate aberrant BM microenvironment and inflammation-related changes in progression of the MDS.Citation5,8 MDS has also higher rate of incidence and earlier onset among patients with autoimmune diseases.Citation4,9-11
MDS treatment was rather ineffective until recent introduction of two new therapeutic approaches: immunomodulation therapy with lenalidomide and hypomethylating therapy with nucleotide analogs 5-azacytidine (5-AC) and 5-aza-2′-deoxycytidine (decitabine).
Both hypomethylating agents are currently considered as a standard treatment for high-risk MDS (i.e., intermediate 2 and high-risk, according to the International Prognostic Scoring System [IPSS]).Citation12 5-AC significantly prolongs overall survival (OS) of patients with high-risk MDS, leads to improvements in cytopenias, reduces leukemic progression and improves quality of life.Citation13-16 5-AC induces 50%–60% responses in those patients, including 10%–20% complete remissions according to International Working Group (IWG) 2006 criteria.Citation13,14
The mechanism of action of 5-AC is not yet fully understood. Beside the DNA demethylation, 5-AC induces DNA damage and apoptosis, and it probably exerts also immunomodulatory effects.Citation17,18
As 5-AC therapy induces not only transient but also long-term changes of the cell populations in BM niche, it is of growing importance to measure and analyze these changes during the treatment. Despite the urgent need to elucidate the cytokine landscapes, however, a comprehensive analysis focusing on changes of proinflammatory cytokines in BM niche over the course of MDS is still lacking. Inspired by such pressing and clinically relevant unmet need, here we evaluated the levels of main proinflammatory cytokines in BM plasma during progression of MDS and assessed the impact of 5-AC treatment, both in clinical specimens and cultured model cancer cell lines.
Results
Therapeutic doses of 5-azacytidine induce expression of proinflammatory cytokines in vitro
Genotoxic effects of nucleotide analogs promote DNA damage response (DDR)Citation19 that is associated with modified expression of several cytokines.Citation20 Therefore, we first examined whether 5-AC used in the clinic to treat high-risk MDS patients is capable of modulating cytokine expression similarly to some other nucleotide analogs. For this purpose, we exposed HeLa cells and the immortalized BM HS-5 cells to doses of 5-AC within the range of 5-AC concentrations reached in clinical use (see Materials and Methods for details) and measured mRNA levels of 84 cytokines included in the Human Common Cytokines PCR Array at day 7 of the daily administration of 5-AC. In HeLa cells treated with 2 µM 5-AC for 7 d, 27 cytokines were at least 2-fold upregulated and three cytokines downregulated. Among the analyzed cytokines, mRNA levels of IL6, IL8, IL24, IL11, OSM and IL20 were upregulated the most (see Table S1a). Similarly, HS-5 cells exposed to 0.5 µM 5-AC resulted in the elevation of mRNA levels of 37 cytokines and 1 downregulation (Table S1b), where transcripts of OSM, IL20 and IL24 were again the most 5-AC-induced cytokines (transcripts of IL6 and IL11 were also upregulated). In summary, prolonged administration of 5-AC altered the level of several cytokine transcripts in two different cancer cell models, in a partly cell-type-dependent manner.
Apart from profiling the cytokine mRNAs, protein levels of 11 proinflammatory cytokines (IFNγ, IL1α, IL1β, IL6, IL8, IL10, IL12p70, IL27, IP10, TNFα, and MCP1) were also estimated in culture medium after the treatment with 5-AC. shows that the levels of IL1α, IL6, IL8 and MCP1 were significantly elevated in supernatants of HS-5 cells treated with 0.5 µM 5-AC for 7 d. Cytokines altered at the protein level in other cell types including several AML cell lines (BM-MNC, HL-60, OCI-M2, SKM-1, HeLa, MCF7) are summarized in .
Figure 1. In vitro changes in proinflammatory cytokines after treatment with 5-AC. Changes in proinflammatory cytokines secreted by HS-5 cells after treatment with 0.5 µM 5-AC for 7 d (A), in freshly isolated BM-MNCs from five healthy donors treated with 4 µM 5-AC for 3 d (B), in HL-60 cells treated with 1 µM 5-AC for 7 d (C), in OCI-M2 cells treated with 1 µM 5-AC for 7 d (D), in SKM-1 cells treated with 0.5 µM 5-AC for 7 d (E), in HeLa cells treated with 2 µM 5-AC for 7 d (F), and in MCF7 cells treated with 2 µM 5-AC for 7 d (G). The changes in cytokine secretion are given only for the cytokines with measured values above the lower limit of detection. The data represent the mean of at least two independent experiments +/– SD for all cell types, all treated in at least triplicates. The resulting measurements were analyzed by paired t-test. p values < 0.05 were considered as statistically significant. Following proinflammatory cytokine changes reached the statistical significance: for HS-5 cell line (A) IL1α (p = 0.017), IL6 (p = 0.003), IL8 (p = 0.031), MCP1 (p = 0.025), IP10 (p < 0.001); for HL-60 cells (C) MCP1 (p = 0.005); for OCI-M2 cells (D) IL6 (p = 0.002), IL8 (p = 0.042), IP10 (p = 0.023); for SKM-1 cells (E) MCP1 (p = 0.042); for HeLa cells (F) IL6 (p = 0.031) and IL8 (p = 0.031); and for MCF7 cells (G) IL6 (p = 0.001) and IL8 (p < 0.001). In case of freshly isolated BM-MNCs, only the downregulation of IP10 after 5-AC administration reached the statistical significance (p = 0.002).
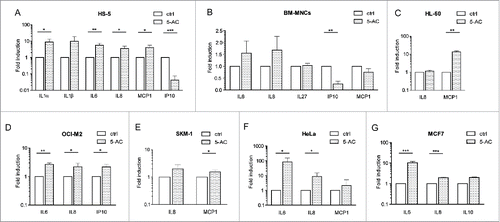
Together, our data show that 5-AC can modify the expression of several cytokines in human cells cultured in vitro and that the spectrum of affected cytokine species is cell-type-dependent. Several proinflammatory cytokines including IL1α, IL6, IL8 and MCP1 were induced in vitro by the addition of 5-AC.
Several proinflammatory cytokines are elevated in bone marrow of MDS patients
Before considering the effect of 5-AC therapy on cytokine milieu in BM of MDS patients, levels of 11 cytokines were estimated in BM plasma of large cohort of 188 MDS (both high- and low-risk) patients and compared with BM plasma levels in healthy donors. Based on robust linear mixed effects model analysis, IL8, IP10, MCP1 and IL27 were significantly elevated in BM of high-risk and low-risk MDS compared to healthy donors (). The levels of IFNγ, IL1α, IL1β, IL6, IL10, and TNFα were not significantly altered in analyzed MDS samples (for details, see Fig. S1) As for IL12p70, its level in MDS was significantly lower (p = 0.02; Fig. S1).
Figure 2. Changes in cytokines in low-risk MDS, high-risk MDS and AML compared to healthy donors. The box plot graphs demonstrate the upper and lower quartiles, and the median is represented by a short black line within the box for each group. The p value signifies the difference for the given cytokine measurements between all three groups. The data set was analyzed by robust linear mixed effects model at the 95% confidence interval. p values < 0.05 were considered as statistically significant. Each significantly upregulated cytokine is depicted in a separate graph, i.e., for (A) IL8 (p = 0.01), for (B) IL27 (p = 0.04), for (C) IP10 (p = 0.01) and for (D) MCP1 (p < 0.01).
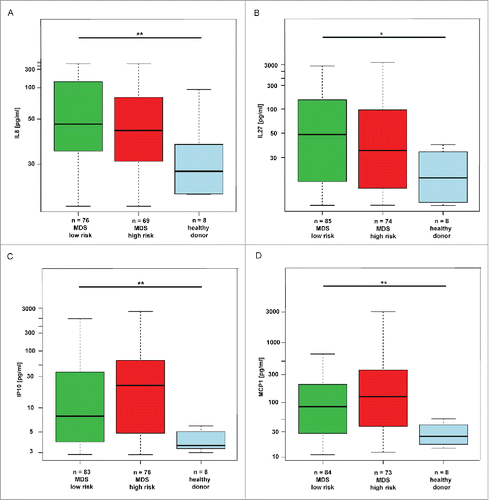
Altogether, our data showed that interleukins IL8 and IL27 and chemokines IP10 and MCP1 were elevated in BM of all groups of MDS patients.
IL8, IL27 and MCP1 are further elevated in bone marrow plasma of MDS patients during 5-azacytidine therapy
To investigate the effect of 5-AC therapy on the level of proinflammatory cytokines in BM of MDS patients, BM plasma samples of MDS patients (mostly high-risk—see for details) were collected before the initiation of therapy, after the end of the 4th and 8th cycle (mostly at day 7 after the last dose of 5-AC) and levels of the 11 cytokines were measured as above. The statistical comparison of six groups (healthy donors, low-risk MDS, AML, 5-AC-treated before therapy, after four cycles of 5-AC and after eight cycles of 5-AC) using Kruskal–Wallis ANOVA revealed that the levels of IL8, IP10 and MCP1 were significantly altered in low-risk MDS, 5-AC-treated before therapy and in AML patients in comparison to healthy controls () underscoring the values obtained in a large non-structured cohort of patients. The increase in IL27 in this cohort did not reach the statistical significance (p = 0.070). Strikingly, when examined by robust linear mixed effects model analysis, the levels of IL8, IL27 and MCP1 in the 5-AC-treated group significantly increased during 5-AC therapy when compared to initial values before therapy (). As for IP10, changes observed during the course of therapy were not significant.
Figure 3. Changes in IL8, MCP1, IP10 and IL27 in BM of MDS patients during therapy with 5-AC. The box plot graphs demonstrate the upper and lower quartiles, and the median is represented by a short black line within the box for each group. The empty circles represent measurements for individual patients whose measured values were numerically distant from the other measurements (outliers; outside 1.5 times the interquartile range above the upper quartile and below the lower quartile). The changes of cytokine values among the individual 5-AC-treated patient (n = 38) are depicted as colored dots of either pink for responders (n = 20) or dark red for non-responders connected by the lines of the same color. Kruskal–Wallis ANOVA compared the changes among the cytokine values of healthy donors, AML patients and low-risk MDS (IPSS low) with the following results: IL8, p = 0.041 (A); IL27, p = 0.07 (B); IP10, p = 0.002 (C) and MCP1, p = 0.018 (D). The robust linear mixed effects model analysis compared changes in cytokine values in repeated samples of 38 5-AC-treated patients and showed the increase during 5-AC therapy when compared to initial values before therapy for IL8, p = 0.01 (A); IL27, p < 0.01(B); MCP1, p < 0.01 (D); but not for IP10, p = 1.00 (C).
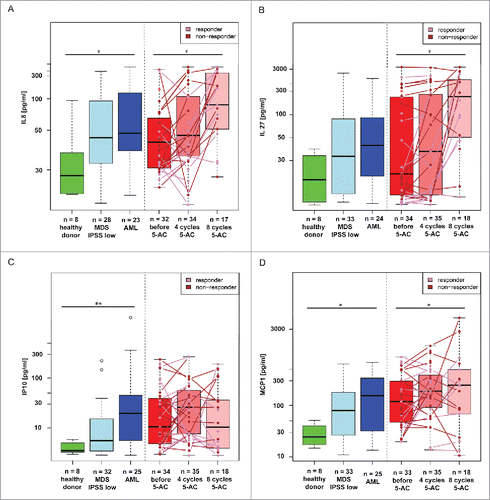
To summarize, our data showed that interleukins IL8 and IL27 and chemokine MCP1 increased in BM of MDS patients during the therapy with 5-AC.
Correlation of cytokine values with the responsiveness to 5-azacytidine therapy
Next, we tried to discriminate whether the increase of IL8, IL27 and MCP1 correlated with the outcome of the 5-AC therapy and hence whether they can be utilized as therapy response factors. For this purpose, the patients receiving 5-AC therapy were analyzed according to criteria of their response (see Materials and Methods for details). When comparing the IL8 levels among responders (n = 20) and non-responders (n = 18; see the colored dots in ), we found that responders have lower median levels of IL8 than non-responders after 4th and 8th cycle of 5-AC therapy; however, this difference did not reach the statistical significance (p = 0.06). A similar scenario was observed for IL27 (), where the levels of IL27 in responders showed again a lower median value compared to non-responders, but this change was not significant (p = 0.22). MCP1 levels did not show any correlation with the response to therapy (p = 0.69).
Among the 11 studied cytokines, the levels of IL8 and IL27 showed a trend of positive correlation with the response to 5-AC therapy in MDS patients; however, a larger cohort of 5-AC-treated MDS patients has to be analyzed in order to reach definitive conclusions.
Inflammatory comorbidities contribute to elevated levels of IL8 and MCP1 in low-risk MDS patients
Next, we asked whether the increased levels of IL8, IL27, IP10 and MCP1 found in our cohort of MDS patients are a characteristic feature of MDS per se or are due to other associated diseases, namely those with inflammatory component.Citation4,9,10 We compared the groups of patients with inflammatory diseases (see Materials and Methods) against those without diagnosed inflammation. Among low-risk MDS patients, significantly higher levels of MCP1 and IL8 were found in patients with ongoing inflammation compared to those without inflammation, indicating that inflammatory comorbidities contribute further to enhanced levels of these two cytokines. On the other hand, among the high-risk MDS patients indication of such correlation, though statistically non-significant, was found only for IL8 (p = 0.07; ). Furthermore, levels of IP10 and IL27 did not show any correlation with inflammatory comorbidities among either low- or high-risk MDS patients ().
Figure 4. Analysis of the effect of inflammatory comorbidities on the cytokine values in low-risk MDS and high-risk MDS and AML. The box plot graphs demonstrate the upper and lower quartiles, and the median is represented by a short black line within the box for each group. The empty circle represents outlier measurement. The data set was analyzed by robust linear mixed effects model at the 95% confidence interval. p values < 0.05 were considered as statistically significant and were calculated for the measured differences among inflamed and non-inflamed MDS patients in their respective risk group, i.e. (A) IL8 for low-risk MDS p = 0.05; for high-risk MDS p = 0.07; (B) IL27 for low-risk MDS p = 0.25; for high-risk MDS p = 0.48; (C) IP10 for low-risk MDS p = 0.37; for high-risk MDS p = 0.30, and (D) MCP1 for low-risk MDS p < 0.01; for high-risk MDS p = 0.61.
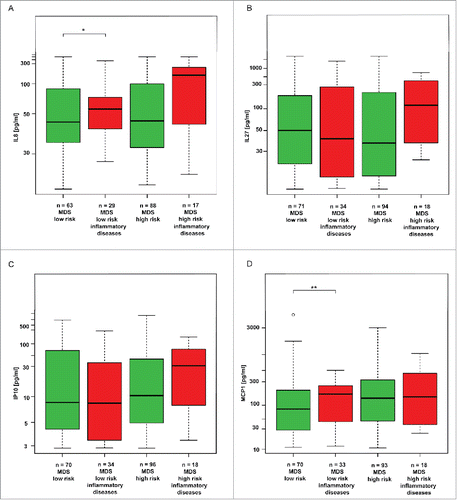
To conclude, inflammatory comorbidities contribute to elevation of IL8 and MCP1 in low-risk MDS patients, while in high-risk patients such contribution was not statistically significant. Among high-risk MDS patients, only IL8 correlated with associated inflammatory diseases, whereas MCP1 levels were not affected by the associated inflammation.
The levels of cytokines in blood plasma do not strictly correlate with the levels in bone marrow plasma
In order to discriminate whether the source of IL8, IL27, IP10 and MCP1 is intramedullary or extramedullary, we have collected peripheral blood (PB) sample from several patients (n = 22) shortly before the BM aspiration and compared the levels of proinflammatory cytokines in both fluids. The cytokine values of IL8 and MCP1 in PB plasma and BM plasma did not correlate well with each other (Fig. S2). For IL27 and IP10, the values appeared to be higher in BM than in PB and thus it is reasonable to assume that the BM was the source tissue. In contrast, for IL8, we observed the opposite tendency, indicating that estimation of cytokines from the PB plasma could be insufficient to obtain an accurate projection about the levels of these four cytokines in the BM milieu.
Discussion
The aberrant cytokine milieu in BM has been considered as one of the hallmarks of MDS. However, due to its more convenient accessibility, the vast majority of studies analyzed cytokine changes in PB.Citation21-23 In this study, we estimated the levels of key proinflammatory cytokines in BM plasma of patients suffering from MDS/AML. We found that IL8, IP10/CXCL10, MCP1/CCL2 and IL27 were significantly elevated and IL12p70 decreased in low-risk, high-risk and AML patients compared to healthy donors. Moreover, by repeated sampling of the high-risk MDS individuals undergoing 5-AC therapy, we found that the levels of IL8, IL27 and MCP1 in BM plasma were progressively and significantly increasing in agreement with our in vitro experiments, where the levels of IL8 and MCP1 and other proinflammatory cytokines were induced in several cells lines in response to short-term exposure to 5-AC.
It is generally accepted that the therapeutic anticancer effects of 5-AC and decitabine are attributable to alterations of epigenetic regulatory mechanisms affecting proliferation of malignant cells.Citation24-26 Moreover, it was shown that decitabine can cause replication stress-associated DDR.Citation27 As a result, DDR-induced block of cell cycle progression by activation of cell cycle checkpoints could act as a parallel mechanism of antiproliferative effects of decitabine and 5-AC. Indeed, in our in vitro experiments with cell lines exposed to therapeutic doses of 5-AC following the clinical regime of 5-AC administration in seven consecutive daily doses, we observed that 5-AC caused DNA damage already during 24 h after the first dose, which was associated with induction of p21waf1/cip1, cell cycle block and the development of senescence-like phenotype (data not shown). This is in concert with the previous study of Fandy et al. who showed that 5-AC induces DNA damage in PB mononuclear cells of MDS patients.Citation28 Therefore, both alteration of epigenetic control of gene expression and development of cellular senescence can affect the expression of cytokines, as was indeed confirmed by change of levels of several cytokine transcripts in the cell lines exposed to 5-AC in vitro. Thus, similar to other nucleotide analogs,Citation20 5-AC affects cytokine expression. Although the precise spectra of 5-AC-altered cytokines were cell type-specific, induction of IL6, IL8 and MCP1 were commonly shared, consistent with previous studies using different senescence inductors.Citation29-31
Given the cell type specificity in cytokine response to 5-AC and taking into account the complex signal communication among cells that compose tissues of the organism, presumably the final outcome of 5-AC-induced cytokine response at the systemic level would reflect such intricate interactions. Therefore, we focused on analyses of major proinflammatory cytokines in the BM environment, a tissue niche most intimately associated with MDS. Our analysis of the cohort of 188 MDS/AML patients showed that four cytokines IL8, IP10, MCP1 and IL27 were elevated and IL12p70 decreased in BM of both low-risk and high-risk MDS patients, as well as in AML patients indicating that the inflammatory mediators contribute to the pathogenesis of MDS.
IL8 is a cytokine, whose elevated protein levels have been already described in PB of MDS/AML patients.Citation32 IL8 plays a role in proinflammatory reactions, specifically in the activation and trafficking of neutrophils (reviewed in ref. Citation33). Its elevated levels are also characteristic for the primary myelofibrosis.Citation34,35 It has been proposed that CD34+ blasts are the major producers of IL8 in BM of MDS patients,Citation32 because the initially elevated levels of IL8 in BM serum of MDS or AML patients subsequently decrease with the complete remission and disappearance of CD34+ blasts. Later, Schinke et al. detected the elevated IL8 transcripts in AML/MDS BM samples.Citation36 Moreover, they found that IL8 supports growth and survival of leukemic cell lines and that the short-hairpin RNA-mediated downregulation of IL8 receptor CXCR2 resulted in increased survival of mice xenografted with lymphoma U-937 cells.Citation36 IL8 is elevated in blood also during other inflammatory diseases such as cystic fibrosis, rheumatoid arthritis, systemic lupus erythematosus, psoriasis, etc.Citation33 IL8 is also the factor being almost invariably induced in normal and cancer cells undergoing various types of cellular senescence including oncogene-,Citation29,30 stress/ionizing irradiation-,Citation31 bacterial toxin-,Citation37 drug-induced,Citation20 and replicative senescence.Citation38 Notably, IL8 itself can induce senescence in vitro.Citation29
The mechanism of IL27 induction in MDS patients is unclear. IL27 is a member of IL12 family of cytokines with a pleiotropic role in proinflammatory and anti-inflammatory immune responses and acquired immunity. IL27 is produced by antigen-presenting cells and is regulating the development of Th cells (reviewed in ref. Citation39). Furthermore, IL27 is involved in differentiation of HSC.Citation40 Notably, besides other proinflammatory cytokines,Citation41 IL27 is capable of triggering both IP10 and MCP1Citation42 suggesting its upstream role in the induction of IP10 and MCP1. IL27 was not induced by 5-AC in several cell types in vitro (this study) or other senescence-inducing stimuli, such as ionizing radiation (data not shown). To our knowledge, there is no report (or indication in available transcriptome profiles of senescent cells) that IL27 is induced during the development of senescence and produced by the senescent cells. This finding is unexpected, given that being a STAT1/STAT3 activator, IL27 might be expected to induce cellular senescence in a way similar to IFNγCitation43,44 or IL6.Citation30
Like IL8, IP10 and MCP1 are components of the senescence-associated secretome.Citation20,45 IP10/CXCL10 is also a protein of pleiotropic functions, involved in processes of chemoattraction, cell adhesion, and suggested to support proliferation of cancer cells.Citation46
It was reported that senescent cells are present in BM of MDS patients, as suggested from elevated senescence-associated β-galactosidase (SA-β-Gal) staining and induction of the cyclin-dependent kinase inhibitor p16INK4a in BM.Citation47 Moreover, Xiao et al. showed recently that the number of CD34+ cells positive for SA-β-Gal increased in lower-risk MDS patients and these CD34+ cells were positive for other traits of senescence, such as elevated reactive oxygen species, DNA damage and p21waf1/cip1.Citation48 Besides, several other studies reported the presence of DNA damage, persistent activation of DDR and NFκB signaling in BM of MDS patients.Citation49-53 In addition, it should be noted that prolonged DDR caused by various factors including activated oncogenes is a driver of (proinflammatory) cytokine elevation (for further details, see e.g. refs. Citation31, 54) implicated in the development of leukemia.Citation55
It is well known that several cytokine species are responsible for collateral DNA damage (bystander effect) induced by various settings such as radiotherapy (reviewed in ref. Citation56). Bystander effect is also thought to play an important role in the development of cancer and chronic diseases (reviewed in ref. Citation57). Cellular senescence as a precancerous stateCitation58,59 contributes to the bystander DNA damageCitation60 by secretion of several cytokines that can trigger DDR in the neighboring cells.Citation38,44 Strikingly, MCP1/CCL2 elevated in MDS patients, as shown here, is a factor considered to cause systemic DNA damage.Citation57,61-63 Its source in MDS patients may be both activated macrophagesCitation64 and senescent cells themselves. 5-AC-induced elevation of MCP1 (and IL8) observed in vitro and increasing levels of MCP1 (and IL8) in patients on 5-AC therapy is in line with the ability of 5-AC to induce DNA damageCitation28 and cellular senescence. Hence, the crosstalk among cytokine signaling, together with the ability of some cytokine pathways to induce DNA damage, can form an interlocked self-sustained networkCitation65 that may contribute to the genomic instability in BM responsible both for the development of MDS and its progression to AML. However, further studies are needed to support this hypothesis. Like MCP1, the levels of IL8 and IL27 were even higher in BM plasma in patients undergoing 5-AC therapy.
We also considered the contribution of the inflammatory comorbidities frequently associated with MDS to the elevated levels of IL8, IP10, MCP1 and IL27. Our data showed that inflammatory diseases represent a significant factor supporting the elevation of IL8 both in low-risk and high-risk patients and MCP1 in low-risk patients, but not of IL27 and IP10.
IL12, an early proinflammatory cytokine linking innate and adaptive immunity, represents one of the most important cytokines in antitumor immunity (reviewed in ref. Citation66). This cytokine is produced mainly by antigen-presenting cells, such as dendritic cells, macrophages, monocytes or B cells upon their activation via Toll/like receptor signaling. Here, we report downregulation of IL12 in BM plasma of both low-risk and high-risk MDS as well as in AML patients. Since IL12 is a principle cytokine produced by functional antigen-presenting cells, the observed decrease in its production suggests an impaired capacity to prime effective immune responses among MDS patients. Indeed, Wang et al. described that mesenchymal stem cells derived from high-risk MDS patients impaired functions of dendritic cells, including IL12 secretion.Citation67 On the other hand, levels of IFNγ, Th1 cytokine, which is a crucial mediator of the IL12-primed immune responses, were not significantly changed among the MDS patients, as compared to healthy individuals. So the clinical significance of the IL12 downregulation is unclear, especially considering the effects of another IL12-family member, IL27 that also plays a role in the Th1-response activation.
Due to the diverse pathophysiological functions of the IL8, IL27 and MCP1, we attempted to evaluate, whether their increase during the 5-AC therapy can serve as a prognostic factor of the therapeutic response. Although we observed indication that responders tend to harbor lower levels of IL8 during the course of therapy compared to non-responders, the relatively small sample size did not allow for a definitive conclusion.
Further work is needed to resolve the primary cause of DDR/senescence and impact of senescence and its proinflammatory component on progression of MDS to acute leukemia. Loss-of-function of the key DDR kinase ATM is frequently observed in lymphoproliferative diseases and the consecutive increase of tumor suppressor p19ARFCitation68 (due to loss of the ATM-regulated turnover of ARF protein in such tumors) can promote senescence as a secondary oncogenesis barrier. Indeed, some reports indicate that the loss of p19ARF has a negative impact on survival of AML patients (see e.g., reference Citation69) indicating that the p19ARF pathway may provide an oncogenesis barrier also in AML.
To conclude, all forms of MDS are associated with increased levels of several proinflammatory cytokines in the BM, a feature that is further augmented by therapy with 5-AC.
Materials and methods
Patients
In total, 265 BM samples from 188 patients diagnosed with MDS, AML or chronic myelomonocytic leukemia (CMML) were collected between 2009 and 2015. There were 125 samples from 94 patients with low-risk MDS (IPSS intermediate I or low) and 140 samples from 94 patients with high-risk MDS (IPSS high or intermediate II). Characteristics of patients are presented in . For controls, we used BM aspirates from eight healthy adult donors. Samples were obtained from patients diagnosed and treated at the Department of Haematology, 1st Faculty of Medicine, Charles University and General University Hospital. Written informed consent was provided in accordance with the Helsinki Declaration.
Table 1. Clinical characteristics of 188 patients with samples of BM plasma analyzed for cytokine content with 11-plex.
5-azacytidine-treated MDS cohort
As for BM analysis of 5-AC-treated patients, we collected repeated samples from 38 MDS patients (IPSS int I, int II or IPSS high). Characteristics of 5-AC-treated patients are in . 5-AC was administered subcutaneously for 7 d (regime 5-2-2) at a dose of 75 mg/m2/d. BM aspirates and PB were collected before 5-AC therapy and at day 7 after the completion of the 4th and 8th cycle, at which time the response to treatment was assessed according to IWG criteria.Citation70
Table 2. Patient characteristics.
Bone marrow and peripheral blood plasma collection
BM aspirates were obtained from the posterior iliac crest and collected into EDTA-coated vacutainers (BD 367844, Becton Dickinson) and PB was collected into vacutainers with Lithium heparin (BD 368824, Becton Dickinson) shortly prior to the BM aspiration. The patients were not subjected to any type of transfusion therapy 24 h prior to the BM aspiration. PB plasma and BM plasma were separated from cells shortly after the aspiration by centrifugation at 300 × g for 3 min, resulting in the separation of plasma, which was transferred into microcentrifuge tube and centrifuged again at 3300 × g for 3 min. Resulting supernatants of both BM and PB plasma were snap frozen on dry ice and stored at –80°C until the time of analysis.
Inflammatory cytokine measurement in bone marrow and blood plasma
Inflammatory cytokine content in BM plasma and/or PB plasma were measured using Human Inflammation 11-Plex (IFNγ, IL1α, IL1β, IL6, IL8, IL10, IL12p70, IL27, IP10, MCP1, TNFα; YSL Bioprocess Development Co.). The samples were measured on LSRII flow cytometer (BD Biosciences). The BM aspiration and handling of the healthy donor samples was identical to the patient samples. By doing so, we have reduced the vacutainer coating-related changes in cytokine profiles.Citation71 For the purposes of data analysis, the measurements above the upper detection limits of the assay were set to the value of detection limit and samples with no detected beads for a given cytokine were regarded as missing measurements.
Human Common Cytokines PCR Array (PAHS-021, Qiagen) was done as published previously.Citation20
Cell culture
Human cervical carcinoma cell line HeLa was cultured in Dulbecco's modified Eagle's medium (D-MEM) supplemented with 10% fetal bovine serum (FBS, Gibco, Thermo Fisher Scientific) and 100 U/mL penicillin/100 µg/mL streptomycin (Pen/Strep, Sigma-Aldrich). HPV-16 E6/E7-transformed human mesenchymal stem cell line HS-5 was cultured in Roswell Park Memorial Institute medium (RPMI-1640) supplemented with 10% FBS, 1% non-essential amino acids (NEAA; Sigma-Aldrich) and Pen/Strep (100 U/mL/100 µg/mL). Breast adenocarcinoma cell line MCF7 was cultured in D-MEM, 10% FBS and Pen/Strep (100 U/mL/100 µg/mL). Human promyelocytic leukemia cell line HL-60 was cultured in RPMI-1640, 10% heat-inactivated FBS, Pen/Strep (100 U/mL/100 µg/mL) and 1% NEAA. Human AML cell line OCI-M2 was cultured in Iscove's Modified Dulbecco's Medium supplemented with 20% heat-inactivated FBS, 1% NEAA and Pen/Strep (100 U/mL/100 µg/mL). Human AML cell line SKM-1 was cultured in RPMI-1640 supplemented with 20% heat-inactivated FBS, 1% NEAA and Pen/Strep (100 U/mL/100 µg/mL). Cells were kept at 37°C under 5% CO2 atmosphere and 95% humidity.
Bone marrow cultivation
Fresh BM aspirates from healthy donors (see above for BM aspiration procedure) was subjected to erythrocyte lysis via addition of pre-warmed NH4Cl lysis solution (156 mM NH4Cl, 17 mM NaCl, 126.6 µM EDTA) in 1: 50 ratio. The tube was inverted several times and incubated at 37°C for 10 min. The cells were then pelleted by centrifugation at 400 × g for 10 min at 4°C and subsequently washed with 1 × PBS. The BM mononucleated cells (BM-MNC) were then cultured at density of 0.5–1.2 × 106/mL culture medium for 4 d in RPMI-1640 supplemented with 15% FBS with low endotoxin content, 1% NEAA, Pen/Strep (100 U/mL/100 µg/mL) and 20 µg/mL stem cell factor (SCF; Sigma-Aldrich).
5-azacytidine administration
5-AC was dissolved in distilled water and kept at −80°C in 10 mM aliquots. Daily dose of 5-AC was administered to cell cultures for seven (human cell lines) or three (in vitro BM experiments) consecutive days and cells were harvested 24 h after the last treatment. The concentration of 5-AC was selected according to response of the individual cell type (HeLa, HS-5, MCF7, HL-60, OCI-M2, SKM-1) for the best ratio between the cell cycle block and apoptosis (not shown). The selected dose of 5-AC was in the lower end of the concentration range measured in BM after the administration of 5-ACCitation72: 2 µM for HeLa and MCF7, 0.5 µM for HS-5 and SKM-1, 1 µM for HL-60 and OCI-M2 and 4 µM for BM-MNCs, respectively.
Determination of inflammatory cytokines in cultivation media
The culture medium was collected 24 h after the change of fresh medium (and treatment with 5-AC when needed) and the number of cells per dish was counted. The concentration of secreted cytokines was measured using Human Inflammation 11-plex (YSL Bioprocess Development Co.) on flow cytometer LSRII (BD Biosciences) according to manufacturer's protocol. The cytokine levels were then calculated as pg/mL/105 cells.
Statistics
In vitro cytokine profiles
The obtained cytokine values in cultivation media for each cell line were compared to untreated sample via paired t-test. Analyses were conducted using the GraphPad Prism version 5.00 for Windows, GraphPad Software, San Diego California USA, www.graphpad.com.
5-azacytidine-treated patient cohort
For the purpose of data analysis, 5-AC-treated patients were divided into two groups depending on their response to therapy: responders (hematological improvement only, partial remission, complete remission, and complete remission with incomplete BM recovery) and non-responders (stable disease without HI, progressive disease) and the analyses of changes in cytokine levels were performed. The levels of cytokines in BM plasma of 5-AC-treated patients were compared to the cytokine levels in three other groups. First, eight healthy controls were used to measure the normal cytokine levels in the BM. Second, the BM plasma from 35 treatment-naive MDS patients with IPSS low was used to set the baseline values of the MDS cytokine profile. To assess the BM cytokine levels at the disease final stage, BM aspirates from 24 AML patients on best supportive care therapy that were not treated with hypomethylating agents at least 2 months prior to the BM aspiration and their prior BM aspirations were not included in 5-AC-treated cohort, were used. Obtained cytokine values were transformed using Box-Cox procedure and repeated measurements of 5-AC-treated patients were analyzed using robust linear model with mixed effects. Comparisons of 5-AC-treated patients, MDS low risk, AML patients and healthy controls were subjected to a Kruskal–Wallis ANOVA. p values < 0.05 were considered as statistically significant.
Patient cohort with respect to comorbidities
The enlarged cohort of BM samples included a total number of 125 BM aspirates from 94 low-risk MDS patients and 140 BM aspirates from 94 high-risk patients whose proinflammatory diseases and transfusion dependency were detected at the time of BM aspiration and their diagnostic subgroup and IPSS risk was re-evaluated according to the clinical results at the given time point. For this analysis, other factors such as the ongoing therapy with 5-AC, lenalidomide, hydroxyurea (Litalir) or arabinoside were not taken into account. Inflammatory comorbidities identified at the time of BM aspiration as the potential sources of proinflammatory cytokines are listed in .
Since we were unable to find differences in any of the measured cytokines between the high-risk (IPSS int II and high) MDS and patients at the AML stage, when not controlling for other proinflammatory parameters, we have conducted further analysis by treating the values from high-risk MDS and AML as one group.
Obtained cytokine values were transformed using Box-Cox procedure, and analyzed using robust linear model with mixed effects. The p values <0.05 were considered as statistically significant. Analyses were conducted using the R statistical package, version 3.1.2, R Core Team (2014).
Disclosure of potential conflicts of interest
No potential conflicts of interest were disclosed.
Author contributions
AM performed the research, analyzed the data and wrote the paper, SH and VM performed the research and analyzed the data, MV processed the BM samples, AJ, ZH and JB designed the study, analyzed and interpreted the data and wrote the paper, MR wrote the paper.
KONI_A_1183860_s02.pdf
Download PDF (685.3 KB)Acknowledgments
We would like to thank Václav Čapek for the statistical analysis and Kateřina Krejčíková for technical assistance. We would also like to express our gratitude to all the patients and healthy donors for their generous donation of the BM.
Funding
This study was supported by grant from Internal Grant Agency of Ministry of Health of the Czech Republic (Project NT/14174-3) and by Institutional grant (Project RVO 68378050) and Charles University grant PRVOUK 27/I. LF UK.
References
- Cogle CR, Saki N, Khodadi E, Li J, Shahjahani M, Azizidoost S. Bone marrow niche in the myelodysplastic syndromes. Leuk Res 2015; 39:1020-7; PMID:26276090; http://dx.doi.org/10.1016/j.leukres.2015.06.017
- Abe-Suzuki S, Kurata M, Abe S, Onishi I, Kirimura S, Nashimoto M, Murayama T, Hidaka M, Kitagawa M. CXCL12+ stromal cells as bone marrow niche for CD34+ hematopoietic cells and their association with disease progression in myelodysplastic syndromes. Lab Invest 2014; 94:1212-23; PMID:25199050; http://dx.doi.org/10.1038/labinvest.2014.110
- Balderman SR, Li AJ, Hoffman CM, Frisch BJ, Goodman AN, LaMere MW, Georger MA, Evans AG, Liesveld JL, Becker MW, et al. Targeting of the bone marrow microenvironment improves outcome in a murine model of myelodysplastic syndrome. Blood. 2016 Feb 4; 127(5):616-25; PMID:26637787; http://dx.doi.org/10.1182/blood-2015-06-653113
- Kristinsson SY, Bjorkholm M, Hultcrantz M, Derolf AR, Landgren O, Goldin LR. Chronic immune stimulation might act as a trigger for the development of acute myeloid leukemia or myelodysplastic syndromes. J Clin Oncol 2011; 29:2897-903; PMID:21690473; http://dx.doi.org/10.1200/JCO.2011.34.8540
- Ganan-Gomez I, Wei Y, Starczynowski DT, Colla S, Yang H, Cabrero-Calvo M, Bohannan ZS, Verma A, Steidl U, Garcia-Manero G. Deregulation of innate immune and inflammatory signaling in myelodysplastic syndromes. Leukemia 2015; 29:1458-69; PMID:25761935; http://dx.doi.org/10.1038/leu.2015.69
- Kurotaki H, Tsushima Y, Nagai K, Yagihashi S. Apoptosis, bcl-2 expression and p53 accumulation in myelodysplastic syndrome, myelodysplastic-syndrome-derived acute myelogenous leukemia and de novo acute myelogenous leukemia. Acta Haematol 2000; 102:115-23; PMID:10692673; http://dx.doi.org/10.1159/000040984
- Albitar M, Manshouri T, Shen Y, Liu D, Beran M, Kantarjian HM, Rogers A, Jilani I, Lin CW, Pierce S. Myelodysplastic syndrome is not merely “preleukemia”. Blood 2002; 100:791-8; PMID:12130488; http://dx.doi.org/10.1182/blood.V100.3.791
- Mangan JK, Luger SM. A paraneoplastic syndrome characterized by extremity swelling with associated inflammatory infiltrate heralds aggressive transformation of myelodysplastic syndromes/myeloproliferative neoplasms to acute myeloid leukemia: a case series. Case Rep Hematol 2012; 2012:582950; PMID:22928125; http://dx.doi.org/10.1155/2012/582950
- Anderson LA, Pfeiffer RM, Landgren O, Gadalla S, Berndt SI, Engels EA. Risks of myeloid malignancies in patients with autoimmune conditions. Br J Cancer 2009; 100:822-8; PMID:19259097; http://dx.doi.org/10.1038/sj.bjc.6604935
- Ramadan SM, Fouad TM, Summa V, Hasan S, Lo-Coco F. Acute myeloid leukemia developing in patients with autoimmune diseases. Haematologica 2012; 97:805-17; PMID:22180424; http://dx.doi.org/10.3324/haematol.2011.056283
- Al Ustwani O, Ford LA, Sait SJ, Block AM, Barcos M, Vigil CE, Griffiths EA, Thompson JE, Wang ES, Ambrus J Jr et al. Myelodysplastic syndromes and autoimmune diseases–case series and review of literature. Leuk Res 2013; 37:894-9; PMID:23692654; http://dx.doi.org/10.1016/j.leukres.2013.04.007
- Greenberg P, Cox C, LeBeau MM, Fenaux P, Morel P, Sanz G, Sanz M, Vallespi T, Hamblin T, Oscier D et al. International scoring system for evaluating prognosis in myelodysplastic syndromes. Blood 1997; 89:2079-88; PMID:9058730
- Silverman LR, Demakos EP, Peterson BL, Kornblith AB, Holland JC, Odchimar-Reissig R, Stone RM, Nelson D, Powell BL, DeCastro CM et al. Randomized controlled trial of azacitidine in patients with the myelodysplastic syndrome: a study of the cancer and leukemia group B. J Clin Oncol 2002; 20:2429-40; PMID:12011120; http://dx.doi.org/10.1200/JCO.2002.04.117
- Fenaux P, Mufti GJ, Hellstrom-Lindberg E, Santini V, Finelli C, Giagounidis A, Schoch R, Gattermann N, Sanz G, List A et al. Efficacy of azacitidine compared with that of conventional care regimens in the treatment of higher-risk myelodysplastic syndromes: a randomised, open-label, phase III study. Lancet Oncol 2009; 10:223-32; PMID:19230772; http://dx.doi.org/10.1016/S1470-2045(09)70003-8
- Xie M, Jiang Q, Xie Y. Comparison between decitabine and azacitidine for the treatment of myelodysplastic syndrome: a meta-analysis with 1,392 participants. Clin Lymphoma Myeloma Leuk 2015; 15:22-8; PMID:25042977; http://dx.doi.org/10.1016/j.clml.2014.04.010
- Kornblith AB, Herndon JE, 2nd, Silverman LR, Demakos EP, Odchimar-Reissig R, Holland JF, Powell BL, DeCastro C, Ellerton J, Larson RA et al. Impact of azacytidine on the quality of life of patients with myelodysplastic syndrome treated in a randomized phase III trial: a Cancer and Leukemia Group B study. J Clin Oncol 2002; 20:2441-52; PMID:12011121; http://dx.doi.org/10.1200/JCO.2002.04.044
- Sanchez-Abarca LI, Gutierrez-Cosio S, Santamaria C, Caballero-Velazquez T, Blanco B, Herrero-Sanchez C, García JL, Carrancio S, Hernández-Campo P, González FJ et al. Immunomodulatory effect of 5-azacytidine (5-azaC): potential role in the transplantation setting. Blood 2010; 115:107-21; PMID:19887673; http://dx.doi.org/10.1182/blood-2009-03-210393
- Goodyear OC, Dennis M, Jilani NY, Loke J, Siddique S, Ryan G, Nunnick J, Khanum R, Raghavan M, Cook M et al. Azacitidine augments expansion of regulatory T cells after allogeneic stem cell transplantation in patients with acute myeloid leukemia (AML). Blood 2012; 119:3361-9; PMID:22234690; http://dx.doi.org/10.1182/blood-2011-09-377044
- Hubackova S, Novakova Z, Krejcikova K, Kosar M, Dobrovolna J, Duskova P, Hanzlikova H, Vancurova M, Barath P, Bartek J et al. Regulation of the PML tumor suppressor in drug-induced senescence of human normal and cancer cells by JAK/STAT-mediated signaling. Cell Cycle 2010; 9:3085-99; PMID:20699642; http://dx.doi.org/10.4161/cc.9.15.12521
- Novakova Z, Hubackova S, Kosar M, Janderova-Rossmeislova L, Dobrovolna J, Vasicova P, Vancurova M, Horejsi Z, Hozak P, Bartek J et al. Cytokine expression and signaling in drug-induced cellular senescence. Oncogene 2010; 29:273-84; PMID:19802007; http://dx.doi.org/10.1038/onc.2009.318
- Feng X, Scheinberg P, Wu CO, Samsel L, Nunez O, Prince C, Ganetzky RD, McCoy JP Jr, Maciejewski JP, Young NS. Cytokine signature profiles in acquired aplastic anemia and myelodysplastic syndromes. Haematologica 2011; 96:602-6; PMID:21160069; http://dx.doi.org/10.3324/haematol.2010.030536
- Kornblau SM, McCue D, Singh N, Chen W, Estrov Z, Coombes KR. Recurrent expression signatures of cytokines and chemokines are present and are independently prognostic in acute myelogenous leukemia and myelodysplasia. Blood 2010; 116:4251-61; PMID:20679526; http://dx.doi.org/10.1182/blood-2010-01-262071
- Pardanani A, Finke C, Lasho TL, Al-Kali A, Begna KH, Hanson CA, Tefferi A. IPSS-independent prognostic value of plasma CXCL10, IL-7 and IL-6 levels in myelodysplastic syndromes. Leukemia 2012; 26:693-9; PMID:21912394; http://dx.doi.org/10.1038/leu.2011.251
- Curik N, Burda P, Vargova K, Pospisil V, Belickova M, Vlckova P, Savvulidi F, Necas E, Hajkova H, Haskovec C et al. 5-azacitidine in aggressive myelodysplastic syndromes regulates chromatin structure at PU.1 gene and cell differentiation capacity. Leukemia 2012; 26:1804-11; PMID:22343522; http://dx.doi.org/10.1038/leu.2012.47
- Mund C, Hackanson B, Stresemann C, Lubbert M, Lyko F. Characterization of DNA demethylation effects induced by 5-Aza-2′-deoxycytidine in patients with myelodysplastic syndrome. Cancer Res 2005; 65:7086-90; PMID:16103056; http://dx.doi.org/10.1158/0008-5472.CAN-05-0695
- Gore SD, Baylin S, Sugar E, Carraway H, Miller CB, Carducci M, Grever M, Galm O, Dauses T, Karp JE et al. Combined DNA methyltransferase and histone deacetylase inhibition in the treatment of myeloid neoplasms. Cancer Res 2006; 66:6361-9; PMID:16778214; http://dx.doi.org/10.1158/0008-5472.CAN-06-0080
- Palii SS, Van Emburgh BO, Sankpal UT, Brown KD, Robertson KD. DNA methylation inhibitor 5-Aza-2′-deoxycytidine induces reversible genome-wide DNA damage that is distinctly influenced by DNA methyltransferases 1 and 3B. Mol Cell Biol 2008; 28:752-71; PMID:17991895; http://dx.doi.org/10.1128/MCB.01799-07
- Fandy TE, Herman JG, Kerns P, Jiemjit A, Sugar EA, Choi SH, Yang AS, Aucott T, Dauses T, Odchimar-Reissig R et al. Early epigenetic changes and DNA damage do not predict clinical response in an overlapping schedule of 5-azacytidine and entinostat in patients with myeloid malignancies. Blood 2009; 114:2764-73; PMID:19546476; http://dx.doi.org/10.1182/blood-2009-02-203547
- Acosta JC, O'Loghlen A, Banito A, Guijarro MV, Augert A, Raguz S, Fumagalli M, Da Costa M, Brown C, Popov N et al. Chemokine signaling via the CXCR2 receptor reinforces senescence. Cell 2008; 133:1006-18; PMID:18555777; http://dx.doi.org/10.1016/j.cell.2008.03.038
- Kuilman T, Michaloglou C, Vredeveld LC, Douma S, van Doorn R, Desmet CJ, Aarden LA, Mooi WJ, Peeper DS. Oncogene-induced senescence relayed by an interleukin-dependent inflammatory network. Cell 2008; 133:1019-31; PMID:18555778; http://dx.doi.org/10.1016/j.cell.2008.03.039
- Rodier F, Coppe JP, Patil CK, Hoeijmakers WA, Munoz DP, Raza SR, Freund A, Campeau E, Davalos AR, Campisi J. Persistent DNA damage signalling triggers senescence-associated inflammatory cytokine secretion. Nat Cell Biol 2009; 11:973-9; PMID:19597488; http://dx.doi.org/10.1038/ncb1909
- Hsu HC, Lee YM, Tsai WH, Jiang ML, Ho CH, Ho CK, Wang SY. Circulating levels of thrombopoietic and inflammatory cytokines in patients with acute myeloblastic leukemia and myelodysplastic syndrome. Oncology 2002; 63:64-9; PMID:12187073; http://dx.doi.org/10.1159/000065722
- Scapini P, Lapinet-Vera JA, Gasperini S, Calzetti F, Bazzoni F, Cassatella MA. The neutrophil as a cellular source of chemokines. Immunol Rev 2000; 177:195-203; PMID:11138776; http://dx.doi.org/10.1034/j.1600-065X.2000.17706.x
- Desterke C, Martinaud C, Ruzehaji N, Le Bousse-Kerdiles MC. Inflammation as a keystone of bone marrow stroma alterations in primary myelofibrosis. Mediators of inflamm 2015; 2015:415024; PMID:26640324; http://dx.doi.org/10.1155/2015/415024
- Tefferi A, Vaidya R, Caramazza D, Finke C, Lasho T, Pardanani A. Circulating interleukin (IL)-8, IL-2R, IL-12, and IL-15 levels are independently prognostic in primary myelofibrosis: a comprehensive cytokine profiling study. J Clin Oncol 2011; 29:1356-63; PMID:21300928; http://dx.doi.org/10.1200/JCO.2010.32.9490
- Schinke C, Giricz O, Li W, Shastri A, Gordon S, Barreyro L, Bhagat T, Bhattacharyya S, Ramachandra N, Bartenstein M et al. IL8-CXCR2 pathway inhibition as a therapeutic strategy against MDS and AML stem cells. Blood 2015; 125:3144-52; PMID:25810490; http://dx.doi.org/10.1182/blood-2015-01-621631
- Blazkova H, Krejcikova K, Moudry P, Frisan T, Hodny Z, Bartek J. Bacterial Intoxication Evokes Cellular Senescence with Persistent DNA Damage and Cytokine Signaling. J Cell Mol Med 2010; 14:357-67; PMID:19650831; http://dx.doi.org/10.1111/j.1582-4934.2009.00862.x
- Hubackova S, Krejcikova K, Bartek J, Hodny Z. IL1- and TGFbeta-Nox4 signaling, oxidative stress and DNA damage response are shared features of replicative, oncogene-induced, and drug-induced paracrine ‘Bystander senescence’. Aging (Albany NY) 2012; 4:932-51; PMID:23385065; http://dx.doi.org/10.18632/aging.100520
- Kastelein RA, Hunter CA, Cua DJ. Discovery and biology of IL-23 and IL-27: related but functionally distinct regulators of inflammation. Annu Rev Immunol 2007; 25:221-42; PMID:17291186; http://dx.doi.org/10.1146/annurev.immunol.22.012703.104758
- Seita J, Asakawa M, Ooehara J, Takayanagi S, Morita Y, Watanabe N, Fujita K, Kudo M, Mizuguchi J, Ema H et al. Interleukin-27 directly induces differentiation in hematopoietic stem cells. Blood 2008; 111:1903-12; PMID:18042804; http://dx.doi.org/10.1182/blood-2007-06-093328
- Guzzo C, Che Mat NF, Gee K. Interleukin-27 induces a STAT1/3- and NF-kappaB-dependent proinflammatory cytokine profile in human monocytes. J Biol Chem 2010; 285:24404-11; PMID:20519510; http://dx.doi.org/10.1074/jbc.M110.112599
- Wong CK, Chen da P, Tam LS, Li EK, Yin YB, Lam CW. Effects of inflammatory cytokine IL-27 on the activation of fibroblast-like synoviocytes in rheumatoid arthritis. Arthritis Res Ther 2010; 12:R129; PMID:20604932; http://dx.doi.org/10.1186/ar3067
- Kim KS, Kang KW, Seu YB, Baek SH, Kim JR. Interferon-gamma induces cellular senescence through p53-dependent DNA damage signaling in human endothelial cells. Mech Ageing Dev 2009; 130:179-88; PMID:19071156; http://dx.doi.org/10.1016/j.mad.2008.11.004
- Hubackova S, Kucerova A, Michlits G, Kyjacova L, Reinis M, Korolov O, Bartek J, Hodny Z. IFN[gamma] induces oxidative stress, DNA damage and tumor cell senescence via TGF[beta]/SMAD signaling-dependent induction of Nox4 and suppression of ANT2. Oncogene 2015; 35:1236-49; PMID:25982278; http://dx.doi.org/10.1038/onc.2015.162
- Iannello A, Thompson TW, Ardolino M, Lowe SW, Raulet DH. p53-dependent chemokine production by senescent tumor cells supports NKG2D-dependent tumor elimination by natural killer cells. J Exp Med 2013; 210:2057-69; PMID:24043758; http://dx.doi.org/10.1084/jem.20130783
- Ejaeidi AA, Craft BS, Puneky LV, Lewis RE, Cruse JM. Hormone receptor-independent CXCL10 production is associated with the regulation of cellular factors linked to breast cancer progression and metastasis. Exp Mol Pathol 2015; 99:163-72; PMID:26079660; http://dx.doi.org/10.1016/j.yexmp.2015.06.002
- Wang YY, Cen JN, He J, Shen HJ, Liu DD, Yao L, Qi XF, Chen ZX. Accelerated cellular senescence in myelodysplastic syndrome. Exp Hematol 2009; 37:1310-7; PMID:19748549; http://dx.doi.org/10.1016/j.exphem.2009.09.002
- Xiao Y, Wang J, Song H, Zou P, Zhou D, Liu L. CD34+ cells from patients with myelodysplastic syndrome present different p21 dependent premature senescence. Leuk Res 2013; 37:333-40; PMID:23219618; http://dx.doi.org/10.1016/j.leukres.2012.11.006
- Novotna B, Bagryantseva Y, Siskova M, Neuwirtova R. Oxidative DNA damage in bone marrow cells of patients with low-risk myelodysplastic syndrome. Leuk Res 2009; 33:340-3; PMID:18687469; http://dx.doi.org/10.1016/j.leukres.2008.07.005
- Grosjean-Raillard J, Tailler M, Ades L, Perfettini JL, Fabre C, Braun T, De Botton S, Fenaux P, Kroemer G. ATM mediates constitutive NF-kappaB activation in high-risk myelodysplastic syndrome and acute myeloid leukemia. Oncogene 2009; 28:1099-109; PMID:19079347; http://dx.doi.org/10.1038/onc.2008.457
- Novotna B, Neuwirtova R, Siskova M, Bagryantseva Y. DNA instability in low-risk myelodysplastic syndromes: refractory anemia with or without ring sideroblasts. Hum Mol Genet 2008; 17:2144-9; PMID:18430715; http://dx.doi.org/10.1093/hmg/ddn113
- Sallmyr A, Fan J, Rassool FV. Genomic instability in myeloid malignancies: increased reactive oxygen species (ROS), DNA double strand breaks (DSBs) and error-prone repair. Cancer Lett 2008; 270:1-9; PMID:18467025; http://dx.doi.org/10.1016/j.canlet.2008.03.036
- Boehrer S, Ades L, Tajeddine N, Hofmann WK, Kriener S, Bug G, Ottmann OG, Ruthardt M, Galluzzi L, Fouassier C et al. Suppression of the DNA damage response in acute myeloid leukemia versus myelodysplastic syndrome. Oncogene 2009; 28:2205-18; PMID:19398952; http://dx.doi.org/10.1038/onc.2009.69
- Orjalo AV, Bhaumik D, Gengler BK, Scott GK, Campisi J. Cell surface-bound IL-1alpha is an upstream regulator of the senescence-associated IL-6/IL-8 cytokine network. Proc Natl Acad Sci U S A 2009; 106:17031-6; PMID:19805069; http://dx.doi.org/10.1073/pnas.0905299106
- Takacova S, Slany R, Bartkova J, Stranecky V, Dolezel P, Luzna P, Bartek J, Divoky V. DNA Damage Response and Inflammatory Signaling Limit the MLL-ENL-Induced Leukemogenesis In Vivo. Cancer Cell 2012; 21:517-31; PMID:22516260; http://dx.doi.org/10.1016/j.ccr.2012.01.021
- Klammer H, Mladenov E, Li F, Iliakis G. Bystander effects as manifestation of intercellular communication of DNA damage and of the cellular oxidative status. Cancer Lett 2013; PMID:24370566; http://dx.doi.org/10.1016/j.canlet.2013.12.017
- Martin OA, Redon CE, Nakamura AJ, Dickey JS, Georgakilas AG, Bonner WM. Systemic DNA damage related to cancer. Cancer Res 2011; 71:3437-41; PMID:21558390; http://dx.doi.org/10.1158/0008-5472.CAN-10-4579
- Bartkova J, Rezaei N, Liontos M, Karakaidos P, Kletsas D, Issaeva N, Vassiliou LV, Kolettas E, Niforou K, Zoumpourlis VC et al. Oncogene-induced senescence is part of the tumorigenesis barrier imposed by DNA damage checkpoints. Nature 2006; 444:633-7; PMID:17136093; http://dx.doi.org/10.1038/nature05268
- Di Micco R, Fumagalli M, Cicalese A, Piccinin S, Gasparini P, Luise C, Schurra C, Garre' M, Nuciforo PG, Bensimon A et al. Oncogene-induced senescence is a DNA damage response triggered by DNA hyper-replication. Nature 2006; 444:638-42; PMID:17136094; http://dx.doi.org/10.1038/nature05327
- Nelson G, Wordsworth J, Wang C, Jurk D, Lawless C, Martin-Ruiz C, von Zglinicki T. A senescent cell bystander effect: senescence-induced senescence. Aging Cell 2012; 11:345-9; PMID:22321662; http://dx.doi.org/10.1111/j.1474-9726.2012.00795.x
- Martin OA, Redon CE, Dickey JS, Nakamura AJ, Bonner WM. Para-inflammation mediates systemic DNA damage in response to tumor growth. Commun Integr Biol 2011; 4:78-81; PMID:21509186; http://dx.doi.org/10.4161/cib.13942
- Redon CE, Dickey JS, Nakamura AJ, Kareva IG, Naf D, Nowsheen S, Kryston TB, Bonner WM, Georgakilas AG, Sedelnikova OA. Tumors induce complex DNA damage in distant proliferative tissues in vivo. Proc Natl Acad Sci U S A 2010; 107:17992-7; PMID:20855610; http://dx.doi.org/10.1073/pnas.1008260107
- Bartek J, Mistrik M, Bartkova J. Long-distance inflammatory and genotoxic impact of cancer in vivo. Proc Natl Acad Sci 2010; 107:17861-2; PMID:20926747; http://dx.doi.org/10.1073/pnas.1013093107
- Kitagawa M, Saito I, Kuwata T, Yoshida S, Yamaguchi S, Takahashi M, Tanizawa T, Kamiyama R, Hirokawa K. Overexpression of tumor necrosis factor (TNF)-alpha and interferon (IFN)-gamma by bone marrow cells from patients with myelodysplastic syndromes. Leukemia 1997; 11:2049-54; PMID:9447819; http://dx.doi.org/10.1038/sj.leu.2400844
- Bartek J, Hodny Z, Lukas J. Cytokine loops driving senescence. Nat Cell Biol 2008; 10:887-9; PMID:18670449; http://dx.doi.org/10.1038/ncb0808-887
- Tugues S, Burkhard SH, Ohs I, Vrohlings M, Nussbaum K, Vom Berg J, Kulig P, Becher B. New insights into IL-12-mediated tumor suppression. Cell Death Differ 2015; 22:237-46; PMID:25190142; http://dx.doi.org/10.1038/cdd.2014.134
- Wang Z, Tang X, Xu W, Cao Z, Sun L, Li W, Li Q, Zou P, Zhao Z. The different immunoregulatory functions on dendritic cells between mesenchymal stem cells derived from bone marrow of patients with low-risk or high-risk myelodysplastic syndromes. PLoS One 2013; 8:e57470; PMID:23469196; http://dx.doi.org/10.1371/journal.pone.0057470
- Velimezi G, Liontos M, Vougas K, Roumeliotis T, Bartkova J, Sideridou M, Dereli-Oz A, Kocylowski M, Pateras IS, Evangelou K et al. Functional interplay between the DNA-damage-response kinase ATM and ARF tumour suppressor protein in human cancer. Nat Cell Biol 2013; 15:967-77; PMID:23851489; http://dx.doi.org/10.1038/ncb2795
- Muller-Tidow C, Metzelder SK, Buerger H, Packeisen J, Ganser A, Heil G, Kügler K, Adigüzel G, Schwäble J, Steffen B et al. Expression of the p14ARF tumor suppressor predicts survival in acute myeloid leukemia. Leukemia 2004; 18:720-6; PMID:14973498; http://dx.doi.org/10.1038/sj.leu.2403296
- Cheson BD, Greenberg PL, Bennett JM, Lowenberg B, Wijermans PW, Nimer SD, Pinto A, Beran M, de Witte TM, Stone RM et al. Clinical application and proposal for modification of the International Working Group (IWG) response criteria in myelodysplasia. Blood 2006; 108:419-25; PMID:16609072; http://dx.doi.org/10.1182/blood-2005-10-4149
- Tvedt TH, Rye KP, Reikvam H, Brenner AK, Bruserud O. The importance of sample collection when using single cytokine levels and systemic cytokine profiles as biomarkers–a comparative study of serum versus plasma samples. J Immunol Methods 2015; 418:19-28; PMID:25637409; http://dx.doi.org/10.1016/j.jim.2015.01.006
- Rudek MA, Zhao M, He P, Hartke C, Gilbert J, Gore SD, Carducci MA, Baker SD. Pharmacokinetics of 5-azacitidine administered with phenylbutyrate in patients with refractory solid tumors or hematologic malignancies. J Clin Oncol 2005; 23:3906-11; PMID:15851763; http://dx.doi.org/10.1200/JCO.2005.07.450