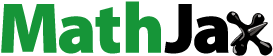
ABSTRACT
Cryoablation is one of treatment modalities for kidney cancer and is expected to induce strong local immune responses as well as systemic T-cell-mediated immune reactions that may lead to the regression of distant metastatic lesions. Thus, the characterization of T cell repertoire and immune environment in tumors before and after treatment should contribute to the better understanding of the cryoablation-induced anticancer immune responses. In this study, we collected tumor tissues from 22 kidney cancer patients, before cryoablation and at 3 mo after cryoablation. In addition, blood samples were collected from 14 patients at the same time points. We applied a next generation sequencing approach to characterize T cell receptor β (TCRB) repertoires using RNAs isolated from tumor tissues and peripheral blood mononuclear cells. TCRB repertoire analysis revealed the expansion of certain T cell clones in tumor tissues by cryoablation. We also found that proportions of abundant TCRB clonotypes (defined as clonotypes with ≥ 1% frequency among total TCRB reads) were significantly increased in the post-cryoablation tissue samples than those of pre-cryoablation tumor samples. Some of these TCRB clonotypes were found to be increased in peripheral blood. Expression analysis of immune-related genes in the tissues of pre- and post-cryoablation showed significantly elevated transcriptional levels of CD8+, CD4+, Granzyme A (GZMA), and CD11c along with a high CD8/FOXP3 ratio in the post-cryoablation tissue samples. Our findings revealed that cryoablation could induce strong immune reactions in tumors with oligoclonal expansion of antitumor T cells, which circulate systemically.
Abbreviations
CDR3 | = | complementarity determining region 3 |
HLA | = | human leucocyte antigen |
PBMC | = | peripheral blood mononuclear cells |
TCR | = | T cell receptor |
TCRB | = | T cell receptor β. |
Introduction
Cryoablation is one of promising cancer treatments and has some advantages over other modalities such as minimum invasiveness and less damage to surrounding structures.Citation1,Citation2 As this technology has been improved over the decades, cryoablation is now widely used in the treatment of various types of cancer including kidney cancer.Citation3-10 Interestingly, the treatment causes not only local elimination of tumors, but also sometimes causes regression of distant and untreated tumor legions after certain time lag.Citation11,Citation12 These findings evoke a hypothesis that the cryoablation may induce strong and long-lasting anticancer immune responses in the body. So far, this unique feature of cryoablation has been reproduced in animal models in which the prevention effects for re-challenge of tumor cells or regression of untreated tumors were mainly examined.Citation11,Citation13 In human, only a few studies have examined the cryoablation-induced immune response showing an increase of interferon-γ-producing T cells against human cancer cell lines.Citation14,Citation15 However, the molecular mechanisms of the immune response, particularly for T cells that play fundamental roles in attacking cancer cells, still remain unclear.
T cell receptors (TCR), most of which are a heterodimer of TCR α (TCRA) and β (TCRB) chains, are present on the surface of T cells. To recognize a huge number of antigens, TCR genes undergo somatic V-(D)-J recombination that generates a huge diversity of T cell repertoire.Citation16,Citation17 To obtain comprehensive T cell repertoire information in various disease conditions including cancer, we recently established a TCR analysis method with next-generation sequencers using RNAs extracted from blood, ascites, and tumor tissues.Citation18-24
In the present study, we demonstrated that cryoablation could induce strong immune reactions characterized by the expansion of oligoclonal T cells in tumor tissues. Interestingly, an increase of some T cell clonotypes, which were expanded in tumor tissues, was also detected in peripheral blood, indicating a possibility of systemic antitumor response that might attack cancer cells in distant tumor lesions (and possibly circulating tumor cells). Furthermore, we found the infiltration of a large number of CD11c-positive cells, probably representing macrophages and dendritic cells into the post-cryoablation tissues. Collectively, TCR repertoire analysis and immune profiling of cryoablation-treated cancer patients may lead to a better understanding of the cryoablation-induced anticancer immune response.
Results
Clonal T-cell expansion in tumors after cryoablation
To examine the effect of cryoablation on T cell repertoire in tumor tissues, we performed TCRB sequencing on pre- and post-cryoablation tissue samples obtained from 22 kidney cancer patients. The clinical characteristics of all patients are summarized in . Through cDNA sequencing of TCRB, we obtained total sequence reads of 1,132,906 ± 815,953 (average ± one standard deviation) mapped to V, D, J, and C segments for TCRB (Table S1). From these TCRB reads, we identified unique TCRB complementarity determining region 3 (CDR3) clonotypes of 34,487 ± 41,724 in individual tissues. After sorting out CDR3 clonotypes according to their frequencies in tumor tissue samples, we counted the proportions of abundant CDR3 clonotypes, which were defined as the clonotypes with the frequency of 1% or higher, in each tumor tissue, and found that the sum of the abundant CDR3 clonotypes was increased by cryoablation in 15 of the 22 cases (); in the remaining seven tumor tissues, four patients (CK1, CK7, CK30 and CK31) revealed the very strong clonal expansion of T cells in pre-cryoablation tumor tissues. Overall, on average, the sum of abundant TCRB CDR3 clonotypes (defined by frequency of ≥ 1%) was significantly increased from 27.3% ± 27.4% in pre- cryoablation tumor tissues to 46.4% ± 31.0% in post-cryoablation tissues (p = 0.024, ). We also measured the TCR diversity by calculating the inverse Simpson's diversity index (1/Ds) that becomes high when TCR clones are evenly distributed whereas becomes low with the enrichment of certain T cell clones. As expected, the diversity indexes for TCRB were significantly lower in tissues after cryoablation in comparison with those of pre-treatment tumor samples (p = 0.021, ). We further investigated whether TCRB CDR3 clonotypes enriched in post-cryoablation tissues were present in pre-cryoablation tumors in 15 cases that had significant increase in certain CDR3 clonotypes by cryoablation. As shown in and Table S2, most of the enriched CDR3 clonotypes in post-cryoablation tissues were absent (bars in red color) or rarely present (bars in green color) in pre-treatment tumors, suggesting that the death of cancer cells induced by the cryoablation activated T lymphocytes that probably recognize cancer-specific antigens.
Table 1. Clinical information of 22 kidney cancer patients performed cryoablation
Figure 1. Clonal T cell expansion in post-cryoablation tissues. (A) The distribution of the abundant TCRB CDR3 clonotypes with the read frequency of 1% or higher is presented in pie charts (green color: pre-cryoablation; blue color: post- cryoablation). Gray color indicates portion of CDR3 clonotypes below the read frequency of 1%. (B) The total proportion of the abundant TCRB CDR3 clonotypes with the frequency of 1% or higher was significantly increased in post-cryoablation tissues (p = 0.024). (C) After cryoablation, tissue samples revealed significantly lower TCRB diversity index compared with that in pre-cryoablation (p = 0.021). The Mann—Whitney U test was performed for the comparison of total proportion of the most abundant CDR3 clonotypes (frequency of ≥ 1%) and the diversity index of TCRB between pre- and post-cryoablation.
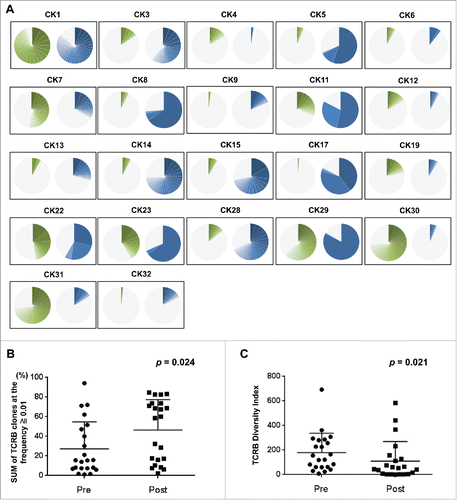
Figure 2. Cryoablation stimulated expansion of unique T cell repertoires in tumor tissues. (A) The 20 most abundant TCRB CDR3 clonotypes newly appeared or drastically increased in the tissues after cryoablation (green bars: clonotypes that were rarely present in pre- cryoablation tissues; red bars: clonotypes that were completely absent in pre-cryoablation tissues; blue bars: 20 most abundant clonotypes observed in post-cryoablation tissues). Figures are from 15 representative cases that revealed a drastic increase of oligoclonal CDR3 sequences in post-cryoablation tissues in . (B) One TCRB CDR3 clonotype was shared in two patients with HLA-A24:02 genotype. Yellow-color bars indicate the identical CDR3 clonotype.
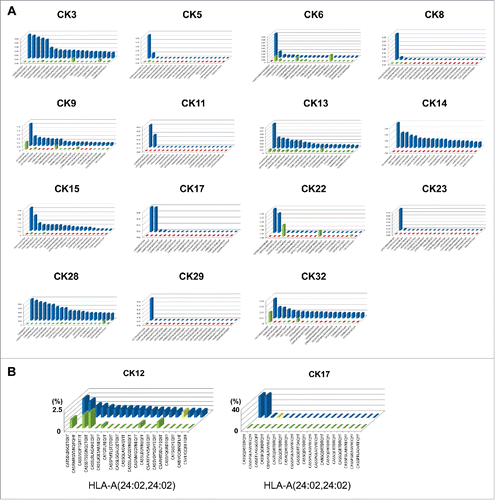
We subsequently examined the presence of common CDR3 clonotypes among patients by comparison of 20 most abundant CDR3 clonotypes of each tissue sample. We found that one TCRB CDR3 clonotype was shared in post-cryoablation tissues of patients who have the same HLA class I subtype, HLA-A24:02 ().
Unique TILs are also detected in systemic circulation
Accumulating evidences have suggested that cryoablation can stimulate adaptive and systemic antitumor immune responses.Citation11,Citation13-15 We further examined the frequencies of TCRB CDR3 clonotypes, which were enriched in post-cryoablation tissues, in peripheral blood samples. Among 14 patients from which we obtained PBMC samples, we examined 10 patients (CK3, CK5, CK8, CK9, CK14, CK15, CK17, CK22, CK28, and CK32) who showed the expansion of oligoclonal TCRB CDR3 clonotypes (frequency of ≥ 1%) in post-cryoablation tissues. As a result, certain TCRB CDR3 clonotypes in each sample were significantly increased at 3 mo after the cryoablation compared with pre-cryoablation samples of PBMC (p values less than 3.6 × 10−4 were considered to be significant as described in Materials and methods, and Fig. S1). These findings may indicate the presence of the cryoablation-induced T cell-mediated systemic anticancer immune responses.
Figure 3. A set of T cell clones identified in both tumor tissues and systemic circulation. Heatmaps showed the distribution of TCRB CDR3 clonotypes in PBMC samples (the top 20 CDR3 clonotypes of post-cryoablation tissue were selected) of five patients who had dominant T cell clones (frequency of ≥ 10%) in post-cryoablation tissues. The asterisks indicate CDR3 clonotypes that were statistically significantly increased at 3 mo post-cryoablation compared with pre-cryoablation samples of PBMC. +, ++ and +++ indicate CDR3 clonotypes that had frequency of 10—20%, 20—30% and more than 30%, respectively.
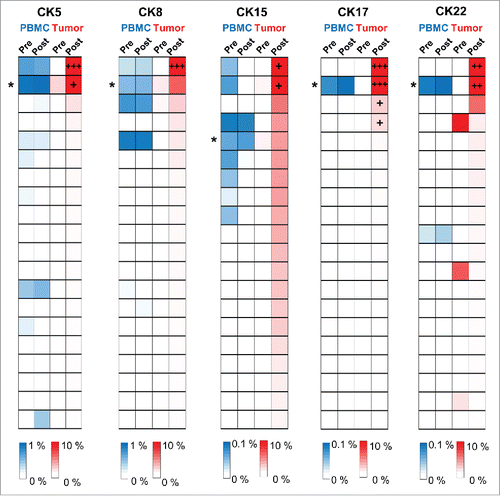
Antitumor immune-related genes are upregulated after cryoablation
To characterize changes of T cell subtypes in the tissues, we examined transcriptional levels of CD3, CD4, CD8, FOXP3, GZMA, PRF1, IDO1, IL10, TIM3, CD11C, and HMGB1 in the tumors with the real-time RT-PCR method (). Higher expression levels of CD8, GZMA, and PRF1 are considered as higher cytolytic activity in tumor tissues, while those of FOXP3, IDO1, IL10, and TIM3 represent higher immunosuppressive activity. For each of these molecules, we calculated the ratio of post/pre-cryoablation expression levels. In the tissues after cryoablation, we found significant increase of CD4, CD8, and GZMA expression levels, implying that CD4+ or CD8+ T cells were strongly infiltrated and/or expanded by cryoablation. The ratio of CD8/FOXP3 expression was significantly elevated, while that of FOXP3/CD4 became significantly decreased, in post-cryoablation tissues compared with the pre-treatment tumors. With respect to the immunosuppressive markers, IDO1 expression was significantly decreased after cryoablation, whereas TIM3 was increased. We also evaluated correlation between CD8 and HLA-A expression levels as a representative of HLA class I molecule as HLA class I molecules are known to play essential roles in cancer cells for the presentation of tumor-associated antigens. As shown in , there was a significant correlation (p = 0.0085) between CD8 and HLA-A expression levels in pre-cryoablation tumor tissues, although the correlation between CD4 and HLA-DRB1 (one of the three major HLA class II molecules) was not observed (p = 0.47, ). We also found that the post/pre-cryoablation ratio of the sum of abundant TCRB CDR3 clonotypes (defined by frequency of ≥ 1%) in tissues was significantly correlated with HLA-A expression in pre-cryoablation tumor tissues (p = 0.046, ). In addition, these HLA-A expression levels in pre-cryoablation tumor tissues were higher in tissues sample where the expansion of certain TCRB CDR3 clonotypes was observed (p = 0.032, ). These results indicate that the basal expression level of HLA class I molecules is an important factor to effectively induce clonal expansion of T cells by cryoablation.
Figure 4. Expression of immune-related genes in tumor tissues. (A) Expression level of each gene was calculated relative to that of GAPDH. The x-axis indicates the ratio of post-cryoablation /pre-cryoablation in the log2 transformed values. *p < 0.01, **p < 0.05 indicates the significant difference by the Mann—Whitney U test. (B) and (C) Correlation analysis between HLA-A and CD8 expression or HLA-DRB1 and CD4 expression in pre-cryoablation tumor tissues. HLA-A and HLA-DRB1 represent one of the major HLA class I and class II molecule, respectively. (D) Correlation analysis between HLA-A expression in pre-cryoablation tumor tissues and the post/pre-cryoablation ratio of the sum of the abundant TCRB CDR3 clonotypes (frequency of ≥ 1%). (E) Comparison of basal HLA-A expression levels in the pre-cryoablation tumor tissues where total proportion of the abundant TCRB CDR3 clonotypes (frequency of ≥ 1%) were decreased (left) or increased (right) by cryoablation.
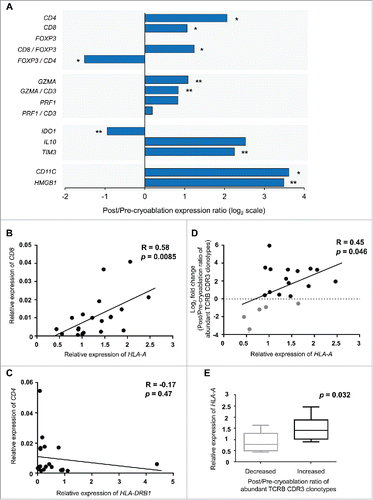
Drastic increase of CD11c+ cells (macrophages and dendritic cells) in post-cryoablation tissues
To further investigate the changes of antigen-presenting cells in the tissues, we evaluated CD11c+ cells that represent macrophages and dendritic cells by transcriptional levels and immunohistochemcal analysis. As shown in , we found that CD11C transcriptional levels were drastically increased in the post-cryoablation tissues, indicating that a large number of macrophages and dendritic cells infiltrated into tumor tissues by the stimulation of cryoablation. We also found the increase of HMGB1 expression level probably through the induction of damage-associated molecular patterns (DAMPs) that were released from the dead/damaged cancer cells. Through the immunohistochemistry (IHC) analysis of CD8 and CD11c on a subset of samples (, Fig S2), we confirmed the significant increase of infiltrated CD8+ T and CD11c+ cells in post-cryoablation tissues.
Figure 5. Drastic increase of CD8+ T cells and CD11c+ cells in post-cryoablation tissues. Representative immunohistochemistry results for CD8 (A) and CD11c (B) staining in tumor tissues of case CK3 before and after cryoablation. Magnification: 200 × for upper row, 400 × for lower row. Scale bars = 200 μm.
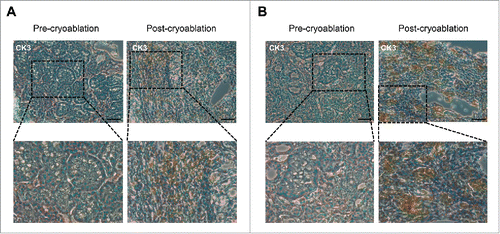
Discussion
Significant improvement in cryoablation devices has provided some advantages in cryoablation treatment compared with the standard surgical treatment; cryoablation reveals minimum invasiveness with the preservation of organ function.Citation1,Citation2 In addition to these benefits, cryoablation sometimes induces the shrinkage of untreated tumor lesions.Citation11,Citation12 However, the molecular mechanism of anticancer immune reaction generated by cryoablation is still largely unknown. For the better understanding of the cryoablation-induced anticancer immune reactions, many animal models have been tested; for example, Gazzaniga et al. reported the infiltration of macrophages and dendritic cells into the peritumoral areas at an early time point after cryoablation.Citation25 In addition, cryoablation was indicated to activate tumor-specific T cells in tumor draining lymph nodes.Citation26 In this manuscript, we performed TCRB repertoire profiling combined with the expression analysis of immune-related genes in tumor tissues treated with cryoablation to investigate the local and systemic T cell responses after cryoablation in human patients.
Through TCRB repertoire analysis in this study, we have demonstrated several novel findings that may explain cryoablation-induced anticancer immune responses. First, we found that a small subset of T cells was significantly expanded in tumor tissues after cryoablation. Most of these T cell clones were not detected in pre-cryoablation tissues, suggesting that cryoablation strongly stimulated local immune responses, possibly against cancer cells, in tumor tissues. Interestingly, some of these T cell clones were also detected in peripheral blood, indicating that clonally expanded T cells can circulate systemically in the body. These findings may partly explain the mechanism why cryoablation can sometimes induce the regression of untreated tumor legions, and propose future application of cryoablation in the cancer patients having metastatic lesions, preferably in the combination of cancer vaccine treatment and/or immune checkpoint blockade. Second, we detected drastic increase of CD11c+ cells (macrophages and dendritic cells) in post-cryoablation tumor tissues. By freezing and thawing cycles of cancer tissues, extracellular and intracellular ice crystals mechanically cause the collapse of cancer cells. However, unlike the radiofrequency ablation therapy, cancer cell death by freezing may preserve the antigenicity of cancer-cell-specific molecules, possibly due to the minimum effect on the denaturation of proteins in cancer cells.Citation27-30 In addition, tissue damages may have produced DAMPs, as evidenced by the increased level of HMGB1, which can activate immune-response characterized by the infiltration of macrophages and dendritic cells,Citation31,Citation32 in post-cryoablation tissues in our study. Third, we found that HLA-A expression levels in the cancer tissues were significantly correlated with CD8+ expression levels ( = the extent of CD8 T cell infiltration) in pre-cryoablation tumor tissues. It is well known that CD8+ T cells play a central role in cancer immunosurveillance and the loss or downregulation of HLA class I molecule expression is one of the major mechanisms that cancer cells escape from the host immune system.Citation33,Citation34 We previously reported that intratumoral high expression of HLA-A might be one of the predictive markers for clinical responses to anti-PD-1 therapy in metastatic melanoma.Citation22 In the present study, the high expression level of HLA-A in tumor tissues also revealed correlation with the cryoablation-induced oligoclonal expansion of T cells. These results further support the hypothesis that HLA class I expression levels might be one of important determinants for strong T cell-mediated anticancer immune response by cryoablation.
Finally, we found that the expression of TIM3, which is an immune suppressive molecule against T cell responses,Citation35,Citation36 was significantly increased in post-cryoablation tissues. Several studies involving mice or human have shown that chronic infections induced high expression levels of TIM3 as the negative feedback system after the activation of CD8+ and CD4+ T cells.Citation37-41 Because these tissue samples in our study were collected 3 mo after cryoablation, the increased expression of TIM3 might reflect the activation of the negative feedback mechanism in tumor tissues at the time of tissue collection.
In summary, our results imply that cryoablation could induce both local and systemic immune responses associated with the infiltration of CD11c-positive cells and oligoclonal expansion of (possible) antitumor T cells. For the translation into clinics, unique TCR sequences identified in post-cryoablation tissues in individual patients can be applied to personalized TCR-engineered T cell therapy.
Materials and methods
Study design
Between June 2014 and August 2016, a total of 22 kidney cancer patients were enrolled in this study. All patients were confirmed to have kidney cancer by needle biopsy before cryoablation. Computed tomography (CT)-guided percutaneous cryoablation was performed using CRYO-HIT System (Galil Medical Ltd, Yokneam, Israel) under local anesthesia. Multiple (typically, three) 17 gauge probes were percutaneously inserted under CT fluoroscopy guidance, and ice ball formation was monitored by CT imaging to ensure a > 5 mm safety margin around the renal tumor. Two freezing and thawing cycles of cryoablation were routinely repeated to increase the tumor cell injury. At 3 mo after cryoablation, all patients underwent follow-up dynamic CT and needle biopsy to confirm no residual tumors.
Tumor tissues were collected at the time points of pre-cryoablation and 3 mo after cryoablation. In addition, peripheral blood samples were obtained from 14 patients (CK1, CK3, CK5, CK8, CK9, CK14, CK15, CK17, CK19, CK22, CK28, CK30, CK31, and CK32) at the time points of pre- cryoablation and 3 mo after cryoablation. The study protocol was approved by the Institutional Review Board of University of Chicago (approval number 13—0797) and Osaka University (approval number 14265). All patients provided written informed consent.
Patient characteristics
The clinical characteristics of all patients are summarized in . Twenty-one tumors were histologically diagnosed as clear cell renal cell carcinoma and one was as a chromophobe renal cell carcinoma. Patients’ ages were 52—88. The median size of tumor was 23 mm with a range of 14 —38 mm at clinical stage I. No recurrence was detected with the median follow-up period of 13.2 mo (range: 3.7—26.7 mo). To evaluate kidney function, we calculated the estimated glomerular filtration rate (eGFR) before and 3 mo after cryoablation. The mean rate of kidney functional deterioration was 6.2%. Perirenal hematoma developed in two patients that spontaneously regressed without any interventions.
TCR sequencing
Total RNAs from tumor tissues and PBMC were isolated using AllPrep DNA/RNA Mini kit (Qiagen, Valencia, CA). Sequencing libraries of TCRB were prepared as described previously,Citation41,Citation42 and subjected to sequencing on the Illumina Miseq platform, using 600 cycles Miseq Reagent Kit V3 (Illumina, San Diego, CA).
Sequence analysis
To identify V, D, J, and C segments in individual TCRB sequencing reads, each of the sequence reads in FASTQ files was mapped to the reference sequences provided by IMGT/GENE-DBCitation43 using Bowtie2 aligner (Version 2.1.0).Citation44,Citation45 Raw FASTQ files were analyzed using Tcrip software.Citation41
Human leukocyte antigen (HLA) typing
Genomic DNA was isolated from PBMC using AllPrep DNA/RNA Mini kit (Qiagen). PCR amplicon-based high-resolution typing on MiSeq (Illumina) was performed for HLA-A, HLA-B, and HLA-C, in Scisco Genetics Inc. (Seattle, WA).Citation46 HLA genotypes of all patients were summarized in Table S3.
Gene expression analysis
cDNA was synthesized from RNA using Superscript III first-strand synthesis kit (Invitrogen, Carlsbad, CA). Real-time RT-PCR was performed using Taqman gene expression assay (Life Technologies, Grand Island, NY) in the ABI ViiA 7 system (Applied Biosystems, Foster City, CA), according to the manufacturer's instructions. The TaqMan probes are listed in Table S4. Since we used total amount of mRNA for TCR sequencing in two cases (CK1 and CK4), these were excluded from the analysis.
Immunohistochemical studies
Paraffin-embedded tissues were cut into 5-μm-thick sections and tissue slides were stained on Leica Bond RX automatic stainer (Leica Biosystems, Wetzlar, Germany). Epitope retrieval solution I (Leica Biosystems, Wetzlar, Germany) was used for 20 min treatment. Anti-human CD8 antibody (clone C8/144B, Dako, Carpinteria, CA) or anti-human CD11c antibody (clone 5D11, Leica Biosystems) were applied on tissue sections for 25 min incubation and the antigen-antibody binding was visualized by Bond polymer refine detection solution (Leica Biosystems) according to manufacturer's protocols.
Statistical analysis
The diversity index (inverse Simpson's index) in CDR3 sequences was calculated as follows:where K is the total number of CDR3 clonotypes, ni is the number of sequences belonging to the ith clonotype, and N is the total number of identified CDR3 sequences.
The Mann—Whitney U test (two-tailed) was performed for the comparison of total proportion of the most abundant CDR3 clonotypes (≥ 1% frequency among total TCRB reads), the diversity index of TCRB, gene expression levels between pre- and post- cryoablation, correlations between HLA-A and CD8 or HLA-DRB1 and CD4 expression in pre-cryoablation tissues and the correlation between HLA-A expression and post/pre-cryoablation ratio of total proportion of the most abundant CDR3 clonotypes (frequency of ≥ 1%) using GraphPad Prism version 6.0 (GraphPad software, La Jolla, CA). To analyze the changes of CDR3 clonotypes in PBMC, we focused on a total of 139 abundant CDR3 clonotypes (≥ 1% frequency among total TCRB reads) that were expanded in post-cryoablation tissues in 10 cases (CK3, CK5, CK8, CK9, CK14, CK15, CK17, CK22, CK28, and CK32). Then, we applied Fisher's exact test to examine the significance in the increase of each of these CDR3 clonotype in PBMC samples. In this analysis, we set a significant level of p value < 3.6 × 10−4 (0.05 of 139) according to the Bonferroni correction. In other analyses, differences were considered significant at p value < 0.05.
Disclosure of potential conflicts of interest
No potential conflicts of interest were disclosed.
Author Contributions
Y.N. planned and supervised the entire project; J.P. provided the study design and the working hypothesis and completed the article; T.K. designed the study, conducted experiments, performed data analysis, and drafted the article; T.I. conducted experiments and performed data analysis; Y.I. and K.K. conducted data analysis and provided scientific advice; N.N. and U.M. provided the study design and completed the article; A.N. performed data analysis and provided scientific advice; H.H. and K.O. performed cryoablation and completed the article.
Supplementary_materials.zip
Download Zip (1.4 MB)Acknowledgments
We thank Dr. Mutsumi Tsuchiya for samples processing and data collection. We also thank Drs. Rui Yamaguchi, Seiya Imoto, and Satoru Miyano in The University of Tokyo for developing the algorithm of TCR repertoire analysis and helpful support in data management. The super-computing resource (http://sc.hgc.jp/shirokane.html) was provided by Human Genome Center, the Institute of Medical Science, The University of Tokyo.
References
- Lanza E, Palussiere J, Buy X, Grasso RF, Beomonte Zobel B, Poretti D, Pedicini V, Balzarini L, Cazzato RL. Percutaneous image-guided cryoablation of breast cancer: a systematic review. J Vasc Interv Radiol 2015; 26:1652-7 e1; PMID:26342882; https://doi.org/10.1016/j.jvir.2015.07.020
- Rodriguez Faba O, Akdogan B, Marszalek M, Langenhuijsen JF, Brookman-May S, Stewart GD, Capitanio U, Sanguedolce F, Renal cancer working group of the Young Academic Urologists Working Party of the European Association of Urology. Current status of focal cryoablation for small renal masses. Urology 2016; 90:9-15; PMID:26743392; https://doi.org/10.1016/j.urology.2015.11.041
- Levy D, Avallone A, Jones JS. Current state of urological cryosurgery: prostate and kidney. BJU Int 2010; 105:590-600; PMID:20149203; https://doi.org/10.1111/j.1464-410X.2010.09235.x
- Ritch CR, Katz AE. Prostate cryotherapy: current status. Current Opinion Urol 2009; 19:177-81; PMID:19195130; https://doi.org/10.1097/MOU.0b013e32831e16ce
- Bhardwaj N, Gravante G, Strickland AD, Ahmad F, Dormer J, Dennison AR, Lloyd DM. Cryotherapy of the liver: a histological review. Cryobiology 2010; 61:1-9; PMID:20599888; https://doi.org/10.1016/j.cryobiol.2010.06.003
- Littrup PJ, Jallad B, Chandiwala-Mody P, D'Agostini M, Adam BA, Bouwman D. Cryotherapy for breast cancer: a feasibility study without excision. J Vasc Interv Radiol 2009; 20:1329-41; PMID:19800542; https://doi.org/10.1016/j.jvir.2009.06.029
- Sabel MS, Kaufman CS, Whitworth P, Chang H, Stocks LH, Simmons R, Schultz M. Cryoablation of early-stage breast cancer: work-in-progress report of a multi-institutional trial. Ann Surg Oncol 2004; 11:542-9; PMID:15123465; https://doi.org/10.1245/ASO.2004.08.003
- Inoue M, Nakatsuka S, Jinzaki M. Cryoablation of early-stage primary lung cancer. BioMed Res Int 2014; 2014:521691; PMID:24991559; https://doi.org/10.1155/2014/521691
- Pua BB, Thornton RH, Solomon SB. Ablation of pulmonary malignancy: current status. J Vasc Interv Radiol 2010; 21:S223-32; PMID:20656232; https://doi.org/10.1016/j.jvir.2010.01.049
- Kokoszka A, Scheinfeld N. Evidence-based review of the use of cryosurgery in treatment of basal cell carcinoma. Dermatol Surg 2003; 29:566-71; PMID:12786697; https://doi.org/10.1046/j.1524-4725.2003.29151b.x 10.1097/00042728-200306000-00002
- Tanaka S. Immunological aspects of cryosurgery in general surgery. Cryobiology 1982; 19:247-62; PMID:7105777; https://doi.org/10.1016/0011-2240(82)90151-1
- Uhlschmid G, Kolb E, Largiader F. Cryosurgery of pulmonary metastases. Cryobiology 1979; 16:171-8; PMID:477364; https://doi.org/10.1016/0011-2240(79)90028-2
- Sabel MS, Nehs MA, Su G, Lowler KP, Ferrara JL, Chang AE. Immunologic response to cryoablation of breast cancer. Breast Cancer Res Treat 2005; 90:97-104; PMID:15770533; https://doi.org/10.1007/s10549-004-3289-1
- Si T, Guo Z, Hao X. Immunologic response to primary cryoablation of high-risk prostate cancer. Cryobiology 2008; 57:66-71; PMID:18593573; https://doi.org/10.1016/j.cryobiol.2008.06.003
- Thakur A, Littrup P, Paul EN, Adam B, Heilbrun LK, Lum LG. Induction of specific cellular and humoral responses against renal cell carcinoma after combination therapy with cryoablation and granulocyte-macrophage colony stimulating factor: a pilot study. J Immunother 2011; 34:457-67; PMID:21577139; https://doi.org/10.1097/CJI.0b013e31821dcba5
- Scaviner D, Lefranc MP. The human T cell receptor alpha variable (TRAV) genes. Exp Clin Immunogenet 2000; 17:83-96; PMID:10810225; https://doi.org/10.1159/000019128 10.1159/000019129
- Folch G, Lefranc MP. The human T cell receptor beta variable (TRBV) genes. Exp Clin Immunogenet 2000; 17:42-54; PMID:10686482; https://doi.org/10.1159/000019130 10.1159/000019123
- Liu X, Venkataraman G, Lin J, Kiyotani K, Smith S, Montoya M, Nakamura Y, Kline J. Highly clonal regulatory T-cell population in follicular lymphoma—inverse correlation with the diversity of CD8 T cells. Oncoimmunology 2015; 4:e1002728; PMID:26155390; https://doi.org/10.1080/2162402X.2014.1002728
- Jang M, Yew PY, Hasegawa K, Ikeda Y, Fujiwara K, Fleming GF, Nakamura Y, Park JH. Characterization of T cell repertoire of blood, tumor, and ascites in ovarian cancer patients using next generation sequencing. Oncoimmunology 2015; 4:e1030561; PMID:26451311; https://doi.org/10.1080/2162402X.2015.1030561
- Park JH, Jang M, Tarhan YE, Katagiri T, Sasa M, Miyoshi Y, Kalari KR, Suman VJ, Weinshilboum R, Wang L et al. Clonal expansion of antitumor T cells in breast cancer correlates with response to neoadjuvant chemotherapy. Int J Oncol 2016; 49:471-8; PMID:27278091; https://doi.org/10.3892/ijo.2016.3540
- Tamura K, Hazama S, Yamaguchi R, Imoto S, Takenouchi H, Inoue Y, Kanekiyo S, Shindo Y, Miyano S, Nakamura Y et al. Characterization of the T cell repertoire by deep T cell receptor sequencing in tissues and blood from patients with advanced colorectal cancer. Oncol Lett 2016; 11:3643-9; PMID:27284367; https://doi.org/10.3892/ol.2016.4465
- Inoue H, Park JH, Kiyotani K, Zewde M, Miyashita A, Jinnin M, Kiniwa Y, Okuyama R, Tanaka R, Fujisawa Y et al. Intratumoral expression levels of PD-L1, GZMA, and HLA-A along with oligoclonal T cell expansion associate with response to nivolumab in metastatic melanoma. Oncoimmunology 2016; 5:e1204507; PMID:27757299; https://doi.org/10.1080/2162402X.2016.1204507
- Chapman CG, Yamaguchi R, Tamura K, Weidner J, Imoto S, Kwon J, Fang H, Yew PY, Marino SR, Miyano S et al. Characterization of T-cell receptor repertoire in inflamed tissues of patients with Crohn's disease through deep sequencing. Inflamm Bowel Dis 2016; 22:1275-85; PMID:27135481; https://doi.org/10.1097/MIB.0000000000000752
- Yew PY, Alachkar H, Yamaguchi R, Kiyotani K, Fang H, Yap KL, Liu HT, Wickrema A, Artz A, van Besien K et al. Quantitative characterization of T-cell repertoire in allogeneic hematopoietic stem cell transplant recipients. Bone Marrow Transpl 2015; 50:1227-34; PMID:26052909; https://doi.org/10.1038/bmt.2015.133
- Gazzaniga S, Bravo A, Goldszmid SR, Maschi F, Martinelli J, Mordoh J, Wainstok R. Inflammatory changes after cryosurgery-induced necrosis in human melanoma xenografted in nude mice. J Invest Dermatol 2001; 116:664-71; PMID:11348453; https://doi.org/10.1046/j.0022-202x.2001.01313.x
- Sabel MS, Arora A, Su G, Chang AE. Adoptive immunotherapy of breast cancer with lymph node cells primed by cryoablation of the primary tumor. Cryobiology 2006; 53:360-6; PMID:16973145; https://doi.org/10.1016/j.cryobiol.2006.07.004
- Gage AA, Baust JM, Baust JG. Experimental cryosurgery investigations in vivo. Cryobiology 2009; 59:229-43; PMID:19833119; https://doi.org/10.1016/j.cryobiol.2009.10.001
- Takeda H, Maruyama S, Okajima J, Aiba S, Komiya A. Development and estimation of a novel cryoprobe utilizing the Peltier effect for precise and safe cryosurgery. Cryobiology 2009; 59:275-84; PMID:19723517; https://doi.org/10.1016/j.cryobiol.2009.08.004
- Hoffmann NE, Bischof JC. The cryobiology of cryosurgical injury. Urology 2002; 60:40-9; PMID:12206847; https://doi.org/10.1016/S0090-4295(02)01683-7
- Erinjeri JP, Clark TW. Cryoablation: mechanism of action and devices. J Vasc Interv Radiol 2010; 21:S187-91; PMID:20656228; https://doi.org/10.1016/j.jvir.2009.12.403
- Krysko DV, Garg AD, Kaczmarek A, Krysko O, Agostinis P, Vandenabeele P. Immunogenic cell death and DAMPs in cancer therapy. Nat Rev Cancer 2012; 12:860-75; PMID:23151605; https://doi.org/10.1038/nrc3380
- Yatim N, Cullen S, Albert ML. Dying cells actively regulate adaptive immune responses. Nat Rev Immunol 2017; 17:262-75; PMID:28287107; https://doi.org/10.1038/nri.2017.9
- Khong HT, Restifo NP. Natural selection of tumor variants in the generation of "tumor escape" phenotypes. Nat Immunol 2002; 3:999-1005; PMID:12407407; https://doi.org/10.1038/ni1102-999
- Marincola FM, Jaffee EM, Hicklin DJ, Ferrone S. Escape of human solid tumors from T-cell recognition: molecular mechanisms and functional significance. Adv Immunol 2000; 74:181-273; PMID:10605607; https://doi.org/10.1016/S0065-2776(08)60911-6
- Anderson AC. Tim-3: an emerging target in the cancer immunotherapy landscape. Cancer Immunol Res 2014; 2:393-8; PMID:24795351; https://doi.org/10.1158/2326-6066.CIR-14-0039
- Dardalhon V, Anderson AC, Karman J, Apetoh L, Chandwaskar R, Lee DH, Cornejo M, Nishi N, Yamauchi A, Quintana FJ et al. Tim-3/galectin-9 pathway: regulation of Th1 immunity through promotion of CD11b+Ly-6G+ myeloid cells. J Immunol 2010; 185:1383-92; PMID:20574007; https://doi.org/10.4049/jimmunol.0903275
- Jones RB, Ndhlovu LC, Barbour JD, Sheth PM, Jha AR, Long BR, Wong JC, Satkunarajah M, Schweneker M, Chapman JM et al. Tim-3 expression defines a novel population of dysfunctional T cells with highly elevated frequencies in progressive HIV-1 infection. J Exp Med 2008; 205:2763-79; PMID:19001139; https://doi.org/10.1084/jem.20081398
- Qiu Y, Chen J, Liao H, Zhang Y, Wang H, Li S, Luo Y, Fang D, Li G, Zhou B et al. Tim-3-expressing CD4+ and CD8+ T cells in human tuberculosis (TB) exhibit polarized effector memory phenotypes and stronger anti-TB effector functions. PLoS Pathog 2012; 8:e1002984; PMID:23144609; https://doi.org/10.1371/journal.ppat.1002984
- Jin HT, Anderson AC, Tan WG, West EE, Ha SJ, Araki K, Freeman GJ, Kuchroo VK, Ahmed R. Cooperation of Tim-3 and PD-1 in CD8 T-cell exhaustion during chronic viral infection. Proc Natl Acad Sci U S A 2010; 107:14733-8; PMID:20679213; https://doi.org/10.1073/pnas.1009731107
- Cyktor JC, Carruthers B, Beamer GL, Turner J. Clonal expansions of CD8+ T cells with IL-10 secreting capacity occur during chronic Mycobacterium tuberculosis infection. PLoS One 2013; 8:e58612; PMID:23472214; https://doi.org/10.1371/journal.pone.0058612
- Fang H, Yamaguchi R, Liu X, Daigo Y, Yew PY, Tanikawa C, Matsuda K, Imoto S, Miyano S, Nakamura Y. Quantitative T cell repertoire analysis by deep cDNA sequencing of T cell receptor alpha and beta chains using next-generation sequencing (NGS). Oncoimmunology 2014; 3:e968467; PMID:25964866; https://doi.org/10.4161/21624011.2014.968467
- Choudhury NJ, Kiyotani K, Yap KL, Campanile A, Antic T, Yew PY, Steinberg G, Park J-H, Nakamura Y, O'Donnell PH. Low T-cell receptor diversity, high somatic mutation burden and high neoantigen load as predictors of clinical outcome in muscle-invasive bladder cancer. European Urology Focus 2016; 2:445-52; https://doi.org/10.1016/j.euf.2015.09.007
- Giudicelli V, Chaume D, Lefranc MP. IMGT/GENE-DB: a comprehensive database for human and mouse immunoglobulin and T cell receptor genes. Nucleic Acids Res 2005; 33:D256-61; PMID:15608191; https://doi.org/10.1093/nar/gki010
- Langmead B, Salzberg SL. Fast gapped-read alignment with Bowtie 2. Nat Methods 2012; 9:357-9; PMID:22388286; https://doi.org/10.1038/nmeth.1923
- Lefranc MP, Giudicelli V, Kaas Q, Duprat E, Jabado-Michaloud J, Scaviner D, Ginestoux C, Clement O, Chaume D, Lefranc G. IMGT, the international ImMunoGeneTics information system. Nucleic Acids Res 2005; 33:D593-7; PMID:15608269; https://doi.org/10.1093/nar/gki065
- Nelson WC, Pyo CW, Vogan D, Wang R, Pyon YS, Hennessey C, Smith A, Pereira S, Ishitani A, Geraghty DE. An integrated genotyping approach for HLA and other complex genetic systems. Hum Immunol 2015; 76:928-38; PMID:26027777; https://doi.org/10.1016/j.humimm.2015.05.001