ABSTRACT
IL-18 is an inflammasome-related cytokine, member of the IL-1 family, produced by a wide range of cells in response to signals by several pathogen- or damage-associated molecular patterns. It can be highly represented in tumor patients, but its relevance in human cancer development is not clear. In this study, we provide evidence that IL-18 is principally expressed in tumor cells and, in concert with other conventional Th1 cell-driven cytokines, has a pivotal role in establishing a pro-inflammatory milieu in the tumor microenvironment of human non-small cell lung cancer (NSCLC). Interestingly, the analysis of tumor-infiltrating CD8+ T cell populations showed that (i) the relative IL-18 receptor (IL-18R) is significantly more expressed by the minority of cells with a functional phenotype (T-bet+Eomes+), than by the majority of those with the dysfunctional phenotype T-bet−Eomes+ generally resident within tumors; (ii) as a consequence, the former are significantly more responsive than the latter to IL-18 stimulus in terms of IFNγ production ex vivo; (iii) PD-1 expression does not discriminate these two populations. These data indicate that IL-18R may represent a biomarker of the minority of functional tumor-infiltrating CD8+ T cells in adenocarcinoma NSCLC patients. In addition, our results lead to envisage the possible therapeutic usage of IL-18 in NSCLC, even in combination with other checkpoint inhibitor approaches.
Introduction
Lung cancer is the major cause of cancer-related death worldwide, and non-small cell lung cancer (NSCLC) accounts for 85% of lung cancer cases.Citation1 NSCLC is a heterogeneous tumor known to have diverse pathological features.Citation2 Most of the NSCLC patients fatally undergo metastatic disease or tumor recurrence, despite the availability of various chemotherapy or target therapy regimes developed in these last decades.Citation3 Therefore, novel treatment approaches urgently need to be investigated.
Antitumor immune responses exert clinically relevant pressure against tumor progression, but in the long run, they generally become unable to eradicate tumors due to various causes, such as local immunosuppressive effects, accumulation of a variety of suppressor cells, dysfunction/exhaustion of tumor-specific T cells.Citation4 A very promising immunotherapy approach for the treatment of tumors including adenocarcinoma (ADC) NSCLC is the use of monoclonal antibodies (mAbs) specific to various inhibitory signaling pathways (immune checkpoints) that block CD8+ T cell activation (e.g., programmed death [PD]-1 or cytotoxic T-lymphocyte antigen-4) that are strongly upregulated by tumor-infiltrating lymphocytes.Citation5 These mAbs (defined as checkpoint inhibitors) work by unleashing antitumor T cell responses, leading to a significant shrinkage of several metastatic tumors. However, checkpoint inhibitors currently used to target CD8+ T cells (e.g., IpilumumAb and NivolumAb) have not been shown to be efficient for all tumor types and may cause a partial remission in the majority of tumors. Innovative immunotherapy strategies are in progress particularly based on the combination of different approaches including new and current checkpoint inhibitors, even associated with personalized tumor vaccines against patient-specific neo-antigens that are generated as a consequence of the amazing diversity of tumor genome mutations.Citation6 In this context, novel therapeutic approaches targeting agents secreted by the tumor microenvironment may enlarge the combinatorial immunotherapy strategies required for enhancing antitumor immune responses. Among the huge repertoire of molecules contained within the tumor secretome, the cocktail of cytokines produced has a key role in tumor development, providing opposite effects (carcinogenesis versus protection) according to the balance between suppressive and stimulatory cytokines, the cross-talk of cytokines with immune, stromal or tumor cells or the synergistic effects by the combination of various cytokines.Citation7,8 Recent years have seen several cytokines, such as granulocyte-macrophage (GM)-CSF, IL-7, IL-12, IL-15, IL-18 and IL-21, or, alternatively, of antibodies (Abs) neutralizing suppressive cytokines (such as IL-10 and TGF-β) become possible targets for the treatment of patients with metastatic cancer.Citation9
Because cytokines potentially affecting immune response can be produced by various cellular players (tumor cells, immune cells, stromal cells, etc.) within the tumor microenvironment, here we detected a broad array of cytokines in the total supernatant from surgically excised fresh tumor tissues cultured for few hours (irrespective of the cellular source form which they derived), explored the interplay between the more abundantly secreted cytokines and the function of tumor-infiltrating T cells, and then visualized which cells in the tumor microenvironment were the key producers by immunohistochemical (IHC) analysis. By this multisystemic approach, we provided evidence that IL-18 is a predominant cytokine selectively produced by ADC NSCLC-derived tumor cells contributing to drive type 1 (IFNγ producing) responses of CD8+ T cells expressing high levels of IL-18 receptor (IL-18R) that are counterbalanced by dysfunctional T cells expressing low levels of IL-18R in ADC NSCLC. Our data pave the way for exploiting both IL-18R as a biomarker of functional type-1 CD8+ T cells infiltrating ADC NSCLC and IL-18 as a boosting cytokine for immune checkpoint antagonists in restoring T cell responses.
Materials and methods
Human samples and processing
Human studies were performed in accordance with the ethical guidelines of the 1975 Declaration of Helsinki and approved by the Institutional Ethical Committee (No. 2926). Informed consent was obtained from all patients.
We enrolled ADC (N = 43) and squamous (N = 8) NSCLC patients; however, only the size of ADC cohort achieved the statistical power and was included in the present work.
Peripheral blood (PB), non-tumor tissue (NTUM) obtained from lung tissue at least 10 cm from tumor site, and tumor (TUM) specimens were obtained from ADC NSCLC patients, not previously treated with adjuvant chemotherapy. Patient characteristics are listed in Table S1.
Peripheral blood mononuclear cells were isolated from the PB of patients by density gradient centrifugation with Lympholyte (Cedarlane) and collected in complete RPMI 1640 medium containing 10% FBS (HyClone GE Healthcare Life Sciences), 2 mM L-glutamine (Sigma-Aldrich), penicillin/streptomycin (EuroClone), nonessential amino acids (EuroClone) and sodium pyruvate (EuroClone) and 50 μM 2-mercaptoethanol (Sigma-Aldrich). Mononuclear cells (MN) were extracted from non-tumoral parenchyma (NTUM) or tumor (TUM) tissues. The fragments were minced and transferred in GentleMACS C tubes (Miltenyi Biotec) containing calcium- and magnesium-supplemented HBSS with 0.5 mg/mL Collagenase IV (Sigma), 50 ng/mL DNAse I (Worthington), 2% FBS and 10% BSA.
Tissue dissociation was made on a GentleMACS Octo Dissociator with Heaters (Miltenyi Biotec), applying a modified version of the program “h_tumor 01_03” at 37 °C and lasting totally 30’. Single cell suspensions were obtained by disrupting the remaining small fragments through a cell strainer with a syringe plunger, washing with cold HBSS. Then cells were pelleted and finally centrifuged over a Lympholyte density gradient.
Flow-cytometry analysis
The list of antibodies is available on Table S2.
Flow cytometry (FC) analysis was performed on ADC NSCLC patients in PB, NTUM and TUM specimens.
Dead cells in all samples were excluded using Fixable Viability Dye eFluor®780 (eBioscience). Surface staining was performed by incubating the cells with selected Abs at 4 °C for 20 min in PBS 2% FBS.
Intracellular staining of cytokines and transcription factors was performed by FOXP3/Transcription factor staining buffer set (eBioscience). Surface CD218β staining was performed at room temperature for 30 min.
To perform IFNγ intracellular staining cells were stimulated for 4 h at 37 °C with Cell Stimulation Cocktail plus protein transport inhibitors (eBioscience).
Samples were acquired on the BD LSRFortessa cell analyzer (BD Biosciences) and analyzed with FlowJo software, version 10.0.8r1 (Treestar).
Cytokine analysis
Cytokine dosage was performed by ProcartaPlex Human and Cytokine & Chemokine Panel 1A (EXP340-12167-901, eBioscience) using Bioplex 200 (Biorad). The amount of cytokines was detected in Plasma (N = 7) of HD, Plasma (N = 15) of NSCLC patients and in tissue-conditioned medium generated by incubating, at 37 °C for 6 h, NTUM (N = 5) and TUM (N = 10) fragments in 1 mL of complete medium per mg of tissue.
IHC analysis
Immunohistochemistry was performed on formalin fixed paraffin-embedded tissue samples (N = 3) using an anti-IL18 mAb (ab191152-Abcam) and the streptavidin–biotin–peroxidase complex method. IL-18 expression was assessed on formalin fixed paraffin-embedded sections of three NSCLC specimens. IL-18 immunoreactions were revealed by Bond Polymer Refine Detection (Leica Biosystem, Milan, Italy) on an automated autostainer (BondTM Max, Leica). The evaluation of IL-18 in cancer tissues was performed independently by two investigators blinded to patient data.
Ex vivo assays
MN enriched from PB, NTUM and TUM were seeded ON at 37 °C in U-bottomed 96 well plate with Dynabeads Human T-Activator CD3/CD28 (Invitrogen) at a ratio 1:4 with or without human recombinant IL-18 (10 ng, MBL), plus IL-2 (100U/mL, Roche). For IFNγ production MN were stimulated and labeled, as described above. FC analysis was performed in gated CD8+ T cells. Ex vivo analysis was performed in five independent experiments, on five different patients giving similar results.
Statistical analysis
Statistical analysis was performed using Prism software (version 6.0c, GraphPad).
To analyze in vitro data were used two-way-ANOVA test (Tukey's multiple comparison test), Mann–Whitney test, 2-tailed, Wilcoxon matched-pairs test, 2-tailed or paired matched t-test, 2-tailed were applied to compare groups of ex vivo analysis. Mann–Whitney test, 2-tailed was applied to analyze cytokine amounts. Pearson test was considered for correlation of amount of cytokines. p < 0.05 was considered statistically significant, in all tests.
Results and discussion
First, we detected by FC the percentage of naive (N; CCR7+CD45RA+), central memory (CM; CCR7+CD45RA−), effector memory (EM; CCR7−CD45RA−), terminal effector memory RA+ (EMRA; CCR7−CD45RA+) cells within CD8+ T cells, in PB, NTUM and TUM districts of NSCLC patients. As expected, the CD8+ N cell subset was minimally represented in the tissue districts, whereas the EM cell subset was preferentially accumulated in those districts and significantly in TUM district ().Citation10 On the contrary, a significant reduction of CD8+ TEMRA cells was shown in TUM district (), supporting that the tumor microenvironment may disturb the full differentiation of CD8+ T cells with terminal effector functions. Deepened FC analyses reinforced this hypothesis, by analyzing the expression of nuclear transcription factors T-box (T-bet) and Eomesodermin (Eomes), allowing to discriminate CD8+ T cells into functional T-bet+Eomes+ and dysfunctional T-bet−Eomes+ cells, respectively, on the basis of previous experimental data showing that T-bet expression in memory CD8+ T cells is associated with the control of chronic infections or tumors through the downregulation of the inhibitory PD-1 receptor, and that Eomes expression characterizes dysfunctional memory CD8+ T cells when it is not co-expressed with T-bet.Citation11 Our data showed that the TUM district contained a significantly lower percentage of cells expressing a functional T-bet+Eomes+ phenotype than the other districts, and as a consequence, a more abundant percentage of the dysfunctional T-bet−Eomes+ counterpart (). This finding was confirmed at the level of the EM and EMRA cell populations (Fig. S1A and B). In addition, CD8+ T cells from TUM tissues contained a significantly higher percentage of cells expressing the exhaustion marker PD-1 than those from the other districts (; Fig. S1C). The accumulation of PD-1+ cells was significantly more evident also at the level of both the EM and EMRA subpopulations (Fig. S1D), as well as within T-bet+Eomes+ and T-bet−Eomes+ subsets from TUM, compared with peripheral counterparts (). Notably, PD-1 was significantly more expressed or tended to be more expressed by T-bet−Eomes+ than T-bet+Eomes+ subset from NTUM and TUM, respectively (). This finding is consistent with evidence showing that T-bet−Eomes+PD-1+ CD8+ T cells represent frankly exhausted cells, whereas the T-bet+Eomes+PD-1+ subset can be rescued by blockade of the PD-1 pathway.Citation11 These data suggest that two divergent T cell populations with functional and dysfunctional/exhaustion phenotype can simultaneously be harbored in the TUM district of NSCLC patients and that the latter are significantly more represented than the former.
Figure 1. Dysfunctional CD8+ T cells were accumulated in TUM district of NSCLC patients. (A) Representative CCR7/CD45RA staining of FC (upper graph) and percentage of CM, N, EM and EMRA (bottom graphs) in gated CD8+ T cells in PB, NTUM and TUM specimens of NSCLC patients (N = 25); *p <0.05, **p < 0.01, ***p < 0.005 Wilcoxon matched pairs test, 2-tailed. (B) Representative T-bet/Eomes staining by FC (upper graph) and percentage of T-bet+Eomes−, T-bet+Eomes+, T-bet−Eomes+ (bottom graphs) in gated CD8+ T cells in PB, NTUM and TUM (N = 16); *p < 0.05, **p < 0.01 Wilcoxon matched pairs test, 2-tailed. (C) Percentage of PD-1+ in gated CD8+ T cells in PB, NTUM and TUM (N = 22); (The representative FC is shown in Fig. S1C); **p < 0.01 Wilcoxon matched pairs test, 2-tailed. (D) Graph of PD-1 MFI in gated T-bet+Eomes−, T-bet+Eomes+ and T-bet−Eomes+ CD8+ T cell subpopulations in PB, NTUM and TUM (N = 16); *p < 0.05 paired matched t-test 2-tailed in PB, NTUM and TUM within T-bet+Eomes+ and T-bet+Eomes− subsets and Wilcoxon matched pairs test, 2-tailed between T-bet+Eomes−, T-bet+Eomes+ and T-bet−Eomes+ subsets.
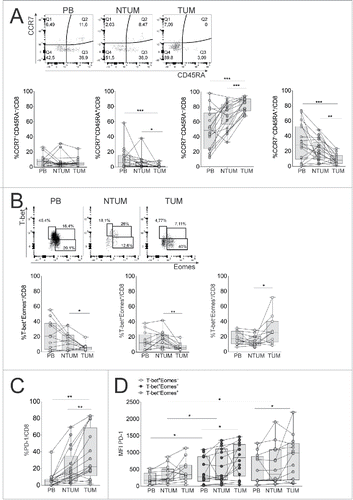
To explore which type of signals might contribute to condition the divergent functions of tumor-infiltrating CD8+ T cell populations, we detected a wide array of cytokines and chemokines in tissue-conditioned media derived from TUM and NTUM tissues. We could observe a set of different pro-inflammatory cytokines and chemokines that were particularly enriched in these tissues, such as IL-18, MCP-1, IL-6, SDF1-a IL-8, IL1Ra, IP-10 (). Remarkably, IL-18 was the prominent cytokine that was especially enriched in TUM compared with NTUM, and was followed by a concomitant enrichment of IFNγ (). IL-18, a member of the IL-1 family, is a key pro-inflammatory cytokine of the inflammasome platform produced by macrophages and a wide range of innate immune or non-lymphoid cells.Citation12 It is strongly involved in the polarization of functional T cell pathways, according to the different milieu in which it operates. Indeed, when associated with IL-12, it contributes to induce Th1-like cell responsesCitation13 that potentially play a pivotal role in tumor immune-surveillance. Though, in the absence of IL-12, it plays a role in Th2 cell-mediated diseases,Citation14 as well as it can induce Th17 cell responses in combination with IL-23.Citation15 In the context of tumor microenvironments, IL-18 has been proposed to have opposing effects: it may promote tumor progression, particularly in the absence of Th1-like cytokines, Citation8,16,17 or it may promote adaptive immunity with antitumor effect when associated with other cytokines.Citation13,18 In line with these observations, we could observe a positive correlation between the amount of IL-18 and conventional Th1-associated cytokines (i.e., IFNγ, TNF-α and IL-12), especially in TUM district (), supporting the idea that IL-18 can act as a driver of antitumor Th1-like responses in the NSCLC milieu. Interestingly, the preponderant source of IL-18 was represented by tumor cells, as detected by IHC analysis in tumor sections (). Importantly, the high expression of IL-18 observed by IHC corresponded to IL-18 amounts detected in conditioned media by the ProcartaPlex assay-based evaluation. This result is reminiscent of previous reports, showing that IL-18 expression in prostate cancers was associated with a favorable outcome, suggesting a role of IL-18 in the control of tumor spreading, in line with antitumor properties of IL-18 observed in colon cancer. Citation19,20
Figure 2. IL-18 was particularly represented in TUM of NSCLC patients. (A) Cytokines/chemokines amount (evaluated as pg/mL of mean values) is detected in Plasma of HD (N = 7), Plasma of NSCLC patients (N = 15) and in tissue conditioned media derived from NTUM (N = 5) and TUM (N = 10) of NSCLC patients. (B) Representative graph showing the amount of IL-18 and IFNγ evaluated as pg/mL; *p < 0.05, **p < 0.01, ***p <0.005, ****p < 0.0001 Mann–Withney test, 2-tailed. (C) Person correlation between IL-18 versus IFNγ and IL-12 amount in term of pg/mL. (D) IHC analysis (100× magnification) of IL-18 in NSCLC representative (IL-18low/high) patients (N = 2). The arrows indicated IL-18+ tumor cells.
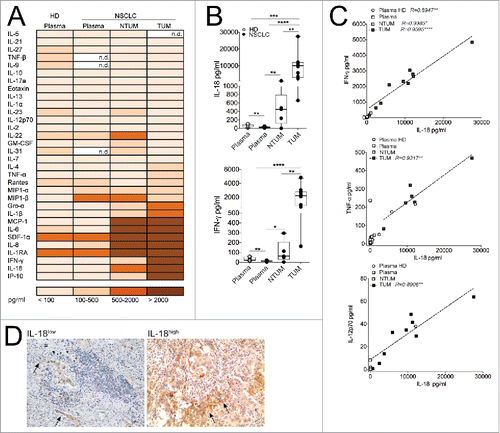
However, to elucidate the possible role of IL-18 on CD8+ T cells, in NCSLC, we questioned whether different levels of surface IL-18R expression would contribute to a different bioactivity of IL-18.Citation13,21 IL-18 binds the IL-18Rα (CD218α) chain requiring the expression of the co-receptor IL-18Rβ (CD218β) to form a high affinity heterodimer complex providing productive signals into cells, ultimately causing NF-κB activation and gene transcription of pro-inflammatory cytokines.Citation13,21 Il18r1 is a direct target gene of STAT-4 suggesting that both IL-12-dependent STAT-4 and IL-18-dependent NF-κB signaling may induce synergistic transcription of IFNγ.Citation22,23
We demonstrated the presence of a peculiar CD8+ T cell subset expressing IL18R in both TUM and NTUM (). In addition, only a minority of cells within the entire CD8+ T cell population co-expressed both the CD218α and CD218β chains (, right panel), whereas the majority of them expressed either CD218α or CD218β, of which only the former was significantly more expressed in TUM-derived than in NTUM-derived CD8+ T cells (, left/middle panels). However, single CD218α+, single CD218β+ cells or those co-expressing both the chains, were significantly more represented within T-bet+Eomes+ than T-bet−Eomes+ CD8+ T cells (, left/ middle/right panels). Taken together, these data support that the full IL-18R expression required for delivering the signals ultimately causing IFNγ production is associated with the minority of CD8+ T cells with a functional phenotype (T-bet+Eomes+).
Figure 3. CD8+ T cells expressed high levels of CD218α and CD218β receptor in TUM. (A) Representative CD218α and β staining by FC (upper graphs) and percentage of CD218α+ CD218β+ and CD218α/β+ (bottom graphs) gated in CD8+ T cells in HD (N = 6), PB, NTUM and TUM (N = 14); *p < 0.05, **p < 0.01, ****p < 0.0001 paired matched t-test 2-tailed between different districts (PB, NTUM and TUM) of the same patient and *p < 0.05 Student t-test, unpaired between HD and patients (CD218α+ graph); *p < 0.05 Paired matched t-test 2-tailed between different districts (PB, NTUM and TUM) of the same patient and *p < 0.05, **p < 0.01 student t-test, unpaired between HD and patients (CD218β+ graph). (B) Percentage of CD218α+, CD218β+ and CD218α/β+ in gated T-bet+Eomes−, T-bet+Eomes+, T-bet+Eomes+ in pooled cohorts (PB, NTUM and TUM) (N = 14); **p < 0.01 Paired matched t-test 2-tailed (CD218α+ graph); **p < 0.01 Paired matched t-test 2-tailed (CD218β+ graph); *p < 0.05 paired matched t-test 2-tailed (CD218α/β+ graph). (C) Representative IFNγ/CD45RA staining by FC, in both CD218α+ and CD218α− (left graph) and percentage of IFNγ+ cells in gated CD218α+ and CD218α− CD8+ T cells (right graph) in PB, NTUM and TUM specimens (N = 12); *p < 0.05 paired matched t-test 2-tailed.
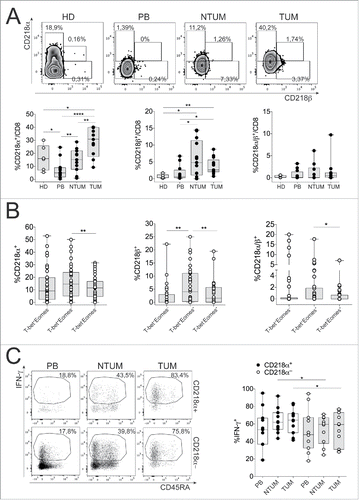
Accordingly, CD218α+ cells produced significantly higher levels of IFNγ than CD218α− ones ex vivo (). These results confirmed that TUM tissue can simultaneously contain functional and dysfunctional CD8+ T cell populations, whose ratio is frankly unbalanced toward the latter, and that the level of CD218α expression can help to discriminate them. These data are consistent with a previous report showing that exhausted CD8+ T cells are associated with the downregulation of CD218α expression in a chronic infected environment.Citation24 Importantly, the evidence that IL-18 alone further improved IFNγ-production by CD218α+ cells (T-bet+Eomes+) within different T cell populations isolated from the various NSCLC districts ex vivo ( and ), supports that it may provide a favorable effect in NSCLC through the expansion of type-1 CD8+ T cell responses with potential antitumor competence within the minor CD8+ T cell population with functional phenotype present in NSCLC tissues. Contextually, our preliminary evidences showed that IFNγ production by TUM-derived CD8+ T cells tended to increase upon the simultaneous contact with IL-18 and IL-12, irrespective of the presence of TCR stimuli, supporting that IL-18 can synergize with other cytokines to elicit favorable responses by tumor-infiltrating CD8+ T cells irrespective of their ability to receive TCR-dependent signals (data not shown).
Figure 4. IL-18 increased IFNγ production, especially in CD218α+ and T-bet+Eomes+ CD8+ T cells. MCs enriched from PB, NTUM and TUM, were seeded overnight without (NS = not stimulated) or with IL-18 (10 ng/mL), plus Beads (anti-CD3 and anti-CD28) and IL-2 (100U/mL). (A) The graphs show MFI of IFNγ in gated CD8+ T cells and in gated CD218α+ and CD218α− CD8+ T cells; *p < 0.05, **p < 0.01, ***p < 0.005, ****p < 0.0001 two-way-Anova (Tukey's multiple comparison test). (B) The graphs show MFI of IFNγ respectively in gated T-bet+Eomes−, Tbet+Eomes+ and T-bet−Eomes+; *p < 0.05, **p < 0.01, ***p < 0.005, ****p < 0.0001 two-way-Anova (Tukey's multiple comparison test). The graphs show a representative patient (N = 3) out of five showing similar results (Fig. S2). Test significance is shown for difference between stimulated (IL-18) and un-stimulated (NS) samples (black symbols) and between PB, NTUM and TUM districts (gray symbols).
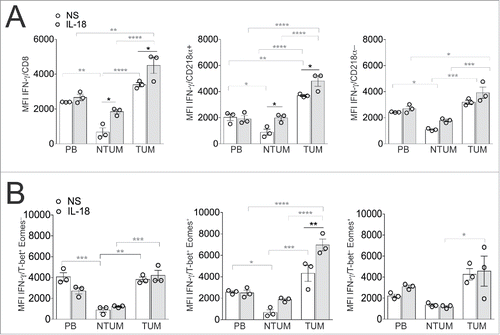
In conclusion, the high concentration of IL-18 in NSCLC TUM district, mostly produced by tumor cells could contribute (in association with classical Th1-associated cytokines – i.e., IFNγ, TNF-α and IL-12 – shown in this tumor milieu) to the expansion of IL18R+ CD8+ T cells expressing T-bet and IFNγ within the tumor microenvironment. Strikingly, these data suggest that tumors are not completely immunosuppressive, but they can simultaneously deliver signals either favoring or inhibiting the immune responses. The selection of this balance, would contribute to establish a state of chronic low-level inflammation allowing both a long-lasting survival of the host and a slow tumor progression that ultimately becomes invariably and rapidly fatal, in relation with the progressive failure of the mechanisms maintaining tumor dormancy.Citation4 Importantly, our data provide intriguing insights into the possible therapeutic usage of IL-18 addressed to foster CD8+ T cell responses in the tumor milieu, even in combination with immune checkpoint inhibitors to counterbalance dysfunctional/exhausted CD8+ T cells. The evidence provided by clinical trials in advanced cancer showing a limited effectiveness of IL-18 monotherapy favors its possible usage in combination with other immunostimulatory approaches.Citation25,26 Clinical long-term follow-up studies are in progress to determine if IL-18R+ or IL-18R− T cells are associated with favorable or unfavorable outcome, respectively, in NSCLC.
Disclosure of potential conflicts of interest
No potential conflicts of interest were disclosed.
Supplementary_materials.doc
Download MS Word (3.2 MB)Acknowledgments
We thank all patients and healthy donors who participated in this study. We thank Cristiano Alessandri, Tania Colasanti (Dipatimento di Medicina Interna e Specialità Mediche, “Sapienza” Università di Roma) and Carlo Colombo (Prodotti Gianni, Rome) for technical support. CF was supported by a 2015 Fellowship from Fondazione Veronesi.
Funding
This work was supported by the following grants: Associazione Italiana per la Ricerca sul Cancro (AIRC) (progetto “Investigator Grant” [IG]-2014 id. 15199); Ministero della Salute (Ricerca finalizzata [RF-2010–2310438 and RF 2010–2318269]); Fondazione Italiana Sclerosi Multipla (FISM) onlus (cod. 2015/R/04); Ministero dell'Istruzione, dell'Università e della Ricerca (MIUR) (PRIN 2010–2011 prot. 2010LC747T_004); Fondo per gli investimenti di ricerca di base (FIRB)-2011/13 (no. RBAP10TPXK); Istituto Pasteur Italia – Fondazione Cenci Bolognetti (grant 2014–2016); International Network Institut Pasteur, Paris – “Programmes Transversaux De Recherche” (PTR n. 20–16).
References
- Siegel R, Ma J, Zou Z, Jemal A. Cancer statistics. CA Cancer J Clin 2014; 64:9-29; PMID:24399786; https://doi.org/10.3322/caac.21208
- Chen Z, Fillmore CM, Hammerman PS, Kim CF, Wong KK. Non-small-cell lung cancers: a heterogeneous set of diseases. Nat Rev Cancer 2014; 14:535-46; PMID:25056707; https://doi.org/10.1038/nrc3775
- Rosell R, Bivona TG, Karachaliou N. Genetics and biomarkers in personalisation of lung cancer treatment. Lancet 2013; 382:720-31; PMID:23972815; https://doi.org/10.1016/S0140-6736(13)61715-8
- Schreiber RD, Old LJ, Smyth MJ. Cancer immunoediting: integrating immunity's roles in cancer suppression and promotion. Science 2011; 331:1565-70; PMID:21436444; https://doi.org/10.1126/science.1203486
- Sharma P, Allison JP. The future of immune checkpoint therapy. Science 2015; 348:56-61; PMID:25838373; https://doi.org/10.1126/science.aaa8172
- Schumacher TN, Schreiber RD. Neoantigens in cancer immunotherapy. Science 2015; 348:69-74; PMID:25838375; https://doi.org/10.1126/science.aaa4971
- Dranoff G. Cytokines in cancer pathogenesis and cancer therapy. Nat Rev Cancer 2004; 4:11-22; PMID:14708024; https://doi.org/10.1038/nrc1252
- Mantovani A, Allavena P, Sica A, Balkwill F. Cancer-related inflammation. Nature 2008; 454:436-44; PMID:18650914; https://doi.org/10.1038/nature07205
- Lee S, Margolin K. Cytokines in cancer immunotherapy. Cancers (Basel) 2011; 3:3856-93; PMID:24213115; https://doi.org/10.3390/cancers3043856
- Sallusto F, Lenig D, Forster R, Lipp M, Lanzavecchia A. Pillars article: two subsets of memory T lymphocytes with distinct homing potentials and effector functions. Nature. 1999; 401:708-712. J Immunol 2014; 192:840-4; PMID:10537110; https://doi.org/10.1038/44385
- Wherry EJ, Kurachi M. Molecular and cellular insights into T cell exhaustion. Nat Rev Immunol 2015; 15:486-99; PMID:26205583; https://doi.org/10.1038/nri3862
- Dinarello CA. Overview of the interleukin-1 family of ligands and receptors. Semin Immunol 2013; 25:389-93; PMID:24275600; https://doi.org/10.1016/j.smim.2013.10.001
- Novick D, Kim S, Kaplanski G, Dinarello CA. Interleukin-18, more than a Th1 cytokine. Semin Immunol 2013; 25:439-48; PMID:24275602; https://doi.org/10.1016/j.smim.2013.10.014
- Nakanishi K, Yoshimoto T, Tsutsui H, Okamura H. Interleukin-18 is a unique cytokine that stimulates both Th1 and Th2 responses depending on its cytokine milieu. Cytokine Growth Factor Rev 2001; 12:53-72; PMID:11312119; https://doi.org/10.1016/S1359-6101(00)00015-0
- Lalor SJ, Dungan LS, Sutton CE, Basdeo SA, Fletcher JM, Mills KH. Caspase-1-processed cytokines IL-1beta and IL-18 promote IL-17 production by gammadelta and CD4 T cells that mediate autoimmunity. J Immunol 2011; 186:5738-48; PMID:21471445; https://doi.org/10.4049/jimmunol.1003597
- Garlanda C, Dinarello CA, Mantovani A. The interleukin-1 family: back to the future. Immunity 2013; 39:1003-18; PMID:24332029; https://doi.org/10.1016/j.immuni.2013.11.010
- Terme M, Ullrich E, Aymeric L, Meinhardt K, Desbois M, Delahaye N, Viaud S, Ryffel B, Yagita H, Kaplanski G et al. IL-18 induces PD-1-dependent immunosuppression in cancer. Cancer Res 2011; 71:5393-9; PMID:21724589; https://doi.org/10.1158/0008-5472.CAN-11-0993
- Fabbi M, Carbotti G, Ferrini S. Context-dependent role of IL-18 in cancer biology and counter-regulation by IL-18BP. J Leukoc Biol 2015; 97:665-75; PMID:25548255; https://doi.org/10.1189/jlb.5RU0714-360RR
- Lebel-Binay S, Thiounn N, De Pinieux G, Vieillefond A, Debre B, Bonnefoy JY, Fridman WH, Pagès F. IL-18 is produced by prostate cancer cells and secreted in response to interferons. Int J Cancer 2003; 106:827-35; PMID:12918059; https://doi.org/10.1002/ijc.11285
- Pages F, Berger A, Henglein B, Piqueras B, Danel C, Zinzindohoue F, Thiounn N, Cugnenc PH, Fridman WH. Modulation of interleukin-18 expression in human colon carcinoma: consequences for tumor immune surveillance. Int J Cancer 1999; 84:326-30; PMID:10371355; https://doi.org/10.1002/(SICI)1097-0215(19990621)84:3%3c326::AID-IJC22%3e3.0.CO;2-K 10.1002/(SICI)1097-0215(19990621)84:3%3c326::AID-IJC22%3e3.3.CO;2-B
- Dinarello CA, Novick D, Kim S, Kaplanski G. Interleukin-18 and IL-18 binding protein. Front Immunol 2013; 4:289; PMID:24115947; https://doi.org/10.3389/fimmu.2013.00289
- Yang J, Zhu H, Murphy TL, Ouyang W, Murphy KM. IL-18-stimulated GADD45 beta required in cytokine-induced, but not TCR-induced, IFN-gamma production. Nat Immunol 2001; 2:157-64; PMID:11175814; https://doi.org/10.1038/89769 10.1038/84264 10.1038/35052105
- O'Shea JJ, Lahesmaa R, Vahedi G, Laurence A, Kanno Y. Genomic views of STAT function in CD4+ T helper cell differentiation. Nat Rev Immunol 2011; 11:239-50; PMID:21436836; https://doi.org/10.1038/nri2958
- Ingram JT, Yi JS, Zajac AJ. Exhausted CD8 T cells downregulate the IL-18 receptor and become unresponsive to inflammatory cytokines and bacterial co-infections. PLoS Pathog 2011; 7:e1002273; PMID:21980291; https://doi.org/10.1371/journal.ppat.1002273
- Srivastava S, Salim N, Robertson MJ. Interleukin-18: biology and role in the immunotherapy of cancer. Curr Med Chem 2010; 17:3353-7; PMID:20712569; https://doi.org/10.2174/092986710793176348
- Tian H, Shi G, Yang G, Zhang J, Li Y, Du T, Wang J, Xu F, Cheng L, Zhang X et al. Cellular immunotherapy using irradiated lung cancer cell vaccine co-expressing GM-CSF and IL-18 can induce significant antitumor effects. BMC Cancer 2014; 14:48; PMID:24475975; https://doi.org/10.1186/1471-2407-14-48