ABSTRACT
The administration of ex vivo-expanded Natural Killer (NK) cells in leukemia therapy is still challenging, in part due to the difficulty to generate in sufficient quantities fully mature and functional NK cells and Identification of surface markers indicative of NK maturation and functionality is therefore needed. Here, based on the analysis of surface receptors of ex vivo-expanded NK cells, we identified CD94 as a surface marker correlating with high lytic potential against leukemic cell lines and immunological synapse formation. CD94-positive ex vivo-expanded NK cells displayed higher expression of NKG2 receptors and the adhesion molecule LFA-1, as compared with their CD94-negative counterparts. We also tested the in vivo anti-leukemic capacity of ex vivo-expanded NK cells against patient-derived acute myeloid leukemia cells. Although no anti-leukemic effect was detected, we noticed that only CD94-positive ex vivo-expanded NK cells were detected in leukemic mice at the end of the 2-week treatment. Moreover, flow cytometry analysis showed a subpopulation harboring CD94 (NK) and CD34 (leukemic cells) double staining, indicative of conjugate formation. Therefore surface expression of CD94 on ex vivo-differentiated NK cells emerged as an indicator of in vitro and in vivo killer cell functionality.
Introduction
The first breakthrough in immunotherapy for Acute Myeloid Leukemia (AML) treatment lies in Hematopoietic Stem Cell Transplantation (HSCT).Citation1,2 In fact, the most important curative effect of HSCT relies on the graft-versus-leukemia (GvL) effect, which is an immune response of the donor's lymphocytes against the residual disease in the recipient.Citation3-5 Therefore, one strategy for enhancing the GvL effect is to give donor T lymphocyte infusions after allogenic HSCT.Citation6,7 However, the beneficial effect of this adoptive transfer of lymphocytes is limited by graft-vs.-host disease (GVHD)Citation8: the allogeneic T lymphocytes will attack not only residual leukemic cells, but also host cells resulting in severe organ damages.Citation9
Different from T cells, NK cells are CD3−CD56+ lymphocytes that belong to the innate immune system and do not require pre-stimulation to exert their effect or functions.Citation10,11 Most importantly, they can exert a GvL effectCitation12-14 without attacking host cellsCitation15,16 which makes them the ideal candidate for adoptive immunotherapyCitation17,18 in the treatment of acute leukemiaCitation19 especially in the post-transplant setting.Citation20
NK cells from healthy donors are not easily available, therefore methods for generating allogeneic, cancer-reactive NK cells are being developed.Citation21 Ex vivo expansion of NK cells from haematopoietic stem cells (HSC) is one promising approach,Citation22-25 with umbilical cord blood (UCB) being used as readily available source of CD34+haematopoietic progenitor cells (HPC).Citation24,26-32 However, despite these ex vivo-generated NK cells (evNK) display toxicity against some well established leukemic cell lines such as K562 cells, their lytic potential may not be as efficient as that of NK from healthy donors and fully mature state based on expression of NK receptors and markers is not reached. Using a described previously cytokine-based culture method in the presence of HOXB4-transduced stromal MS-5 cells,Citation33,34 we show that the lytic potential of ex vivo differentiated NK cells (evNK) is associated with the surface expression of CD94 in vitro. We further characterized these cells by investigating their NK receptor panel and their in vivo toxicity, biodistribution, and anti-leukemic potential after adoptive transfer in immunodeficient mice engrafted with primary acute myeloid leukemia (AML) cells (patient-derived xenograft model). We demonstrated that in vitro CD94 expression on evNK is associated with higher lytic potential, higher expression of NKG2 receptors and LFA-1 molcule, and higher ability to form immunological synapses with leukemic target cells, and that only CD94+ NK cells form in vivo conjugates with leukemic cells.
Material and methods
In vitro generation of human NK cells from UCB-derived CD34+ -HPC
Umbilical cord blood samples were collected from healthy new-borns with mothers' consent in accordance with the institutional review boards of the Etablissement Français du Sang, Créteil France, and the Institut National de la Santé et de la Recherche Médicale, Paris, France. Mononuclear cells were isolated from UCB by a Ficoll method. CD34+ cells were further isolated using a dextran/ficoll based procedure followed by immuno-magnetic separation (MACS, Miltenyi Biotec)(purity ≥ 80%) and transferred into 12-well plates (25.103cells/well) in a co-culture system using feeder murine MS-5 cells engineered to actively secrete the human HOXB4 protein, as described previously.Citation33,34 CD34+ cells were cultured in a humidified atmosphere containing 5% CO2 at 37°C for 4 weeks in RPMI-1640 media containing 10% pooled human serum (Jacques Boy), 5% horse serum (Stem Cell Technology), 1% Penicilline-Streptomycin (Invitrogen) and the following cytokines: human recombinant Stem Cell Factor (SCF, 50 ng/mL), interleukin-2 (IL-2, 200 UI/mL), IL-7 (20 ng/mL), IL-15 (20 ng/mL), and FLT-3 (50 ng/mL) (all from Milteny Biotec). Cells were splitted with new media and cytokinestwice a week. After 4 weeks, CD56+-evNK cells were isolated using the MACS system (Miltenyi Biotec) (purity ≥ 90%). CD56+-evNK cells were subsequently cultured in RPMI-1640 media added with 10% pooled human serum (Jacques Boy), 5% Horse Serum (Stem Cell Technology), 1% Penicilline-Streptomycin (Invitrogen), and IL-2 (200 UI/ml, Miltenyi).
Isolation of NK cells from healthy donor-peripheral blood
NK from healthy donors (NKhd) were obtained from fresh apheresis products after Ficoll and CD56 purification using the MACS system (Miltenyi Biotec) (purity ≥90%). CD56+-NKhdwere subsequently cultured in RPMI-1640 media added with 10% pooled human serum (Jacques Boy), 5% Horse Serum (Stem Cell Technology), 1% Penicilline-Streptomycin, and IL-2 (200 UI/ml, Miltnyi Biotec).
Culture of leukemic and stromal MS-5 cell lines
K562, U937, and HL-60cells were grown in RPMI-1640 medium (Invitrogen) supplemented with 10% fetal bovine serum (Invitrogen) and 1% penicillin-streptomycin. Mouse stromal cells MS5-HOXB4 cells were cultured in MEM medium (Invitrogen) supplemented with 10% fetal bovine serum and 1% Penicillin-Streptomycin. All cells were grown in a humidified atmosphere containing 5% CO2 at 37°C.
Chromium (Cr51) release assay
The cytotoxic activity of the ex-vivo differentiated NK cells (evNK) was measured by a conventional 4-hour 51Cr-release assay in round-bottom 96-well plates. The K562, U937, HL-60 cell linesand patient-derived acute myeloid leukemia cells were used as targets (103 cells/well). Experiments were performed in triplicate. Data were expressed as the percentage of 51Cr release from target cells, calculated as (experimental release − spontaneous release)/(maximum release − spontaneous release) × 100.
Flow cytometry analysis
Flow cytometry analysis for evNK phenotyping was performed using a C6 cytometer and data were processed using FlowJo software. CD94-based cell sorting was performed on an ARIA cytometer. Monoclonal anti-human antibodies recognizing the following surface markers were from Biolegend: anti-CD337-PE (NKp30), -CD336-PE (NKp44), -CD335-PE (NK p46), -CD16-PE, -CD161-PE, -CD226-PE (DNAM1). The following anti-human antibodies were from Miltenyi Biotec: anti-CD56-APC, -CD7-PE, -CD45RA-PE, -CD94-FITC, -CD117-PE, -CD158A-PE (KIR2DL1), -CD158B-PE (KIR2DL2/DL3), -CD158E-PE (KIR3DL1), -CD158I-PE (KIR2DS4), -NKG2A-PE, -NKG2D-PE.
Analysis of immune synapse formation
K562, U937, and HL-60leukemia cells were spread on poly-L-lysine-coated coverslips for 2 hours at 37°C. CD94-positive and –negative evNK cells were then added at 2:1 effector-to-target ratio. After a co-culture of 30 min, cells were fixed (4% PFA, 30 min), permeabilized (0.1% Triton, 20 min), blocked with 10% FBS for 20 min, and stained with rhodamine-phalloidine (1/500, Molecular Probes). Coverslips were washed 3 times in PBS and mounted in Vectashield mounting medium containing DAPI (Vector Laboratories) before imaging (IX83 microscope; Olympus) and analysis (CellSense Dimension software; Olympus). Percentage of NK forming immune synapses with target leukemic cells was calculated as (number of NK involved in immune synapses)/(total NK number) × 100.
UCB derived-NK cell adoptive transfer in leukemic NSG-mice
Immunodeficient NOD/SCID IL2-Rγ−/− (NSG) mice (6–8 weeks old) were bred and housed under specific pathogen-free conditions at the animal facility of Gustave Roussy Cancer Center. All animal experiments were conducted in accordance with institutional and national guidelines under the permit number E-94–076–11.
For leukemic engraftment of NSG mice, CD3-depleted mononuclear cells of a freshly diagnosed AML patientCitation35 (source: peripheral blood; ) were used after informed consent and approval by local Research Ethics Committees of Saint-Antoine hospital (Paris, France). 2.106AML cells were intravenously injected (retro-orbital venous sinus) to mice 24 h after irradiation with an X-Ray source (dose: 2.5 Gy). After 31 days, mononuclear cells collected from AML engrafted-mice blood were counted and quadruple-stained with anti-mouse CD45, anti-human CD45, anti-human CD19, anti-human CD33 (all from BD PharMingen). Stained cells were analyzed on a FACScanto I cytometer, and the presence of a single CD34+ population in the human CD45+ population was considered as AML engraftment.Citation36
Table 1. Characteristics of AML patient.
Treatment was initiated when human leukemic cells in the mice blood reached 3%, which was achieved 31 d after the initial injection of AML cells. Mice were then randomized into 3 groups of treatment: evNK (n = 6), NKhd (n = 6), and control (n = 5). Each 3–4 d during 14 days, 5.106 evNK or 5 × 106 NKhd were intravenously injected per mouse, each in 200 μl PBS, via the retro-orbital venous sinus and under isoflurane gas-anesthesia. Namely, each NK-treated mouse received 107 NK per week. In parallel, control animals received 200 μl PBS. All mice received interleukin-15 (0.5 μg/mouse in 200 μl PBS) via intraperitoneal injection concomitantly with NK or PBS treatment.Citation37 Mice were monitored once a week by weight assessment and blood collection for determination of leukemic burden. Mice were killed by cervical dislocation after 2 weeks of treatment. In addition, for each group, 1 mouse was kept alive for 2 additional weeks without any treatment to detect a possible delayed effect of NK immunotherapy. At sacrifice, bone marrow (BM) and spleens were analyzed for total cell number and the presence of leukemic cells and NK cells. Flow cytometry analysis was performed using: anti-mouse CD45, anti-human CD45, anti-human CD34, anti-human CD34, and anti-human CD56 (all from BD PharMingen). White blood cell (WBC) count was determined on a Melet Schloesing MS9–5V hematology analyzer (Melet Schloesing Laboratoires). BM and spleen cell numbers were counted using a Sysmex counter (Sysmex) according to the manufacturer's instructions. The absolute number of human leukemic cells in the BM and spleen was calculated by the equation: total cell number × % of human CD45+ CD34+ cells. The absolute number of NK cells in the BM and spleen was calculated by the equation: total cell number × % of human CD45+ CD56+ cells.
Statistical analyses
In vitro data are expressed as mean or mean ± SD. In vivo data were analyzed with GraphPad Prism. Two-tailed unpaired student t test was used for single comparisons. Statistically significant differences are indicated by asterisks (*, p < 0.05; **, p < 0.005; ***p < 0.0005).
Results
CD94 surface expression by ex vivo differentiated natural killer correlates with their cytotoxic activity
We aimed to identify a functional marker that would reflect the lytic potential of evNK. Therefore, we selected 4 samples of evNK (issued from 4 different UCB-donors) that displayed variability in their cytotoxic activity against K562 cells after 4 weeks of in vitro co-culture with MS5 stromal cells and cytokines. As shown in , percentage of lysis varied from 0 to 28% between the 4 samples of evNK. A phenotypic analysis was performed by flow cytometry on these evNK and NK cells from healthy donors were used asa reference. We evaluated the expression NK cell-associated antigens including the receptor for the Fc portion of IgG CD16, the inhibiting receptors CD161, NKG2A, the activating receptors CD226, NKp30, NKp44, NKp46, NKG2D, the KIR receptors CD158A, CD158B, CD158E, CD158I, the memory receptor CD45RA, the lineage marker CD7, and receptors reported to be associated with NK maturation including CD94 and CD117.Citation38,39
Figure 1. Heterogeneity of cytotoxic potential and surface receptor expression among evNK issued from different UCB-donors. (A) Cytotoxic activity of evNK issued from 4 different donors against K562 target cells. Cytotoxicity was determined by a conventional 4-hr Cr51-release assay at different E:T ratios(100:1, 30:1, 10:1, 3:1).(B) Flow cytometry analysis of the surface expression of the indicated NK receptor on evNK derived from UCB-donors of . Percentage of positive cells was measured on CD56-purified evNK cells.
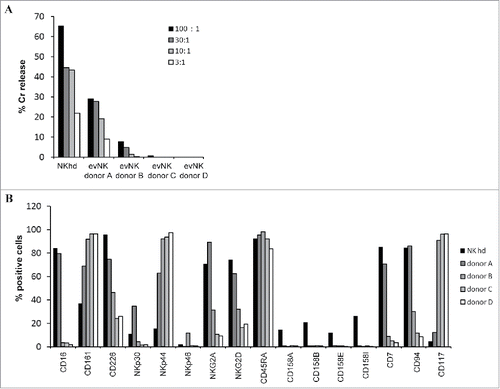
As shown in , only NKhd expressed detectable levels of KIR receptors. Apart from KIR receptors, donor A-evNK and NKhd displayed similar expression levels for CD16, NKG2A, NKG2D, CD7, CD94, and CD117 receptors. All NK showed a comparable level for CD45RA. Various expression levels were found for CD226, NKp30, NKp44, and NKp46.Comparison with the lytic potential of evNK indicates a direct correlation with the expression level of the surface differentiation marker CD94 and an inverse correlation with the expression level of CD117 ().
To confirm a potential link between CD94 expression and cytotoxic activity of evNK, we performed a CD94-based cell sorting on evNK cells derived from 3 additional UCB-donors at the end of the 4 week-co-culture and compared the cytolytic activity of CD94+ and CD94−evNK against the leukemic cell lines K562, U937 and HL-60. As shown in , , , CD94+-evNK from the 3 UCB-donors showed a consistent higher cytotoxicity against K562 (), U937 () and HL-60 () cell lines as compared with CD94−-evNK.
Figure 2. CD94 surface expression on evNK correlates with higher lytic potential. Cytotoxic activity of evNK derived from different UCB-donors was measured against K562 (A), U937 (B), and HL-60 (C) target cells at different E:T ratios (30:1, 10:1, 3:1, 1:1) by a conventional 4-hr Cr51-release assay. EvNK were either sorted based on CD94 surface expression (evNK CD94+; evNK CD94−) or not (evNK).
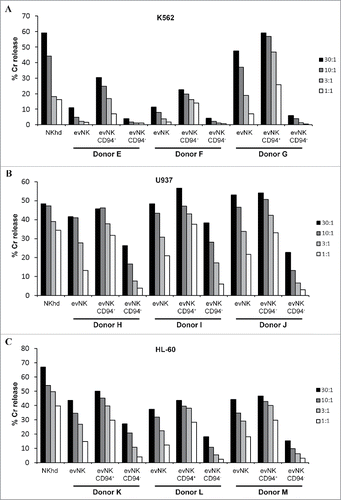
CD94+-evNK cells display higher expression of LFA-A1 and NKG2 receptors than CD94−
To better delineate the role of CD94 in evNK cytolytic functions, CD94+- and CD94−-evNK issued from CD56+-evNK from 3 UCB-donors were sorted by flow cytometry and analyzed for the expression of NK receptors including CD16, CD161, CD226, NKp30, NKp44, NKp46, NKG2A, NKG2C, NKG2D, CD7, CD45RA, and the adhesion molecule LFA-A1 recently reported to be associated with CD94 expression and NK maturation.Citation40 As shown in , CD94+- and CD94−-evNK expressed comparable levels of CD161 and NKp44, but differed with respect to CD16, CD226, NKp30,NKp46, NKG2A, NKG2C, NKG2D, CD7, CD45RA, and LFA-A1 receptors that were consistently higher expressed in CD94+- than in CD94−evNK (, , ). Of note, since CD94 forms heterodimers with NKG2 receptors, it is expected to find increased expression of NKG2A, C, D receptors on CD94+-evNK.
Figure 3. CD94-positive evNK display higher expression of NKG2 receptors and LFA-1. (A) (B) (C) Comparative flow cytometry analysis of surface expression ofthe indicated NK receptors on evNK derived from 3 UCB donors and sorted (CD94+; CD94−) or not (Tot) based on CD94 surface expression. Percentage of positive cells was measured on CD56-purified evNK cells (Tot), CD56-purified then CD94-positive sorted evNK cells (CD94+), and CD56-purified then CD94-negative sorted evNK cells (CD94−).
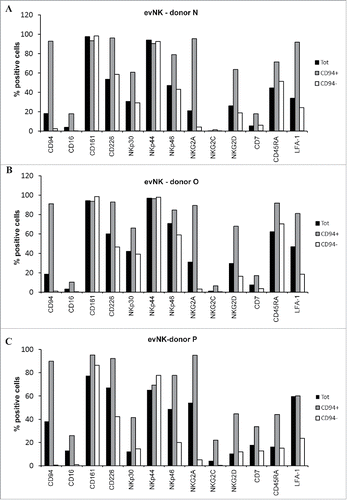
CD94+-evNK and not CD94−-evNK form immune synpases with leukemic cell lines
To further investigate the role of CD94 in evNK cytolytic functions, we studied the ability of CD94+- and CD94−- evNK to form immune synapses with K562, U937, and HL-60 target cells. NK and target cells co-cultures were stained to visualize F-actin accumulation at the immune synapse, the first critical event for NK immune synapse formation and functions.Citation41 Clearly, CD94+-evNK were more able to form synapses with K562, U937, and HL-60 than CD94−-evNK as shown in ( and ). We observed consistently more F-actin accumulation and polarization within synaptic zone between CD94+-evNK and leukemic cells than between CD94−-evNK and leukemic cells. This suggests that CD94+-evNK are capable of interacting with leukemic cells whereas CD94−-evNK are not.
Figure 4. CD94-positive evNK are efficient to form immune synapses with target leukemic cells unlike CD94-negative ones. (A) Quantification of CD94-positive and CD94-negative evNK involved in immune synapse formation with K562, U937, and HL-60target cells. EvNK were sorted based on CD94 expression and CD94-positive and –negative evNK were subsequently co-cultured for 30 min with target leukemic cells. Results are means of 2 independent experiments and are expressed as percent of evNK cells involved in immune synapses/total evNK. *p < 0,05. (B) Representative illustration of immune synapse formation between K562, U937, or HL-60 leukemic cells and CD94+-evNK. Immune synapses are visualized by F-actin staining with rhodamine-phalloidine (purple). DAPI (blue) is used for nuclei labeling. Scale bars, 5 µm. L: leukemic cells; NK: NK cells.
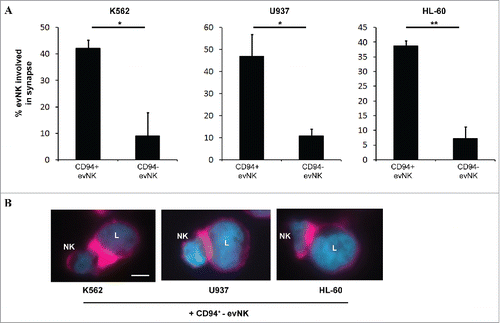
This interaction is not due to an interaction of CD94 with its ligand HLA-E on target cells.Citation42-44 Indeed, K562, U937 and HL-60 cells were negative for HLA-E expression by flow cytometry (data not shown). However, we cannot exclude a contribution of LFA-1Citation45 since these target cells strongly expressed the LFA-A1 receptor ICAM-1Citation46 (data not shown).
CD94+ cells form conjugates with primary leukemic cells in vivo following adoptive transfer of evNK in leukemic NSG mice
We first evaluated in vitro the cytotoxicity of evNK and NKhd against patient-derived AML cells, using K562 cells as positive control for NKhd and evNK cytotoxicity. AML cells were found to be completely resistant to evNK and NKhd as compared with K562 cells (). We continued using these AML cells for adoptive transfer, questioning whether the in vivo mouse environment would alter the cytotoxic response of NK cells or the sensitivity of AML cells.
Figure 5. Patient-derived AML cells are resistant to evNK and NKhd. Cytotoxic activity of evNK and NKhd was measured against patient-derived AML cells at different E:T ratios (30:1, 10:1, 3:1, 1:1). K562 cells were used as positive control of NK cytotoxicity.
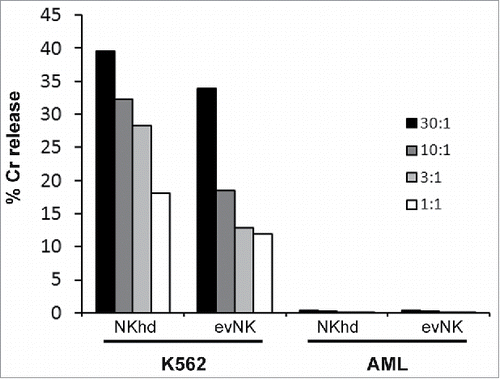
To evaluate in vivo the toxicity, tissue distribution and anti-leukemic potential of evNK, AML-engrafted immunodeficient mice were adoptively transferred with evNK and NKhd were used as reference. After 2 weeks of treatment, there was no evidence of toxicity of evNKs or NKhd cells in mice since any death nor did weight loss occur among treated mice (data not shown). Organ distribution analysis showed that evNK and NKhd preferentially circulated in the blood and rested in the spleen with a minority of cells reaching the BM (). However, NKhds reached higher levels than evNKs ().
Figure 6. Organ distribution of evNK and NKhd in AML-engrafted mice. 5.106 evNK or NKhd were intravenously injected per mouse each 3–4 d for 14 d in AML-engrafted NSG mice. Percentages (A) and absolute numbers (B) of evNK and NKhd isolated from blood, spleen, and BM of AML-engrafted mice. EvNK and NKhd were identified using anti-human CD45 and CD56 antibodies. Mouse cells were excluded using anti-mouse CD45 antibody. n = 6–7 for evNK or NKhd groups.
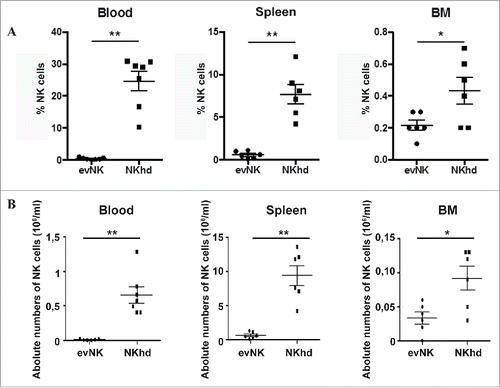
EvNK and NKhd did not show anti-leukemic activitysince the absolute numbers of leukemic cells in AML-engrafted mice after the 2 week-treatmentdid not decrease (). This correlated with in vitro data ().
Figure 7. Absence of anti-leukemic effect of evNKand NKhd in AML-engrafted mice. Percentages (A) and absolute numbers (B) of AML cells isolated from blood, spleen, and BM of AML-engrafted NSG mice at day 14 after evNK and NKhd intravenous injection. AML were identified using anti-human CD45 and CD34 antibodies. Mouse cells were excluded using anti-mouse CD45 antibody. n = 6–7 for control, evNK, or NKhd groups.
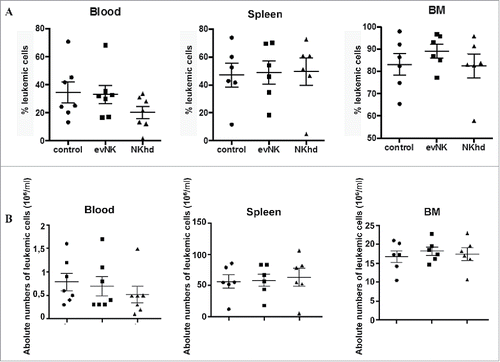
Interestingly, phenotypic analysis of blood from evNK-injected mice revealed the presence of a population stained with CD56+ and CD34+ (). This population was not present in the leukemic mice not injected with NK (), suggesting conjugate formation between NK and leukemic cells in vivo. This “double positive” population also expressed CD94. Of note, the entireev NK cells found in vivo 2 weeks after the adoptive transfer expressed the CD94 receptor ()whereas no more than 20% of the injected evNK were positive for this marker (data not shown). Immmunophenotyping of NKhd found in mice blood at 2 weeks also showed the double-stained population and contained the same proportions of CD94+ cells ().
Figure 8. CD94-positive evNK interact with AML cells. Representative dot plots of CD56, CD94, and CD34 expression in blood isolated from AML-engrafted NSG mice injected with either evNK (A) or NKhd (B) at day 14 after NK cell intravenous injection. Untreated AML-engrafted mice were used as control (C). Anti-human CD45, CD56 and CD94 antibodies were used for NK cell identification. Anti-human CD45 and CD34 antibodies were used for AML cell identification. Bold numbers represent the percentage of cells in the corresponding quadrant. The percentages indicated in the third to fifth columns are calculated within human cells (P2 gate, hCD45+).
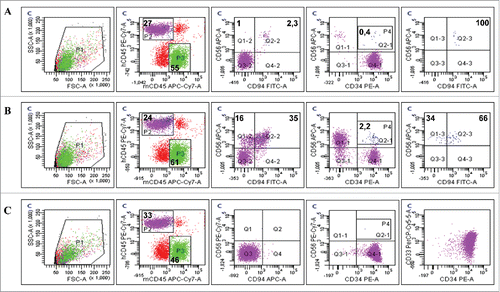
As mentioned previously (see Mat. and Meth. Section), one mouse from each treatment group was kept alive for 2 additional weeks without NK administration to detect an eventual delayed anti-leukemic effect of NK. At sacrifice (at day 28 after the first treatment injection), there was no delayed effect of NK immunotherapy (). In fact, absolute numbers of human CD45+CD34+ leukemic cells were similar across the 3 treatment groups as shown in . Strickingly, we noticed that evNK maintained their level in blood, spleen, and BM whereas NKhd levels decreased to reach the same level as evNK cells in organs. Absolute numbers of both types of NKs were very low in the blood 4 weeks after adoptive transfer (). Remarkably, almost all the remaining evNK displayed high level of expression of CD94 () just as NKhd (), raising the hypothesis that CD94-positive evNK are long-living cells.
Figure 9. Absence of delayed anti-leukemic effect by evNK of NKhd at day 28 post-NK injection. Absolute numbers of AML (A) and evNK/NKhd (B) cells in the blood, spleen, and BM of AML-engrafted NSG mice at day 28 after the first NK intravenous injection. n = 1 for control, evNK, and NKhd groups.
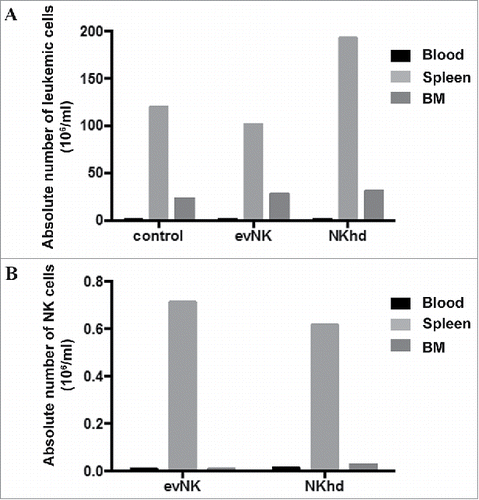
Figure 10. Residual evNK at day 28 are CD94-positive. Representative dot plots of CD56, CD94 and CD34 expression in blood isolated from AML-engrafted NSG mice injected with either evNK (A) or NKhd (B) at day 28 after the first NK cell intravenous injection. Anti-human CD45, CD56 and CD94 antibodies were used for NK identification. Anti-human CD45 and CD34 antibodies were used for AML identification. Bold numbers represent the percentage of cells in the corresponding quadrant. The percentages indicated in the third to fifth columns are calculated within human cells (P2 gate, hCD45+).
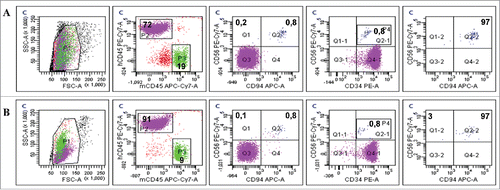
Discussion
Our study and othersCitation24-32 confirm that generation of functional human NK cells derived from CD34+ cells isolated from freshly collected UCB units is feasible. However, parameters involved in generating fully functional and mature NK cells remain a subject of investigations.
Here we first show that at the term of the 4-week differentiation process, evNK display variability in their cytotoxic function and the expression of NK receptors despite their acquired CD56 expression. In particular, NK receptor associated with lymphocyte lineage (CD7) and NK maturation such as CD94 and CD117 were differentially expressed, which may reflect different kinetics associated with UCB-donors.
We also show that the expression of the CD94 receptor on evNK is associated with a high cytotoxic potential in these cells.CD94+-evNK displayed a more mature phenotype characterized by a higher expression of CD16, CD226, NKp30, NKp46, NKG2A, NKG2C, NKG2D, CD7, CD45RA, and LFA-A1 receptors. Acquisition of CD94 by evNK seems to illustrate a developmental stage in NK differentiation that correlates with the acquisition of a more mature and functional phenotype. Our results parallel those of Freud et al. who studied NKs freshly isolated from human secondary lymphoid tissues (SLT)and depicted 4 differentiation stages for human NK cells in SLT, the last and more mature stage (stage 4) being characterized by CD94 expression and CD117 loss.Citation39 In reference to this classification, donors C and D may correspond to stage 3 (CD94−/CD117+).Citation39 Grzywacz et al. also proposed that CD94 expression on evNK corresponds to a more mature stage of differentiation associated with the acquisition of natural cytoxicity and IFNγ production.Citation38
CD94+-evNK form significantly more synapses with leukemic target cells as compared with CD94−-evNK. Since K562 target cells did not express the CD94/NKG2 ligand HLA-E, CD94 may not directly drive interactions with target cells. Rather, since LFA-1 is known to be important for NK synapse formation,Citation45 the increased LFA-1 expression on CD94+-evNK may be involved in their interaction with leukemic target cells that display high levels of ICAM-1, the LFA-1 receptor.Citation46 Inaddition, LFA-1 expression has been associated with late development stage of NK cells.Citation40 Blockade of CD94 will shed light on its exact function in synapse formations. CD94 expression in evNK may also be associated with a global transcriptional profile associated with more mature stages of evNK compatible with target cell recognition and interaction.
Which factors govern the acquisition of CD94 by evNK are not known, nor yet we know which cytokine combination or concentration to use to increase CD94 rates in evNK population. A role for IL-12 is likely since IL-12 has been involved in CD94 upregulation on evNK.Citation47 In addition, Boieri et al. have recently reported that IL-12 and IL-18, in association with IL-15, are able to increase the lytic potential of NK.Citation37,48 Future studies are warranted to refine culture parameters to optimize the rate of CD94+-evNK.
In mice, the role of CD94 is still a subject of debate. A study by Orr et al., who generated C57Bl/6 CD94−deficient mice by backcrossing CD94-deleted I29/SvJ embryonic cells to C57Bl/6 genome, concluded that NK cells derived from CD94-deficient mice develop normally and efficiently kill NK cell-susceptible YAC tumor and splenocyte target cells.Citation49 In another hand, Yu et al. demonstrated that CD94+ NK derived from wild type C57Bl/6 mice were more cytolytic than their CD94− counterpart.Citation50 These discrepancies between these studies were suggested to be related to the differential Ly49 inhibitory repertoire expressed by NK from transgenic CD94-deficient C57Bl/6NK cells (containing the I29/SvJ haplotype) and NK from wild type C57BI/6 NK cells. Further studies are still warranted to uncover the role of mouse CD94 receptor in NK cell-mediated toxicity.
This study also aimed to evaluate the toxicity, biodistribution and anti-leukemic potential of evNK following adoptive transfer in immune-deficient mice. EvNK were not toxic in accordance with other studies of adoptive transfer of UCB-derived NK cells in mice.Citation31,37
Concerning organ distribution, BM remained a sanctuary for leukemic cells but not for both evNK and NKhd. This separated distribution of AML and NK cells may account for the absence of anti-leukemic effect. Therefore, it is of interest to understand why NK cells are driven away from BM. That could be due to changes in chemokine microenvironment in BM induced by AML and resulting in driving NK outside this organ. This phenomenon was described in multiple myeloma-bearing mice.Citation51 Also, the CXCR4 receptor, reported to retain NK cells in the BM,Citation52 may be lacking in evNK and NKhd. A study of the panel of chemokines/chemokine receptors of evNK cells and AML cells should be performed to help understanding NK biodistribution.Citation53,54
Only 2 previous studies by Cany et al. evaluated adoptive transfer of UCB-derived evNK in leukemic mice. The authors used mice engrafted with K562 cells which are constitutively sensitive to NK lysis, and showed that treatment with UCB-NK cells potently inhibited progression of K562 leukemic cells, corroborating in vitro data.Citation31,37 Here we used a patient-derived AML strain for mice engraftment that was resistant in vitro to NK-mediated lysis questioning the impact of mice environment on AML response to NK. EvNK as well as NKhd intra-venous transfer did not decrease leukemic cell numbers in mice in the different organs, demonstrating that mice environment did not impact on NK lytic potential or AML sensitivity. But, at the end of NK treatment after mice sacrifice, we identified in mice blood a subpopulation of cells double-stained for the human leukemic marker CD34 and the human NK cell marker CD56. This suggests conjugate formation between leukemic and NK cells. Since this phenomenon did not lead to killing of leukemic cells by NK, additional elucidation of AML resistance mechanisms to NK lysis is needed. Interestingly, all the evNK cells analyzed after 2 weeks of treatment expressed the CD94 whereas no more than 20% of the injected evNK were positive for this marker. Whether it was by in vivo selection of CD94+evNKor by in vivo differentiation is not known and should be investigated. CD94 expression on all NK cells was maintained after 4 weeks, raising the hypothesis that CD94 positive NKs could be long living cells. This phenomenon of long-lasting NKs was described after human CMV reactivation, where the viral infection promoted the expansion of a cytolytic, durable subtype of NK cells, which was termed by the authors as “adaptive NK cells.” These cells expressed NKG2C, had a specific methylation profile and played a role in preventing leukemia relapse.Citation55
In conclusion, evNK differentiation from UCB-CD34+ cells is feasible and yields functional NK, and CD94 was found to be a marker of in vitro and in vivo functionality. Nevertheless, further understanding of the role of CD94 in the cytotoxicity displayed by evNK is needed, as well as the culture parameters that govern its expression by evNK. As for therapeutic implications, cell sorting by CD94 selection may represent an interesting option in the future to select highly functional evNK cells before adoptive transfer.
Disclosure of potential conflicts of interest
No potential conflicts of interest were disclosed.
Funding
This work was supported by the Qatar foundation (AESF 01–008–3–003), the french “Institut National du Cancer” (PRT-K 2012), and the french “Ligue contre le Cancer” (LNCC-Equipe labéllisée).
References
- Copelan EA. Hematopoietic stem-cell transplantation. N Engl J Med 2006; 354:1813-26; PMID:16641398; https://doi.org/10.1056/NEJMra052638
- Appelbaum FR. Haematopoietic cell transplantation as immunotherapy. Nature 2001; 411:385-9; PMID:11357147; https://doi.org/10.1038/35077251
- Kolb HJ. Graft-versus-leukemia effects of transplantation and donor lymphocytes. Blood 2008; 112:4371-83; PMID:19029455; https://doi.org/10.1182/blood-2008-03-077974
- Horowitz MM, Gale RP, Sondel PM, Goldman JM, Kersey J, Kolb HJ, Rimm AA, Ringdén O, Rozman C, Speck B, et al. Graft-versus-leukemia reactions after bone marrow transplantation. Blood 1990; 75:555-62; PMID:2297567.
- Barrett AJ. Understanding and harnessing the graft-versus-leukaemia effect. Br J Haematol 2008; 142:877-88; PMID:18564358; https://doi.org/10.1111/j.1365-2141.2008.07260.x
- Marks DI, Lush R, Cavenagh J, Milligan DW, Schey S, Parker A, Clark FJ, Hunt L, Yin J, Fuller S, et al. The toxicity and efficacy of donor lymphocyte infusions given after reduced-intensity conditioning allogeneic stem cell transplantation. Blood 2002; 100:3108-14; PMID:12384406; https://doi.org/10.1182/blood-2002-02-0506
- Takami A, Yano S, Yokoyama H, Kuwatsuka Y, Yamaguchi T, Kanda Y, Morishima Y, Fukuda T, Miyazaki Y, Nakamae H, et al. Donor lymphocyte infusion for the treatment of relapsed acute myeloid leukemia after allogeneic hematopoietic stem cell transplantation: A retrospective analysis by the Adult Acute Myeloid Leukemia Working Group of the Japan Society for Hematopoietic Cell Transplantation. Biol Blood Marrow Transplant 2014; 20:1785-90; PMID:25034960; https://doi.org/10.1016/j.bbmt.2014.07.010
- Ferrara JL, Levine JE, Reddy P, Holler E. Graft-versus-host disease. Lancet 2009; 373:1550-61; PMID:19282026; https://doi.org/10.1016/S0140-6736(09)60237-3
- Reddy P, Ferrara JL. Immunobiology of acute graft-versus-host disease. Blood Rev 2003; 17:187-94; PMID:14556773
- Topham NJ, Hewitt EW. Natural killer cell cytotoxicity: How do they pull the trigger? Immunology 2009; 128:7-15; PMID:19689731; https://doi.org/10.1111/j.1365-2567.2009.03123.x
- Bryceson YT, March ME, Ljunggren HG, Long EO. Activation, coactivation, and costimulation of resting human natural killer cells. Immunol Rev 2006; 214:73-91; PMID:17100877; https://doi.org/10.1111/j.1600-065X.2006.00457.x
- Ruggeri L, Capanni M, Casucci M, Volpi I, Tosti A, Perruccio K, Urbani E, Negrin RS, Martelli MF, Velardi A. Role of natural killer cell alloreactivity in HLA-mismatched hematopoietic stem cell transplantation. Blood 1999; 94:333-9; PMID:10381530
- Ruggeri L, Capanni M, Urbani E, Perruccio K, Shlomchik WD, Tosti A, Posati S, Rogaia D, Frassoni F, Aversa F, et al. Effectiveness of donor natural killer cell alloreactivity in mismatched hematopoietic transplants. Science 2002; 295:2097-100; PMID:11896281; https://doi.org/10.1126/science.1068440
- Ruggeri L, Mancusi A, Capanni M, Urbani E, Carotti A, Aloisi T, Stern M, Pende D, Perruccio K, Burchielli E, et al. Donor natural killer cell allorecognition of missing self in haploidentical hematopoietic transplantation for acute myeloid leukemia: Challenging its predictive value. Blood 2007; 110:433-40; PMID:17371948; https://doi.org/10.1182/blood-2006-07-038687
- Olson JA, Leveson-Gower DB, Gill S, Baker J, Beilhack A, Negrin RS. NK cells mediate reduction of GVHD by inhibiting activated, alloreactive T cells while retaining GVT effects. Blood 2010; 115:4293-301; PMID:20233969; https://doi.org/10.1182/blood-2009-05-222190
- Uharek L, Glass B, Gaska T, Zeiss M, Gassmann W, Loffler H, Müller-Ruchholtz W. Natural killer cells as effector cells of graft-versus-leukemia activity in a murine transplantation model. Bone Marrow Transplant 1993; 12(Suppl 3):S57-60; PMID:8124260
- Geller MA, Miller JS. Use of allogeneic NK cells for cancer immunotherapy. Immunotherapy 2011; 3:1445-59; PMID:22091681; https://doi.org/10.2217/imt.11.131
- Guillerey C, Huntington ND, Smyth MJ. Targeting natural killer cells in cancer immunotherapy. Nat Immunol 2016; 17:1025-36; PMID:27540992; https://doi.org/10.1038/ni.3518
- Locatelli F, Moretta F, Brescia L, Merli P. Natural killer cells in the treatment of high-risk acute leukaemia. Semin Immunol 2014; 26:173-9; PMID:24613727; https://doi.org/10.1016/j.smim.2014.02.004
- Gill S, Olson JA, Negrin RS. Natural killer cells in allogeneic transplantation: Effect on engraftment, graft- versus-tumor, and graft-versus-host responses. Biol Blood Marrow Transplant 2009; 15:765-76; PMID:19539207; https://doi.org/10.1016/j.bbmt.2009.01.019
- Chouaib S, Pittari G, Nanbakhsh A, El Ayoubi H, Amsellem S, Bourhis JH, Spanholtz J. Improving the outcome of leukemia by natural killer cell-based immunotherapeutic strategies. Front Immunol 2014; 5:95; PMID:24672522; https://doi.org/10.3389/fimmu.2014.00095
- Koepsell SA, Miller JS, McKenna DH Jr. Natural killer cells: A review of manufacturing and clinical utility. Transfusion 2013; 53:404-10; PMID:22670662; https://doi.org/10.1111/j.1537-2995.2012.03724.x
- Freud AG, Becknell B, Roychowdhury S, Mao HC, Ferketich AK, Nuovo GJ, Hughes TL, Marburger TB, Sung J, Baiocchi RA, et al. A human CD34(+) subset resides in lymph nodes and differentiates into CD56bright natural killer cells. Immunity 2005; 22:295-304; PMID:15780987; https://doi.org/10.1016/j.immuni.2005.01.013
- Miller JS, Verfaillie C, McGlave P. The generation of human natural killer cells from CD34+/DR- primitive progenitors in long-term bone marrow culture. Blood 1992; 80:2182-7; PMID:1384796
- Luevano M, Madrigal A, Saudemont A. Generation of natural killer cells from hematopoietic stem cells in vitro for immunotherapy. Cell Mol Immunol 2012; 9:310-20; PMID:22705914; https://doi.org/10.1038/cmi.2012.17
- Mrozek E, Anderson P, Caligiuri MA. Role of interleukin-15 in the development of human CD56+ natural killer cells from CD34+ hematopoietic progenitor cells. Blood 1996; 87:2632-40; PMID:8639878
- Kao IT, Yao CL, Kong ZL, Wu ML, Chuang TL, Hwang SM. Generation of natural killer cells from serum-free, expanded human umbilical cord blood CD34+ cells. Stem Cells Dev 2007; 16:1043-51; PMID:17999637; https://doi.org/10.1089/scd.2007.0033
- Luevano M, Domogala A, Blundell M, Jackson N, Pedroza-Pacheco I, Derniame S, Escobedo-Cousin M, Querol S, Thrasher A, Madrigal A, et al. Frozen cord blood hematopoietic stem cells differentiate into higher numbers of functional natural killer cells in vitro than mobilized hematopoietic stem cells or freshly isolated cord blood hematopoietic stem cells. PloS One 2014; 9:e87086; PMID:24489840; https://doi.org/10.1371/journal.pone.0087086
- Vasu S, Berg M, Davidson-Moncada J, Tian X, Cullis H, Childs RW. A novel method to expand large numbers of CD56(+) natural killer cells from a minute fraction of selectively accessed cryopreserved cord blood for immunotherapy after transplantation. Cytotherapy 2015; 17:1582-93; PMID:26432560; https://doi.org/10.1016/j.jcyt.2015.07.020
- Spanholtz J, Tordoir M, Eissens D, Preijers F, van der Meer A, Joosten I, Schaap N, de Witte TM, Dolstra H. High log-scale expansion of functional human natural killer cells from umbilical cord blood CD34-positive cells for adoptive cancer immunotherapy. PloS One 2010; 5:e9221; PMID:20169160; https://doi.org/10.1371/journal.pone.0009221
- Cany J, van der Waart AB, Tordoir M, Franssen GM, Hangalapura BN, de Vries J, Boerman O, Schaap N, van der Voort R, Spanholtz J, et al. Natural killer cells generated from cord blood hematopoietic progenitor cells efficiently target bone marrow-residing human leukemia cells in NOD/SCID/IL2Rg(null) mice. PloS One 2013; 8:e64384; PMID:23755121; https://doi.org/10.1371/journal.pone.0064384
- Ayello J, van de Ven C, Fortino W, Wade-Harris C, Satwani P, Baxi L, Simpson LL, Sanger W, Pickering D, Kurtzberg J, et al. Characterization of cord blood natural killer and lymphokine activated killer lymphocytes following ex vivo cellular engineering. Biol Blood Marrow Transplant 2006; 12:608-22; PMID:16737934; https://doi.org/10.1016/j.bbmt.2006.01.009
- Amsellem S, Pflumio F, Bardinet D, Izac B, Charneau P, Romeo PH, Dubart-Kupperschmitt A, Fichelson S. Ex vivo expansion of human hematopoietic stem cells by direct delivery of the HOXB4 homeoprotein. Nat Med 2003; 9:1423-7; PMID:14578882; https://doi.org/10.1038/nm953
- Nanbakhsh A, Pochon C, Amsellem S, Pittari G, Tejchman A, Bourhis JH, Chouaib S. Enhanced cytotoxic activity of ex vivo-differentiated human natural killer cells in the presence of HOXB4. J Immunother 2014; 37:278-82; PMID:24810639; https://doi.org/10.1097/CJI.0000000000000039
- Hirsch P, Zhang Y, Tang R, Joulin V, Boutroux H, Pronier E, Moatti H, Flandrin P, Marzac C, Bories D, et al. Genetic hierarchy and temporal variegation in the clonal history of acute myeloid leukaemia. Nat Commun 2016; 7:12475; PMID:27534895; https://doi.org/10.1038/ncomms12475
- Taussig DC, Miraki-Moud F, Anjos-Afonso F, Pearce DJ, Allen K, Ridler C, Lillington D, Oakervee H, Cavenagh J, Agrawal SG, et al. Anti-CD38 antibody-mediated clearance of human repopulating cells masks the heterogeneity of leukemia-initiating cells. Blood 2008; 112:568-75; PMID:18523148; https://doi.org/10.1182/blood-2007-10-118331
- Cany J, van der Waart AB, Spanholtz J, Tordoir M, Jansen JH, van der Voort R, Schaap NM, Dolstra H. Combined IL-15 and IL-12 drives the generation of CD34+-derived natural killer cells with superior maturation and alloreactivity potential following adoptive transfer. Oncoimmunology 2015; 4:e1017701; PMID:26140247; https://doi.org/10.1080/2162402X.2015.1017701
- Grzywacz B, Kataria N, Sikora M, Oostendorp RA, Dzierzak EA, Blazar BR, Miller JS, Verneris MR. Coordinated acquisition of inhibitory and activating receptors and functional properties by developing human natural killer cells. Blood 2006; 108:3824-33; PMID:16902150; https://doi.org/10.1182/blood-2006-04-020198
- Freud AG, Yokohama A, Becknell B, Lee MT, Mao HC, Ferketich AK, Caligiuri MA. Evidence for discrete stages of human natural killer cell differentiation in vivo. J Exp Med 2006; 203:1033-43; PMID:16606675; https://doi.org/10.1084/jem.20052507
- Ahn YO, Blazar BR, Miller JS, Verneris MR. Lineage relationships of human interleukin-22-producing CD56+ RORgammat+ innate lymphoid cells and conventional natural killer cells. Blood 2013; 121:2234-43; PMID:23327921; https://doi.org/10.1182/blood-2012-07-440099
- Rak GD, Mace EM, Banerjee PP, Svitkina T, Orange JS. Natural killer cell lytic granule secretion occurs through a pervasive actin network at the immune synapse. PLoS Biol 2011; 9:e1001151; PMID:21931536; https://doi.org/10.1371/journal.pbio.1001151
- Braud VM, Allan DS, O'Callaghan CA, Soderstrom K, D'Andrea A, Ogg GS, Lazetic S, Young NT, Bell JI, Phillips JH, et al. HLA-E binds to natural killer cell receptors CD94/NKG2A, B and C. Nature 1998; 391:795-9; PMID:9486650; https://doi.org/10.1038/35869
- Kaiser BK, Pizarro JC, Kerns J, Strong RK. Structural basis for NKG2A/CD94 recognition of HLA-E. Proc Natl Acad Sci U S A 2008; 105:6696-701; PMID:18448674; https://doi.org/10.1073/pnas.0802736105
- Lee N, Llano M, Carretero M, Ishitani A, Navarro F, Lopez-Botet M, Geraghty DE. HLA-E is a major ligand for the natural killer inhibitory receptor CD94/NKG2A. Proc Natl Acad Sci U S A 1998; 95:5199-204; PMID:9560253; https://doi.org/10.1073/pnas.95.9.5199
- Orange JS, Harris KE, Andzelm MM, Valter MM, Geha RS, Strominger JL. The mature activating natural killer cell immunologic synapse is formed in distinct stages. Proc Natl Acad Sci U S A 2003; 100:14151-6; PMID:14612578; https://doi.org/10.1073/pnas.1835830100
- Marlin SD, Springer TA. Purified intercellular adhesion molecule-1 (ICAM-1) is a ligand for lymphocyte function-associated antigen 1 (LFA-1). Cell 1987; 51:813-9; PMID:3315233; https://doi.org/10.1016/0092-8674(87)90104-8
- Lehmann D, Spanholtz J, Sturtzel C, Tordoir M, Schlechta B, Groenewegen D, Hofer E. IL-12 directs further maturation of ex vivo differentiated NK cells with improved therapeutic potential. PloS One 2014; 9:e87131; PMID:24498025; https://doi.org/10.1371/journal.pone.0087131
- Boieri M, Ulvmoen A, Sudworth A, Lendrem C, Collin M, Dickinson AM, Kveberg L, Inngjerdingen M. IL-12, IL-15, and IL-18 pre-activated NK cells target resistant T cell acute lymphoblastic leukemia and delay leukemia development in vivo. Oncoimmunology 2017; 6:e1274478; PMID:28405496; https://doi.org/10.1080/2162402X.2016.1274478
- Orr MT, Wu J, Fang M, Sigal LJ, Spee P, Egebjerg T, Dissen E, Fossum S, Phillips JH, Lanier LL. Development and function of CD94-deficient natural killer cells. PloS One 2010; 5:e15184; PMID:21151939; https://doi.org/10.1371/journal.pone.0015184
- Yu J, Wei M, Mao H, Zhang J, Hughes T, Mitsui T, Park IK, Hwang C, Liu S, Marcucci G, et al. CD94 defines phenotypically and functionally distinct mouse NK cell subsets. J Immunol 2009; 183:4968-74; PMID:19801519; https://doi.org/10.4049/jimmunol.0900907
- Ponzetta A, Benigni G, Antonangeli F, Sciume G, Sanseviero E, Zingoni A, Ricciardi MR, Petrucci MT, Santoni A, Bernardini G. Multiple myeloma impairs bone marrow localization of effector natural killer cells by altering the chemokine microenvironment. Cancer Res 2015; 75:4766-77; PMID:26438594; https://doi.org/10.1158/0008-5472.CAN-15-1320
- Mayol K, Biajoux V, Marvel J, Balabanian K, Walzer T. Sequential desensitization of CXCR4 and S1P5 controls natural killer cell trafficking. Blood 2011; 118:4863-71; PMID:21911833; https://doi.org/10.1182/blood-2011-06-362574
- Maghazachi AA. Role of chemokines in the biology of natural killer cells. Curr Top Microbiol Immunol 2010; 341:37-58; PMID:20369317; https://doi.org/10.1007/82_2010_20
- Robertson MJ. Role of chemokines in the biology of natural killer cells. J Leukoc Biol 2002; 71:173-83; PMID:11818437
- Schlums H, Cichocki F, Tesi B, Theorell J, Beziat V, Holmes TD, Han H, Chiang SC, Foley B, Mattsson K, et al. Cytomegalovirus infection drives adaptive epigenetic diversification of NK cells with altered signaling and effector function. Immunity 2015; 42:443-56; PMID:25786176; https://doi.org/10.1016/j.immuni.2015.02.008