ABSTRACT
We have previously conducted a phase I trial to test the efficacy of a glypican-3 (GPC3) peptide vaccine in patients with advanced hepatocellular carcinoma (HCC); however, its immunological mechanism of action remains unclear. Here, we report a pilot study conducted to evaluate the immunological mechanisms of action of this GPC3 peptide vaccine (UMIN-CTR number 000005093). Eleven patients with advanced HCC were vaccinated with the GPC3 peptide in this trial. The primary end point was GPC3 peptide-specific immune response induced by the GPC3 peptide vaccination. The secondary endpoints were clinical and biologic outcomes. We demonstrated that the present vaccine induced GPC3 peptide-specific cytotoxic T lymphocytes (CTLs), which were found to infiltrate into the tumor. Moreover, we established GPC3 peptide-specific CTL clones from a biopsy specimen: these cells exhibited GPC3 peptide-specific cytokine secretion and cell cytotoxicity. The plasma GPC3 level tended to decrease temporarily at least once during the follow-up period. The GPC3-specific CTL frequency after vaccination was correlated with overall survival. The degree of skin reactions at the injection site correlated with the GPC3 peptide-specific CTLs. Furthermore, we sequenced the T cell receptors (TCRs) of tumor-infiltrating lymphocyte (TIL) clones, and confirmed the existence of this TCR repertoire in both tumor tissue and PBMCs. In response to these data, we are developing TCR-engineered T cell therapy using TCR sequences obtained from GPC3 peptide-specific CTL clones for improved efficacy in patients with advanced HCC.
Abbreviations
AFP | = | α-fetoprotein |
CTL | = | cytotoxic T lymphocyte |
GPC3 | = | glypican-3 |
HCC | = | hepatocellular carcinoma |
HLA | = | human leukocyte antigen |
IFA | = | incomplete Freund's adjuvant |
IFN-γ | = | interferon-γ |
MHC | = | major histocompatibility complex |
PBMC | = | peripheral blood mononuclear cell |
PIVKA-II | = | protein induced by vitamin K absence or antagonist-II |
RFA | = | radiofrequency ablation |
TNF-α | = | tumor necrosis factor-α |
Introduction
Hepatocellular carcinoma (HCC) is the sixth most common cancer and the second leading cause of cancer-related deaths. Worldwide, nearly 782,000 new cases of HCC are diagnosed each year, with 788,000 annual deaths. HCC most often develops in patients predisposed to chronic liver disease as a result of chronic alcohol abuse, hepatitis C virus (HCV) infection, non-alcoholic fatty liver disease (NAFLD), or nonalcoholic steatohepatitis (NASH).Citation1 The currently available treatment options include more established traditional methodologies such as surgery and adjuvant chemo/radiation therapy. Patients with early-stage HCC who receive standard curative treatments, such as surgical resection or local radiofrequency ablation (RFA), have a good prognosis, with a 5-year survival rate of > 70%. However, patients with HCC are often diagnosed at an advanced stage because of a lack of specific symptoms; the prognosis of these patients is generally poor, resulting in an overall 5-year survival rate of < 16%.Citation2-4 Chemotherapy is moderately tolerated owing to the coexistence of liver cirrhosis in most patients with HCC; however, this therapeutic strategy is not sufficiently effective. Sorafenib, a tyrosine kinase inhibitor that is used to treat HCC as well as other cancer types, has been found to prolong overall survival in patients with advanced HCC and thus represents the standard drug for first-line systemic treatment.Citation5-7 However, according to the Response Evaluation Criteria in Solid Tumors (RECIST), the response rate for sorafenib is very low and the incidence of adverse drug reactions high. Therefore, new treatment modalities are urgently needed to prolong survival in patients with advanced HCC while minimizing the risk of adverse events.
Immune-checkpoint inhibitors have shown substantial clinical benefits in the treatment of several cancers such as melanoma, renal cell carcinoma, lung cancer, bladder cancer, ovarian cancer, Hodgkin's lymphoma, and gastrointestinal (GI) and endometrial cancers associated with defects in DNA mismatch repair. Therefore, these compounds represent potentially attractive therapeutic options for HCC.Citation8-15 Cancer peptide vaccines, which utilize tumor-associated antigens (TAAs), especially cancer testis or oncofetal antigens that are recognized by the adaptive immune system, represent examples of such immunotherapies. Carcinoembryonic antigen glypican-3 (GPC3) is an ideal target for a peptide vaccine against HCC as this antigen is specifically overexpressed in HCC (72–81%) and correlates with unfavorable clinical outcomes.Citation16-20 To validate the GPC3-derived peptide vaccine as a new and effective therapeutic strategy against HCC, we conducted a phase I trial in patients with advanced HCC.Citation21 This study confirmed the safety of the GPC3-derived peptide vaccine and demonstrated that GPC3-specific CTL frequency was correlated with overall survival in patients with advanced HCC. However, as clinical studies performed during early phases of drug development are generally conducted in patients with advanced-stage disease, it is difficult to evaluate the expected survival benefits of the treatment being tested.
The improvement of treatment efficacy and survival outcomes for the GPC3-derived peptide vaccine requires detailed understanding of the immunological mechanisms of action of this vaccine. The aim of this pilot study was to evaluate the clinical responses and immunological mechanisms of action of GPC3-derived peptide vaccine in patients with advanced HCC, and to evaluate the clinical validity of this vaccine. We have previously published a case report describing Patient 3 who, despite exhibiting a massive degree of tumor necrosis, died of circulatory failure as a result of tumor embolism in the right atrium. In the present study, we report clinical and immunological data for all 11 patients enrolled this study.
Results
Patient characteristics
Eleven patients were enrolled in this study (). A larger number of patients with progressive disease who did not respond to sorafenib were included relative to the phase I study. Five patients received the HLA-A2-restricted GPC3144–152 peptide (FVGEFFTDV), and 6 patients received the HLA-A24-restricted GPC3298–306 peptide (EYILSLEEL). None of the patients dropped out because of adverse events caused by peptide vaccination. All patients received appropriate follow-up to monitor toxicity. The median follow-up period was 5 months (range 2–21 months). The patients' average age was 65.8 y (range 54–73 years). Nine of the 11 patients were male. One patient had a performance status (PS) of 1 and all others had a PS of 0. Staging was performed according to the TNM classification for HCC (Union for International Cancer Control). Five patients were diagnosed with Stage IVB disease. One patient had Child-Pugh class B disease, and all of others Child-Pugh class A disease. Respectively, 2 and 6 patients had hepatic B (18%) and C (55%) virus infection. All patients had been treated with conventional therapy including surgery, RFA, trans-catheter arterial embolization (TACE), and radiation therapy before receiving GPC3 peptide vaccine therapy. Although 6 (55%) patients received sorafenib before the present therapy, these patients dropped out because of adverse events (Patient 4, erythema multiforme; Patient 8, liver dysfunction) or disease progression. All other patients also showed disease progression before enrollment in this study.
Table 1. Patient characteristics and clinical response.
We evaluated the expression of GPC3 and HLA class I in primary tumors obtained before the first vaccine was administered. GPC3 expression was detected in all patient before the first vaccination (11/11, 100%), whereas surface HLA class I expression was observed in 8 of the 11 patients (73%) ().
Table 2. GPC3 specific CTL response.
Clinical responses
Patient characteristics and clinical responses in relation to GPC3-specific CTLs are shown in . Patient 6 had stable disease (SD), while all the other patients had progressive disease (PD) for 2 months according to RECIST and mRECIST assessment criteria. The median time to tumor progression (TTP) was 1.9 months (95% confidence interval [CI], 1.7 to 2.0). The median overall survival was 5.0 months (95% CI, 0.3 to 9.7) (). In Patient 3, most multiple tumors in the liver that had been detected by early phase contrast-enhanced CT scan did not exhibit contrast enhancement after GPC3 peptide vaccine therapy; these findings suggest tumor necrosis or partial tumor reduction that did not meet the PR criteria.
Circulating levels of AFP and PIVKA-II represent useful tumor markers of HCC.Citation22 It was recently reported that the level of GPC3 also serves as a predictive marker and improves diagnostic efficacy when used with other routine parameters in HCC.Citation23,24 Therefore, we evaluated the level of these tumor markers before and after vaccinations and their utility in GPC3 peptide vaccine therapy. GPC3 levels were detectable in the plasma of all patients before vaccination; these decreased temporarily at least once in Patients 1, 5, 6, 7, 8, 9, 10, and 11 during the follow-up period (8/11 patients, 72.7%; ). In 5 (Patients. 1,5,6,7, and 10) of these 8 patients, the GPC3 level fell than that of pre-vaccination baseline values. However, the serum levels of AFP and PIVKA-II increased slightly during the follow up period in nearly all patients. These results suggest that, although the GPC3 peptide vaccine therapy was unable to induce tumor responses over a large duration, some therapeutic effects against GPC3-positive cancer cells were present.
Figure 1. The plasma and serum levels of 3 tumor markers—GPC3 (pg/mL), AFP (mg/mL), and PIVKA-II (AU/mL)—in 11 patients during follow-up. The cut-off thresholds for AFP and PIVKA-II were 10 ng/mL and 40 mAU/mL, respectively.
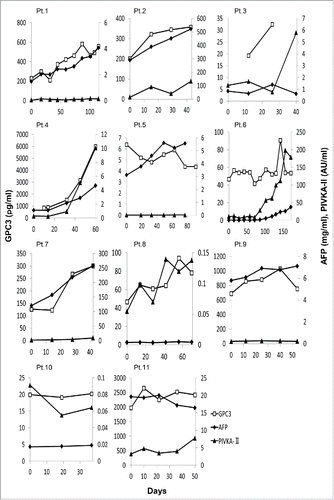
We could compare the expression of GPC3 between before and after vaccination in 4 cases (Patients 1, 3, 6, and 8) (). Representative cases 1 and 3 are shown (Fig. S2). In case 1, the expression level of GPC3 in cancer cells at pre vaccination (+++) had decreased to (++) at post vaccination, in case 3, pre (+) decreased to post (weak). In all these 4 cases, the expression of GPC3 at post vaccination was lower than that at pre vaccination (). These result may show that cancer cells that are strongly expressed GPC3 have been removed by the effect of the peptide vaccine.
Furthermore, we statistically analyzed the correlation between GPC3 expression level in tumor tissue and its plasma level at the pre vaccination ( and Fig. S3). There was a statistically significant between GPC3 expression level in tumor tissue and its plasma level at the pre vaccination (Spearman rank correlation: p = 0.02).
We also statistically analyzed the correlation between GPC3 expression level in tumor tissue and the survival of the patients (). There was not statistically significant between them (Spearman rank correlation).
And we statistically analyzed the correlation between plasma GPC3 level and the spot number of GPC3 specific CTL (). There was not statistically significant between them (Spearman rank correlation).
The frequency of vaccine-induced GPC3 peptide-specific CTLs correlated with overall survival
In cancer immunotherapy, CTLs are often the final effectors of immune-mediated cancer regression. To investigate whether the GPC3 peptide vaccine induced a specific immune response, peripheral blood mononuclear cells (PBMCs) obtained from all patients before and after vaccination were examined by ex vivo IFN-γ ELISPOT assay. After the third vaccination, the number of GPC3 peptide-specific CTLs in 5 × 105 PBMCs was found to have increased in 9 of 11 patients except for Patients. 9 and 11 (). Patients with high GPC3 peptide-specific CTL frequencies exhibited stronger skin reactions at the injection site than counterparts with low frequencies, suggesting that the degree of these reactions likely correlated with the number of GPC3 peptide-specific CTLs post-vaccination (). We previously showed that the GPC3-specific CTL frequency after vaccination was correlated with overall survival in the phase I trial. In this study, we compared patients with GPC3-specfic CTL frequencies ≥ 50 (N = 5) with those with GPC3-specific CTL frequencies < 50 (N = 6) and found that the median overall survival was 16.9 months (95% CI, 4.2 to 21.1) in patients with GPC3-specific CTL frequencies ≥ 50, compared with 4.3 months (95% CI, 2.8 to 8.4) in those with GPC3-specific CTL frequencies < 50 (p = 0.178). The maximum value of GPC3 peptide-specific CTLs after vaccination was significantly correlated with overall survival (p = 0.032, r = 0.645) (). We additionally evaluated patients with GPC3-specific CTL frequencies ≥ 50, except for Patient 2 who had a PS of 1, and Patient 3 who had been diagnosed as Child-Pugh class B. In patients with PS 0 and Child Pugh class A, the median overall survival was 20.4 months (95% CI, 16.8 to 22.3) and the maximum value of GPC3 peptide-specific CTLs after vaccination was correlated more significantly with overall survival (p = 0.010, r = 0.798) (Fig. S1).
Figure 2. Skin reactions at the injection site in patients after third vaccination. The numbers within the parentheses represent the maximum spot number of GPC3-specific CTLs after vaccination. The 2 patients on the left exhibited stronger injection site reactions relative to those shown on the right.
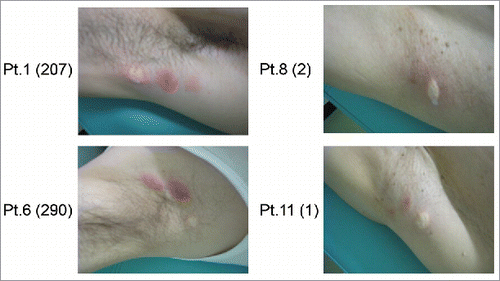
Figure 3. GPC3 peptide vaccine improved overall survivals correlated with peptide-specific CTLs. (A) Kaplan-Meier curves for overall survival. Patients with GPC3-specific CTL frequencies ≥ 50 exhibited longer survival than those with GPC3-specfic CTL frequencies < 50 (p = 0.178). (B) Correlation between GPC3-specfic CTL frequencies and overall survival. GPC3-specfic CTL frequencies after vaccination were significantly correlated with overall survival (p = 0.032, r = 0.645).
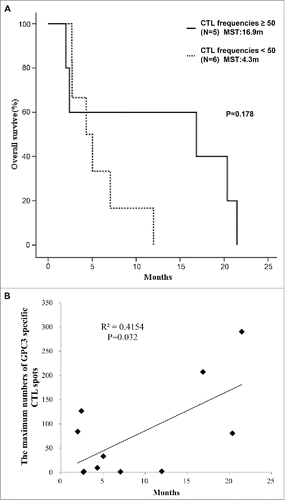
GPC3 peptide-specific CTLs infiltrated into the tumor following peptide vaccination
To confirm whether tumor-infiltrating lymphocytes were GPC3 peptide-specific CTLs, we attempted to detect GPC3 peptide-specific CTLs in biopsy specimens from vaccinated patients. Tumor biopsy specimens after administration of the third GPC3 peptide vaccine were obtained from Patients 1, 6, and 8. In Patient 6, who had the most frequent post-vaccine GPC3 peptide-specific CTLs and the best prognosis of all patients, the frequency of GPC3 peptide-specific CTLs after vaccination (290 of 5 × 105 PBMCs) was higher than that before vaccination (0 of 5 × 105 PBMCs) ex vivo in IFN-γ ELISPOT assay (A). We additionally analyzed the GPC3-specific CTL frequency of this patient by flow cytometry using the GPC3 peptide Dextramer. The GPC3-specific CTL frequency is indicated as the percentage of both Dextramer-positive and CD8-positive cells before and after vaccination. After the forth vaccination, the frequency of GPC3 peptide-specific CTLs from PBMCs and a biopsy specimen increased from 0.0% to 0.7% and 0.0% to 0.8% respectively (B). Moreover, we established GPC3 peptide-specific CTL clones from a biopsy specimen by single-cell sort using GPC3-Dextramer (C). These GPC3-Dextramer+ CTL clones showed GPC3 peptide-specific IFN-γ secretion. Moreover, the CTL clone (TIL. 1) showed cytotoxicity and IFN-γ, granzyme B, and tumor necrosis factor-α (TNF-α) secretion against GPC3-transfected or GPC3298-306 peptide-pulsed target cells, but not against vector-transfected or HIV583–591-pulsed target cells (D-G). Analysis of TCRs from isolated TIL clones revealed similar sequences to those of CTLs in peripheral blood, thereby confirming the similar TCR repertoires between these peripheral cell populations ().
Figure 4. Immunological monitoring of GPC3 peptide-specific T cell responses (A) Ex vivo IFN-γ ELISPOT assay for GPC3 in 5 × 105 PBMCs was performed before and after vaccination in Patient 6. The spot number indicates the number of GPC3 peptide-specific CTLs. The number of IFN-γ-positive spots increased from 0 to 290 in wells preincubated with GPC3 peptide. (B) Ex vivo GPC3 Dextramer staining before and after vaccination in Patient 6. GPC3 peptide-specific CTL frequency is indicated as the percentage of Dextramer-positive CTLs to CD8-positive cells in PBMCs and tumor specimens. (C) Establishment of GPC3 peptide-specific CTL clones in the tumor specimen. Dextramer analysis (left) and IFN-γ ELISPOT assay (right) of the established clones are shown. (D) CTL clone reactivity (TIL 1) against cancer cell lines. Cytotoxic effects of CTL clones against peptide-pulsed T2A24 target cells. HIV583–591 peptide-pulsed targets were used as negative controls. (E) IFN-γ ELISPOT assay against SK-Hep-1/vec, SK-Hep-1/hGPC3, and peptide-pulsed T2A24. Effector/target (E/T) ratio = 0.2. (F-G) Cytokine production by CTL clones (1.0 × 105 cells/well) after 24-h co-culture with the indicated target cells (5 × 104 cells/well). Data represent mean ± SD of triplicate cultures.
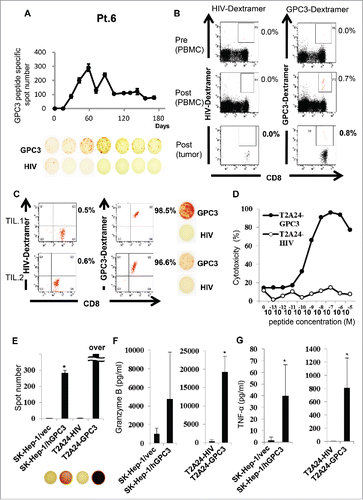
Table 3. Sequence analysis of GPC3 specific CTLs sorted from PBMCs.
Adverse events
Adverse events that were considered by the investigators to be related to treatment occurred in 100% of the patients treated with GPC3-peptide vaccine therapy (Table S1). Unfortunately, Patient 3 diagnosed as Child-Pugh class B died after the second vaccination as a result of tumor embolism in the inferior vena cava and the right atrium.Citation25 This patient was judged to have disease progression, but was not removed from the analyses at the advice of the effect and safety evaluation committee, including those of the external members. In terms of immune-related events, all patients experienced grade 1 or 2 local skin reactions at the injection site. Transient immune-related events, including drug fever, rash, and flushing, were observed in most patients. In non-immune-related events, grade 3 laboratory adverse events (impaired liver function) were observed in Patients 2, 3, and 9. These 3 patients had progressively massive or multiple liver tumors. As immune-related adverse events were within an acceptable range and laboratory adverse events were mainly caused by disease progression, these results suggest that GPC3 peptide vaccine therapy was well-tolerated.
Discussion
The aim of a therapeutic cancer vaccine is to induce the activation and proliferation of tumor associated antigen (TAA)-specific CTLs that specifically recognize and kill cancer cells, leading to improved therapeutic outcomes. We previously demonstrated the presence of GPC3 peptide-specific CTLs in peripheral blood, and showed that numerous CD8-positive T cells infiltrated tumors following GPC3 peptide vaccination in patients showing partial response. In this study, we demonstrated that GPC3 peptide-specific CTLs induced by GPC3 peptide vaccination infiltrated into the tumor tissue. These findings provide proof-of concept for GPC3 peptide vaccine therapy.
It was additionally found that GPC3-specific CTL frequency tended to correlate with overall survival, which was consistent with the findings of the previous phase I study; however, no statistical significance was observed. An explanation for this finding may be that a larger number of patients were treated with sorafenib than in the phase I study, as this drug was approved by the drug administration in Japan in 2009. Accordingly, it is possible that patients with poor sensitivity to sorafenib exhibited poor prognosis, resulting in progression of the disease despite treatment. However, the plasma GPC3 levels decreased temporarily at least once in numerous patients during the follow-up period. Therefore, the presence of vaccine-induced GPC3 peptide-specific CTLs likely conveyed positive therapeutic effects by impairing the proliferation of GPC3-positive cancer cells, since patients with low GPC3 expression tended to show unfavorable clinical outcomes. Nevertheless, large-scale, multi-institution studies should be performed to verify the therapeutic efficacy of this GPC3 peptide vaccine.
Additionally, patients with strong skin reactions at the injection site are likely to exhibit more frequent induction of GPC3 peptide-specific CTLs post-vaccination. It is possible that the skin reaction to GPC3 peptide vaccine therapy is a biomarker of an immunological response conducive to the optimal outcome. Further work is necessary to determine the mechanistic significance of the skin reaction. The presence or absence of a GPC3 peptide vaccine therapy-induced skin reaction at the injection site may be used for identification of patients who might benefit from more prolonged treatment with the present GPC3 peptide vaccine therapy or other agents.
GPC3 peptide vaccine therapy was well tolerated by patients who were unable to continue sorafenib treatment because of severe side effects. Patient 3 died after the second vaccination; the main cause of death was diagnosed as circulatory failure resulting from a tumor embolism that occupied most of the right atrium. Histological examination of this patient showed central necrosis in most of the tumor and infiltration of CD8-positive T cells in the residual HCC. In addition, 3 patients in the phase I study were found to have undergone tumor necrosis according CT scans; we detected a massive infiltration of CD8-positive T cells in the remaining liver tumor in one of 3 patients. This present study demonstrated that GPC3 peptide-specific CTLs were induced as a result of the infiltration of the GPC3 peptide vaccination into the tumor. In addition, the rate of spontaneous partial regression among patients with HCC was rare (0.406%) compared with the control arm of a randomized clinical trial.Citation26 Collectively, these findings suggest that infiltration of GPC3-specific CTLs into the tumor is correlated with tumor necrosis. Furthermore, the clinical response, together with the vaccination-induced production of GPC3-peptide specific CTLs, indicates that GPC3 peptide vaccination represents a promising approach for the treatment of HCC.
However, previous studies have reported that GPC3 peptide vaccination failed to demonstrate adequate antitumor efficacy, with no complete responses in clinical trials involving patients with advanced HCC and ovarian clear cell carcinoma.Citation21,27 The GPC3 peptide vaccine therapy as adjuvant after surgical resection has also shown limited clinical efficacy.Citation28,29 TCR-engineered T cell therapy represents an alternative to TIL therapy. TCR-engineered T cell therapy, in which highly avid TCRs are cloned from naturally occurring T cells and introduced into lymphocytes using gene transfer vectors, was applied for generation of large quantities of antigen-specific CTLs for treatment.Citation30-32 To date, clinical TCR-engineered T cells against melanoma-associated antigen recognized by T cells 1 (MART-1), glycoprotein (gp) 100, carcinoembryonic antigen (CEA), p53, melanoma-associated antigen (MAGE-)A3, and New York esophageal squamous cell carcinoma 1 antigen (NY-ESO-1) have been reported.Citation33-36 Collectively, these trials have demonstrated not only feasibility, but also significant clinical responses in patients with several cancers. The first step in TCR-engineered T cell therapy is to isolate a high-affinity T cell clone for a defined target antigen. However, these TCRs are rarely isolated from patients with high reactive T cell clones that recognize and lyse target cancer cells.Citation37 We established HLA-A*24:02 -restricted GPC3 peptide-specific CTL clones; these are of high potential utility as HLA-A*24:02 represents the most common HLA class I allele in Japan. These CTL clones show high avidity and antigen-specific killing activity against tumor cells. Furthermore, we identified TCR sequences from one patient in this study. We additionally established HLA-A*02:01-restricted GPC3 peptide-specific CTL clones; HLA-A*02:01 represents the major common HLA class I allele in Caucasians. We are currently developing TCR-engineered T cell therapy using the TCRs of these GPC3 peptide-specific CTL clones.
To improve the limited clinical efficacy of conventional peptide vaccines, a novel approach is currently being explored to target tumor-specific antigens generated via cancer-cell-specific gene mutations that occur during neoplastic transformation. Theoretically, these so-called neoantigens are not attenuated by host central tolerance in the thymus and specifically appear on cancer cells, and are therefore eliminated as non-self. As neoantigens exhibit the potential to induce more robust and specific anti-tumor T-cell responses compared with conventional TAA-targeted vaccine, these represent an attractive source of targets for vaccine therapy.Citation38-40 However, to translate this neoantigen-based approach to clinical practice, several conceptual and technical barriers must be overcome: for example, one of the major challenges is the rapid selection of immunogenically potent antigens. However, patients treated with MART1-specific CTLs generated by priming with peptide-pulsed dendritic cells demonstrated a significantly increased reactivity to other melanoma-associated proteins such as NY-ESO-1, gp100, tyrosinase, and MAGE A3, and showed good prognosis.Citation41 This result suggests that released tumor antigens presented by local antigen-presenting cells may have promoted activation of new responses to non-targeted antigens, including neoantigens, even if these tumor antigens are conventional TAAs. Therefore, the antitumor effect of the GPC3-derived peptide vaccine may be improved via an alternative approach, e.g. by overcoming T-cell exhaustion through the development of new adjuvants, novel vaccine formulations, or combination therapies with immune-checkpoint inhibitors.
In conclusion, this pilot clinical study demonstrated that GPC3 peptide-specific CTLs infiltrated into tumor tissue following peptide vaccination. We established HLA-A*24:02–restricted GPC3 peptide-specific CTL clones from tumors after vaccination, and identified TCR sequences capable of recognizing and lysing target cancer cells. Although patients with advanced HCC who did not exhibit response to sorafenib showed little clinical response, those with frequent and high induction of GPC3-specific CTLs tended to have longer prognoses with a good quality of life. We are currently developing TCR-engineered T cell therapy using TCRs of GPC3 peptide-specific CTL clones to improve efficacy in patients with advanced HCC. TCR-engineered T cells are expected to represent one of the most clinically effective immunotherapies against HCC.
Materials and methods
Patient eligibility
This clinical pilot study was performed between 2011 and 2013. Patients with advanced or metastatic HCC were enrolled after providing written, informed consent. The following eligibility criteria were used: diagnosis of HCC on the basis of imaging modalities or histological examinations; no expectation of response to other therapies including surgery, RFA, or chemotherapy (e.g., sorafenib); an Eastern Cooperative Oncology Group performance status of 0–1; age between 20 and 85 years; no prior therapy within 2 weeks; tumor tissue biopsy being practicable; HLA-A24- or HLA-A2-positive status as determined using commercially-available genomic DNA typing tests (Mitsubishi Chemical Medience, Tokyo, Japan); Child-Pugh liver function class A–B; and appropriate organ function (Neutrophil count ≥ 1,500/μL, hemoglobin ≥ 8.0 g/dL, platelets ≥ 50,000/μL, total bilirubin ≤ 3.0 mg/dL, albumin ≤ 2.8 g/dL, aspartate aminotransferase ≤ 150 IU/L, alanine aminotransferase ≤ 150 IU/L, and serum creatinine ≤ 1.5 mg/dL). The following exclusion criteria were applied: uncontrolled pleural effusion or ascites; other active malignancies; pregnancy or lactation, planned pregnancy; clinically serious infection; clinically significant heart disease (myocardial infarction or unstable angina within the past 6 months, or uncontrolled cardiac arrhythmias); severe complications including cardiac failure, renal failure, liver failure, active gastro-duodenal ulcer, ileus, and uncontrolled diabetes mellitus; severe psychiatric disorder; past history of severe drug allergy; past history of administration of GPC3 peptide vaccination; and unsuitability for the trial based on clinical judgment.
Study design and endpoints
This pilot study was a single-arm, non-randomized, open-label clinical trial aimed at evaluating the immunological efficacy of the GPC3 peptide vaccination in patients with advanced HCC. HLA-A*24:02-restricted GPC3298–306 peptide (EYILSLEEL) (American Peptide Company, Sunnyvale, CA) was administered to HLA-A24-positive patients and HLA-A*02:01-restricted GPC3144–152 peptide (FVGEFFTDV) (American Peptide Company) to HLA-A2-positive patients. Peptides were administered in liquid form, emulsified with incomplete Freund's adjuvant (IFA) (Montanide ISA-51VG, SEPPIC, Paris, France), via intradermal injection every 2 weeks. The peptides and IFA were synthesized according to Good Manufacturing Practice guidelines. Patients were administered 3.0 mg/body doses of peptide. The primary end point was GPC3 peptide-specific immune-responses induced by GPC3 peptide vaccination. The secondary endpoints were clinical and biologic outcomes. This study was approved by the Ethics Committee of the National Cancer Center, and conformed to the ethical guidelines of the 1975 Declaration of Helsinki. The trial has been registered with the University Hospital Medical Information Network Clinical Trials Registry (UMIN-CTR number 000005093).
Evaluation of toxicity and clinical response
Patients were examined for signs of toxicity during and after vaccination. Adverse events were graded according to the Common Terminology Criteria for Adverse Events v4.0 (CTCAE). Hematological examinations were conducted before each vaccination. The tumor size was evaluated by computed tomography or magnetic resonance imaging before vaccination, and then every 8–12 weeks after the first vaccination. Tumor responses were evaluated according to the Response Evaluation Criteria in Solid Tumors (RECIST) guidelines and the modified RECIST (mRECIST) assessment.Citation42
Measurement of serum GPC3, AFP, and PIVKA-II concentrations
Plasma GPC3 concentrations were measured using a fully automated assay kit provided by Sysmex Corporation (Kobe, Japan). Briefly, a biotinylated monoclonal antibody reagent was used to capture GPC3 from clinical plasma samples of patients. Further, streptavidin-coated magnetic beads were used to capture the immune complexes. Following magnetic separation and washing for B/F (bound/free fraction) separation, a second monoclonal antibody labeled with alkaline phosphatase was reacted with the immune complex. After a second round of B/F separation, the immune complex was quantified using the HISCL chemiluminescent reagent. All reactions were performed at 42°C in the HISCL–800 fully automated immunoassay system, within 17 minutes. Serum AFP and PIVKA-II concentrations in patients were measured at the time of plasma collection for detection of GPC3 using a commercially available electrochemiluminescence immunoassay kit (Roche Co., Tokyo, Japan) and a chemiluminescent enzyme immunoassay kit (Eisai Co., Tokyo, Japan), respectively. In this study, the cut-off values for AFP and PIVKA-II were set as 10 ng/mL and 40 mAU/mL, respectively.
Cell lines
T2A24 (HLA-A*24:02+, TAP−) was pulsed with GPC3298–306 peptide or HIV583–591 peptide at 25°C for 1 h. The human liver cancer cell line SK-Hep-1 (GPC3−, HLA-A*02:01/A*24:02) and derivatives transfected with human GPC3, SK-Hep-1/hGPC3 (GPC3+, HLA-A*02:01/A*24:02), were used as target cells. These cell lines were maintained in our laboratory.
Measurement of immunological response
PBMCs and ex vivo IFN-γ enzyme-linked immunospot (ELISPOT) assay
An ex vivo IFN-γ ELISPOT assay was performed to evaluate the antigen-specific CTL response, as described previously.Citation43 Peripheral blood (20 mL) was obtained from each patient before the first vaccination and 2 weeks after each vaccination, and centrifuged with a Ficoll-Paque gradient at 1300 g for 30 min in a Kubota 5220 centrifuge (KUBOTA Co., Tokyo, Japan). Isolated PBMCs were then frozen before immunological analysis. All PBMCs obtained from an individual patient were incubated in the same plate and simultaneously analyzed via an ex vivo IFN-γ ELISPOT assay. Non-cultured PBMCs (5 × 105 /well) were added to plates in the presence of peptide antigens (10 µg/mL) and incubated for 20 h at 37°C in 5% CO2. The antigen for GPC3 was either the HLA-A2-restricted GPC3144–152 (FVGEFFTDV) peptide or the HLA-A*24:02-restricted GPC3298–306 peptide (EYILSLEEL). PBMCs plus HLA-A2-restricted HIV19–27 (TLNAWVKVV) peptide (ProImmune) or HLA-A*24:02-restricted HIV583–591 (RYLKDQQLL) (ProImmune) were used as negative controls. All analyses were performed in duplicate.
Dextramer staining and flow cytometry analysis
PBMCs were stained with HLA-A*02:01 Dextramer-RPE (GPC3 144–152 [FVGEFFTDV], HIV19–27 [TLNAWVKVV]; Immudex, Copenhagen, Denmark) and HLA-A*24:02 Dextramer-RPE (GPC3 298–306 [EYILSLEEL], HIV583–591 [RYLKDQQLL]; Immudex) for 10 min at room temperature and anti-CD8-FITC (ProImmune) for 20 min at 4°C. Flow cytometry was performed using a FACSAria cell sorter (BD Biosciences).
Cytotoxicity assay
Calcein-AM-labeled T2A24 target cells were pulsed with various concentrations of peptide, starting at 10-6 M and decreasing by log steps to 10-14 M. The CTL clones were incubated with the T2A24 target cells at an effector:target (E/T) ratio of 10:1 for 4 h. The recognition efficiencies of the CTL clones were defined as described previously.Citation43
Cytokine measurements
Granzyme B and TNF-α levels in the culture supernatants were evaluated using Cytometric Bead Array Flex Sets (BD Bioscience), according to the manufacturer's protocol. The resulting data were analyzed using FCAP Array Software 3.0.
Immunohistochemical analysis
Biopsy or resected specimens were obtained from all vaccinated patients before first vaccination and a subset of post-vaccination cases when collection was possible. Patients provided informed consent for this procedure. Specimens were stained with hematoxylin and eosin or monoclonal antibodies against GPC3 (clone 1G12; 1:300 dilution; BioMosaics) and HLA class I (clone EMR8/5; 1:2,500 dilution; Hokudo), according to the manufacturers' instructions.
Generation of CTL clones
CD3+CD8+GPC3-Dextramer+ cells from PBMCs and enzyme-treated tumors were sorted using a FACSAria cell sorter and seeded in a 96-well plate (1 cell/well). Then, cells were stimulated by the addition of irradiated (100 Gy) allogeneic PBMCs (8 × 104 cells/well) as feeder cells, in AIM-V medium supplemented with 10% human AB serum, IL-2 (200 U/mL), and phytohemagglutinin-P (PHA) (5 μg/ml) for 14–21 days, as described previously.Citation43
Analysis of T cell receptor (TCR) sequences
The GPC3-Dextramer+CD8+ T cells were sorted into PCR tubes (1 cell/tube) containing cDNA reaction mix, and identification of TCR sequences was performed as described in the previous report.Citation44 In brief, cDNA was amplified using 24 TCR-BV gene family-specific forward primers and a constant region-specific reverse primer. Next, the PCR products were amplified by semi-nested PCR for screening the BV gene family. PCR products identified by specific primers were sequenced. The International Immunogenetics Information System (IMGT) database site (http://imgt.cines.fr) was used to identify the human TCR-BV gene family.
Statistical analysis
All statistical analyses were performed using R software packages (R Foundation for Statistical Computing; http://www.r-project.org). Survival rates were analyzed by the R package survfit (Kaplan-Meier method). HRs and significance levels were analyzed by the R package coxph (Cox proportional hazard analysis). Spearman rank correlations were used for correlation analysis. Statistical significance was defined as a p < 0.05.
Disclosure of potential conflicts of interest
T. N. is a scientific advisor for Ono Pharmaceutical Co., Ltd. T. N., T.Y. and K.S. are supported by fundamental research funding from Takeda Pharmaceutical Co., Ltd. T. N., N.F., and K.S. are supported by fundamental research funding from Sysmex Co., Ltd. The other authors have no potential conflicts of interest to declare regarding this study.
KONI_A_1346764_supplementary_data.zip
Download Zip (1.4 MB)Acknowledgements
This study was supported in part by the National Cancer Center Research and Development Fund (25-A-7) and (28-A-8), as well as Health and Labor Science Research Grants for Clinical Research, Japan and joint research funding from Takeda Pharmaceutical Co, Ltd. and Sysmex Co, Ltd. This study was performed as a part of a research program of the Project for Development of Innovative Research on Cancer Therapeutics (P-Direct), Ministry of Education, Culture, Sports, Science and Technology of Japan. We also thank Manami Shimomura, Kayoko Shoda, and Yukiko Kozaki for technical assistance.
Funding
This work was supported in part by the National Cancer Center Research and Development Fund (25-A-7) and (28-A-8), as well as Health and Labor Science Research Grants for Clinical Research, Japan, a research program of the Project for Development of Innovative Research on Cancer Therapeutics (P-Direct), Ministry of Education, Culture, Sports, Science and Technology of Japan and joint research funding from Takeda Pharmaceutical Co, Ltd. and Sysmex Co., Ltd.
References
- Janevska D, Chaloska-Ivanova V, Janevski V. Hepatocellular carcinoma: Risk factors, diagnosis and treatment. Open Access Maced J Med Sci 2015; 3:732-6; PMID: 27275318; https://doi.org/10.3889/oamjms.2015.111
- Altekruse SF, McGlynn KA, Reichman ME. Hepatocellular carcinoma incidence, mortality, and survival trends in the United States from 1975 to 2005. J Clin Oncol 2009; 27:1485-91; PMID: 19224838; https://doi.org/10.1200/JCO.2008.20.7753
- Izumi N. Diagnostic and treatment algorithm of the Japanese society of hepatology: A consensus-based practice guideline. Oncology 2010; 78 Suppl 1:78-86; PMID: 20616588; https://doi.org/10.1159/000315234
- Fitzmorris P, Shoreibah M, Anand BS, Singal AK. Management of hepatocellular carcinoma. J Cancer Res Clin Oncol 2015; 141:861-76; PMID: 25158999; https://doi.org/10.1007/s00432-014-1806-0
- Cheng AL, Kang YK, Chen Z, Tsao CJ, Qin S, Kim JS, Luo R, Feng J, Ye S, Yang TS, et al. Efficacy and safety of sorafenib in patients in the Asia-Pacific region with advanced hepatocellular carcinoma: A phase III randomised, double-blind, placebo-controlled trial. Lancet Oncol 2009; 10:25-34; PMID: 19095497; https://doi.org/10.1016/S1470-2045(08)70285-7
- Llovet JM, Ricci S, Mazzaferro V, Hilgard P, Gane E, Blanc JF, de Oliveira AC, Santoro A, Raoul JL, Forner A, et al. Sorafenib in advanced hepatocellular carcinoma. N Engl J Med 2008; 359:378-90; PMID: 18650514; https://doi.org/10.1056/NEJMoa0708857
- Morimoto M, Numata K, Kondo M, Hidaka H, Takada J, Shibuya A, Kobayashi S, Ohkawa S, Okuse C, Morita S, et al. Higher discontinuation and lower survival rates are likely in elderly Japanese patients with advanced hepatocellular carcinoma receiving sorafenib. Hepatol Res 2011; 41:296-302; PMID: 21348907; https://doi.org/10.1111/j.1872-034X.2011.00778.x
- Hodi FS, O'Day SJ, McDermott DF, Weber RW, Sosman JA, Haanen JB, Gonzalez R, Robert C, Schadendorf D, Hassel JC, et al. Improved survival with ipilimumab in patients with metastatic melanoma. N Engl J Med 2010; 363:711-23; PMID: 20525992; https://doi.org/10.1056/NEJMoa1003466
- Topalian SL, Hodi FS, Brahmer JR, Gettinger SN, Smith DC, McDermott DF, Powderly JD, Carvajal RD, Sosman JA, Atkins MB, et al. Safety, activity, and immune correlates of anti-PD-1 antibody in cancer. N Engl J Med 2012; 366:2443-54; PMID: 22658127; https://doi.org/10.1056/NEJMoa1200690
- Motzer RJ, Escudier B, McDermott DF, George S, Hammers HJ, Srinivas S, Tykodi SS, Sosman JA, Procopio G, Plimack ER, et al. Nivolumab versus Everolimus in advanced renal-cell carcinoma. N Engl J Med 2015; 373:1803-13; PMID: 26406148; https://doi.org/10.1056/NEJMoa1510665
- Brahmer J, Reckamp KL, Baas P, Crino L, Eberhardt WE, Poddubskaya E, Antonia S, Pluzanski A, Vokes EE, Holgado E, et al. Nivolumab versus docetaxel in advanced squamous-cell non-small-cell lung cancer. N Engl J Med 2015; 373:123-35; PMID: 26028407; https://doi.org/10.1056/NEJMoa1504627
- Powles T, Eder JP, Fine GD, Braiteh FS, Loriot Y, Cruz C, Bellmunt J, Burris HA, Petrylak DP, Teng SL, et al. MPDL3280A (anti-PD-L1) treatment leads to clinical activity in metastatic bladder cancer. Nature 2014; 515:558-62; PMID: 25428503; https://doi.org/10.1038/nature13904
- Hamanishi J, Mandai M, Ikeda T, Minami M, Kawaguchi A, Murayama T, Kanai M, Mori Y, Matsumoto S, Chikuma S, et al. Safety and antitumor activity of Anti-PD-1 antibody, nivolumab, in patients with Platinum-Resistant ovarian cancer. J Clin Oncol 2015; 33:4015-22; PMID: 26351349; https://doi.org/10.1200/JCO.2015.62.3397
- Le DT, Uram JN, Wang H, Bartlett BR, Kemberling H, Eyring AD, Skora AD, Luber BS, Azad NS, Laheru D, et al. PD-1 Blockade in tumors with mismatch-repair deficiency. N Engl J Med 2015; 372:2509-20; PMID: 26028255; https://doi.org/10.1056/NEJMoa1500596
- Ansell SM, Lesokhin AM, Borrello I, Halwani A, Scott EC, Gutierrez M, et al. PD-1 blockade with nivolumab in relapsed or refractory Hodgkin's lymphoma. N Engl J Med 2015; 372:311-9; PMID: 25482239; https://doi.org/10.1056/NEJMoa1411087
- Nakatsura T, Nishimura Y. Usefulness of the novel oncofetal antigen glypican-3 for diagnosis of hepatocellular carcinoma and melanoma. BioDrugs 2005; 19:71-7; PMID: 15807627; https://doi.org/10.2165/00063030-200519020-00001
- Nakatsura T, Yoshitake Y, Senju S, Monji M, Komori H, Motomura Y, Hosaka S, Beppu T, Ishiko T, Kamohara H, et al. Glypican-3, overexpressed specifically in human hepatocellular carcinoma, is a novel tumor marker. Biochem Biophys Res Commun 2003; 306:16-25; PMID: 12788060; https://doi.org/10.1016/S0006-291X(03)00908-2
- Capurro M, Wanless IR, Sherman M, Deboer G, Shi W, Miyoshi E, Filmus J. Glypican-3: A novel serum and histochemical marker for hepatocellular carcinoma. Gastroenterology 2003; 125:89-97; PMID: 12851874; https://doi.org/10.1016/S0016-5085(03)00689-9
- Shirakawa H, Kuronuma T, Nishimura Y, Hasebe T, Nakano M, Gotohda N, Takahashi S, Nakagohri T, Konishi M, Kobayashi N, et al. Glypican-3 is a useful diagnostic marker for a component of hepatocellular carcinoma in human liver cancer. Int J Oncol 2009; 34:649-56; PMID: 19212669
- Shirakawa H, Suzuki H, Shimomura M, Kojima M, Gotohda N, Takahashi S, Nakagohri T, Konishi M, Kobayashi N, Kinoshita T, et al. Glypican-3 expression is correlated with poor prognosis in hepatocellular carcinoma. Cancer Sci 2009; 100:1403-7; PMID: 19496787; https://doi.org/10.1111/j.1349-7006.2009.01206.x
- Sawada Y, Yoshikawa T, Nobuoka D, Shirakawa H, Kuronuma T, Motomura Y, Mizuno S, Ishii H, Nakachi K, Konishi M, et al. Phase I trial of a glypican-3-derived peptide vaccine for advanced hepatocellular carcinoma: Immunologic evidence and potential for improving overall survival. Clin Cancer Res 2012; 18:3686-96; PMID: 22577059; https://doi.org/10.1158/1078-0432.CCR-11-3044
- Nobuoka D, Kato Y, Gotohda N, Takahashi S, Nakagohri T, Konishi M, Kinoshita T, Nakatsura T. Postoperative serum alpha-fetoprotein level is a useful predictor of recurrence after hepatectomy for hepatocellular carcinoma. Oncol Rep 2010; 24:521-8; PMID: 20596642
- Ofuji K, Saito K, Suzuki S, Shimomura M, Shirakawa H, Nobuoka D, et al. Perioperative plasma glypican-3 level may enable prediction of the risk of recurrence after surgery in patients with stage I hepatocellular carcinoma. Oncotarget 2016; 8:37835-44; PMID: 28035063; https://doi.org/10.18632/oncotarget.14271
- Attallah AM, El-Far M, Omran MM, Abdelrazek MA, Attallah AA, Saeed AM, Farid K. GPC-HCC model: A combination of glybican-3 with other routine parameters improves the diagnostic efficacy in hepatocellular carcinoma. Tumour Biol 2016; 37:12571-7; PMID: 27380057; https://doi.org/10.1007/s13277-016-5127-6
- Sawada Y, Yoshikawa T, Fujii S, Mitsunaga S, Nobuoka D, Mizuno S, Takahashi M, Yamauchi C, Endo I, Nakatsura T. Remarkable tumor lysis in a hepatocellular carcinoma patient immediately following glypican-3-derived peptide vaccination: An autopsy case. Hum Vaccin Immunother 2013; 9:1228-33; PMID: 23466818; https://doi.org/10.4161/hv.24179
- Oquinena S, Guillen-Grima F, Inarrairaegui M, Zozaya JM, Sangro B. Spontaneous regression of hepatocellular carcinoma: A systematic review. Eur J Gastroenterol Hepatol 2009; 21:254-7; PMID: 19279469; https://doi.org/10.1097/MEG.0b013e328324b6a2
- Suzuki S, Sakata J, Utsumi F, Sekiya R, Kajiyama H, Shibata K, Kikkawa F, Nakatsura T. Efficacy of glypican-3-derived peptide vaccine therapy on the survival of patients with refractory ovarian clear cell carcinoma. Oncoimmunology 2016; 5:e1238542; PMID: 27999758; https://doi.org/10.1080/2162402X.2016.1238542
- Sawada Y, Sakai M, Yoshikawa T, Ofuji K, Nakatsura T. A glypican-3-derived peptide vaccine against hepatocellular carcinoma. Oncoimmunology 2012; 1:1448-50; PMID: 23243625; https://doi.org/10.4161/onci.21351
- Sawada Y, Yoshikawa T, Ofuji K, Yoshimura M, Tsuchiya N, Takahashi M, Nobuoka D, Gotohda N, Takahashi S, Kato Y, et al. Phase II study of the GPC3-derived peptide vaccine as an adjuvant therapy for hepatocellular carcinoma patients. Oncoimmunology 2016; 5:e1129483; PMID: 27467945; https://doi.org/10.1080/2162402X.2015.1129483
- Morgan RA, Dudley ME, Wunderlich JR, Hughes MS, Yang JC, Sherry RM, Royal RE, Topalian SL, Kammula US, Restifo NP, et al. Cancer regression in patients after transfer of genetically engineered lymphocytes. Science 2006; 314:126-9; PMID: 16946036; https://doi.org/10.1126/science.1129003
- Thomas S, Stauss HJ, Morris EC. Molecular immunology lessons from therapeutic T-cell receptor gene transfer. Immunology 2010; 129:170-7; PMID: 20561357; https://doi.org/10.1111/j.1365-2567.2009.03227.x
- Coccoris M, Straetemans T, Govers C, Lamers C, Sleijfer S, Debets R. T cell receptor (TCR) gene therapy to treat melanoma: Lessons from clinical and preclinical studies. Expert Opin Biol Ther 2010; 10:547-62; PMID: 20146634; https://doi.org/10.1517/14712591003614756
- Johnson LA, Morgan RA, Dudley ME, Cassard L, Yang JC, Hughes MS, Kammula US, Royal RE, Sherry RM, Wunderlich JR, et al. Gene therapy with human and mouse T-cell receptors mediates cancer regression and targets normal tissues expressing cognate antigen. Blood 2009; 114:535-46; PMID: 19451549; https://doi.org/10.1182/blood-2009-03-211714
- Parkhurst MR, Yang JC, Langan RC, Dudley ME, Nathan DN, Feldman SA, Davis JL, Morgan RA, Merino MJ, Sherry RM, et al. T Cells targeting carcinoembryonic antigen can mediate regression of metastatic colorectal cancer but induce severe transient colitis. Mol Ther 2011; 19:620-6; https://doi.org/10.1038/mt.2010.272
- Morgan RA, Chinnasamy N, Abate-Daga D, Gros A, Robbins PF, Zheng Z, Dudley ME, Feldman SA, Yang JC, Sherry RM, et al. Cancer regression and neurological toxicity following anti-MAGE-A3 TCR gene therapy. J Immunother 2013; 36:133-51; PMID: 23377668; https://doi.org/10.1097/CJI.0b013e3182829903
- Robbins PF, Morgan RA, Feldman SA, Yang JC, Sherry RM, Dudley ME, Wunderlich JR, Nahvi AV, Helman LJ, Mackall CL, et al. Tumor regression in patients with metastatic synovial cell sarcoma and melanoma using genetically engineered lymphocytes reactive with NY-ESO-1. J Clin Oncol 2011; 29:917-24; PMID: 21282551; https://doi.org/10.1200/JCO.2010.32.2537
- Johnson LA, Heemskerk B, Powell DJ, Jr., Cohen CJ, Morgan RA, Dudley ME, Robbins PF, Rosenberg SA. Gene transfer of tumor-reactive TCR confers both high avidity and tumor reactivity to nonreactive peripheral blood mononuclear cells and tumor-infiltrating lymphocytes. J Immunol 2006; 177:6548-59; PMID: 17056587; https://doi.org/10.4049/jimmunol.177.9.6548
- Vonderheide RH, Nathanson KL. Immunotherapy at large: The road to personalized cancer vaccines. Nat Med 2013; 19:1098-100; PMID: 24013748; https://doi.org/10.1038/nm.3317
- Fritsch EF, Hacohen N, Wu CJ. Personal neoantigen cancer vaccines: The momentum builds. Oncoimmunology 2014; 3:e29311; PMID: 25101225; https://doi.org/10.4161/onci.29311
- Trajanoski Z, Maccalli C, Mennonna D, Casorati G, Parmiani G, Dellabona P. Somatically mutated tumor antigens in the quest for a more efficacious patient-oriented immunotherapy of cancer. Cancer Immunol Immunother 2015; 64:99-104; PMID: 25164877; https://doi.org/10.1007/s00262-014-1599-7
- Chapuis AG, Roberts IM, Thompson JA, Margolin KA, Bhatia S, Lee SM, Sloan HL, Lai IP, Farrar EA, Wagener F, et al. T-Cell therapy using Interleukin-21-Primed Cytotoxic T-Cell lymphocytes combined with Cytotoxic T-Cell Lymphocyte Antigen-4 blockade results in long-term cell persistence and durable tumor regression. J Clin Oncol 2016; 34:3787-95; PMID: 27269940; https://doi.org/10.1200/JCO.2015.65.5142
- Lencioni R, Llovet JM. Modified RECIST (mRECIST) assessment for hepatocellular carcinoma. Semin Liver Dis 2010; 30:52-60; PMID: 20175033; https://doi.org/10.1055/s-0030-1247132
- Yoshikawa T, Nakatsugawa M, Suzuki S, Shirakawa H, Nobuoka D, Sakemura N, Motomura Y, Tanaka Y, Hayashi S, Nakatsura T. HLA-A2-restricted glypican-3 peptide-specific CTL clones induced by peptide vaccine show high avidity and antigen-specific killing activity against tumor cells. Cancer Sci 2011; 102:918-25; PMID: 21281401; https://doi.org/10.1111/j.1349-7006.2011.01896.x
- Tanaka-Harada Y, Kawakami M, Oka Y, Tsuboi A, Katagiri T, Elisseeva OA, Nishida S, Shirakata T, Hosen N, Fujiki F, et al. Biased usage of BV gene families of T-cell receptors of WT1 (Wilms' tumor gene)-specific CD8+ T cells in patients with myeloid malignancies. Cancer Sci 2010; 101:594-600; PMID: 20132220; https://doi.org/10.1111/j.1349-7006.2009.01453.x