ABSTRACT
Vγ9Vδ2 T cells contribute to the immune response against many tumor types through their direct cytotoxic activity and capacity to regulate the biological functions of other immune cells, such as dendritic cells and IFN-γ-producing CD8+ T cells. However, their presence in the tumor microenvironment has also been associated with poor prognosis in breast, colon and pancreatic cancers. Additionally, recent studies demonstrated that cytokines can confer some plasticity to Vγ9Vδ2 T cells and promote their differentiation into cells with regulatory functions. Here, we demonstrated that activation of Vγ9Vδ2 T cells isolated from healthy donors and cultured in the presence of IL-21 favors the emergence of a subpopulation of Vγ9Vδ2 T cells that express the ectonucleotidase CD73 and inhibits T cell proliferation in a CD73/adenosine-dependent manner. This subpopulation produces IL-10 and IL-8 and displays lower effector functions and cytotoxic activity than CD73-negative Vγ9Vδ2 T cells. We also showed, in a syngeneic mouse tumor model, the existence of a tumor-infiltrating γδ T cell subpopulation that produces IL-10 and strongly expresses CD73. Moreover, maturation, IL-12 production and induction of antigen-specific T cell proliferation are impaired in DC co-cultured with IL-21-amplified Vγ9Vδ2 T cells. Altogether, these data indicate that IL-21 promotes Vγ9Vδ2 T cell regulatory functions by favoring the development of an immunosuppressive CD73+ subpopulation. Thus, when present in the tumor microenvironment, IL-21 might negatively impact γδ T cell anti-tumor functions.
Introduction
γδ T cells belong to the non-conventional or innate lymphocyte family. In human adults, most peripheral blood γδ T cells express a specific T cell receptor (TCR) composed of Vγ9 and Vδ2 chains. Vγ9Vδ2 T cells share functional characteristics with conventional effector αβ T cells. For instance, they display cytotoxic activity against infected or transformed cells and produce pro-inflammatory cytokines, such as tumor necrosis factor α (TNF-α), interleukin-17 (IL-17) and interferon-γ (IFN-γ).Citation1-3 However, they also display specific properties, such as the TCR-dependent recognition of non-peptidic phosphorylated antigens, called phosphoantigens. Natural phosphoantigens, such as (E)-4-hydroxy-3-methyl-but-2-enyl pyrophosphate (HMBPP), are produced by many bacteria through the prokaryotic isoprenoid pathway (also called non-mevalonate isoprenoid pathway, or Rohmer pathway) and are extremely potent activators of human Vγ9Vδ2 T cells.Citation4,5 Phosphoantigens can be produced also by eukaryotic cells (for instance, isopentenyl pyrophosphateCitation6) via the mevalonate isoprenoid pathway that plays a key role in multiple cellular processes, such as membrane maintenance and hormone synthesis. Because of their increased metabolism and extensive use of this pathway, tumor cells stimulate the overproduction that are sensed by Vγ9Vδ2 T cells as tumor-related antigens.Citation6 Therefore, through their unique capacity to recognize phosphoantigens, Vγ9Vδ2 T cells play an essential role in anti-infection immunity and in tumor immune surveillance.Citation7,8
Thanks to the availability of synthetic clinical-grade phosphoantigens or pharmacological inhibitors of the mevalonate pathway that can trigger Vγ9Vδ2 T cell proliferation and anti-tumor response, these cells have become an attractive therapeutic tool for anti-tumor therapies. Many clinical trials have been carried out based on the adoptive transfer of ex vivo stimulated Vγ9Vδ2 T cells or on their in vivo stimulation using clinical-grade agonists.Citation9,10 Clinical trials in small cohorts based on the in vivo stimulation and amplification of Vγ9Vδ2 T cells showed objective responses in 10 to 33% of patients with hematologic and solid malignancies.Citation11 In some cases, the lack of response to therapy could be attributed to deficient expansion of effector Vγ9Vδ2 T cells.Citation12-14 However, many patients who did not respond to the treatment exhibited significant and sustained Vγ9Vδ2 T cell activation and proliferation. These results suggest that the current Vγ9Vδ2 T cell-based treatments are feasible and safe, but require a better understanding of the regulation of Vγ9Vδ2 T cell effector functions to improve their efficacy.Citation11
Interestingly, recent in vitro and in vivo data highlighted some degree of plasticity of Vγ9Vδ2 T cells driven by environmental signals that can modify their anti-tumor function and limit their efficacy.Citation12 Specifically, it has been observed that γδ T cells with pro-tumor effects produce IL-17 in mouse models of breast, hepatocellular and ovarian cancers,Citation15-17 and also in human colorectal cancer.Citation18 Moreover, γδ T cells immunosuppressive functions have been associated with DC senescence induction in human breast cancer.Citation19 More recently, Daley et al. showed that in murine and human pancreatic ductal adenocarcinoma, γδ T cells directly inhibit αβ T cell activation and infiltration via PD-L1 checkpoint ligation, thereby allowing tumor progression.Citation20 They also found that depletion or inhibition of γδ T cells is markedly protective in this cancer type.Citation20 Overall, these data support the idea that γδ T cells can be immunosuppressive in selected solid tumor types.
Therefore, much research efforts are currently focused on understanding the molecular mechanisms that govern the functional plasticity of Vγ9Vδ2 T cells as well as the role of cancer cells and cells from the tumor microenvironment in their recruitment, polarization and regulation. Because of their high plasticity, the tumor microenvironment could tilt effector Vγ9 Vδ2 T cells towards a regulatory phenotype, or favor the recruitment and development of regulatory subsets.
IL-21Citation21,22 is predominantly secreted by natural killer T (NKT) cells, T follicular helper (Tfh) cells and Th17 cells, and plays a role in the differentiation and proliferation of B cells and of CD4+ and CD8+ T lymphocytes.Citation21-23 Moreover, IL-21 exerts anti-tumor effects by inducing and expanding the pool of cytotoxic CD8+ T, NK and NKT cells, while suppressing FoxP3 expression and the expansion of regulatory T cells.Citation24-27 In line with these observations, IL-21 has been associated with clinical antineoplastic activity.Citation21 However, IL-21 is also involved in the generation of regulatory B cells that are found, together with IL-21-producing T cells, in the tumor microenvironment of several solid tumors.Citation28 Finally, IL-21 has been associated with opposing effects in hematological malignancies because it supports tumor cell proliferation in multiple myeloma, Hodgkin's lymphoma and Burkitt's lymphoma, but induces growth arrest or apoptosis of malignant lymphoid cells in non-Hodgkin B-cell lymphoma.Citation29
IL-21 can promote various functional Vγ9Vδ2 T cell phenotypes. It has been reported that IL-21 potentiates the cytolytic activity and pro-inflammatory responses of long-term cultured Vγ9Vδ2 T cells.Citation30 It also favors the differentiation of a Vγ9Vδ2 T cell sub-population into B-helper T cells.Citation31,32 Moreover, the expression of inhibitory NK receptors (NKG2A, ILT2 and CD244) is increased and that of costimulatory NK receptors (NKG2D) is reduced in IL-21-amplified Vγ9Vδ2 T cells. However, the functional significance of these phenotypic alterations is still unclear.Citation30
As it was suggested that IL-21 could be a good candidate to improve Vγ9Vδ2 T cell-based treatments, a better knowledge of its effects on Vγ9Vδ2 T cells is required. In this study, we investigated the long-term effects of IL-21 on Vγ9Vδ2 T cells. We demonstrated that activation of Vγ9Vδ2 T cells isolated from healthy donors and cultured in the presence of IL-21 amplified a subpopulation of Vγ9Vδ2 T cells that expresses the CD73 ectonucleotidase and generates adenosine. This subpopulation also produces IL-10 and IL-8 and displays lower effector functions and cytotoxic activity than CD73-negative Vγ9Vδ2 T cells. Furthermore, we showed that DC maturation and functions are impaired when they are co-cultured with IL-21-amplified Vγ9Vδ2 T cells. Finally, in the presence of AMP, supernatants from IL-21-amplified Vγ9Vδ2 T cells impaired conventional T cell proliferation in a CD73/adenosine-dependent manner. Altogether, these data indicate that IL-21 promotes Vγ9Vδ2 T cell regulatory functions by favoring the development of an immunosuppressive CD73-positive subpopulation. Thus, IL-21, which can be present in the microenvironment of some tumors, might negatively influence the anti-tumor immune response by promoting regulatory Vγ9Vδ2 T cells.
Results
Functional expression of the IL-21 receptor in Vγ9Vδ2 T cells
Several studies reportedCitation30,33 that IL-21 receptors (IL-21R) are weakly expressed in non-activated Vγ9Vδ2 T cells, and are transiently up-regulated following TCR activation, but nothing is known about IL-21R expression after TCR activation and long-term culture in the presence of IL-21. Here, we confirmed that IL-21R was weakly expressed in Vγ9Vδ2 T cells freshly isolated from peripheral blood mononuclear cells (PBMC) (, left panel). Moreover, its expression level did not change after TCR activation by HMBPP followed by 15 days of culture in the presence or not (control) of IL-21 (, right panel).
Figure 1. Expression of IL-21R in Vγ9Vδ2 T cells and induction of STAT3 phosphorylation by IL-21. (A) Representative histograms showing IL-21R expression in freshly isolated Vγ9Vδ2 T cells (left panel) and after 2 weeks of culture in the presence of IL-21 or not (control) (right panel). Overlay of isotype control (solid grey), control Vγ9Vδ2 T cells (black line) and IL-21-cultured Vγ9Vδ2 T cells (grey line). (B) Western blot analysis of STAT3 phosphorylation induced by IL-21 and total STAT3 in control (Ctrl) and IL-21-cultured Vγ9Vδ2 T cells (IL-21). Data are representative of two independent experiments performed with cells from different donors.
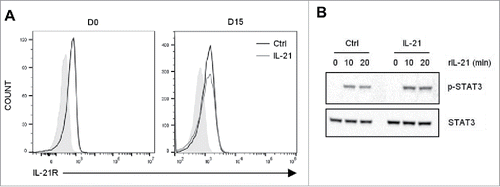
Through its receptor, IL-21 can activate the JAK-STAT pathway, and specifically STAT3 in T cells.Citation23 We thus studied IL-21R functionality in Vγ9Vδ2 T cells by analyzing STAT3 phosphorylation. To this aim, after 15 days of culture, we added recombinant human IL-21 in Vγ9Vδ2 T cells starved of cytokines for the last 24 h (). We observed STAT3 phosphorylation at 10 and 20 min after IL-21 addition in both IL-21-cultured and control Vγ9Vδ2 T cells (). These results indicate that long-term culture in the presence of IL-21 did not affect IL-21R expression and functionality in Vγ9Vδ2 T cells.
As previously described,Citation30 long-term culture with IL-21 alone did not induce sustained proliferation of Vγ9Vδ2 T cells and did not modify the expansion of Vγ9Vδ2 T cells induced by IL-2 present in the culture medium (data not shown). Moreover, IL-21 did not modify Vγ9Vδ2 T cell differentiation as indicated by the finding that at day 15 of culture most cells (control and IL-21-cultured) displayed an effector memory phenotype (CD27−/CD45RA−) (supplementary Fig S1).
IL-21-cultured Vγ9Vδ2 T cells express CD73
Extracellular ATP and adenosine act as positive and negative regulators, respectively, of the immune response. It has been recently reported that ectonucleoside triphosphate diphosphorylase 1 (ENTPD1 or CD39), which catalyzes the phosphohydrolysis of extracellular ATP into ADP and of ADP into AMP, is expressed by Vγ9Vδ2 T cells only after TCR activation. Conversely, the ecto-5′-nucleotidase CD73, which completes AMP dephosphorylation into adenosine, is expressed by circulating naive Vγ9Vδ2 T cells, but silenced upon cell activation.Citation34
In agreement, we found that after TCR activation, most Vγ9Vδ2 T cells expressed CD39 and its expression was not affected by IL-21 ( and ). Conversely, less than 3% of Vγ9Vδ2 T cells (control) expressed CD73, but this fraction increased up to 20% among IL-21-cultured cells ( and ). In comparison IL-6, a cytokine described to induce CD39 and CD73 expression in Th17 cells through a STAT3 activation mechanism,Citation35 did not modify the expression of these two ectonucleotidases by Vγ9Vδ2 T cells (). RT-qPCR analysis showed similar CD39 mRNA levels in control and IL-21-cultured Vγ9Vδ2 T cells, while CD73 mRNA was detectable only in IL-21-cultured Vγ9Vδ2 T cells (supplementary Fig. S2). Moreover, the co-expression of CD39 and CD73 by a significant percentage of IL-21-cultured Vγ9Vδ2 T cells compared with control () suggests that they might mediate the entire phosphohydrolysis process from ATP to adenosine. To address this hypothesis, we quantified AMP and adenosine levels by MALDI-TOF mass spectrometry. Control and IL-21-amplified Vγ9Vδ2 T cells produced the same amount of AMP in the presence of ATP (data not shown), whereas only IL-21-amplified cells generated adenosine in the presence of AMP (). These results demonstrate that, when cultured in the presence of IL-21, Vγ9Vδ2 T cells express CD73 and suggest that they could act as immunosuppressive cells.
Figure 2. Expression of CD39 and CD73 in Vγ9Vδ2 T cells and adenosine production. (A) Representative flow cytometry dot plots showing the percentage CD39+, CD73+ and CD39+CD73+ control (Ctrl), IL-21- (IL-21) and IL-6-cultured (IL-6) Vγ9Vδ2 T cells. This experiment was repeated 4 times and performed each time with cells from different donors. Graphs show the percentage of CD39+ (B), CD73+ (C) and CD39+/CD73+ (D) control (Ctrl) and IL-21-cultured (IL-21) Vγ9Vδ2 T cells. (E) Production of adenosine by control (Ctrl) and IL-21-cultured (IL-21) Vγ9Vδ2 T cells assessed by MALDI-TOF mass spectrometry. *p<0.05 and ***p<0.001 (paired Wilcoxon test). (F) Representative flow cytometry histogram showing CFSE staining of T cells grown in the presence of immobilized anti-CD3 monoclonal antibody and the supernatant from IL-21-amplified Vγ9Vδ2 T cells cultured with or without AMP and APCP (CD73 inhibitor) (left panel) for 4 days. The proliferation index was calculated relative to T cell proliferation in medium alone and is represented as the mean of 6 experiments performed with cells from different donors (right panel). NS non-significant, *p<0.05 (paired Wilcoxon test).
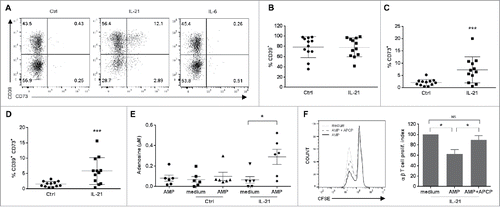
To determine whether IL-21-cultured Vγ9Vδ2 T cells could inhibit T cell proliferation through a CD73/adenosine-dependent pathway, we collected supernatants from IL-21-amplified Vγ9Vδ2 T cells after incubation (or not) with AMP for 30 min to allow the generation of adenosine. Then, we evaluated proliferation of CFSE-labeled T cells grown in the presence of immobilized anti-CD3 monoclonal antibodies and Vγ9Vδ2 T cell supernatants. T cell proliferation was reduced by ≈50% when CD3-activated T cells were cultured with supernatants collected after incubation with AMP (AMP) compared with control (supernatant without AMP) (). Pre-treating IL-21-cultured Vγ9Vδ2 T cells with the CD73 inhibitor APCP (AMP+ APCP) efficiently reversed this effect (). Conversely, we observed no difference in proliferation when T cells were cultured with supernatants from control Vγ9Vδ2 T cells with/without AMP and APCP (data not shown). Overall, these results demonstrate that IL-21-cultured Vγ9Vδ2 T cells display regulatory functions that affect the proliferative capacity of other immune cells, such as T cells, through the CD73/adenosine pathway.
IL-21-cultured Vγ9Vδ2 T cells produce IL-10 and IL-8
Analysis of cytokine production by Vγ9Vδ2 T cells showed that the percentage of IFN-γ+ and TNF-α+ cells and the release of these two cytokines were comparable in control and IL-21-cultured Vγ9Vδ2 T cells (supplementary Fig. S3).
We then asked whether IL-21-amplified Vγ9Vδ2 T cells could also produce regulatory cytokines, such as IL-10 and TGF-β. In the absence of activation by HMBPP, we detected both IL-10 mRNA expression (data not shown) and IL-10 protein () in IL-21-cultured Vγ9Vδ2 T cells, but not in control cells. Moreover, in IL-21-amplified Vγ9Vδ2 T cells, IL-10 basal production was further increased upon incubation with HMBPP (). In contrast, we did not detect TGF-β in any of the tested conditions (data not shown).
Figure 3. Production of IL-10 and IL-8 by Vγ9Vδ2 T cells. Quantification of IL-10 (A) and IL-8 (B) by ELISA in the supernatant of control (Ctrl) and IL-21-cultured (IL-21) Vγ9Vδ2 T cells activated or not with HMBPP for 6h; *p<0.05 and **p<0.01 (paired Wilcoxon test).
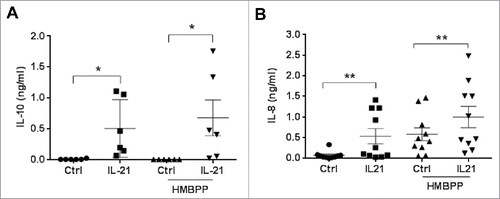
In cancer, IL-17A-producing γδ T cells also produce IL-8 and have been described as immunosuppressive cells.Citation18 We thus analyzed IL-17A and IL-8 expression in long-term cultured Vγ9Vδ2 T cells. We did not detect IL-17A mRNA or protein expression in both control and IL-21-cultured Vγ9Vδ2 T cells (data not shown). Conversely, we found a significantly higher expression of IL-8 mRNA (data not shown) and protein () in IL-21-cultured Vγ9Vδ2 T cells compared with control cells. As observed for IL-10, IL-8 basal production by IL-21-cultured Vγ9Vδ2 T cells was further increased following specific activation by HMBPP. Incubation with HMBPP also induced IL-8 production by control Vγ9Vδ2 T cells, although to a lower extent than in activated IL-21-cultured Vγ9Vδ2 T cells (). These results suggest that through the secretion of IL-10 and IL-8, IL-21-cultured Vγ9Vδ2 T cells could contribute to create a tumor microenvironment that favors tumor development and growth.
IL-21-cultured Vγ9Vδ2 T cells impair DC maturation and functions
Several reports indicate that Vγ9Vδ2 T cells modulate DC activity by favoring DC maturation and IL-12 productionCitation36 and also restore DC functions that have been impaired during pathogen infection.Citation37 To compare the effect of control and IL-21-cultured Vγ9Vδ2 T cells on DC maturation and activity, we co-cultured monocyte-derived DC with HMBPP-activated Vγ9Vδ2 T cells. Analysis of DC maturation by quantification of CD83 () and CD86 expression () after 48 h of co-culture showed that co-culture with control Vγ9Vδ2 T cells increased both the expression (MFI) of CD83 and CD86 and the percentage of positive DC compared with DC cultured alone. Conversely, co-culture with activated IL-21-amplified Vγ9Vδ2 T cells did not upregulate the expression of these two maturation markers ( and ). We next performed similar experiments with a double chamber system to address the respective role of soluble factors and contact-dependent mechanisms. We found that in the absence of cell contact DC maturation was partially impaired and similar whether DC were co-cultured with control or IL-21-amplified γδ T cells, indicating that soluble factors by themselves could not explained the differential effect of control versus IL-21-cultured γδ T cells on DC maturation (data not shown).
Figure 4. Effect of IL-21-cultured Vγ9Vδ2 T cells on dendritic cells. Monocyte-derived DC were co-cultured with control (Ctrl) or IL-21-cultured (IL-21) Vγ9Vδ2 T cells for 48 hours. Then, expression of CD83 (A) and CD86 (B) in DC was assessed by flow cytometry analysis. Both the percentage of positive DC (right panels) and the MFI (left panels) are shown. (C) Representative flow cytometry histograms of FITC-conjugated dextran endocytosis by DC with or without LPS (left panel) and by DC co-cultured with HMBPP-activated control (Ctrl, medium panel) and IL-21-cultured (IL-21, right panel) Vγ9Vδ2 T cells for 48h. Each analysis was repeated twice and performed each time with cells from different donors. (D) Percentages of IL-12+ DC following 18h of co-culture with control (Ctrl) and IL-21-amplified (IL-21) Vγ9Vδ2 T cells activated or not with HMBPP. (E) Analysis of T cell proliferation induced by DC loaded or not with CMV and EBV (peptides) after co-culture with control (Ctrl) or IL-21-amplified (IL-21) Vγ9Vδ2 T cells for 18h. Proliferation was assessed at day 6. This experiment was repeated three times and performed each time with cells from different donors. *p<0.05, **p<0.01 and ***p<0.001 (paired Wilcoxon test).
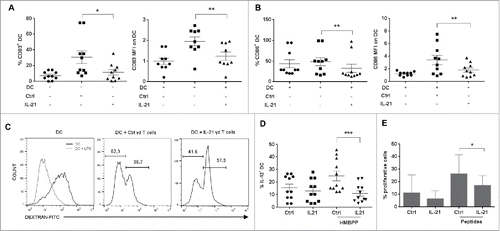
To investigate whether the DC maturation status correlated with a modification of DC functional properties, we assessed DC antigen-uptake activity (by analyzing the endocytosis of FITC-conjugated dextran) and IL-12 production. As control, we first showed that immature DC (high MFI peak), but not LPS-treated mature DC (low MFI peak, , left panel) could endocytose dextran. When co-cultured with activated control Vγ9Vδ2 T cells, about 70% of DC lost this capacity, suggesting their maturation (, middle panel). In contrast, when co-cultured with IL-21-amplified Vγ9Vδ2 T cells, most DC could still uptake dextran (about 60% high dextran peak). Furthermore, only 10% of DC co-cultured with IL-21-cultured Vγ9Vδ2 T cells expressed IL-12, compared with 25–30% of DC co-cultured with control Vγ9Vδ2 T cells ().
DC maturation is associated with an increase of their antigen presentation capacity and the induction of naive T cell proliferation. Therefore, we pre-incubated DC with control or IL-21-cultured Vγ9Vδ2 T cells for 18 h, and then loaded them with a pool of CMV and EBV peptides before adding CFSE-stained autologous T cells. After 6 days, analysis of the CFSE-staining of T cells by flow cytometry showed that the percentage of proliferating T cells was significantly higher when peptide-loaded DC were incubated with control than with IL-21-cultured Vγ9Vδ2 T cells (). Importantly, control experiments indicated that while LPS-maturated DC loaded with peptides induced T cell proliferation, both control and IL-21-cultured Vγ9Vδ2 T cells did not (data not shown).
These results demonstrate that DC maturation and cytokine production as well as DC-mediated T cell proliferation are impaired by IL-21-cultured Vγ9Vδ2 T cells.
CD73+ Vγ9Vδ2 T cells display regulatory activities
As all the previous results were obtained using bulk IL-21-cultured Vγ9Vδ2 T cells, we wanted to know whether the entire population or a specific subpopulation displayed such regulatory functions. As only a small portion of Vγ9Vδ2 T cells co-expressed CD39 and CD73 (), we focused our investigation on FACS-sorted CD73+ Vγ9Vδ2 T cells. As expected, the CD73+ subpopulation generated more adenosine than CD73− cells or the bulk population (). Moreover, the CD73+ subpopulation constitutively expressed higher amounts of IL-10 mRNA () and secreted more IL-10 than CD73− Vγ9Vδ2 T cells (). IL-10 secretion by CD73+ cells was further increased following HMBPP stimulation, while it remained very low in activated CD73− Vγ9Vδ2 T cells. Conversely, CD73+ Vγ9Vδ2 T cells were less efficient as cytotoxic effectors. Indeed, fewer CD73+ cells were IFN-γ+ and TNF-α+ () and they displayed lower cytotoxic activity against Daudi lymphoma cells, as revealed by their lower CD107 a expression ( and ), compared with CD73− cells.
Figure 5. Characterization of CD73+ Vγ9Vδ2 T cells. (A) Adenosine production by control (Ctrl), bulk IL-21-cultured (IL-21), CD73− IL-21-cultured (CD73-) and CD73+ (CD73+) IL-21-cultured Vγ9Vδ2 T cells was measured by MALDI-TOF mass spectrometry. Representative results of one of four experiments performed each time with cells from different donors. (B) Relative quantification of IL-10 mRNA in Ctrl, CD73− and CD73+ Vγ9Vδ2 T cells. This experiment was performed twice, each time with cells from different donors. (C) IL-10 production by Ctrl, CD73−, CD73+ Vγ9Vδ2 T cells, stimulated or not with HMBPP. This experiment was performed twice, each time with cells from different donors. (D) Graphs show the percentage of IFN-γ+ (left panel) and TNF-α+ (right panel) cells in the CD73− and CD73+ subpopulations of Il-21-cultured Vγ9Vδ2 T cells. (E) Representative flow cytometry histograms showing CD107a membrane expression in CD73− and CD73+ IL-21-cultured Vγ9Vδ2 T cells after HMBPP stimulation or co-cultured with Daudi cells for 6h. (F) The graph show the geometric mean of CD107a membrane expression in non-activated (N/A) CD73− and CD73+ IL-21-cultured Vγ9Vδ2 T cells and after HMBPP stimulation or co-cultured with Daudi cells for 6h. This experiment was performed six times, each time with cells from different donors; *p<0.05, **p<0.01 and ***p<0.001 (paired Wilcoxon test).
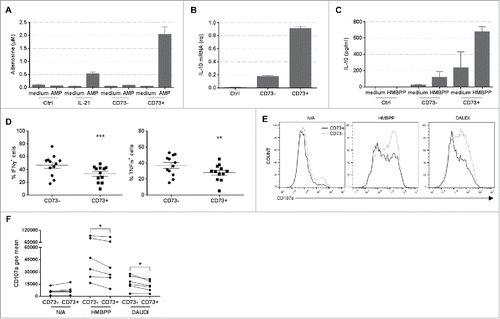
These results suggest that IL-21 favors the development of a Vγ9Vδ2 T cell subset that expresses CD73 and displays regulatory functions.
Evidence of a CD73+ γδ T cell population with a regulatory phenotype in tumor-bearing mice
We then asked whether mouse and human γδ T cells respond in a similar fashion to IL-21. We thus studied in vitro the impact of IL-21 on splenic γδ T cells from C57BL6 mice. We analyzed CD73 expression after concanavalin A (Con A) activation in the presence of IL-2 or IL-2 + IL-21. Both percentages and expression levels (geometric mean) of CD73 were significantly increased in splenic γδ T cells cultured in the presence of IL-21 than in the presence of IL-2 alone (supplementary Fig S4).
Then, to assess the in vivo relevance of our findings obtained in human γδ T cells, we used a syngeneic cancer tumor model in which C57BL6 mice were grafted with mouse TC1 cells. In this tumor model, immune infiltrate (CD45+ cells) in 3 weeks-grafted tumors represented 15–25% of total tumor cells, CD3+ cells representing about 20% of CD45+ cells and γδ T cells about 10% of CD3+ cells. Comparison of the expression of CD73, IFN-γ, and IL-10 in tumor-infiltrating γδ T cells and in splenic γδ T cells isolated from non-grafted (naive) and tumor-bearing mice showed that ≈70% of splenic γδ T cells expressed CD73 in both naive and grafted mice. This percentage was significantly higher for tumor-infiltrating γδ T cells (up to 95% of CD73+ γδ T cells in some tumors) (). We could detect IL-21 mRNA expression in the spleen and tumors of grafted mice, but not in the spleen of naive mice. Moreover, IL-21 mRNA level was higher in tumors than in spleens (). Analysis of IL-10 expression in splenic and tumor-infiltrating γδ and CD4+ T cells showed that upon activation with PMA and ionomycin (PMA), the percentage of IL-10+ γδ T cells was higher in spleen cells from tumor-bearing than from naive mice and this percentage was significantly increased in the tumors (). Conversely, the percentage of IL-10+ CD4+ T cells was highest in control spleens than in spleens and tumors from grafted mice (). Finally, we found that the tumor to spleen ratio for CD73 expression was equal to 1 for IFN-γ+ γδ T cells and significantly higher for IL-10+ γδ T cells, indicating that CD73 expression was higher in tumor-infiltrating IL-10+ γδ T cells. In comparison, in CD4+ T cells the ratio was 1 for both IFN-γ+ and IL-10+ cells. Overall, these data suggest that tumor development may promote the expansion in spleen and tumors of a regulatory subpopulation of γδ T cells that strongly expresses CD73 and produces IL-10.
Figure 6. Analysis of γδ T cell populations in naive or TC1-grafted mice. (A) Percentage of CD73+ γδ T cells from the spleen of naive and from the spleen and tumor of TC1-grafted C57BL6 mice. (B) Relative quantification of IL-21 mRNA in total cells from the spleen of naive mice and from the spleen and tumor of TC1-grafted C57BL6 mice. Results were normalized to CD45 mRNA level. (C) Percentage of IL-10+ γδ T cells and (D) of IL-10+ CD4+ T cells from the spleen of naive and TC1-grafted C57BL6 mice and from TC1 tumors, after cell activation with PMA and ionomycin. (E) CD73 expression (Geo mean) by IFN-γ- and IL-10-producing γδ T cells and CD4+ T cells calculated as the ratio between the expression in tumor-infiltrating cells and in splenocytes of TC1-grafted mice. *p<0.05, **p<0.01 and ***p<0.001 (paired Wilcoxon test).
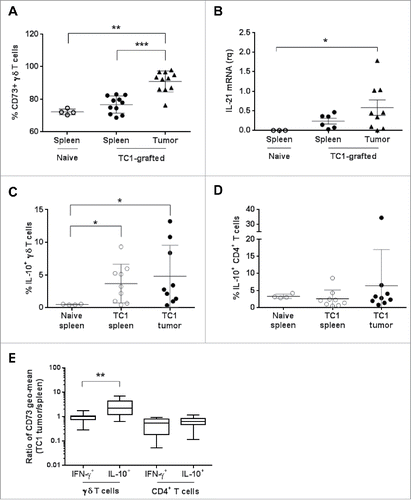
Discussion
In this study, we assessed the role of IL-21 in Vγ9Vδ2 T cell polarization (i.e. effector versus regulatory functions) and found that it promotes Vγ9Vδ2 T cell regulatory functions by favoring the development of an immunosuppressive CD73+ subpopulation. Specifically, we demonstrated that, when cultured in the presence of IL-21, Vγ9Vδ2 T cells express CD73 (a negative regulator of the immune response) and display regulatory functions that affect the proliferative capacity of other immune cells, such as T cells, through the CD73/adenosine pathway. Moreover, IL-21-cultured Vγ9Vδ2 T cells secrete IL-10 and IL-8 cytokines, and induce DC maturation and DC-mediated T cell proliferation less efficiently than control Vγ9Vδ2 T cells (no IL-21). Finally, data obtained in a syngeneic tumor model suggest that tumor development could promote the expansion of a regulatory subpopulation of γδ T cells that strongly express CD73 and produce IL-10.
The CD39/CD73/adenosine pathway is a crucial actor of tumor development and tumor escape to immune surveillance.Citation38,39 Recently, Gruenbacher et al. demonstrated that CD39, but not CD73, is expressed by Vγ9Vδ2 T cells following TCR activation.Citation34 Here, we found that IL-21 does not modify CD39 expression. However, in the presence of IL-21 we observed that a subset of Vγ9Vδ2 T cells also expresses CD73 and generates adenosine. These data bring a molecular rationale to the recent work of Hu and colleagues who reported an important infiltration of CD39+ γδ T lymphocytes in human colorectal cancer tissues and highlighted their regulatory action through their capacity to produce adenosine.Citation40 Indeed, high quantities of IL-21 are found in the microenvironment of colorectal cancers and they could influence CD73 expression by γδ T cells.
We also demonstrated that CD73-positive Vγ9Vδ2 T cells produce IL-10 and secrete less pro-inflammatory cytokines, such as IFN-γ and TNF-α, than CD73-negative cells, suggesting that CD73 could be a marker of a regulatory Vγ9Vδ2 T cell subpopulation. We did not detect the expression of other main suppressive molecules, such as TGF-β or IDO (data not shown), or of the regulatory T cell-associated FOXP3 gene in IL-21-cultured Vγ9Vδ2 T cells (data not shown). While IL-10 is known as one of the most potent immunosuppressive cytokines, several studies, in both mouse and human cancer models, reported that γδ T cells display pro-tumor activity in an IL-17A-dependent manner. For example, in a hepatocellular carcinoma mouse model, γδ T cells through IL-17A production are involved in the recruitment of myeloid-derived suppressor cells (MDSC) to the tumor site in a CXCL5/CXCR2–dependent pathway.Citation16 In another murine model, IL-17A-producing Vγ6+ γδ T cells promote ovarian cancer growth via mobilization of small peritoneal pro-tumor macrophages.Citation17 In our experimental conditions, we never detected IL-17A mRNA or protein in IL-21-cultured Vγ9Vδ2 T cells. Interestingly, in human colorectal cancer, γδ T cells are the main source of IL-17A, but also secrete other cytokines, including IL-8 that attracts polymorphonuclear myeloid-derived suppressor cells (PMN-MDSCs) in the tumor and sustains local immunosuppression.Citation18 Other recent data reported a pro-tumor function for IL-8 through promotion of MDSC recruitment and induction of epithelial-mesenchymal transition.Citation18 Based on our finding that IL-21-cultured Vγ9Vδ2 T cells, but not control cells, secrete significant amounts of IL-8, we propose that besides adenosine and IL-10, IL-8 production could also be part of Vγ9Vδ2 T cell immunosuppressive arsenal that will favor tumor development.
It is likely that CD73 expression does not identify a strictly immunosuppressive Vγ9Vδ2 T cell subpopulation because a part of these cells still produce pro-inflammatory cytokines. Further studies, such as transcriptomic analysis of the CD73+ Vγ9Vδ2 T cell subpopulation, should determine more precisely the signature of these cells that could then be used for bioinformatics analysis of tumor microarrays.Citation41 Although IL-21 promotes the expansion of the CD73+ sub-population, it would also be interesting to determine whether IL-21 is required for the acquisition of regulatory features by the CD73+ Vγ9Vδ2 subpopulation or whether IL-21 promotes the expansion of a pre-existing suppressive sub-population. Another pending question is the degree of reversibility of this regulatory phenotype.
One of the major challenges associated with the characterization of the CD73+ subpopulation resides in assessing its existence in vivo as well as its relevance in pathologies, such as cancer. We used here a syngeneic mouse tumor model to analyze the phenotype of tumor-infiltrating lymphocytes. We demonstrated the existence of a γδ T cell subpopulation that expresses CD73 and produces IL-10 both in the spleen and the tumor of tumor-bearing mice. Moreover, in the tumor, CD73 expression was significantly higher in γδ T cells that produce IL-10 than in those that produce IFN-γ, and IL-21 mRNA was expressed in the tumors. Although we demonstrated that murine and human γδ T cells respond in a similar fashion to IL-21 in vitro (CD73 expression), results in tumor-bearing mice cannot be directly transposed to human γδ T cells, but they are encouraging and suggest that the tumor microenvironment could favor a suppressive CD73+ subpopulation among the tumor-infiltrating γδ T cells. But so far, we could not establish a formal link between IL-21, immunosuppressive functions of γδ T cells and their impact on tumor development and cancer progression. Further experiments with neutralizing anti-IL-21 antibodies might help to establish the role of IL-21 on tumor infiltrating γδ T cells, however we have to keep in mind the pleiotropic action of this cytokine which could promote regulatory γδ T cells as demonstrated here, but could also promote anti-tumor effects through its ability to induce or expand cytotoxic cells such as CD8 T cells and NK cells.Citation21
Overall, we demonstrated that long-term exposure of Vγ9Vδ2 T cells to IL-21 promotes their regulatory properties and suppressive capacities, both directly through production of adenosine and IL-10 by the CD73+ subset, and indirectly through their effect on DC. Our study highlights new molecular mechanisms associated with Vγ9Vδ2 T cell regulatory functions and bring evidence of their possible favorable effect on tumor development.
Material and methods
Antigens, antibodies and reagents
HMBPP ((E) -4 hydroxy -3 methyl –but -2 enyl –pyrophosphate) was a generous gift from J.L. Montero (Institut des Biomolecules Max Mousseron, CNRS UMR 5247, Montpellier, France). Rat anti-human IL-10 monoclonal antibody and its isotype control were purchased from Biolegend and all other anti-human and their isotypically matched control mouse antibodies were from BD Biosciences (San Jose, CA, USA): anti-CD1a, anti-CD3, anti-CD27, anti-CD39, anti-CD45RA, anti-CD73, anti-CD83, anti-CD86, anti-CD107a, anti-IFN-γ, anti-IL-12, anti-TNF-α and anti-γ9 TCR. Recombinant human GM-CSF and IL-4 from ImmunoTools (Friesoyte, Germany) were used at a concentration of 15 ng/ml and 10 ng/ml respectively. Recombinant human IL-2 (rhIL-2) was from Novartis Pharma (Rueil-Malmaison, France) and recombinant IL-21 (rhIL-21) was from Miltenyi Biotec (Paris, France).
Cell culture
PBMC were obtained by density centrifugation on Ficoll-Paque (Eurobio, Les Ulis, France) from the blood of healthy donors provided by the Etablissement Français du Sang (EFS). CD14+ monocytes were purified from PBMC by magnetic positive separation using magnetic beads (Miltenyi Biotec). Total γδ T cells were isolated from the remaining cells using pan γδ T cell magnetic beads according to the manufacturer's instructions (Miltenyi Biotec). Purified γδ T cells were stimulated with 10 nM HMBPP, resulting in the specific amplification of Vγ9Vδ2 T cells. They were expanded in RPMI 1640/Glutamax medium supplemented with 5% human AB serum and 5% fetal calf serum (FCS) in the presence of IL-2 (20 ng/ml), IL-2 + IL-21 (25 ng/ml) or IL-2 + IL-6 (20 ng/ml) at 37°C in a 5% CO2 humidified atmosphere. γδ T cells were cultured for 3 weeks before phenotyping and functional analysis. Monocytes (0.7 × 106 cells/ml) were further differentiated into immature DC in complete culture medium (RPMI 1640/Glutamax) supplemented with 10% FCS in the presence of 15 ng/ml GM-CSF and 10 ng/ml IL-4 for 6 days. Fresh medium plus cytokines were added every two days and cell cultures contained >90% of immature (CD1a+/CD14-) DC as assessed by flow cytometry analysis at day 6. Raji and Daudi cells were cultured in RPMI 1640/Glutamax medium supplemented with 10% FCS at 37°C in a 5% CO2 humidified atmosphere. TC1 cells were cultured in RPMI 1640/Glutamax medium supplemented with 10% FCS, 1 mM pyruvate, 0.1 mM minimal essential medium with nonessential amino acids at 37°C in a 5% CO2 humidified atmosphere.
Flow cytometry analysis
Cells were incubated with antibodies at 4°C in PBS/2% FCS for 30 min, washed and fixed in 1% PFA, or fixed and permeabilized at 4°C with BD FixPerm Kit for 30 min (BD Biosciences), and then incubated with antibodies at 4°C for 30 min for intracellular staining. Golgi PLUG (BD Biosciences) was used for the detection of IFN-γ and TNF-α and Golgi STOP for the detection of IL-8, IL-10, and IL-12. Data were acquired with a Cytoflex cytometer (Beckman-Coulter) and analyzed with the FlowJo software.
Total cell extract preparation and western blot analysis
γδ T cells were cytokine-starved for 24 h and stimulated with rhIL-21. At various time points, cells were lysed and proteins extracted with the NuPage Kit (Life Technologies), according to the manufacturer's instructions. Proteins were separated on SDS-PAGE gradient gels (4–12%), transferred to nitrocellulose membranes (Millipore), and detected with the anti-phosphorylated STAT3 (1:1000) and anti-STAT3 (1:1000) antibodies (Cell Signaling Technology). A horseradish peroxidase-conjugated anti-rabbit antibody (1:2000) was used for detection and immunoreactive bands were visualized with the chemiluminescence western blotting and PXI image analysis system (Syngene).
ELISA
2.106 γδ T cells/ml were grown in fresh medium without cytokines in the presence or not of 10 nM HMBPP for 6 h and then supernatants were collected. IFN-γ, TNF-α, IL-8 and IL-10 were assessed using the BD Biosciences Opteia Kits. The mean values of duplicate samples from the same experiment are shown for each data point with their standard error of the mean (SEM).
RNA isolation and quantitative RT-PCR
γδ T cells (2.106 cells/ml) were stimulated or not with HMBPP for 6 h. RNA was extracted from cell pellets using the RNA Extraction Kit from Qiagen. The quality and concentration of RNA samples were assessed using a Nanodrop device (Thermofisher). After DNase inactivation, RNA was reverse transcribed (RT) using qScript XLT cDNA Super Mix (Quanta Biosciences), according to the manufacturer's instructions. Quantification by real-time polymerase chain reaction (PCR) was carried out with the LightCycler 480 II System (Roche Diagnostics, Basel, Switzerland) using the SYBR Premix Ex Taq (Tli RNaseH Plus) Kit (Ozyme, Saint-Quentin-en-Yvelines, France). The forward and reverse primers used for the PCR reaction were as follow: GUS-forward 5′-GATTGCCAATGAAACCAGGTATCC-3′, GUS-reverse 5′-ACACGCAGGTGGTATCAGTCTTG-3′, IL-8-forward 5′- ACTGAGAGTGATTGAGAGTGGAC-3′, IL-8-reverse 5′- AACCCTCTGCACCCAGTTTTC-3′, IL-10-forward 5′-ACAAGAGCAAGGCCGTGGAG-3′, IL-10-reverse 5′-GTCGCCACCCTGATGTCTCA-3′, INF-g-forward 5′-TCATCCAAGTGATGGCTGAA-3′, INF-g-reverse 5′-ATATTGCAGGCAGGACAACC-3′, TNF-a-forward 5′-AGCCCATGTTGTAGCAAACC-3′, TNF-a-reverse 5′-TGAGGTACAGGCCCTCTGAT-3′, CD39-forward 5′-GACTCTTCCATTCCAGCAGTTTGAAATCC-3′, CD39-reverse 5′- TGATGGCATTGTTGATAGTTTCCAATACCC-3′ and CD73-forward 5′-GACTGATCGAGCCACTCCTC-3′, CD73-reverse 5′-ACTGAGAGTGATTGAGAGTGGAC-3′.
Adenosine measurement by Maldi TOF spectrometry
γδ T cells (2 × 106 cells/mL) were washed in cold PBS and resuspended in PBS supplemented with 50 μM AMP at 4°C for 30 min. After centrifugation, adenosine levels in cell supernatants were analyzed by MALDI-TOF mass spectrometry, as previously described by Bastid et al.Citation42
T cell proliferation
αβ T cells (1 × 107 cells/mL) were incubated with 0.75 μmol/L CFSE (Molecular Probes) at 37°C for 12min. For experiments with supernatants, γδ T cells (2 × 107 cells/mL) were washed and resuspended in complete medium supplemented or not with 50 μM AMP at 4°C for 30 min. After centrifugation, γδ T cell supernatants and CFSE-labeled αβ T cells were added to flat-bottom plates in the presence of 10 μg/mL immobilized anti-CD3 antibody (clone UCHT1). Proliferation of αβ T cells was analyzed by flow cytometry at day 4. For antigen presentation experiments, monocyte-derived DC were co-cultured with γδ T cells (ratio 1:1) for 18 hours, and then loaded with a peptide cocktail of 0.3 nM EBV and 0.3 nM CMV (Miltenyi). CFSE-labeled autologous αβ T cells (1 × 106 cells/mL) were then added to the culture. Proliferation of αβ T cells was analyzed by flow cytometry at day 6.
In vivo tumor-infiltrating lymphocyte phenotyping
C57BL6 mice were grafted subcutaneously with 0.5 × 106 TC1 cells. After three weeks, tumor and spleen were isolated and dissociated to obtain a single-cell suspension. Cells were stimulated or not with 20 ng/ml PMA and 1 µg/ml ionomycin in RPMI/10% FCS in the presence of Golgi PLUG and Golgi STOP for 6 hours. Then, cells were incubated with anti-CD45, -CD3, -CD4 and -γδ TCR antibodies (extracellular staining) before fixation and permeabilization with the BD FixPerm Kit and intracellular staining for IFN-γ and IL-10. Data were acquired on a Cytoflex cytometer (Beckman Coulter) and results were analyzed using the FlowJo software.
Ethics statement
All procedures for animal handling and experiments were approved by the local animal facility “ComEth” Institutional Review Board under the supervision of the French LR Regional CEEA ethics committee on animal experimentation (Chairman: Pr M Michel, Montpellier).
Statistical analysis
Results were compared using the paired Wilcoxon test. A P value <0.05 was considered as statistically significant. Analyses of statistical significance were performed using the Prism software (GraphPad Software).
Grant support and acknowledgements
This work was supported by the Institute National de la Santé et de la Recherche Médicale (INSERM); Université Montpellier; the Institut Régional du Cancer de Montpellier (ICM), the Institut National du Cancer (INCa, # R14146NN) and the Ligue contre le Cancer. We acknowledge all the members of NB's team for their stimulating comments.
References
- O'Brien RL, Roark CL, Born WK. IL-17-producing gammadelta T cells. Eur J Immunol. 2009;39:662-6. doi:10.1002/eji.200839120. PMID:19283718
- Bonneville M, O'Brien RL, Born WK. Gammadelta T cell effector functions: a blend of innate programming and acquired plasticity. Nat Rev Immunol. 2010;10:467-78. doi:10.1038/nri2781. PMID:20539306
- Hayday AC. [gamma][delta] cells: a right time and a right place for a conserved third way of protection. Annual Rev Immunol. 2000;18:975-1026. doi:10.1146/annurev.immunol.18.1.975.
- Hintz M, Reichenberg A, Altincicek B, Bahr U, Gschwind RM, Kollas AK, Beck E, Wiesner J, Eberl M, Jomaa H. Identification of (E)-4-hydroxy-3-methyl-but-2-enyl pyrophosphate as a major activator for human gammadelta T cells in Escherichia coli. FEBS Letters. 2001;509:317-22. doi:10.1016/S0014-5793(01)03191-X. PMID:11741609
- Altincicek B, Moll J, Campos N, Foerster G, Beck E, Hoeffler JF, Grosdemange-Billiard C, Rodríguez-Concepción M, Rohmer M, Boronat A, et al. Cutting edge: human gamma delta T cells are activated by intermediates of the 2-C-methyl-D-erythritol 4-phosphate pathway of isoprenoid biosynthesis. J Immunol. 2001;166:3655-8. doi:10.4049/jimmunol.166.6.3655. PMID:11238603
- Gober HJ, Kistowska M, Angman L, Jeno P, Mori L, De Libero G. Human T cell receptor gammadelta cells recognize endogenous mevalonate metabolites in tumor cells. J Exp Med. 2003;197:163-8. doi:10.1084/jem.20021500. PMID:12538656
- Bonneville M, Scotet E. Human Vgamma9Vdelta2 T cells: promising new leads for immunotherapy of infections and tumors. Curr Opinion Immunol. 2006;18:539-46. doi:10.1016/j.coi.2006.07.002.
- Riganti C, Massaia M, Davey MS, Eberl M. Human gammadelta T-cell responses in infection and immunotherapy: common mechanisms, common mediators? Eur J Immunol. 2012;42:1668-76. doi:10.1002/eji.201242492. PMID:22806069
- Catros V, Toutirais O, Bouet F, Cabillic F, Desille M, Fournie JJ. Tgammadelta lymphocytes in oncology: unconventional killer lymphocytes. Med Sci. 2010;26:185-91.
- Buccheri S, Guggino G, Caccamo N, Li Donni P, Dieli F. Efficacy and safety of gammadeltaT cell-based tumor immunotherapy: a meta-analysis. J Biol Regulators Homeostatic Agents. 2014;28:81-90.
- Gomes AQ, Martins DS, Silva-Santos B. Targeting gammadelta T lymphocytes for cancer immunotherapy: from novel mechanistic insight to clinical application. Cancer Res. 2010;70:10024-7. doi:10.1158/0008-5472.CAN-10-3236. PMID:21159627
- Dieli F, Vermijlen D, Fulfaro F, Caccamo N, Meraviglia S, Cicero G, Roberts A, Buccheri S, D'Asaro M, Gebbia N, et al. Targeting human {gamma}delta} T cells with zoledronate and interleukin-2 for immunotherapy of hormone-refractory prostate cancer. Cancer Res. 2007;67:7450-7. doi:10.1158/0008-5472.CAN-07-0199. PMID:17671215
- Meraviglia S, Eberl M, Vermijlen D, Todaro M, Buccheri S, Cicero G, La Mendola C, Guggino G, D'Asaro M, Orlando V, et al. In vivo manipulation of Vgamma9Vdelta2 T cells with zoledronate and low-dose interleukin-2 for immunotherapy of advanced breast cancer patients. Clin Exp Immunol. 2010;161:290-7. PMID:20491785
- Wilhelm M, Kunzmann V, Eckstein S, Reimer P, Weissinger F, Ruediger T, Tony HP. Gammadelta T cells for immune therapy of patients with lymphoid malignancies. Blood. 2003;102:200-6. doi:10.1182/blood-2002-12-3665. PMID:12623838
- Coffelt SB, Kersten K, Doornebal CW, Weiden J, Vrijland K, Hau CS, Verstegen NJM, Ciampricotti M, Hawinkels LJAC, Jonkers J, et al. IL-17-producing gammadelta T cells and neutrophils conspire to promote breast cancer metastasis. Nature. 2015;522:345-8. doi:10.1038/nature14282. PMID:25822788
- Ma S, Cheng Q, Cai Y, Gong H, Wu Y, Yu X, Shi L, Wu D, Dong C, Liu H. IL-17A produced by gammadelta T cells promotes tumor growth in hepatocellular carcinoma. Cancer Res. 2014;74:1969-82. doi:10.1158/0008-5472.CAN-13-2534. PMID:24525743
- Rei M, Goncalves-Sousa N, Lanca T, Thompson RG, Mensurado S, Balkwill FR, Kulbe H, Pennington DJ, Silva-Santos B. Murine CD27(-) Vgamma6(+) gammadelta T cells producing IL-17A promote ovarian cancer growth via mobilization of protumor small peritoneal macrophages. Proc Natl Acad Sci USA. 2014;111:E3562-70. doi:10.1073/pnas.1403424111. PMID:25114209
- Wu P, Wu D, Ni C, Ye J, Chen W, Hu G, Wang Z, Wang C, Zhang Z, Xia W, et al. gammadeltaT17 cells promote the accumulation and expansion of myeloid-derived suppressor cells in human colorectal cancer. Immunity. 2014;40:785-800. doi:10.1016/j.immuni.2014.03.013. PMID:24816404
- Peng G, Wang HY, Peng W, Kiniwa Y, Seo KH, Wang RF. Tumor-infiltrating gammadelta T cells suppress T and dendritic cell function via mechanisms controlled by a unique toll-like receptor signaling pathway. Immunity. 2007;27:334-48. doi:10.1016/j.immuni.2007.05.020. PMID:17656116
- Daley D, Zambirinis CP, Seifert L, Akkad N, Mohan N, Werba G, Barilla R, Torres-Hernandez A, Hundeyin M, Mani VR, et al. gammadelta T Cells support pancreatic oncogenesis by restraining alphabeta T cell activation. Cell. 2016;166:1485-99 e15. doi:10.1016/j.cell.2016.07.046.
- Croce M, Rigo V, Ferrini S. IL-21: a pleiotropic cytokine with potential applications in oncology. J Immunol Res. 2015;2015:696578. doi:10.1155/2015/696578. PMID:25961061
- Spolski R, Leonard WJ. Interleukin-21: basic biology and implications for cancer and autoimmunity. Annual Rev Immunol. 2008;26:57-79. doi:10.1146/annurev.immunol.26.021607.090316.
- Leonard WJ, Wan CK. IL-21 signaling in immunity. F1000Research 2016; 5:224–234. doi:10.12688/f1000research.7634.1. PMID:26966515
- Parrish-Novak J, Dillon SR, Nelson A, Hammond A, Sprecher C, Gross JA, Johnston J, Madden K, Xu W, West J, et al. Interleukin 21 and its receptor are involved in NK cell expansion and regulation of lymphocyte function. Nature. 2000;408:57-63. doi:10.1038/35040504. PMID:11081504
- Zeng R, Spolski R, Finkelstein SE, Oh S, Kovanen PE, Hinrichs CS, Pise-Masison CA, Radonovich MF, Brady JN, Restifo NP, et al. Synergy of IL-21 and IL-15 in regulating CD8+ T cell expansion and function. J Exp Med. 2005;201:139-48. doi:10.1084/jem.20041057. PMID:15630141
- Coquet JM, Kyparissoudis K, Pellicci DG, Besra G, Berzins SP, Smyth MJ, Godfrey DI. IL-21 is produced by NKT cells and modulates NKT cell activation and cytokine production. J Immunol. 2007;178:2827-34. doi:10.4049/jimmunol.178.5.2827. PMID:17312126
- Fantini MC, Rizzo A, Fina D, Caruso R, Becker C, Neurath MF, Macdonald TT, Pallone F, Monteleone G. IL-21 regulates experimental colitis by modulating the balance between Treg and Th17 cells. European J Immunol. 2007;37:3155-63. doi:10.1002/eji.200737766.
- Lindner S, Dahlke K, Sontheimer K, Hagn M, Kaltenmeier C, Barth TF, Beyer T, Reister F, Fabricius D, Lotfi R, et al. Interleukin 21-induced granzyme B-expressing B cells infiltrate tumors and regulate T cells. Cancer Res. 2013;73:2468-79. doi:10.1158/0008-5472.CAN-12-3450. PMID:23384943
- Ma J, Ma D, Ji C. The role of IL-21 in hematological malignancies. Cytokine. 2011;56:133-9. doi:10.1016/j.cyto.2011.07.011. PMID:21824785
- Thedrez A, Harly C, Morice A, Salot S, Bonneville M, Scotet E. IL-21-mediated potentiation of antitumor cytolytic and proinflammatory responses of human V gamma 9 V delta 2 T cells for adoptive immunotherapy. J Immunol. 2009;182:3423-31. doi:10.4049/jimmunol.0803068. PMID:19265120
- Eberl M, Engel R, Beck E, Jomaa H. Differentiation of human gamma-delta T cells towards distinct memory phenotypes. Cell Immunol. 2002;218:1-6. doi:10.1016/S0008-8749(02)00519-1. PMID:12470608
- Caccamo N, Todaro M, La Manna MP, Sireci G, Stassi G, Dieli F. IL-21 regulates the differentiation of a human gammadelta T cell subset equipped with B cell helper activity. PLoS One. 2012;7:e41940. doi:10.1371/journal.pone.0041940. PMID:22848667
- Bansal RR, Mackay CR, Moser B, Eberl M. IL-21 enhances the potential of human gammadelta T cells to provide B-cell help. European J Immunol. 2012;42:110-9. doi:10.1002/eji.201142017.
- Gruenbacher G, Gander H, Rahm A, Idzko M, Nussbaumer O, Thurnher M. Ecto-ATPase CD39 inactivates Isoprenoid-Derived Vgamma9Vdelta2 T cell phosphoantigens. Cell Reports. 2016;16:444-56. doi:10.1016/j.celrep.2016.06.009. PMID:27346340
- Chalmin F, Mignot G, Bruchard M, Chevriaux A, Vegran F, Hichami A, Ladoire S, Derangère V, Vincent J, Masson D, et al. Stat3 and Gfi-1 transcription factors control Th17 cell immunosuppressive activity via the regulation of ectonucleotidase expression. Immunity. 2012;36:362-73. doi:10.1016/j.immuni.2011.12.019. PMID:22406269
- Ismaili J, Olislagers V, Poupot R, Fournie JJ, Goldman M. Human gamma delta T cells induce dendritic cell maturation. Clin Immunol. 2002;103:296-302. doi:10.1006/clim.2002.5218. PMID:12173304
- Ni M, Martire D, Scotet E, Bonneville M, Sanchez F, Lafont V. Full restoration of Brucella-infected dendritic cell functionality through Vgamma9Vdelta2 T helper type 1 crosstalk. PLoS One. 2012;7:e43613. doi:10.1371/journal.pone.0043613. PMID:22928003
- Bastid J, Cottalorda-Regairaz A, Alberici G, Bonnefoy N, Eliaou JF, Bensussan A. ENTPD1/CD39 is a promising therapeutic target in oncology. Oncogene. 2013;32:1743-51. doi:10.1038/onc.2012.269. PMID:22751118
- Bonnefoy N, Bastid J, Alberici G, Bensussan A, Eliaou JF. CD39: A complementary target to immune checkpoints to counteract tumor-mediated immunosuppression. Oncoimmunology. 2015;4:e1003015. doi:10.1080/2162402X.2014.1003015. PMID:26155397
- Hu G, Wu P, Cheng P, Zhang Z, Wang Z, Yu X, Shao X, Wu D, Ye J, Zhang T, et al. Tumor-infiltrating CD39+gammadeltaTregs are novel immunosuppressive T cells in human colorectal cancer. Oncoimmunology. 2017;6:e1277305. doi:10.1080/2162402X.2016.1277305. PMID:28344891
- Tosolini M, Pont F, Poupot M, Vergez F, Nicolau-Travers ML, Vermijlen D, Sarry JE, Dieli F, Fournié JJ. Assessment of tumor-infiltrating TCRVgamma9Vdelta2 gammadelta lymphocyte abundance by deconvolution of human cancers microarrays. Oncoimmunology. 2017;6:e1284723. doi:10.1080/2162402X.2017.1284723. PMID:28405516
- Bastid J, Regairaz A, Bonnefoy N, Dejou C, Giustiniani J, Laheurte C, Cochaud S, Laprevotte E, Funck-Brentano E, Hemon P, et al. Inhibition of CD39 enzymatic function at the surface of tumor cells alleviates their immunosuppressive activity. Cancer Immunol Res. 2015;3:254-65. doi:10.1158/2326-6066.CIR-14-0018. PMID:25403716