ABSTRACT
Acute myeloid leukemia (AML) is a heterogeneous disease whose therapies currently show elevated toxicity and a high rate of relapse. Recently, the burgeoning of new anti-tumor therapeutic strategies aimed at enhancing the immune response has pushed natural killer cells (NKs) into the spotlight. These cells are powerful warriors that can bring about the lysis of tumor cells through their cytotoxic ability. However, tumor cells have developed strategies to evade recognition mediated by NKs. Here, we review the mechanisms triggered by AML cells and discuss the emerging immunotherapeutic strategies that potentiate the anti-tumor functions of NKs.
1. Introduction
Acute myeloid leukemia (AML) is an aggressive malignancy of the bone marrow characterized by the uncontrolled proliferation of undifferentiated myeloid cells. It originates from a hematopoietic stem cell that acquires genetic and/or epigenetic mutations and is able to self-renew to maintain the disease.Citation1
Studies based on new sequencing technologies have made it possible to determine that the majority of AML cells originate from a founder clone with a driver mutation. A study of more than 1500 AML patients found that in the majority of them, the driver mutation was located in genes involved in epigenetic mechanisms (DNMT3A, ASXL1, IDH1/2, TET2).Citation2 Additionally, the founder clone can acquire subsequent mutations that generate subclones, each of which has different cellular morphologies and functionalities.Citation3 These mutations are located in signaling pathways (FLT3, KIT, K-RAS), myeloid transcription factors (CEBPA, RUNX1) or in tumor suppressor genes (TP53, WT1, PHF6).Citation4 The mutational pattern of each patient may be indicative of the prognosis of their disease. In this way, it has been reported that AML patients in complete remission (CR) but with persistent driver mutations have a higher incidence of relapse and poorer survival.Citation5
AML has usually been classified by the French–American–British (FAB) group into different subtypes (M0-M7) depending on the type of cell that proliferates and its state of maturation. However, this classification has no prognostic value and the World Health Organization (WHO) established a new classification in 2008 based on cytogenetic analysis that is periodically revised.Citation6
Therapeutic options for treating AML are still very limited and have changed very little in the last 30 years. The induction phase, known as “7 + 3”, based on an intensive chemotherapy regimen using standard doses of cytarabine for 7 days followed by anthracycline for 3 days. This therapy achieves CR from the disease in 60–80% of patients (age < 60 years) and in 40–60% of elderly patients (age ≥ 60 years).Citation7 After CR, it is necessary to continue with an appropriate consolidation phase to eradicate the minimal residual disease and to avoid possible relapses. This treatment is based mainly on a high-dose of cytarabine followed by autologous or allogenic hematopoietic cell transplantation (HCT). Patients unsuitable for intensive therapy, especially elderly patients, receive treatments with a low-dose of cytarabine or therapy with hypomethylating agents such as decitabine (Dacogen®, DAC). Although these therapeutic strategies have improved survival rates of younger AML patients in recent years, they are still unsatisfactory in adverse-risk and most older patients.Citation8 As a consequence, current research is directed towards identifying new therapeutic strategies that improve patients’survival and quality of life. In recent years, the greatest successes achieved in AML have been those that aim to enhance the immune response to increase the recognition and elimination of AML cells.
2. Impaired recognition of AML by natural killer cells
One of the first studies to demonstrate the role of natural killer (NK) cells in the immunosurveillance of cancer revealed that individuals who have NK cells with a low cytotoxicity capacity had a higher probability of developing some type of cancer during an 11-year follow-up.Citation9 NK cells can recognize tumor cells independently of MHC, whereby inhibitory and activating receptors of NK cells bind to its ligands, which are expressed on the surface of tumor cells. Some of the first evidence that NK cells can kill AML cells was the absence of relapse of the disease after HCT transplantation containing alloreactive NKs.Citation10 However, during cancer development, NK and AML cells can develop strategies to avoid recognition and allow the tumor to propagate.
2.1. Repertoire of NK cell receptors and their ligands
Conventional NK cells are key lymphocytes of the innate immune system, currently considered a prototype of the innate lymphoid cell (ILC) family. They exert anti-tumor effects mediated by their cytotoxic and cytokine-secreting capacity independently of MHC-based antigen recognition. Human NK cells comprise two main phenotypes based on the CD56 and CD16 markers. The CD56dim CD16+ NK subset has great cytotoxic potential and is predominant in blood, whereas the CD56high CD16− subset produces abundant cytokines and is more abundant in lymph nodes. The functionality of NK cells is mediated and controlled by the balance between the expression of activating and inhibitory receptors.Citation11 The main activating receptors are natural cytotoxic receptors (NCR; NKp30, NKp46, NKp44), NKG2D and DNAM-1, whereas the killer-immunoglobulin-like receptor (KIR) family and NKG2A are inhibitory receptors. During NK cell maturation, the inhibitory receptors (mainly KIRs) interact with self-molecules (self-MHC class I) to avoid the recognition of self-cells in a process known as “licensing” or “education” of NK cells.Citation12 The unbalanced expression of these receptors and their ligands hinders the recognition of tumor cells and allows tumor progression.
In a high percentage of AML patients, the expression levels of some activating receptors (NKG2D, NKp30, NKp46, DNAM-1) are null or very low ().Citation13 The inverse correlation between the DNAM-1 expression levels and one of its ligands (CD112) in AML patients suggests that the expression of activating receptors depends on their ligands being present on the surface of AML cells.Citation14 Moreover, the status of AML patients also affects the repertory of NK cell receptors. Fauriat et al.Citation15 reported that the NKp30 and NKp46 expression is reduced in AML patients at diagnosis but is partially recovered after CR and decreases again in an episode of disease relapse. It is also clearly established that high levels of expression of NKp30 and NKp46 are associated with higher rates of overall, relapse-free and progression-free survival compared with patients with reduced expression of their receptors.Citation16,Citation17
Figure 1. Critical role of tumor microenvironment in NK-AML recognition. AML cells can create an immunosuppressive microenvironment that avoids recognition mediated by NK cells, triggering tumor immune escape. This tumor microenvironment results in decreased activity of NK cells by several mechanisms: a) Modulation of the NK receptor repertoire. NK functions are exhaustively regulated by the balance between activating and inhibitory receptors. However, during AML development the repertoire of NK cells is modified, reducing the level of expression of activating receptors (NKG2D, DNAM-1, NKp30) and increasing that of inhibitory receptors (KIRs and NKG2A). Additionally, ligands for NKG2D-activating receptor (NKG2DL) can be regulated by DNA methylation (red circles), reducing its level of expression on the cell surface of AML cells, or they can be released into the tumor environment in a soluble form that promotes the internalization and degradation of NKG2D; b) Defective NK maturation. NK cells of some AML patients feature defects in their maturation. AML patients show three different NK maturation profiles: hypomaturation (CD56bright/dim KIRs− CD57−), intermediate (CD56dim KIR−/+ CD57−/+) and hypermaturation (CD56dim KIRs+ CD57+). Elevated levels of miR-29b in AML patients reduce the cytotoxic ability of hypermature NK cells, indicating a poor level of recognition of tumor cells, and damage NK maturation, thereby increasing the number of hypomature NK cells; and c) Lysis inhibition by immune checkpoints. PD-1, TIM3 and TIGIT expressed on the cell surface of NK cells, recognize their ligands (PD-L1, Gal-9, or CD112/CD155 respectively), which are expressed on the cell surface of AML cells. As a consequence, activating pathways involved NK cell regulation (PI3K, ERK, PKCƟ) are inhibited, promoting NK cell anergy.
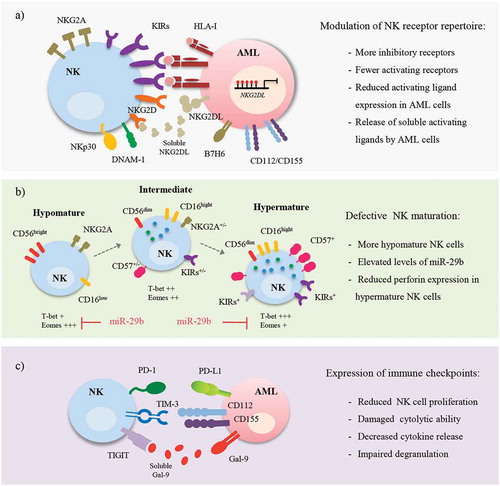
Expression of the ligands of the activating receptors is also modulated in AML (). Several research groups have shown that NKG2D ligands (NKG2DL; MICA/MICB and ULBPs1–3) are absent or expressed at very low levels on the cell surface of myeloid blasts.Citation18,Citation19 Expression of these ligands depends on the cellular subtype showing little or no expression in myeloblastic cells but a high level of expression in monoblastic cells, suggesting that NKG2DL are acquired during late stages of maturation.Citation20 An important strategy developed by AML cells to avoid immune recognition is the release of these ligands in a soluble form from the cell surface through cleavage by metalloproteases or into exosomes. Two studies have shown that these ligands are frequently detected in the serum of AML patients, promoting the downregulation of NKG2D and thereby the loss of cytotoxicity capacity of NKs.Citation21,Citation22 Therefore, AML patients with low levels of soluble MICA, MICB and ULBP1 are more likely to achieve CR and live more than one year.Citation21 In contrast, little is known about the expression of other activating ligands. DNAM-1 ligands (CD112, CD155) are expressed in a high percentage (around 85%) of AML patients.Citation14 However, B7H6, a ligand for NKp30, is expressed in a low number of AML blasts but is released into the serum of some patients (23.5%).Citation16 The chronic exposure to these ligands might induce the downregulation of their receptors, leading to the evasion of NK cell immunosurveillance and facilitating tumor progression. Further studies are needed to confirm this hypothesis and to determine whether high levels of these activating receptors could improve the immune recognition of AML blasts.
Altered expression of the inhibitory receptors has also been described. CD158b (KIR2DL2/L3) and NKG2A inhibitory receptors are upregulated in AML patients with respect to healthy donors, irrespective of cellular subtype, impairing the cytotoxic ability and cytokine production (IFN-γ, TNF-α) of NK cells.Citation13,Citation23
2.2. Defects in the maturation status of NK cells
NK cell maturation involves various stages of differentiation that are controlled by cytokines and transcription factors. An NK cell precursor originates from a common lymphoid progenitor, which is transformed into an immature NK cell that matures and acquires a migratory capacity and a repertoire of receptors that trigger its cytotoxic capability.Citation24 An accurate maturation process is necessary for NK cells to be able to recognize and eliminate cancer cells. However, the maturation status of those cells can be modulated during AML development. The analysis of the maturation profile of autologous NK from a murine model of AML, using specific murine maturation markers, showed that the immature phenotype (CD27+CD11b−) is upregulated, while the intermediate (CD27+CD11b+) is nearly absent, suggesting that the tumor microenvironment might modulate the maturation profile.Citation25
In humans, NK cell maturation is identified by the differential expression of the CD56, CD16, NKG2A, KIRs and CD57 markers.Citation26 CD56bright CD16low NK cells correspond to the most immature subset (). In CD56dim NK cells, different maturation stages are characterized by the loss of NKG2A and the sequential acquisition of CD57 and KIR receptors. In AML patients, three different groups of patients were defined according to their NK maturation profile: hypomaturation (CD56bright/dim KIRs− CD57−), intermediate (CD56dim KIR−/+ CD57−/+) and hypermaturation (CD56dim KIRs+ CD57+) ().Citation27 Patients with the hypomaturation profile (around 9%) showed a poor 3-year overall survival and relapse-free survival, suggesting an altered maturation in some patients with relevant clinical outcomes. Defects in the NK cell maturation from leukemic mice and AML patients are associated with elevated levels of miR-29b in those cells.Citation25 This microRNA inhibits the expression of the T-bet and EOMES transcription factors, which are essential for controlling the final stages of NK cell differentiation. This has a dual effect: reduced T-bet expression leads to low levels of perforin in mature NK cells, decreasing their cytolytic ability, and the damage to the development of intermediate NK cells that require expression of both transcription factors.
NK cell maturation also enables the generation of memory-like NK cells. The first time that it was observed that NKs could acquire immunological memory was in a murine model deficient in T and B cells, in which prior contact with a hapten produced hypersensibility.Citation28 Subsequent studies showed that pre-activated NK cells with cytokines (IL-12, IL-15 and IL-18) induce the differentiation of memory-like NK cells, characterized by enhanced IFN-γ production and cytotoxic ability against human AML blasts.Citation29,Citation30 The potent antileukemia effect was dependent on the increased expression of activating and cytokine receptors (NKG2D, NKp30, NKp44, CD25) and cytotoxic molecules, but regardless of the KIR-ligand interactions.
2.3. Expression of molecular immune checkpoint inhibitors
Immune checkpoint molecules are proteins that help maintain an adequate immune response, playing a key role in self-tolerance and the avoidance of indiscriminate lysis of self-cells. In recent years, targeting immune checkpoints with specific inhibitory antibodies has revolutionized the treatment of cancer by restoring the functionality of exhausted T cells against tumor cells. However, NK cell activity can also be regulated by immune checkpoints such as PD-1, TIM-3 or TIGIT.Citation31
PD-1 is expressed in mature (CD56dim CD16+) NK cells under sustained stimulation by MHC class I-deficient tumor cells or infected cells. PD-1+ NK cells have reduced proliferative and cytolytic abilities, and the impaired cytokine production typical of an exhausted phenotype. In fact, blockade of the PD-1/PDL-1 interaction with specific antibodies restores the functions of NK cells, promoting an anti-tumor response to several cancers, such as myeloma and digestive tumors.Citation32,Citation33 Although the role of PD-1 in NK cell function in AML is poorly understood, there is clear evidence that PD-L1 is expressed in some AML patients, where it impairs immune recognition ().Citation34 Accordingly, several clinical trials evaluating PD-1/PD-L1 blockade are under way (). Recently, high levels of DNA methylation of PD-L1 gene have been associated with reduced PD-L1 expression in some AML cells, and a consequently lower risk of relapse and prolonged overall survival in those patients.Citation35 Methylation of PD-L1 could be an effective biomarker for stratifying patients who could benefit from PD-1 checkpoint inhibition.
Table 1. Ongoing clinical trials using NK cells to enhance the innate immune response in AML.
TIM-3 is highly expressed on the surface of human NK cells and binds to its ligand Galectin-9 (Gal-9), which is expressed in AML blasts (). As a consequence of this interaction, TIM-3+ NK cells increase IFN-γ production, leading to increased expression of the indoleamine 2,3-dioxygenase 1 enzyme by AML cells, which, in turn, impairs NK cell degranulation activity and ensures leukemic immune escape.Citation36 Moreover, high levels of soluble Gal-9 were detected in the serum of AML patients, where Gal-9 is able to bind to TIM-3 expressed on the surface of leukemic stem cells that activates the NF-kB and β-catenin pathways involved in the self-renewal of these malignant stem cells.Citation37 Targeting this stimulatory loop could be an efficient target to avoid the development of human AML.
TIGIT is expressed in cytotoxic T cells and in NK cells, and binds to the same ligands as DNAM-1 (CD112 and CD155) (). In AML patients, a large number of dysfunctional CD8+ T cells with increased expression of TIGIT and PD-1 but low levels of DNAM-1 was reported, but TIGIT expression in the NK cells of these patients has not been characterized.Citation38 As previously described, AML patients show low levels of DNAM-1 expression, while its ligands are highly expressed.Citation14 This could favor the binding of TIGIT with CD112 and CD155 ligands, promoting inhibitory signaling and, thereby, tumor immune escape. Recently, it has been shown that blockade of TIGIT prevents NK cell exhaustion and promotes NK cell-mediated anti-tumor effects in several mouse models of cancer (colon, breast, melanoma and fibrosarcoma), but the therapeutic potential in AML patients needs further study.Citation39
Given these findings, the development of new immunotherapies aimed at restoring the functionality of NK cells and the recognition of AML cells is a promising strategy, and one with important clinical implications.
3. Targeting AML cells by NK cell-based immunotherapy
The development of immunotherapy, an approach that aims to fight cancer using the body’s own immune system, has revolutionized the treatment of cancer. Numerous lines of evidence, summarized in this review, support the use of NK cell-based immunotherapy as a strategy for better recognition of AML cells. We also review the promising strategies that are currently proposed in the context of AML.
3.1. Adoptive transfer of NK cells
3.1.1. Autologous NK cells
Infusing NK cells to treat AML was first proposed by the American National Cancer Institute more than thirty years ago.Citation40 This therapy is based on the administration of autologous NKs (1–2 x 107 cells/kg) previously activated with IL-2 or IFN-γ. Subsequently, IL-2 is administered in vivo to allow NK cells to proliferate in patients (). The advantage of this therapy over allo-HCT is that immune suppression and HLA-matching are not necessary. Nevertheless, it also has limitations due to the high toxicity arising from the sustained in vivo administration of IL-2, and to the absence of any clear clinical benefit.Citation41 The lack of improvement of immune recognition may be due to the interaction between KIR receptors expressed in autologous NKs and HLA-I molecules in AML cells.Citation42 This implies that modulation of the KIR/HLA-1 axis could enhance the clinical effect of autologous NKs transplant in AML.
Figure 2. Enhancing AML recognition by immunotherapy techniques. Several strategies based on the use of NK cells have been proposed to allow the recognition of AML cells, such as: a) NK infusion. Autologous NK cells or NK cells from a KIR-ligand mismatched donor, are expanded in vitro in the presence of IL-2, IFN-γ, and/or anti-CD3. AML patients are infused with these cells and treated with IL-2 to promote the expansion of NK cells. An alternative is the infusion of allogeneic CIML cells that are expanded in vitro in the presence of a cytokine cocktail (IL-12, IL-15 and IL-18). An advantage of this treatment is that there is no need to treat the patient with IL-2; b) Epigenetic treatments. Treatment with HDACi and DNMTi restores NKG2DL (MICA and ULBPs 1–3) expression on the cell surface of AML cells. The TIMP3 gene, which is methylated in some AML patients, is also expressed, leading to the inhibition of the main protease involved in the release of NKG2DL, ADAM17. As a consequence, NKG2DL (MICA/B and ULBP2) are not shed from the cell surface and are released in their soluble form (sMICA/B and sULBP2), maintaining the high expression levels on the AML cell surface. c) Immune checkpoint blockade. Specific antibodies against PD-1 (nivolumab, pembrolizumab) or its ligand PD-L1 (durvalumab) block the PD-1/PD-L1 interaction, avoiding the anergy of NK cells; and d) CAR technology. T cells or NK cells collected from the AML patient are transduced with CAR with specific genes (NKG2D, NKp30) or antibodies (α-CD33, α-CD7). Further, these cells are infused in AML patients and when CAR recognizes its antigen, expressed on the surface of AML cell, CAR-T or CAR-NKs are activated.
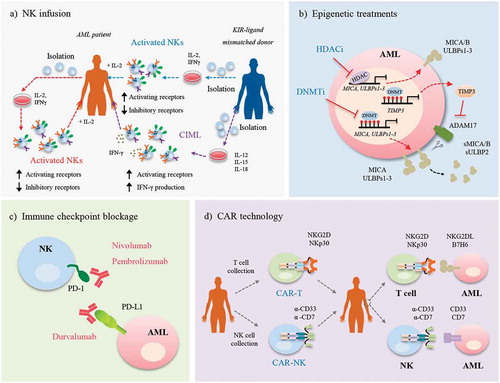
3.1.2. Allogeneic NK cells
Further studies were performed using allogeneic NKs from healthy donors that maintain their function and can be safely administered. As previously described, during NK cell development and to guarantee ‘self-tolerance’, KIR receptors bind with their ligands to ‘license’ the NKs and avoid the recognition and lysis of self-cells.Citation43 Studies were carried out in AML patients using alloreactive NKs pre-activated with IL-2 and with one or more KIR-ligands mismatched to avoid the recognition of self-HLA class I molecules ().Citation44,Citation45 All patients receive immunosuppressive chemotherapy before NK cell infusion, and further exogenous administration of IL-2 in order to activate and expand circulating donor NK cells. One benefit of this therapy is the low incidence of graft versus host disease (GvHD) and the production of a strong graft versus leukemia (GvL) that is associated with better survival and a lower probability of relapse. In elderly patients, whose therapeutic options are very limited, consolidation therapy with these cells is feasible and promotes a better disease-free survival rate.Citation46 Moreover, allogeneic clones can persist for up to 12 months, allowing the elimination of residual blasts.Citation47 Several clinical trials are currently under way using haploidentical NKs as consolidation therapy in AML, or in combination with other therapies, to determine their anti-leukemic effect and any possible secondary effects ().
Ex vivo stimulation of NKs with a cytokine cocktail of IL-12, IL-15 and IL-18 is an established alternative to avoid the in vivo administration of IL-2 after NK cell infusion.Citation48 These cells, known as CIML (cytokine-induced memory-like), proliferate and produce high levels of IFN-γ during the first week, after which its production decreases. However, the in vitro re-stimulation of CIML cells, with the same activation cytokine cocktail or with a target cell (cell line or primary AML cells), restores the production of IFN-γ, suggesting that these cells have memory properties.Citation49 Moreover, these cells more strongly express activating receptors (NKG2D, DNAM-1, NKp30, NKp44, NKp46) relative to unstimulated NKs, implying a greater cytotoxicity to tumor cells and, thereby, a higher frequency of CR in elderly relapsed/refractory AML patients.Citation30 In fact, there have been several phase I and II trials based on relapsed/refractory AML patients using CIML cells, alone (0.5–10 x 10Citation6 cells/kg) or in combination with other treatments ().
Alternative ex vivo expansion methods for NK cells are currently being evaluated in several clinical trials for the treatment of AML patients (). One of them is the infusion of allogeneic CD3−CD19− NKs, a method that increases the proliferation and cytolytic capacity of infused NK cells in patients. Another is the use of an IL-15 antagonist (ALT-803) that increases the activation and cytotoxic capacity of NK cells with respect to IL-2. In addition, one of the most important advances is the enrichment and expansion of terminally differentiated adaptive NK cells (commercially known as FATE-NK100) and that are currently being evaluated in refractory or relapsed AML patients (). These cells are obtained from some specific NK cell subsets (CD57+ NKG2C+) that are expanded following CMV infection, creating a pool of adaptive NK cells with a lower threshold of activation.Citation50 To generate these cells, peripheral blood mononuclear cells from CMV-seropositive donors were depleted of CD3+ T and CD19+ B cells and cultured with IL-15 (a cytokine that drives NK cell proliferation) and a small molecule inhibitor of glycogen synthase kinase 3-beta (GSK3β) for 7–9 days.Citation51 This facilitates the generation of a large number of mature CD57+ NK cells with increased IFN-γ production. In addition to AML, two clinical trials are currently ongoing, one (NTC03319459) in subjects with advanced solid tumors (gastric, colorectal, and head and neck squamous cell carcinoma), and the other (NTC03213964) in women with advanced ovarian, fallopian tube or primary peritoneal cancer.
3.2. Regulation of NK cell ligands by epigenetic mechanisms
To enhance the cytotoxic ability of NK cells it is essential to recognize the engagement between activating receptors and their ligands expressed on the cell surface of target cells. However, as we have remarked above, the expression of these ligands is reduced or absent in AML cells, so their expression must be enhanced in order to facilitate immune recognition mediated by NKs.
Epigenetic modifications that modulate the expression of activating ligands are known to arise during cancer development. Several studies have reported that treatment with inhibitors of histone deacetylases (HDACi; valproic acid, trichostatin A or all-trans-retinoic acid) increases the expression of NKG2DL (MICA/B, ULBPs1-3) and decreases the release of their soluble forms in cell lines and primary AML cells, thereby enhancing the lytic capacity of NKs ().Citation20,Citation52,Citation53 Histone acetylation is also important for regulating other activating ligands in AML, such as CD48 (2B4 ligand).Citation54
DNA methylation is another important epigenetic mechanism of gene regulation. Results from our group revealed that DNA methylation can contribute to the absence of NKG2DL expression observed during tumor development in AML patients.Citation55 We found significantly higher DNA methylation levels in the promoter region of MICA, ULBP1 and ULBP2 genes in AML patients compared with healthy donors, and this was correlated with the absence of transcription for these genes. Moreover, 59% of AML patients had at least one methylated NKG2DL, although the combination of ligands varied between patients. DNA methylation is a reversible mechanism, so treatment with DNA methyltransferase inhibitors (DNMTi) such as azacitidine (Vidaza®, AZA) and DAC, which are commonly used to treat elderly AML patients, restores the expression of NKG2DL on the surface of AML cells (). Our group recently reported that treatment with these drugs also reduces the release of soluble NKG2DL, maintaining their expression on the surface of AML cells by the hypomethylation of TIMP3, an inhibitor of protease ADAM17 ().Citation56 However, the role of DNA methylation in regulating other activating ligands is unknown.
All these studies suggest that the use of epigenetic treatments in combination with other conventional therapies could be an important method for enhancing the expression of activating ligands in AML blasts and thus the recognition mediated by allogeneic NKs.
3.3. Blockade of immune checkpoints
Given the role of immune checkpoints in inhibiting NK cell activation, the effect of blocking these molecules has been evaluated. Blockade of some immune checkpoints (LAG-3, TIM-3, PD-1) with specific antibodies increases the lytic capacity of CIML cells against AML ().Citation57 In an experimental mouse model of lung cancer, the specific blockade of LAG-3 and PD-1 increases the number of mature NKs and thereby reactivates the antitumoral properties (greater production of cytotoxic granules and IFNγ) of CIML cells, allowing the control of the metastasis.Citation58
Within immune checkpoints, PD-1 and its ligand (PD-L1) are the most thoroughly studied due to the excellent results obtained from T cells in several tumors. It has also been reported that PD-1 or PD-L1 blockade increases the degranulation and cytotoxic capacity of NKs, shrinking the tumor grown in xenograft models of digestive and myeloma cancers.Citation33,Citation59 In AML, several clinical trials are currently underway to evaluate the response of this blockade (). To this end, patients with relapse/refractory or high-risk AML are treated with specific antibodies against PD-1 or PD-L1 alone or in combination with other therapies (AZA, chemotherapy, dendritic cell vaccine). Unpublished preliminary data from the NCT02397720 trial show that the combined use of AZA with anti-PD-1 (nivolumab) is well tolerated and produces durable responses in relapsed AML. Therefore, the results of current clinical trials are needed to corroborate the efficiency of this therapy, as has been observed in solid tumors.
Recent significant studies have shown TIGIT and IL-1R8 to be new checkpoint molecules or negative regulators of NK cell function in several solid tumors.Citation39,Citation60 Blockade of these proteins reverses the exhaustion of tumor-infiltrating NK cells and reduces the threshold for NK cell activation, respectively. The study of these mechanisms in AML, in combination or not with other therapeutic approaches, could help enhance the NK-cell mediated anti-tumor response.
3.4. Chimeric antigen receptor-based immunotherapy
Chimeric antigen receptor (CAR) therapy is an emerging immunotherapy that combines specific antigen recognition with signaling capacity to recognize specific targets in cancer cells.Citation61 These CARs can be expressed in immune cells as T lymphocytes (CAR-T therapy) by viral transduction or mRNA transfection, and frequently its specificity is based on scFv regions or TCR binding domains. Several CAR-T therapies directed against some of the most important targets in AML blasts (such as CD33, FLT3 and CD123) have been assayed in recent years, revealing potent activity against tumor cells.Citation62–Citation64 An ideal CAR target should be expressed in a large number of AML blasts and with high-density but in as few normal tissues as possible to avoid undue toxicity. These targets are widely expressed in the majority of AML blasts but, unfortunately, also in some normal hematopoietic stem cells, myeloid cells and other tissues. CAR-T cell immunotherapy aimed at simultaneously targeting two AML antigens (CD33 and CD123) or other candidates identified by integrating proteomic and genomic datasets is a promising strategy for effectively eliminating AML cells and leukemic stem cells, while avoiding relapse and without incurring greater toxicity.Citation65,Citation66
CAR technology may also be useful for enhancing the recognition and lysis mediated by NK cells (). Two strategies have been proposed for this: CAR-T encoding NK cell-activating receptors, and CAR-NK. In the first strategy, the use of a CAR-T with a full-length human NKG2D (NKG2D CAR-T) to target cancer cells expressing NKG2D ligands has been reported.Citation67 In murine models of several tumors (glioblastoma, osteosarcoma) this NKG2D CAR-T can infiltrate the tumor, downregulating its activity and increasing survival.Citation68,Citation69 This therapy protects against future relapses because surviving animals can respond to a new infiltration of tumor cells. However, one disadvantage of this CAR-T model is its high toxicity as a consequence of the increased production of cytokines/chemokines (IFN-γ, IL-6, MCP-1) by activated T cells.Citation70 This could be avoided by employing the alternative strategy of using memory T cells, which are known to be less toxic after CAR-T infusion in murine models of glioblastoma and osteosarcoma.Citation68,Citation69 Although the antitumoral effect of NKG2D CAR-T is unknown in AML models, the Dana-Farber Cancer Institute is conducting a phase I clinical trial (NCT02203825) in which patients with AML, myelodysplastic syndrome and multiple myeloma are infused with a single dose (0.1-3 x 107 cell/kg) of NKG2D CAR-T (). NKp30 is another activating receptor whose use in CAR-T models has been proposed.Citation71 This NKp30 CAR-T increases the production of IFN-γ and the cytotoxic capacity towards malignant cells that express its ligand, B7H6. NKp30 CAR-T improves survival in lymphoma murine models and prevents the development of the tumor after a subsequent challenge of malignant cells, suggesting that an immunological memory is generated. CAR-T technology can also be used to target the ligands of the activating receptors and to enhance the lysis of tumor cells. In this way, a B7H6 CAR-T therapy enhances the release of IFN-γ by transfected T cells against B7H6-expressing cell lines, and prolongs survival in ovarian and lymphoma murine models.Citation72,Citation73
The second strategy for enhancing the recognition between NK and tumor cells is the use of CAR-modified NK cells (). This therapy is attracting attention because of its advantages over T lymphocytes: (1) sensitization is not necessary; (2) there is no MHC restriction; (3) antigen-specific receptors are not required; and (4) there are fewer side effects than with GvHD.Citation74 There are currently two phase I and II clinical trials underway using CD7- or CD33-CAR NK-92 cells in AML patients with relapsed or refractory disease (). The first findings, which have recently been published, show that high doses of CD33-CAR NK cells have no significant adverse effects on the three analyzed AML patients, showing the safety of the treatment, although further studies are needed to establish the clinical efficacy.Citation75
4. Concluding remarks
NK cells are key effectors in cancer immunosurveillance that promote the recognition and lysis of tumor cells. However, the evolution and frequent relapses in AML patients suggest that leukemia cells can escape this recognition. As mentioned above, several mechanisms that impair NK cell functions in AML have been described in recent years that have prompted great efforts aimed at developing new therapeutic strategies that enhance the recognition and lysis of AML cells by NK cells. Currently available immunotherapeutic treatments have yielded promising results in vitro and in mouse models of AML. Nevertheless, it is important to perform in-depth studies to determine the toxicity of these immunotherapies in AML patients. The ability to inhibit AML targets specifically using immune checkpoint blockade or CAR-T/CAR-NK therapies opens up new possibilities for NK cell-based immunotherapy. A considerable number of clinical trials are currently underway using both therapies, alone or in combination with well-established AML treatments. The results of these studies will clarify the clinical value of both immunotherapies as treatments for AML.
Abbreviations
AML | = | acute myeloid leukemia |
CAR | = | chimeric antigen receptor |
CIML | = | cytokine-induced memory-like |
CR | = | complete remission |
FAB | = | French-American-British classification |
GvHD | = | graft versus host disease |
GvL | = | graft versus leukemia |
HSCT | = | hematopoietic stem cell transplant |
NK | = | natural killer |
WHO | = | World Health Organization. |
Conflicts of interest
The authors declare no conflicts of interest.
Additional information
Funding
References
- Pandolfi A, Barreyro L, Steidl U. Concise review: preleukemic stem cells: molecular biology and clinical implications of the precursors to leukemia stem cells. Stem Cells Transl Med. 2013;2(2):143–150. doi:10.5966/sctm.2012-0109.
- Papaemmanuil E, Gerstung M, Bullinger L, Gaidzik VI, Paschka P, Roberts ND, Potter NE, Heuser M, Thol F, Bolli N, et al. Genomic classification and prognosis in acute myeloid leukemia. N Engl J Med. 2016;374(23):2209–2221. doi:10.1056/NEJMoa1516192.
- Klco JM, Spencer DH, Miller CA, Griffith M, Lamprecht TL, O’Laughlin M, Fronick C, Magrini, Demeter RT, Fulton RS, et al. Functional heterogeneity of genetically defined subclones in acute myeloid leukemia. Cancer Cell. 2014;25(3):379–392. doi:10.1016/j.ccr.2014.01.031.
- Aziz H, Ping CY, Alias H, AbMutalib NS, Jamal R. Gene mutations as emerging biomarkers and therapeutic targets for relapsed acute myeloid leukemia. Front Pharmacol. 2017;8:897. doi:10.3389/fphar.2017.00897.
- Rothenberg-Thurley M, Amler S, Goerlich D, Köhnke T, Konstandin NP, Schneider S, Sauerland MC, Herold T, Hubmann M, Ksienzyk B, et al. Persistence of pre-leukemic clones during first remission and risk of relapse in acute myeloid leukemia. Leukemia. 2018;32(7):1598–1608. doi:10.1038/s41375-018-0034-z.
- Arber DA, Orazi A, Hasserjian R, Thiele J, Borowitz MJ, Le Beau MM, Bloomfield CD, Cazzola M, Vardiman JW. The 2016 revision to the World Health Organization classification of myeloid neoplasms and acute leukemia. Blood. 2016;127(20):2391–2405. doi:10.1182/blood-2016-03-643544.
- Döhner H, Estey E, Grimwade D, Amadori S, Appelbaum FR, Büchner T, Dombret H, Ebert BL, Fenaux P, Larson RA, et al. Diagnosis and management of AML in adults: 2017 ELN recommendations from an international expert panel. Blood. 2017;129(4):424–447. doi:10.1182/blood-2016-08-733196.
- Bertoli S, Tavitian S, Huynh A, Borel C, Guenounou S, Luquet I, Delabesse E, Sarry A, Laurent G, Attal M, et al. Improved outcome for AML patients over the years 2000-2014. Blood Cancer J. 2017;7(12):635. doi:10.1038/s41408-017-0011-1.
- Imai K, Matsuyama S, Miyake S, Suga K, Nakachi K. Natural cytotoxic activity of peripheral-blood lymphocytes and cancer incidence: an 11-year follow-up study of a general population. Lancet. 2000;356(9244):1795–1799. doi:10.1016/S0140-6736(00)03231-1.
- Ruggeri L, Capanni M, Urbani E, Perruccio K, Shlomchik WD, Tosti A, Posati S, Rogaia D, Frassoni F, Aversa F, et al. Effectiveness of donor natural killer cell alloreactivity in mismatched hematopoietic transplants. Science. 2002;295(5562):2097–2100. doi:10.1126/science.1068440.
- Pegram HJ, Andrews DM, Smyth MJ, Darcy PK, Kershaw MH. Activating and inhibitory receptors of natural killer cells. Immunol Cell Biol. 2011;89(2):216–224. doi:10.1038/icb.2010.78.
- Orr MT, Lanier LL. Natural killer cell education and tolerance. Cell. 2010;142(6):847–856. doi:10.1016/j.cell.2010.08.031.
- Sandoval-Borrego D, Moreno-Lafont MC, Vazquez-Sanchez EA, Gutierrez-Hoya A, López-Santiago R, Montiel-Cervantes LA, Ramírez-Saldaña M, Vela-Ojeda J. Overexpression of CD158 and NKG2A inhibitory receptors and underexpression of NKG2D and NKp46 activating receptors on NK cells in acute myeloid leukemia. Arch Med Res. 2016;47(1):55–64. doi:10.1016/j.arcmed.2016.02.001.
- Sanchez-Correa B, Gayoso I, Bergua JM, Casado JG, Morgado S, Solana R, Tarazona R. Decreased expression of DNAM-1 on NK cells from acute myeloid leukemia patients. Immunol Cell Biol. 2012;90(1):109–115. doi:10.1038/icb.2011.15.
- Fauriat C, Just-Landi S, Mallet F, Arnoulet C, Sainty D, Olive D, Costello RT. Deficient expression of NCR in NK cells from acute myeloid leukemia: evolution during leukemia treatment and impact of leukemia cells in NCR dull phenotype induction. Blood. 2007;109(1):323–330. doi:10.1182/blood-2005-08-027979.
- Chretien AS, Fauriat C, Orlanducci F, Rey J, Borg GB, Gautherot E, Granjeaud S, Demerle C, Hamel JF, Cerwenka A, et al. NKp30 expression is a prognostic immune biomarker for stratification of patients with intermediate-risk acute myeloid leukemia. Oncotarget. 2017;8(30):49548–49563. doi:10.18632/oncotarget.17747.
- Chretien AS, Devillier R, Fauriat C, Orlanducci F, Harbi S, Le Roy A, Rey J, Bouvier Borg G, Gautherot E, Hamel JF, et al. NKp46 expression on NK cells as a prognostic and predictive biomarker for response to allo-SCT in patients with AML. Oncoimmunology. 2017;6(12):e1307491. doi:10.1080/2162402X.2017.1307491.
- Nowbakht P, Ionescu MC, Rohner A, Kalberer CP, Rossy E, Mori L, Cosman D, De Libero G, Wodnar-Filipowicz A. Ligands for natural killer cell-activating receptors are expressed upon the maturation of normal myelomonocytic cells but at low levels in acute myeloid leukemias. Blood. 2005;105(9):3615–3622. doi:10.1182/blood-2004-07-2585.
- Pende D, Spaggiari GM, Marcenaro S, Martini S, Rivera P, Capobianco A, Falco M, Lanino E, Pierri I, Zambello R, et al. Analysis of the receptor-ligand interactions in the natural killer-mediated lysis of freshly isolated myeloid or lymphoblastic leukemias: evidence for the involvement of the Poliovirus receptor (CD155) and Nectin-2 (CD112). Blood. 2005;105(5):2066–2073. doi:10.1182/blood-2004-09-3548.
- Diermayr S, Himmelreich H, Durovic B, Mathys-Schneeberger A, Siegler U, Langenkamp U, Hofsteenge J, Gratwohl A, Tichelli A, Paluszewska M, et al. NKG2D ligand expression in AML increases in response to HDAC inhibitor valproic acid and contributes to allorecognition by NK-cell lines with single KIR-HLA class I specificities. Blood. 2008;111(3):1428–1436. doi:10.1182/blood-2007-07-101311.
- Hilpert J, Grosse-Hovest L, Grünebach F, Buechele C, Nuebling T, Raum T, Steinle A, Salih HR. Comprehensive analysis of NKG2D ligand expression and release in leukemia: implications for NKG2D-mediated NK cell responses. JImmunol. 2012;189(3):1360–1371. doi:10.4049/jimmunol.1200796.
- Salih HR, Antropius H, Gieseke F, Lutz SZ, Kanz L, Rammensee HG, Steinle A. Functional expression and release of ligands for the activating immunoreceptor NKG2D in leukemia. Blood. 2003;102(4):1389–1396. doi:10.1182/blood-2003-01-0019.
- Stringaris K, Sekine T, Khoder A, Alsuliman A, Razzaghi B, Sargeant R, Pavlu J, Brisley G, de Lavallade H, Sarvaria A, et al. Leukemia-induced phenotypic and functional defects in natural killer cells predict failure to achieve remission in acute myeloid leukemia. Haematologica. 2014;99(5):836–847. doi:10.3324/haematol.2013.087536.
- Geiger TL, Sun JC. Development and maturation of natural killer cells. Curr Opin Immunol. 2016;39:82–89. doi:10.1016/j.coi.2016.01.007.
- Mundy-Bosse BL, Scoville SD, Chen L, McConnell K, Mao HC, Ahmed EH, Zorko N, Harvey S, Cole J, Zhang X, et al. MicroRNA-29b mediates altered innate immune development in acute leukemia. J Clin Invest. 2016;126(12):4404–4416. doi:10.1172/JCI85413.
- Björkström NK, Riese P, Heuts F, Andersson S, Fauriat C, Ivarsson MA, Björklund AT, Flodström-Tullberg M, Michaëlsson J, Rottenberg ME, et al. Expression patterns of NKG2A, KIR, and CD57 define a process of CD56dim NK-cell differentiation uncoupled from NK-cell education. Blood. 2010;116(19):3853–3864. doi:10.1182/blood-2010-04-281675.
- Chretien AS, Fauriat C, Orlanducci F, Galseran C, Rey J, Bouvier Borg G, Gautherot E, Granjeaud S, Hamel-Broza JF, Demerle C, et al. Natural killer defective maturation is associated with adverse clinical outcome in patients with acute myeloid leukemia. Front Immunol. 2017;8:573. doi:10.3389/fimmu.2017.00573.
- O’Leary JG, Goodarzi M, Drayton DL, von Andrian UH. T cell- and B cell-independent adaptive immunity mediated by natural killer cells. Nat Immunol. 2006;7(5):507–516. doi:10.1038/ni1332.
- Leong JW, Chase JM, Romee R, Schneider SE, Sullivan RP, Cooper MA, Fehniger TA. Preactivation with IL-12, IL-15, and IL-18 induces CD25 and a functional high-affinity IL-2 receptor on human cytokine-induced memory-like natural killer cells. Biol Blood Marrow Transplant. 2014;20(4):463–473. doi:10.1016/j.bbmt.2014.01.006.
- Romee R, Rosario M, Berrien-Elliott MM, Wagner JA, Jewell BA, Schappe T, Leong JW, Abdel-Latif S, Schneider SE, Willey S, et al. Cytokine-induced memory-like natural killer cells exhibit enhanced responses against myeloid leukemia. SciTransl Med. 2016;8(357):357ra123. doi:10.1126/scitranslmed.aaf2341.
- Beldi-Ferchiou A, Caillat-Zucman S. Control of NK cell activation by immune checkpoint molecules. Int J Mol Sci. 2017;18(10): pii: E2129. doi:10.3390/ijms18102129.
- Benson DM Jr, Bakan CE, Mishra A, Hofmeister CC, Efebera Y, Becknell B, Baiocchi RA, Zhang J, Yu J, Smith MK, et al. The PD-1/PD-L1 axis modulates the natural killer cell versus multiple myeloma effect: a therapeutic target for CT-011, a novel monoclonal anti-PD-1 antibody. Blood. 2010;116(13):2286–2294. doi:10.1182/blood-2010-02-271874.
- Liu Y, Cheng Y, Xu Y, Wang Z, Du X, Li C, Peng J, Gao L, Liang X, Ma C. Increased expression of programmed cell death protein 1 on NK cells inhibits NK-cell-mediated anti-tumor function and indicates poor prognosis in digestive cancers. Oncogene. 2017;36(44):6143–6153. doi:10.1038/onc.2017.209.
- Berthon C, Driss V, Liu J, Kuranda K, Leleu X, Jouy N, Hetuin D, Quesnel B. In acute myeloid leukemia, B7-H1 (PD-L1) protection of blasts from cytotoxic T cells is induced by TLR ligands and interferon-gamma and can be reversed using MEK inhibitors. Cancer Immunol Immunother. 2010;59(12):1839–1849. doi:10.1007/s00262-010-0909-y.
- Goltz D, Gevensleben H, Grünen S, Dietrich J, Kristiansen G, Landsberg J, Dietrich D. PD-L1 (CD274) promoter methylation predicts survival in patients with acute myeloid leukemia. Leukemia. 2017;31(3):738–743. doi:10.1038/leu.2016.328.
- Folgiero V, Cifaldi L, Li Pira G, Goffredo BM, Vinti L, Locatelli F. TIM-3/Gal-9 interaction induces IFNγ-dependent IDO1 expression in acute myeloid leukemia blast cells. J Hematol Oncol. 2015;8:36. doi:10.1186/s13045-015-0134-4.
- Kikushige Y, Miyamoto T, Yuda J, Jabbarzadeh-Tabrizi S, Shima T, Takayanagi S, Niiro H, Yurino A, Miyawaki K, Takenaka K, et al. A TIM-3/Gal-9 autocrine stimulatory loop drives self-renewal of human myeloid leukemia stem cells and leukemic progression. Cell Stem Cell. 2015;17(3):341–352. doi:10.1016/j.stem.2015.07.011.
- Wang M, Bu J, Zhou M, Sido J, Lin Y, Liu G, Lin Q, Xu X, Leavenworth JW, Shen E. CD8+T cells expressing both PD-1 and TIGIT but not CD226 are dysfunctional in acute myeloid leukemia (AML) patients. Clin Immunol. 2018;190:64–73. doi:10.1016/j.clim.2017.08.021.
- Zhang Q, Bi J, Zheng X, Chen Y, Wang H, Wu W, Wang Z, Wu Q, Peng H, Wei H, et al. Blockade of the checkpoint receptor TIGIT prevents NK cell exhaustion and elicits potent anti-tumor immunity. Nat Immunol. 2018 Jun 18. [Epub ahead of print]. doi:10.1038/s41590-018-0132-0.
- Rosenberg SA, Lotze MT, Muul LM, Chang AE, Avis FP, Leitman S, Linehan WM, Robertson CN, Lee RE, Rubin JT, et al. A progress report on the treatment of 157 patients with advanced cancer using lymphokine-activated killer cells and interleukin-2 or high-dose interleukin-2 alone. N Engl J Med. 1987;316(15):889–897. doi:10.1056/NEJM198704093161501.
- Sievers EL, Lange BJ, Sondel PM, Krailo MD, Gan J, Liu-Mares W, Feig SA. Feasibility, toxicity, and biologic response of interleukin-2 after consolidation chemotherapy for acute myelogenous leukemia: a report from the Children’s Cancer Group. J ClinOncol. 1998;16(3):914–919. doi:10.1200/JCO.1998.16.3.914.
- Ewen EM, Pahl JHW, Miller M, Watzl C, Cerwenka A. KIR downregulation by IL-12/15/18 unleashes human NK cells from KIR/HLA-I inhibition and enhances killing of tumor cells. Eur J Immunol. 2018;48(2):355–365. doi:10.1002/eji.201747128.
- Kim S, Poursine-Laurent J, Truscott SM, Lybarger L, Song YJ, Yang L, French AR, Sunwoo JB, Lemieux S, Hansen TH, et al. Licensing of natural killer cells by host major histocompatibility complex class I molecules. Nature. 2005;436(7051):709–713. doi:10.1038/nature03847.
- Miller JS, Soignier Y, Panoskaltsis-Mortari A, McNearney SA, Yun GH, Fautsch SK, McKenna D, Le C, Defor TE, Burns LJ, et al. Successful adoptive transfer and in vivo expansion of human haploidentical NK cells in patients with cancer. Blood. 2005;105(8):3051–3057. doi:10.1182/blood-2004-07-2974.
- Rubnitz JE, Inaba H, Ribeiro RC, Pounds S, Rooney B, Bell T, Pui CH, Leung W. NKAML: a pilot study to determine the safety and feasibility of haploidentical natural killer cell transplantation in childhood acute myeloid leukemia. J Clin Oncol. 2010;28(6):955–959. doi:10.1200/JCO.2009.24.4590.
- Curti A, Ruggeri L, Parisi S, Bontadini A, Dan E, Motta MR, Rizzi S, Trabanelli S, Ocadlikova, Lecciso M, et al. Larger size of donor alloreactive NKcell repertoire correlates with better response to NK cell immunotherapy in elderly acute myeloid leukemia patients. Clin Cancer Res. 2016;22(8):1914–1921. doi:10.1158/1078-0432.CCR-15-1604.
- Ruggeri L, Mancusi A, Capanni M, Urbani E, Carotti A, Aloisi T, Stern M, Pende D, Perruccio K, Burchielli E, et al. Donor natural killer cell allorecognition of missing self in haploidentical hematopoietic transplantation for acute myeloid leukemia: challenging its predictive value. Blood. 2007;110(1):433–440. doi:10.1182/blood-2006-07-038687.
- Cooper MA, Elliott JM, Keyel PA, Yang L, Carrero JA, Yokoyama WM. Cytokine-induced memory-like natural killer cells. Proc Natl Acad Sci U S A. 2009;106(6):1915–1919. doi:10.1073/pnas.0813192106.
- Romee R, Schneider SE, Leong JW, Chase JM, Keppel CR, Sullivan RP, Cooper MA, Fehniger TA. Cytokine activation induces human memory-like NK cells. Blood. 2012;120(24):4751–4760. doi:10.1182/blood-2012-04-419283.
- Cichocki F, Cooley S, Davis Z, DeFor TE, Schlums H, Zhang B, Brunstein CG, Blazar BR, Wagner J, Diamond DJ, et al. CD56dim CD57+ NKG2C+ NK cell expansion is associated with reduced leukemia relapse after reduced intensity HCT. Leukemia. 2016;30(2):456–463. doi:10.1038/leu.2015.260.
- Parameswaran R, Ramakrishnan P, Moreton SA, Xia Z, Hou Y, Lee DA, Gupta K, deLima M, Beck RC, Wald DN. Repression of GSK3 restores NK cell cytotoxicity in AML patients. Nat Commun. 2016;7:11154. doi:10.1038/ncomms11154.
- Lu X, Ohata K, Kondo Y, Espinoza JL, Qi Z, Nakao S. Hydroxyurea upregulates NKG2D ligand expression in myeloid leukemia cells synergistically with valproic acid and potentially enhances susceptibility of leukemic cells to natural killer cell-mediated cytolysis. Cancer Sci. 2010;101(3):609–615. doi:10.1111/j.1349-7006.2009.01439.x.
- Poggi A, Catellani S, Garuti A, Pierri I, Gobbi M, Zocchi MR. Effective in vivo induction of NKG2D ligands in acute myeloid leukaemias by all-trans-retinoic acid or sodium valproate. Leukemia. 2009;23(4):641–648. doi:10.1038/leu.2008.354.
- Elias S, Yamin R, Golomb L, Tsukerman P, Stanietsky-Kaynan N, Ben-Yehuda D, Mandelboim O. Immune evasion by oncogenic proteins of acute myeloid leukemia. Blood. 2014;123(10):1535–1543. doi:10.1182/blood-2013-09-526590.
- BaragañoRaneros A, Martín-Palanco V, Fernandez AF, Rodriguez RM, Fraga MF, Lopez-Larrea C, Suarez-Alvarez B. Methylation of NKG2D ligands contributes to immune system evasion in acute myeloid leukemia. Genes Immun. 2015;16(1):71–82. doi:10.1038/gene.2014.58.
- Raneros AB, Puras AM, Rodriguez RM, Colado E, Bernal T, Anguita E, Mogorron AV, Gil AC, Vidal-Castiñeira JR, Márquez-Kisinousky L, et al. Increasing TIMP3 expression by hypomethylating agents diminishes soluble MICA, MICB and ULBP2 shedding in acute myeloid leukemia, facilitating NK cell-mediated immune recognition. Oncotarget. 2017;8(19):31959–31976. doi:10.18632/oncotarget.16657.
- Poh SL, Linn YC. Immune checkpoint inhibitors enhance cytotoxicity of cytokine-induced killer cells against human myeloid leukaemic blasts. Cancer Immunol Immunother. 2016;65(5):525–536. doi:10.1007/s00262-016-1815-8.
- Ohs I, Ducimetière L, Marinho J, Kulig P, Becher B, Tugues S. Restoration of natural killer cell antimetastatic activity by IL12 and checkpoint blockade. Cancer Res. 2017;77(24):7059–7071. doi:10.1158/0008-5472.CAN-17-1032.
- Guo Y, Feng X, Jiang Y, Shi X, Xing X, Liu X, Li N, Fadeel B, Zheng C. PD1 blockade enhances cytotoxicity of in vitro expanded natural killer cells towards myeloma cells. Oncotarget. 2016;7(30):48360–48374. doi:10.18632/oncotarget.10235.
- Molgora M, Bonavita E, Ponzetta A, Riva F, Barbagallo M, Jaillon S, Popović B, Bernardini G, Magrini E, Gianni F, et al. IL-1R8 is a checkpoint in NK cells regulating anti-tumour and anti-viral activity. Nature. 2017;551(7678):110–114. doi:10.1038/nature24293.
- June CH, Sadelain M. Chimeric antigen receptor therapy. N Engl J Med. 2018;379(1):64–73. doi:10.1056/NEJMra1706169.
- Kenderian SS, Ruella M, Shestova O, Klichinsky M, Aikawa V, Morrissette JJ, Scholler J, Song D, Porter DL, Carroll M, et al. CD33-specific chimeric antigen receptor T cells exhibit potent preclinical activity against human acute myeloid leukemia. Leukemia. 2015;29(8):1637–1647. doi:10.1038/leu.2015.52.
- Chen L, Mao H, Zhang J, Chu J, Devine S, Caligiuri MA, Yu J. Targeting FLT3 by chimeric antigen receptor T cells for the treatment of acute myeloid leukemia. Leukemia. 2017;31(8):1830–1834. doi:10.1038/leu.2017.147.
- Mardiros A, Dos Santos C, McDonald T, Brown CE, Wang X, Budde LE, Hoffman L, Aguilar B, Chang WC, Bretzlaff W, et al. T cells expressing CD123-specific chimeric antigen receptors exhibit specific cytolytic effector functions and antitumor effects against human acute myeloid leukemia. Blood. 2013;122(18):3138–3148. doi:10.1182/blood-2012-12-474056.
- Perna F, Berman SH, Soni RK, Mansilla-Soto J, Eyquem J, Hamieh M, Hendrickson RC, Brennan CW, Sadelain M. Integrating proteomics and transcriptomics for systematic combinatorial chimeric antigen receptor therapy of AML. Cancer Cell. 2017;32(4):506–19.e5. doi:10.1016/j.ccell.2017.09.004.
- Petrov JC, Wada M, Pinz KG, Yan LE, Chen KH, Shuai X, Liu H, Chen X, Leung LH, Salman H, et al. Compound CAR T-cells as a double-pronged approach for treating acute myeloid leukemia. Leukemia. 2018;32(6):1317–1326. doi:10.1038/s41375-018-0075-3.
- Sentman CL, Meehan KR. NKG2D CARs as cell therapy for cancer. Cancer J. 2014;20(2):156–159. doi:10.1097/PPO.0000000000000029.
- Weiss T, Weller M, Guckenberger M, Sentman CL, Roth P. NKG2D-based CAR T cells and radiotherapy exert synergistic efficacy in glioblastoma. Cancer Res. 2018;78(4):1031–1043. doi:10.1158/0008-5472.CAN-17-1788.
- Fernández L, Metais JY, Escudero A, Vela M, Valentín J, Vallcorba I, Leivas A, Torres J, Valeri A, Patiño-García A, et al. Memory T cells expressing an NKG2D-CAR efficiently target osteosarcoma cells. Clin Cancer Res. 2017;23(19):5824–5835. doi:10.1158/1078-0432.CCR-17-0075.
- Sentman ML, Murad JM, Cook WJ, Wu MR, Reder J, Baumeister SH, Dranoff G, Fanger MW, Sentman CL. Mechanisms of acute toxicity in NKG2D chimeric antigen receptor T cell-treated mice. J Immunol. 2016;197(12):4674–4685. doi:10.4049/jimmunol.1600769.
- Zhang T, Wu MR, Sentman CL. An NKp30-based chimeric antigen receptor promotes T cell effector functions and antitumor efficacy in vivo. Immunol. 2012;189(5):2290–2299. doi:10.4049/jimmunol.1103495.
- Wu MR, Zhang T, DeMars LR, Sentman CL. B7H6-specific chimeric antigen receptors lead to tumor elimination and host antitumor immunity. Gene Ther. 2015;22(8):675–684. doi:10.1038/gt.2015.29.
- Gacerez AT, Hua CK, Ackerman ME, Sentman CL. Chimeric antigen receptors with human scFvs preferentially induce T cell anti-tumor activity against tumors with high B7H6 expression. Cancer Immunol Immunother. 2018;67(5):749–759. doi:10.1007/s00262-018-2124-1.
- Liu D, Tian S, Zhang K, Xiong W, Lubaki NM, Chen Z, Han W. Chimeric antigen receptor (CAR)-modified natural killer cell-based immunotherapy and immunological synapse formation in cancer and HIV. Protein Cell. 2017;8(12):861–877. doi:10.1007/s13238-017-0415-5.
- Tang X, Yang L, Li Z, Nalin AP, Dai H, Xu T, Yin J, You F, Zhu M, Shen W, et al. First-in-man clinical trial of CAR NK-92 cells: safety test of CD33-CAR NK-92 cells in patients with relapsed and refractory acute myeloid leukemia. Am J Cancer Res. 2018;8(6):1083–1089.