ABSTRACT
Gold glyconanoparticles loaded with the listeriolysin O peptide 91–99 (GNP-LLO91-99), a bacterial peptide with anti-metastatic properties, are vaccine delivery platforms facilitating immune cell targeting and increasing antigen loading. Here, we present proof of concept analyses for the consideration of GNP-LLO91-99 nanovaccines as a novel immunotherapy for cutaneous melanoma. Studies using mouse models of subcutaneous melanoma indicated that GNP-LLO91-99 nanovaccines recruite and modulate dendritic cell (DC) function within the tumour, alter tumour immunotolerance inducing melanoma-specific cytotoxic T cells, cause complete remission and improve survival. GNP-LLO91-99 nanovaccines showed superior tumour regression and survival benefits, when combined with anti-PD-1 or anti-CTLA-4 checkpoint inhibitors, resulting in an improvement in the efficacy of these immunotherapies. Studies on monocyte-derived DCs from patients with stage IA, IB or IIIB melanoma confirmed the ability of GNP-LLO91-99 nanovaccines to complement the action of checkpoint inhibitors, by not only reducing the expression of cell-death markers on DCs, but also potentiating DC antigen-presentation. We propose that GNP-LLO91-99 nanovaccines function as immune stimulators and immune effectors and serve as safe cancer therapies, alone or in combination with other immunotherapies.
Introduction
Cutaneous melanoma is probably the most common solid tumour, which originates from melanocytes, exhibits multidrug resistance and has a low survival rate. The annual incidence of melanoma continues to grow in the European Union at the rate of 3–7% per year.Citation1 Melanoma is also the best example of an immunological “hot” tumour as it is heavily infiltrated by immune cells and can be treated with immunological checkpoint inhibitors such as anti-CTLA-4 or anti-PD-1/PD-L1 antibodies that block T-cell-negative regulators.Citation2,Citation3 These new immunotherapies approved by the FDA for advanced melanoma, mainly stages IIIB or IV, are not effective in all melanoma cases due to a low number of responders and other effects that minimize their success, such as the high rate of immunological adverse events, the appearance of immunotherapy resistance or the lack of benefit on long-term survival.Citation2-Citation6
Immunotherapies for solid tumours, which also attenuate bacterial mutants of the human pathogen Listeria monocytogenes (LM) lacking the C-terminal of the bacterial toxin listeriolysin O (LLO), have been widely used in prostate cancer, cervix carcinoma and even pancreatic cancer.Citation7,Citation8 However, cancer patients are immunocompromised individuals and caution is necessary when using attenuated Listeria mutants in cancer patients.Citation9 The main virulence factor of this pathogen, LLO, appears to be responsible for many biological activities related to the ability of LM as anti-tumour therapy such as lower concentrations required in vivo to induce apoptosis than when acting as a bacterial cytolytic toxin, the recruitment of DCs, binding to membranes, the induction of cytotoxic T cell responses and tumour homing.Citation10-Citation13 These LLO properties explain the very low doses of pathogenic LM which disable the immune tolerance of tumours and cause regression of experimental melanoma, while mutants deficient in the gene coding LLO, failed to serve as anti-melanoma therapy.Citation12 To avoid the use of pathogenic LM, but to focus on LLO-based therapies, we identified LLO peptides that can cause melanoma regression and studied the anti-neoplastic properties of the 91–99 peptide of LLO (LLO91–99) to prevent adhesion and dissemination of experimental melanoma-induced carcinomatous peritonitis as adjuvant therapy, either using DCs loaded with this peptideCitation14 or gold nanoparticles (GNPs) loaded with LLO91–99 peptide and β-D-glucose.Citation15 GNPs can be loaded with multiple copies of the desired (bio)molecules (ligands) by means of thiol chemistry,Citation16 and depending on the chosen ligands, can be used to intervene in pathological processes such as metastasis,Citation17 cancer,Citation18-Citation20 bacterial infection,Citation21-Citation23 HIV infectionCitation24,Citation25 and listeriosis.Citation26-Citation28 Thus, we hypothesized that GNPs could also be favourable alternatives to DC-LLO91–99 vaccines and therapies against solid tumours.
In the present study, we evaluated the therapeutic activity of GNP-LLO91-99 nanovaccines as safe immunotherapies for cutaneous melanoma using subcutaneous transplants of primary or metastatic murine melanoma. We also tested, as a proof of concept, GNP-LLO91-99 nanovaccines in combination with immunological checkpoint inhibitors in mouse models and monocyte-derived DCs (MoDC) from melanoma patients.
Results and discussion
Since Coley’s treatment of cancer with bacterial vaccines to boost the immune system against host tumours, and the approved Bacillus Calmette-Guerin (BCG) vaccine for bladder cancer, the immunotherapy field has grown enormously. In this regard, immunological checkpoint inhibitors or LM-based immunotherapies using attenuated LM are two examples of cancer therapies. Several studies have suggested that melanoma might be a good target for LM-based immunotherapies, using either low doses of pathogenic LM, or attenuated LM vaccines expected to lack virulence and cytolysin ability.Citation12,Citation13,Citation29,Citation30 However, the development of severe systemic listeriosis due to the use of one of these attenuated LM vaccines in a cancer trial,Citation9 and significant increases in the annual incidence of listeriosis in several European countries, particularly Spain,Citation31,Citation32 strongly suggest the need to engineer safer LLO-based cancer immune therapies.
We present pre-clinical and proof of concept studies of a novel LM-based nanotherapy for cutaneous melanoma using gold nanoparticles (GNPs) coupled to both β-D-glucose and the 91-99 peptide of LLO, i.e. GNP-LLO91-99 nanovaccines. We studied GNP-LLO91-99 nanovaccines as either monotherapy or combination therapy with immunological checkpoint inhibitors, as a viable alternative to DC cancer vaccines against cutaneous melanoma.Citation33-Citation36
Preparation of GNP-LLO91-99 nanovaccines and cell targeting characterization
GNP-LLO91-99 nanovaccines are synthetic particles with a nanoscale gold core, which maximises antigen loading and provides homogeneous structure and size (chemical structure in Figure S1, panel A and detailed procedure in Supplemental file). This immunotherapy is non-toxic, both in vivo using C57BL/6 mice and in vitro using human monocyte derived DCs (MoDC) (Figure S1, panel B). Similar to pathogenic LM, GNP-LLO91-99 nanovaccines have tumour and DC targeting abilities, as shown by co-localization of GNP-LLO91-99 and MHC-I molecules within intracellular compartments (yellow fluorescent images in Figure S1, panel C). The perinuclear localization of MHC-I and GNP-LLO91-99 containing compartments, appears to correspond with antigen cross-presentation organelles (Figure S1, panel C and single staining shown in Figure S2). We also observed another GNP-LLO91-99 and MHC-I co-localization pattern in cell surface spots of melanoma (left images in Figure S1, panel C), showing that GNP-LLO91-99 nanovaccines induce different signals in tumours and DCs.
Tumour effects in vivo after treatment with GNP-LLO91-99 nanovaccines
On confirmation of the melanoma targeting ability of these nanovaccines (Figure S1, panel C), we investigated the anti-melanoma efficacy of GNP-LLO91-99 nanovaccines. The therapeutic effect on primary B16OVACitation37 or metastatic B16.F10 melanoma started when the subcutaneous (s.c) melanoma size reached 400 m3 on day 7 (Figure S3, panel A) and tumours had a consistent and visible structure (Figure S3, panel B). B16.F10 melanoma resulted in a lower number of surviving mice and larger tumours which appeared earlier than B16OVA melanoma, as expected in metastatic tumours (Figure S3, panels A-C). Treatment was administered intravenously (i.v) as a single dose of GNP-LLO91-99 nanovaccines (50 µg/mouse, n = 10 mice/group). One week later, we assessed tumour size, lung metastases and immune responses in tumour infiltrated lymphocytes (TILs) and spleens (protocol in ). GNP-LLO91-99 nanovaccines caused regression of melanoma auto- and allo-transplants and reduced the tumour volumes of B16.F10 and B16OVA melanoma in C57BL/6 congenic and CD-1 allogenic mice by 97–98% ( and Table S1). GNP-LLO91-99 nanovaccines also inhibited the formation of lung metastasis () and induced lasting responses, as the mice demonstrated 100% survival rates (SR) (grey squares in )) and remained healthy up to 30 days or longer (Table S1). Only 2–3% of non-treated (NT) animals survived up to day 30 (plot with black circles in ) and showed 10-fold higher tumour volumes than the average initial size (day 30 column labelled TV in Table S1).
Figure 1. Efficacy of GNP-LLO91-99 therapy on melanoma regression, metastases and mouse survival. (a) Scheme of GNP-LLO91-99 therapy. B16OVA melanoma cells were auto-transplanted s.c into the right hind flanks of female C57BL/6 mice. Seven days later, the mice were inoculated i.v with a single dose of GNP-LLO91-99 (50 µg/mouse) nanotherapy. Seven days post-nanotherapy, the mice were examined, blood obtained, serum stored for evaluation of cytokine concentrations and the mice were then killed. Spleens were removed to measure general immune responses. Melanomas were homogenized, filtered and centrifuged in Ficoll gradients to isolate TILs in the interphase and melanoma (MEL) in pellets. (b) B16OVA melanoma auto-transplants established s.c (n = 10/group of mice, left plots) were inoculated i.v or not (NT) with a single dose of the following therapies: LLO91-99 or LLO189-201 peptides (50 µg/mouse), control GNP nanovaccines coated with glucose (50 µg/mouse), GNP-LLO91-99 (5 or 50 µg/mouse), GNP-GAPDH1–22 (50 µg/mL) or DC-LLO91-99 (106 cells/mouse). Melanomas were removed and measured with a calliper. Tumour volumes (mm3) are expressed as the mean ± SD. Right images correspond to B16OVA melanoma s.c allo-transplants established in P4 neonates of CD1 mice. After 4 days, the mice were treated in situ with 50 µg/mouse of the following therapies: GNP-LLO91-99, control GNP, LLO91-99 peptide, GNP-GAPDH1-22 or DC-LLO91-99 (106 cells/mouse). (c) The number of lung metastases in mice in B were quantified. The results are expressed as the mean lung metastases ± SD. (d) B16OVA melanoma was s.c auto-transplanted into the right hind flanks of C57BL/6 mice for 7, 14, 23 or 30 days (n = 10/group of mice) and i.v inoculated or not (NT) with a single dose of the following therapies: control GNP nanovaccines coated with glucose (50 µg/mouse), GNP-LLO91-99 (50 µg/mouse), DC-GNP-LLO91-99 (106 cells/mouse), DC-GNP-GAPDH1-22 (106 cells/mouse) or DC-LLO91-99 (106 cells/mouse). The number of surviving mice was counted on day 7, 14, 23 or 20 post-vaccination. Asterisk highlights that plots of DC-GNP-LLO91-99 and GNP-LLO91-99 mice groups, completely overlap. Survival rates (SR) are expressed as the mean ± SD (P ≤ 0.05). Mice survivors of GNP-LLO91-99 groups were separated and maintained for at least 3 months to check for any tumours, or clinical manifestations. Afterwards, mice were scarified and check internally for any tumour metastasis. All survivors remained healthy and without tumours, suggesting tumours cured.
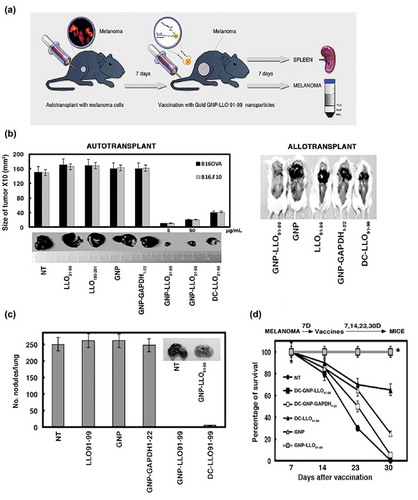
GNP-LLO91-99 nanovaccines showed higher overall therapeutic efficacy than DC-LLO91-99 vaccines, which resulted in a maximum 72% reduction in tumour volume () and Figure S3, panel D), 80% inhibition of metastases () and 60% SR (black triangles in ). Moreover, GNP-LLO91-99 anti-tumour effects were LLO 91-99 peptide specific, as empty GNPs, soluble LLO 91-99 or 189–201 peptides or other LM-based nanovaccines effective in listeriosis prophylaxis,Citation27,Citation28 provided no perceived benefit on tumour growth, metastases, SR or mouse health ()).
GNP-LLO91-99 nanovaccines induce cell death due to immune activities
The blocking of tumour growth by GNP-LLO91-99 nanovaccines also suggested the induction of cell death. In this regard, histochemical analysis of B16OVA and B16.F10 melanoma from mice treated with GNP-LLO91-99 nanovaccines showed high numbers of necrotic foci following haematoxylin-eosin (HE) staining ()). Using FACS analysis, early apoptosis was characterized as single annexin-V+ positive cells and late apoptosis as double 7-AAD+ and annexin-V+ positive cells. Melanoma from transplanted mice indicated that GNP-LLO91-99 nanovaccines increased significantly late apoptosis of primary and metastatic melanoma in vivo, from 45–50% to 73–76%, respectively (), an asterisk indicates a significant induction with nanovaccines, compared to NT). Also, nanovaccines enhanced early apoptosis of metastatic B16.F10 melanoma in vivo, from 9% to 17% (), marked with an asterisk in the NT groups); while caused no significant effect in primary B16OVA melanoma. If nanovaccines are used directly in melanoma (B16OVA, B16.F10, A-375) or other tumours (ovary CHO cells or macrophage-derived cells as IC-21) in vitro, they only induced very low percentages of early apoptosis, less than 10% (single AnnV+ cells) (black bars in lower plot of ). However, if nanovaccines are previously pre-incubated with mouse bone-marrow derived DC for 16 hours, and, next supernatants incubated with melanoma (B16OVA, B16.F10, A-375) or other tumours (ovary CHO or macrophage-derived IC-21 cells), they induce high percentages of early apoptosis in vitro, from 50 to 55% (grey bars in ). Therefore, it seems that nanovaccines induce cell death due to immune activities.
Figure 2. GNP-LLO91-99 nanotherapy affects melanoma programmed cell-death and antigen-presentation markers. (A, B) B16OVA melanoma auto-transplants were established s.c as in (n = 10/group of mice) and vaccinated i.v with control GNP (50 µg/mouse), GNP-LLO91-99 (50 µg/mouse) or non-treated (NT). After 7 days, histochemistry and immunohistochemistry were performed on melanoma embedded in paraffin to calculate the percentage of necrosis (A) or stained (B) with haematoxylin-eosin (HE). Asterisks over NT bars indicate that all groups of mice were compared to NT. The results are expressed as the mean number of necrotic foci ± SD (P ≤ 0.05). In (B), we show representative images of each group of mice stained with HE and mark with an asterisk, the NT group to which we compared all samples. (C) Removed melanoma were analysed for apoptosis by FACS analysis. We measured levels of early apoptosis using single staining with the apoptotic marker annexin-V (Annexin V) or late apoptosis using double staining with the DNA marker 7-AAD and the apoptotic marker annexin-V. Upper plots labelled as in vivo correspond to a representative experiment and the percentages reflect the cells localized in each quadrant from a total of 50.000 cells passed by the flow cytometer. GNP-LLO91-99 treated samples are compared to NT and significant differences are marked with an asterisk (*). The experiment was performed three times and results of each group of 10 mice are the following for late apoptosis (7-AAD+AnnV+ double positive cells): B16OVA/GNP-LLO91-99, 73 % ± 0.5; B16OVA/NT*, 50 % ± 1.5; B16.F10/GNP-LLO91-99, 76 % ± 1.5; B16.F10/NT*, 45 % ± 1.0. Results for early apoptosis (7-AAD−AnnV+) are: B16OVA/GNP-LLO91-99, 7 % ± 0.5; B16OVA/NT, 13 % ± 1.0; B16.F10/GNP-LLO91-99, 17 % ± 1.5; B16.F10/NT, 9 % ± 0.5 (P ≤ 0.05). We also performed in vitro experiments of different tumour cells (lower bar plot), melanoma (B16OVA, B16.F10, A-375), ovary CHO or macrophage IC-21 cell lines treated for 16 h with GNP-LLO91-99 (50 µg/ml) (black bars) or supernatants of DC cells pre-treated with GNP-LLO91-99 (50 µg/ml) (grey bars) and, next, analysed for early apoptosis by FACS. Results correspond to the mean of five different experiments and are expressed as the mean percentage of positive cells ± SD (P ≤ 0.05). (D) Analysis of antigen-presentation and cell-death markers on the cell surface of different melanoma cell lines, either murine B16OVA or human A375 as primary melanoma or murine B16.F10 or human MeWo as metastatic melanoma, were treated in vitro with GNP-LLO91-99 nanovaccines (50 µg/mL, 16 h, 37ºC) (+ GNP bars). Cells were stained with different fluorescent-labelled antibodies and examined by FACS analysis. The results are expressed as the mean percentage of positive cells ± SD (P ≤ 0.05).
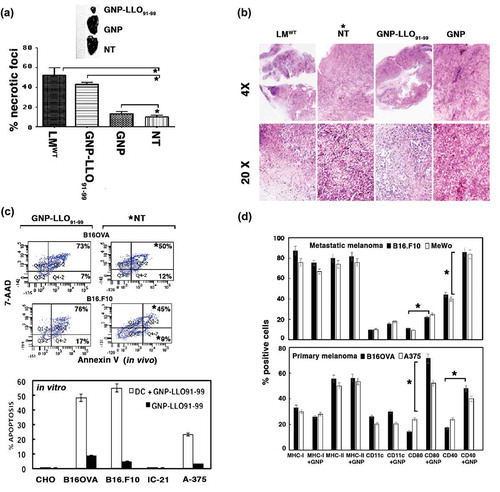
GNP-LLO91-99 nanovaccines also caused significant increases in classic surface markers involved in immune activation and antigen presentation such as CD40 and CD80 (), suggesting that this treatment activated the immune system in primary and metastatic melanoma. The following findings support that GNP-LLO91-99 nanovaccines activate the immune response in melanoma and disable immune tolerance: First, the high numbers of infiltrating DCs observed in transplanted melanoma surrounding the necrotic foci (CD123 brown staining in images of ). Second, the high percentages of activated DCs (84–87%) detected in the tumour infiltrated DC (Ti-DC) with the following phenotype, CD11c+CD8α+iNOS+CD40+CD83+CD86+CD11blow (plot in ). These activated CD11c+CD8 α+ DCs were reported as the cellular targets of IFN-α, responsible for tumour regression and drivers of cytotoxic T cell immunity in tumours.Citation38-Citation40 Third, GNP-LLO91-99 nanovaccines shifted the Th2 cytokine profile to the Th1 cytokine profile in vivo after transplantation with primary B16OVA or metastatic B16.F10 melanoma, increasing the serum levels of Th1 cytokines with anti-tumour potential such as IFN-γ, TNF-α and IL-12, and decreasing Th2 cytokines such as IL-6 and IL-10 (). A classic Th2 profile was detected in mice transplanted with melanoma and in patients with primary or metastatic melanoma (Table S2).Citation15,Citation41 Fourth, GNP-LLO91-99 nanovaccines caused a 5-fold reduction in T regulatory cells (Treg; CD4+CD25+FoxP3+) () and a 2-fold reduction in the percentage of myeloid derived suppressor cells (MDSC; CD11b+Gr1+) (Figure S3, panel E) in TILs; otherwise present at a high proportion in TILs of mice transplanted with melanoma and not receiving any therapy (NT plots in TILs of ) and B16F10 and B16OVA bars in Figure S3, panel E). Fifth, GNP-LLO91-99 nanovaccines also resulted in a 3 to 5-fold increase in the frequency of cytotoxic CD8+ T cells specific for the melanoma epitope OVA257-264 and for the antigen LLO91-99 epitope (B16OVA melanoma) (black and grey bars in plots of ) and a 4-fold increase in the total percentages of CD8+ melanoma specific and IFN-γ producers (B16.F10 melanoma in Figure S3, panel F), with no significant changes in the percentages of CD4+ melanoma specific and IFN-γ producers. We conclude that GNP-LLO91-99 nanovaccines induced a robust LLO and melanoma-specific cytotoxic T cell response, had a concerted action on DCs and CD8+ T cells, disabled the immune tolerance of tumours by increasing the levels of tumoricidal cytokines, and reduced the percentages of negative immune regulators such as Treg or MDSC to cause melanoma cell apoptosis.Citation42
Table 1. Cytokine pattern in mice auto-transplanted with melanoma and treated with GNP-LLO91-99 nanovaccines.
Figure 3. GNP-LLO91-99 nanotherapy as an immune effector: analysis of TILs, spleens and cytokines in sera. (a) B16.F10 and B16OVA melanoma auto-transplants were established s.c as in - (n = 10/group of mice) and then i.v inoculated or not (NT) with a single dose of GNP-LLO91-99 (50 µg/mouse). After 7 days, melanomas were removed and TILs isolated. TILs were analysed by FACS and tumour infiltrated (Ti)-DCs with CD11c+CD8α+ phenotypes examined for different markers with specific monoclonal antibodies. Data in the plots correspond to B16.F10 melanoma and images correspond to melanoma embedded in paraffin and stained with anti-CD123 to stain DCs. We also quantified the amount of CD123+ cells in recovered melanoma by FACS, marked with an asterisk, and detected the following percentages: untreated B16.F10, 10% ± 0.9; GNP-LLO91-99 treated B16.F10, 87% ± 1.3; untreated B16OVA, 9% ± 0.6; GNP-LLO91-99 treated B16OVA, 84% ± 1.2. The results are expressed as percentages of positive cells ± SD (P ≤ 0.05). (b) Treg cell populations in spleens and melanoma were analysed by FACS. GNP-LLO91-99 treated samples are compared to NT samples and marked with an asterisk (*) to indicate significant decreases. These experiments have been performed five times. The summarizing data of percentages of CD4+FoxP3+ positive cells (Treg) are the following in the spleens: NT group: 16 % ± 2.0 and GNP-LLO91-99 group: 14.4 % ± 1.6. While Treg percentages in melanoma are: NT group: 18% ± 1.5 and GNP-LLO91-99 group: 3.6 % ± 0.4 (P ≤ 0.05). (c) CTL activities specific of each peptide were examined with the frequencies of LLO91-99 or OVA257-264 peptide-specific CD8+ cells and IFN-γ producers in TILs. We examined these specific CTL activities using recombinant soluble dimeric mouse H-2b: Ig fusion protein that bind to each peptide, as described in the Methods and materials section. The results are expressed as percentages of positive cells ± SD.
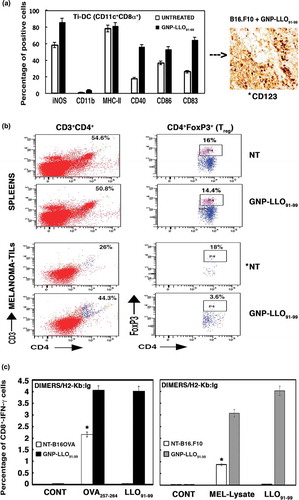
Proof of concept for GNP-LLO91-99 nanovaccines as immunotherapy for melanoma
The actions of GNP-LLO91-99 nanovaccines (–) strongly suggest that they are valid immunotherapies for cutaneous melanoma. To determine the proof of concept, we compared the effects of nanovaccines in vivo with anti-CTLA-4 and anti-PD-1 antibodies,Citation5,Citation43 using the same doses, adjusted for mouse weight, and route of administration used for metastatic melanoma patients.Citation5,Citation6 Mice s.c transplanted with B16OVA melanoma were i.v inoculated with a single dose of anti-CTLA-4 antibody, anti-PD-1 antibody (100 µg/mouse) or GNP-LLO91-99 nanovaccines (50 µg/mouse) and the animals were analyzed 14, 23 or 30 days after treatment (14D, 23D, 30D bars in ). GNP-LLO91-99 treatment was found to be the most efficient monotherapy with rapid and long lasting effects on tumour burden, showing 4-fold and 8-fold reductions, respectively (), and the only monotherapy to improve SR, as all mice survived and remained healthy up to 30 days (black circles in ). Anti-CTLA-4 treatment reduced tumour size 4-fold in a short time, and did not have a lasting effect (grey and white bars in ). Anti-PD-1 antibody had the opposite effect on tumour size, had a long lasting effect with an 8-fold reduction in tumour size and a less prominent reduction within a short time (white bars in ). Anti-CTLA-4 treatment had no benefit on SR or mouse health as most of the mice did not survive after 23 days († labelled white bars in ) and black triangles in ). However, anti-PD-1 therapy improved SR but deteriorated after 14 days (white squares in ). In combination immunotherapy, GNP-LLO91-99 nanovaccines synergised anti-PD-1 treatment to achieve long lasting effects at 14 days and beyond, obtained complete tumour remission (asterisk bars in ) and resulted in 100% SR with sustained lasting effects (similar black quadrangle and circle plots in ). The combination of GNP-LLO91-99 nanovaccines with anti-CTLA-4 treatment also had some benefit on tumour regression () with an 85% SR at 23 days and beyond (white triangles in ). Therefore, GNP-LLO91-99 nanovaccines appear to be efficient immunotherapies for melanoma regression, with significant benefits on SR, and indicated the time-frame where each checkpoint inhibitor failed. In this regard, GNP-LLO91-99 nanovaccines in combination with anti-PD-1 antibodies improved the short-term effects and in combination with anti-CTLA-4 antibodies improved the long-term effects.
Figure 4. Pre-clinical studies with GNP-LLO91-99 nanovaccines as immunotherapies in combination with checkpoint inhibitors in mice and melanoma patients. (a) B16OVA melanoma auto-transplants established as in (n = 10/group of mice) were i.v vaccinated or not (saline) with a single dose of GNP-LLO91-99 (50 µg/mouse) alone or in combination with anti-CTLA-4 or anti-PD-1 (100 µg/mouse every two days). At 7, 14, 23 or 30 days post-transplantation, melanomas were removed and measured with a calliper. Tumour volumes were expressed as the mean of mm3 ± SD. (b) The number of surviving mice in (a) was counted at days 7, 14, 23 or 30 post-vaccination. GNP-LLO91-99 and anti-PD-1 + GNP-LLO91-99 results completely overlaped (mark with an asterisk *). Survival rates (SR) are expressed as the mean percentages ± SD. Mice survivors of GNP-LLO91-99 groups were separated and maintained for 20 months to check for any tumours, or clinical manifestations. Afterwards, mice were scarified and check internally for any tumour metastasis. All survivors remained healthy and without tumours, suggesting tumours cured. (c) CTL activities specific of each peptide were examined with the frequencies of LLO91-99 or OVA257-264 peptide-specific CD8+ cells and IFN-γ producers in TILs as in , in mice non-treated (NT), GNP-LLO91-99, anti-CTLA-4 or anti-PD-1 treated or GNP-LLO91-99 treated in combination either with anti-CTLA-4 or anti-PD-1. We examined these specific CTL activities using recombinant soluble dimeric mouse H-2b: Ig fusion protein that bind to each peptide, as described in the Methods and materials section. The results are expressed as percentages of positive cells ± SD. (d) Ex vivo differentiated MoDC from a stage IIIB melanoma patient with the following phenotype CD45+CD11c+CD14− were incubated or not (CONT) with GNP-LLO91-99 (50 µg/mL), nivolumab (100 µg/mL), ipilimumab (100 µg/mL) or a combination of GNP-LLO91-99 with nivolumab or ipilimumab for 48 h, at the same concentrations as the monotherapies. Antigen-presentation (MHC-I, MHC-II, CD80 and CD86) and cell-death (PD-1, PD-L1 and Annexin-V) surface markers were analysed by FACS using specific monoclonal antibodies. The results are expressed as the mean percentages of positive cells ± SD (P ≤ 0.05). (e) Different melanoma cell lines, either primary melanoma as A-375 or MelJuSo, or metastatic melanoma as MeWo or SK-Mel24 were incubated with 50 µg/ml of GNP-LLO91-99 or ½ supernatants of ex vivo differentiated MoDC from a stage IIIB melnanoma pre-treated for 16 hours with 50 µg/ml of GNP-LLO91-99. Next, early apoptosis was examined by FACS as the percentages of positive cells for annexin V. (f) Model of the action of GNP-LLO91-99 nanovaccines as immunotherapies. GNP-LLO91-99 stimulates the priming and effector arm of immune responses: (1) as immune stimulators, they activate intra-tumoural DCs, as well as DCs in other locations to release cytokines with anti-tumoural capacities such as IL-12 and TNF-α and (2) as immune effectors, they recruit and potentiate the antigen-presentation abilities of DCs to TILs, inducing the activation of antigen- and melanoma-specific CD8+ T cells causing tumour necrosis and diminishing the amount of Treg in TILs. Therefore, dormant specific anti-melanoma immune responses in TILs were stimulated.
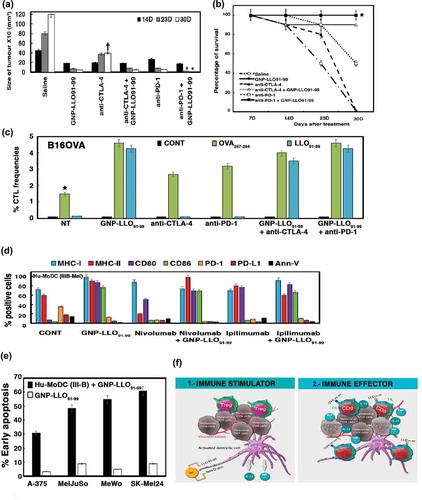
Checkpoint inhibitors can also modulate the antigen-presentation capacities of DCs within the melanoma environment and have been approved by the FDA for patients with stage IIIB and IV melanoma.Citation6,Citation41,Citation44 Therefore, we isolated TILs from mice transplanted s.c with B16OVA melanoma, treated or not with GNP-LLO91-99 nanovaccines or checkpoint inhibitors, and evaluated antigen-presentation markers in DCs such as MHC-I and MHC-II molecules, or the co-stimulatory molecules, CD40, CD80, CD83 and CD86 (Figure S3, panel G). As expected, anti-CTLA-4 and anti-PD-1 antibodies had no effect on antigen-presentation markers in Ti-DCs (CD8α+ cells), as their action is focussed on T cell proliferation.Citation5,Citation6,Citation45 Anti-PD-1 antibodies had a negative effect on the percentages of MHC-II molecules and a small effect on CD80 and CD83, whereas they had no effect on the cell surface expression of CD40 and CD86 molecules (anti-PD-1 and anti-CTLA-4 bars in Figure S3, panel G). However, GNP-LLO91-99 nanovaccines clearly affected all antigen-presentation markers, causing a 2.5-fold increase in the percentages of co-stimulatory molecules, CD40, CD80, CD83 and CD86, either as monotherapy or combination therapy with anti-CTLA-4 or anti-PD-1 antibodies (GNP-LLO91-99 bars in Figure S3, panel G).
We next confirmed the effect of GNP-LLO91-99 in combination with checkpoint inhibitors expanding CTL activities, when we measured melanoma and antigen specific CD8+ frequencies in melanoma TILs. Anti-CTLA-4 or anti-PD-1 only caused a 2-fold increase in the frequency of cytotoxic CD8+ T cell specific for the melanoma epitope OVA257-264 and IFN-γ producers, and, no increased for the antigen LLO91-99 (green and blue bars in ). While we detected a 5-fold increase in the frequency of cytotoxic CD8+ T cells specific for melanoma using GNP-LLO91-99 nanovaccines as monotherapies or in combination with anti-CTLA-4 or anti-PD-1.
We then assessed the effect of GNP-LLO91-99 nanovaccines as potential immunotherapy for patients with cutaneous melanoma, compared with the checkpoint inhibitors, ipilimumab (anti-CTLA-4 antibodies) and nivolumab (anti-PD-1 antibodies), examined the percentages of antigen-presentation and programmed cell-death markers in monocyte-derived DCs (Hu-MoDC) from a stage IIIB melanoma patient who had not received immunotherapy (). Nivolumab and ipilimumab had no effect on the percentages of CD86 molecules, induced a small increase in the percentage of MHC-I and CD80 molecules and reduced the percentages of programmed cell-death markers, PD-1, PD-L1 and annexin-V. In addition, nivolumab reduced the percentages of MHC-II molecules (nivolumab and ipilimumab bars in . GNP-LLO91-99 nanovaccines significantly increased the percentages of all antigen-presentation markers such as MHC-I, MHC-II, CD80 and CD86 and decreased the percentages of PD-1, PD-L1 and annexin-V, the programmed cell-death markers to basal levels (orange, maroon and black bars in ). We also observed similar action of GNP-LLO91-99 nanovaccines in MoDC from patients with early stage melanoma (stages IA or IB) (). We also confirmed that the incubation of GNP-LLO91-99 with MoDC from a stage IIIB melanoma patient was able to induce cell death in different human melanoma, either primary (A-375 or MelJuSo) or metastatic melanoma cell lines (MeWo or SK-Mel24) due to immune activities, since GNP-LLO91-99 alone caused only basal level of early apoptosis (). All together, these results confirmed that GNP-LLO91-99 action is not restricted to the Ti-DC(CD8α+) of transplanted melanoma, but also increased immune activation and the antigen presentation abilities of MoDC in melanoma patients at primary or advanced stages () to promote tumour cell death (). Moreover, this ability of GNP-LLO91-99 nanovaccines was also observed in combination with checkpoint inhibitors that lack these stimulatory capacities (+ GNP-LLO91-99 bars in ), suggesting that this approach can also serve as a tool to confirm the putative action of immunotherapies.
Table 2. GNP-LLO91-99 nanovaccines as human immunotherapies in comparison to check point inhibitors using MoDC.
Conclusions
We propose that GNP-LLO91-99 nanovaccines are safe immunotherapies, which act in the priming and efferent arms of the immune response, disable immune tolerance in melanoma (model in ) and can benefit melanoma patients in the early and late stages of disease as follows: First, as an immune stimulator, GNP-LLO91-99 nanovaccines recruit DC-CD8α+ within the tumour environment, which produce Th1 cytokines with tumour killing potential such as IFN, TNF-α and IL-12, and improve DC antigen-presentation abilities. Second, GNP-LLO91-99 nanovaccines activate DCs and increase the intratumoural percentages of anti-Listeria and melanoma-specific cytotoxic T cells and IFN-γ producers, and, reduce Treg and MDSC suppressor cells. These two concerted actions of GNP-LLO91-99 nanovaccines induce melanoma cell apoptosis which restricts tumour growth and increases mouse survival. The ability of GNP-LLO91-99 nanovaccines to promote MoDC antigen-presentation abilities and synergise with ipilimumab and nivolumab in stage IA, IB and IIIB melanoma patients consolidates GNP-LLO91-99 nanovaccines as immunotherapies with extensive action. We propose that GNP-LLO91-99 nanovaccines are a promising nano-immunotherapy that can improve several immune parameters described in the proposed “cancer immunogram”Citation46 such as: (1) induction of a strong immune status activating all DCs, (2) promotion of immune cell infiltration by cytotoxic T cells and CD8α+DCs, (3) decrease the expression of PD-1 and PD-L1 immune checkpoints, (4) reduce IL-6 and IL-10 soluble Th2 inhibitors and (5) improve tumour sensitivity to immune effectors by increasing the antigen-presentation capacities of DCs and anti-melanoma cytotoxic cells.
GNP-LLO91-99 anti-melanoma abilities seem to be comparable to the STINGVAXCitation47,Citation48 or VACCIMELCitation49,Citation50 vaccines proposed for melanoma, but with several advantages: (i) a synthetic nanostructure with a globular and gold core vs. irradiated allogenic tumour cells in the above-mentioned vaccines, which avoids systemic inflammatory reactions and immune adverse effects; (ii) the anti-neoplastic and cytotoxic abilities of the LLO91-99 peptide enhance anti-melanoma specific cytotoxic T cell responses with no risk when including live bacteria as in the case of VACCIMEL, which includes BCG;Citation49,Citation50 (iii) improves DC antigen-presenting abilities, being a useful adjuvant therapy alone (43) or in combination with anti-PD-1 and anti-CTLA-4 immunotherapies and (v) a simple diagnostic tool to identify melanoma patients who may benefit from these treatments.
Materials and methods
B16OVA transplants and GNP-LLO91-99 therapy, alone or in combination with anti-ctla-4 or anti-pd-1 antibodies
B16OVA or SM1-OVA cells were transplanted into 8 to 12-week-old female C57BL/6 mice (autotransplants) and P4 newborn CD-1 mice were transplanted with B16OVA (allotransplants) by a single subcutaneous injection (106 cells) in a volume of 100 µL (n = 10/group). At 7 days, the melanoma transplanted mice received or not (NT) a single intravenous (i.v.) injection of GNP-LLO91-99 therapy (50 µg/mouse) alone (n = 10/group) or in combination with anti-CTLA-4 or anti-PD-1 antibodies (100 µg/mouse every two days), DC-LLO91-99 vaccines (106 cells), control GNP coated with glucose (GNP, 50 µg/mouse), anti-CTLA-4 or anti-PD-1 (clone RPMI-14) antibodies (100 µg/mouse every two days) or saline (NT, untreated mice). Allotransplants were established subcutaneously (s.c) in P4 mice only for 4 days (n = 5) receiving GNP-LLO91-99 in situ injection for 3 days. On day 7 after injection (auto- and allo-transplants) and before the mice were killed, serum was collected and processed within 50 min and stored at −80°C for cytokine analysis. Spleens and melanomas were also collected. Melanoma size was measured using a calliper. Tumour volume (TV) was calculated using the following formula: (length x (width)2)/2 as reported previously.Citation15 The mean and SD of tumour volume per group were calculated.
Melanomas were minced, homogenized and passed through a 70-µm strainer and TILs isolated by centrifugation over a Ficoll gradient at 1.077 g/mL density (Histopaque-1077, Sigma-Aldrich, St. Louis, MO, USA). We recovered TILs in the interphase gradient, while collecting melanoma cells in pellets.Citation14
Patients
Stage I and III cutaneous melanoma patients (HUMV-1/IIIB, HUMV-2/IA, HUMV-3/IB and HUMV-4/IA) diagnosed during the period 2012–2015 at the Dermatology Department were included in the study before receiving any interferon adjuvant treatment or having received interferon at least 5 years before initiation of this study. Stage IV cutaneous metastatic melanoma patients (ONCM-1, ONCM-2, ONCM-3) diagnosed during the same period at the Medical Oncology Department were included in the study before enrolling in any treatment. Patients participated in the study voluntarily and signed an informed consent at the physician consultation and received an Information Document on the research study. Patients were able to revoke the informed consent at any time. Blood samples were collected in EDTA-tubes at the Dermatology or Medical Oncology Departments of our institution (HUMV, Hospital Universitario Marqués de Valdecilla, Santander, Spain) on the day of patient consultation and processed in the IDIVAL laboratory within 2 h.
Isolation of MoDC
Peripheral blood mononuclear cells (PBMC) from cutaneous melanoma patients or healthy donors were isolated from a Ficoll gradient of whole blood cells (EDTA-containers). PBMC recovered from the interphase were washed twice in Hank´s buffered solution and cultured. In other samples, PBMC were prepared in MACSTM buffer (PBS-0.5% BSA-2 mM EDTA) with microbeads conjugated to mouse IgG2a monoclonal anti-human CD14 antibody (Miltenyi, Bergisch Gladbach, Germany) for monocyte (Mo) positive selection. CD14+ positive cells were selected using MACSTM columns (Miltenyi). FACS analysis following CD14+-MACSTM selection indicated 99% of CD45+CD14+ cells were positive. MoCD14+ cells were differentiated to MoDC at 1 × 106 cells/mL in 6-well plates (FalconTM) over 7 days using GM-CSF (50 ng/mL) and IL-4 (20 ng/mL) in RPMI-20% FCS medium. All differentiated cells were 98% CD45+CD11c+DRlowCD86−CD14− positive cells using specific monoclonal antibodies (Miltenyi).Citation15 MoDC were incubated with GNP-LLO91-99 nanovaccines (50 µg/mL), ipilimumab or nivolumab (100 µg/mL of each checkpoint inhibitor), prepared in RPMI-20% FCS medium for 48 h to determine the cell surface phenotype of activated MoDC by FACS analysis.
FACS analysis and antibodies
Spleen cell surface markers and recovered melanomas were analysed by FACS using the following antibodies for mice: anti-CD11c-PE, anti-MHCII-BV421, anti-CD8-APC, anti-CD4-PE, anti-CD11b-FITC, anti-F480-PE, anti-CD56-BV, anti-CD40-FITC, anti-CD86-APC, anti-CD83-PE, anti-mouse IFN-γ-FITC and anti-CD25-FITC, and the following antibodies for humans: anti-CD11c-PE, anti-CD45-PerCP, anti-CD14-APC, anti-DR-PE (MHC-II), anti-CD80-PerCP, anti-CD84-FITC and anti-CD86-BV (Miltenyi Biotech Inc., Auburn, CA, USA). Intracellular FoxP3 was measured by FACS using FoxP3 Staining Buffer and anti-FoxP3-PE antibody (Miltenyi Biotech Inc.). The frequencies of LLO91-99 or OVA257-264-specific CD8 T cells producing IFN-γ were measured with recombinant soluble dimeric mouse H-2Kb: Ig fusion protein (DimerX I, BD Biosciences, Palo Alto, CA, USA) as reported previously.Citation14 In brief, LLO91–99 or OVA257–264 peptides (40 μM) were pre-incubated with PE-conjugated H-2Kb: Ig (1 μM) in PBS, at 37°C for 16 h. TILs (2 × 107 cells/ml) were incubated with IFN-γ and CD8 antibodies and the staining cocktail mix described above for 10 min at 4°C. The percentages of CD8+ gated cells were expressed as the mean ± SD of triplicate samples (P < 0.05). Data were analysed using FlowJo software. Mouse or human sera were used to quantify cytokines using the CBA Mouse Inflammation Kit or CBA Human Inflammation Kit, respectively (BD Biosciences).
Immunohistochemistry
Melanoma transplanted mice treated or not with GNP-LLO91-99 were immersed in 4% formaldehyde for 24 h. Melanomas were embedded in paraffin, processed and sections stained with hematoxylin-eosin (HE) and immunochemically analysed for antibody staining (Dako, Carpintera, CA, USA).Citation12,Citation14,Citation15 Necrotic foci were analysed and counted as described previously.Citation15
Statistical analysis
For statistical analysis, the Student’s t test was applied. P ≤ 0.05 was considered significant.
Ethics statement
This study was approved by the Ethical Committee of Clinical Research of Cantabria at Instituto de Investigación Marqués de Valdecilla (Santander, Spain) reference number 30.2012. All participants signed the informed consent documents and these documents are in the custody of physicians in accordance with Spanish Law (Ministry of Health). The study was carried out in accordance with the Guide of Care and Use of Laboratory Animals of the Spanish Ministry of Science and Innovation. The Ethical Committee of Animal Experiments of the University of Cantabria approved the protocol (Permit Number: PI-01–17) that followed Spanish legislation (RD 1201/2005). Surgery was performed under sodium pentobarbital anaesthesia, and all efforts were made to minimise suffering. This study is protected by approved patent application PCT/ES2017/0170103.
Author contributions
R C-G, H T-N, E F-C, D S-C, F R, S Y-D and C A-D designed the research, J F and J G-R performed histochemical analyses and interpreted the results, A G-C, V M-C, S Y-D, F R, R C-G and C A-D designed the immunotherapies analysis, S Y-D and F R provided the melanoma patients, obtained and had custody of the informed consents and clinical history of the patients, I G and M M synthesized the GNP-LLO91-99 and GNP controls and performed the control and toxicity analysis, R T helped with statistics, elaboration of the paper and figures, and design of the immunological assays, R C-G, H T-N and C A-D wrote the paper. R C-G, E F-C, I G, S Y-D and C A-D participated as authors in PCT patent application and recent approval PCT/ES2017/0170103.
Abbreviations
ANOVA | = | analysis of variance |
CD | = | cluster differentiation |
DC | = | dendritic cells |
LLO | = | listeriolysin O |
MDSC | = | myeloid-derived suppressor cells |
MHC | = | major histocompatibility complex |
MoDC | = | monocyte-derived dendritic cells |
NK | = | natural killer |
Treg | = | regulatory T cells |
Supplemental Material
Download Zip (5.6 MB)Acknowledgments
We are indebted to the Dermatology and Medical Oncology patients that participated in this study and to the Association Luchamos Por La Vida (LPLV) for the kind donation to support this study. We strongly appreciate the excellent technical assistance of IDIVAL personnel: M. Sierra with cells and assays, D. Merino with FACS analysis, F. Madrazo-Toca with confocal images and the personal support and preparation of patent application of P. Zorrilla. We also acknowledge the critical reviews of L. Liz-Marzan (CIC-biomaGUNE, Donostia-San Sebastian, Spain). B16OVA was kindly provided by I. Melero (CIMA, Pamplona) and SM1-OVA by A. Ribas (UCLA, CA, USA). This work has been supported in part by IDIVAL institutional grants: INNVAL15/01 (to J.F & C. A-D), INNVAL17/01 (to F.R & C. A-D), APG-27 and AIP2014/14/SAF2012-34203 (to C. A-D), National grants from MINECO, FIPSE and ISCIII: SAF2012-34203, 00-00002755-16 (to C. A-D) and DTS18-00022 (to C. A-D and F.R), private funds CI18/09 (to C. A-D and F.R) and the Department of Industry of Basque Country.
Supplementary material
Supplemental data for this article can be accessed here.
Additional information
Funding
References
- González-Vela MC, Val-Bernal JF, González-López MA, Novell M, Fernandez-Llaca H. Collision of pigmented benign tumours: a possible simulator of melanoma. Acta Derm Venereol. 2008;88:92–93. doi:10.2340/00015555-0334.
- Ribas A, Dummer R, Puzanov I, VanderWalde A, Andtbacka RHI, Michielin O, Olszanski AJ, Malvehy J, Cebon J, Fernandez E, et al. Oncolytic Virotherapy promotes intratumoral T cell infiltration and improves anti-PD-1 immunotherapy. Cell. 2017;170(6):1109–1119.e10. doi:10.1016/j.cell.2017.08.027.
- Bathia S, Tykodi SS, Thompson JA. Treatment of metastatic melanoma: an overview. Oncology. 2009;23:488–4962.
- Sharma P, Hu-Lieskovan S, Wargo KA, Ribas A. Primary, adaptive, and acquired resistance to cancer immunotherapy. Cell. 2017;168:707–723. doi:10.1016/j.cell.2017.01.017.
- Selby MJ, Engelhardt JJ, Johnston RJ, Lu L-S, Han M, Thudium K, Yao D, Quigley M, Valle J, Wang C, et al. Preclinical development of ipilimumab and nivolumab comination immunotherapy: mouse tumor models, in vitro functional studies, and cynomolgus macaque toxicology. PLoS One. 2016;11(9):e0161779. doi:10.1371/journal.pone.0161779.
- Gubin MM, Zhang X, Schuster H, Caron E, Ward JP, Noguchi T, Ivanova Y, Hundal J, Arthur CD, Krebber W-J, et al. Checkpoint blockage of cancer immunotherapy targets tumour-specific mutant antigens. Nature. 2014;515:577–581. doi:10.1038/nature13988.
- Wood LM, Guirnalda PD, Seavey MM, Paterson Y. Cancer immunotherapy using Listeria monocytogenes and listerial virulence factors. Immunol. Res.. 2008;42:233–245. doi:10.1007/s12026-008-8087-0.
- Terán-Navarro H, Calderon-Gonzalez R, Salcines-Cuevas D, Garcia I, Marradi M, Freire J, Salmon E, Portillo-Gonzalez M, Frande-Cabanes E, García-Castaño A., et al. Safety and survival with GVAX pancreas prime and Listeria monocytogenes-expressing mesothelin (CRS-207) boost vaccines for metastatic pancreatic cancer. J. Clin. Oncol. 2015; 33(12):1325–1333.
- Sacco J, Evans M, Harrington KJ, Man S, Powell N, Shaw RJ, Jones TM. Systemic listeriosis following vaccination with the attenuated Listeria monocytogenes therapeutic vaccine, ADXS11-001. Hum Vaccin Immunother. 2016;12:1085–1086. doi:10.1080/21645515.2015.1121338.
- Sun R, Liu Y. Listeriolysin O as a strong immunogenic molecule for the development of new anti-tumor vaccines. Hum Vaccin Immunother. 2013;9:1058–1068. doi:10.4161/hv.23871.
- Carrero JA, Vivanco-Cid H, Unanue ER. Listeriolysin o is strongly immunogenic independently of its cytotoxic activity. PLoS One. 2012;7(3):e32310. doi:10.1371/journal.pone.0032310.
- Bronchalo-Vicente L, Rodriguez-Del Rio E, Freire J, Calderon-Gonzalez R, Frande-Cabanes E, Gomez-Roman JJ, Fernández-Llaca H, Yañez-Diaz S, Alvarez-Dominguez C, Chakravortty D. A novel therapy for melanoma developed in mice: transformation of melanoma into dendritic cells with Listeria monocytogenes. PLoS One. 2015;10(3):e0117923. doi:10.1371/journal.pone.0117923.
- Wood LM, Peterson Y. Attenuated Listeria monocytogenes: a powerful and versatile vector for the future of tumour immunotherapy. Front Cell Infect Microbiol. 2014;4:51. doi:10.3389/fcimb.2014.00051.
- Calderon-Gonzalez R, Bronchalo-Vicente L, Freire J, Frande-Cabanes E, Alaez-Alvarez L, Gomez-Roman J, Yañez-Diaz S, Alvarez-Dominguez C. Exceptional anti-neoplastic activity of a dendritic-cell-targetted vaccine loaded with a Listeria peptide proposed against metastatic melanoma. Oncotarget. 2016;7(13):16855–16865. doi:10.18632/oncotarget.7806.
- Calderon-Gonzalez R, Terán-Navarro H, García I, Marradi M, Salcines-Cuevas D, Yañez-Diaz S, Solis-Angulo A, Frande-Cabanes E, Fariñas MC, Garcia-Castaño A, et al. Gold glyconanoparticles coupled to listeriolysin O peptide 91-99 serve as adjuvant therapy for solid tumours. Nanoscale. 2017;9(30):10721–10731. doi:10.1039/c7nr02494k.
- Marradi M, Chiodo F, Garcia I, Penadés S. Glyconanoparticles as multifunctional and multimodal carbohydrate systems. Chem Soc Rev. 2016;42:4728–4745. doi:10.1039/c2cs35420a.
- Rojo J, Díaz V, de la Fuente JM, Segura I, Barrientos AG, Riese HH, Bernad A, Penadés S. Gold glyconanoparticles as new tools in antiadhesive therapy. Chembiochem. 2004;5(3):291–297. doi:10.1002/cbic.200300726.
- Brinas RP, Sundgren A, Sahoo P, Morey S, Rittenhouse-Olson K, Wilding GE, Deng W, Barchi JJ. Design and synthesis of multifunctional gold nanoparticles bearing tumor-associated glycopeptide antigens as potential cancer vaccines. J Bioconjugate Chem. 2012;23:1513–1523. doi:10.1021/bc200606s.
- Parry AL, Clemson NA, Ellis J, Bernhard SSR, Davis BG, Cameron NR. Multicopy multivalent’ glycopolymer-stabilized gold nanoparticles as potential synthetic cancer vaccines. J Am Chem Soc. 2013;135:9362–9365. doi:10.1021/ja4046857.
- Ojeda R, de Paz JL, Barrientos AG, Martín-Lomas M, Penadés S. Preparation of multifunctional glyconanoparticles as a platform for potential carbohydrate-based anticancer vaccines. Carbohydr Res. 2007;342(3–4):448–459. doi:10.1016/j.carres.2006.11.018.
- Safari D, Marradi M, Chiodo F, Th Dekker HA, Shan Y, Adamo R, Oscarson S, Rijkers GT, Lahmann M, Kamerling JP, et al. Gold nanoparticles as carriers for a synthetic Streptococcus pneumoniae type 14 conjugate vaccine. Nanomedicine. 2012;7:651–662. doi:10.2217/nnm.11.151.
- Gregory AE, Judy BM, Qazi O, Blumentritt CA, Brown KA, Shaw AM, Torres AG, Titball RW. A gold nanoparticle-linked glycoconjugate vaccine against Burkholderia mallei. Nanomed-Nanotechnol. 2015;11(2):447–456. doi:10.1016/j.nano.2014.08.005.
- Vetro M, Safari D, Fallarini S, Salsabila K, Lahmann M, Penades S, Lay L, Marradi M, Compostella D. Preparation and immunogeniticity of gold glyconanoparticles as anti-pneumococcal vaccine model. Nanomedicine (Lond). 2017;12(1):13–23. doi:10.2217/nnm-2016-0306.
- Martínez-Ávila O, Hijazi K, Marradi M, Clavel C, Campion C, Kelly C, Penadés S. Gold manno-glyconanoparticles: multivalent systems to block HIV-1 gp120 binding to the lectin DC-SIGN. Chem Eur J. 2009;15:9874–9888. doi:10.1002/chem.v15:38.
- Martinez-Avila O, Bedoya LM, Marradi M, Clavel C, Alcami J, Penades S. Multivalent mano-glyconanoparticles inhibit DC-SIGN mediated HIV-1 trans-infection of human T cells. Chembiochem. 2009;10:1806–1809. doi:10.1002/cbic.200900294.
- Rodriguez-Del Rio E, Marradi M, Calderon-Gonzalez R, Frande-Cabanes E, Penadés S, Petrovsky N, Alvarez-Dominguez C. A gold-glyconanoparticle carrying a listeriolysin O peptide and formulated with Advax™ delta inulin adjuvant induces robust T-cell protection against Listeria infection. Vaccine. 2015;33:1465–1473. doi:10.1016/j.vaccine.2015.01.062.
- Calderon-Gonzalez R, Terán-Navarro H, Frande-Cabanes E, Ferrández-Fernández E, Freire J, Penadés S, Marradi M, García I, Gomez-Román J, Yañez-Díaz S, et al. Pregnancy vaccination with gold glyconanoparticles carrying Listeria monocytogenes peptides protects against listeriosis and brain and cutaneous associated morbidities. Nanomaterials. 2016;6:521. nano6080151. doi:10.3390/nano6080151.
- Calderon-Gonzalez R, Frande-Cabanes E, Teran-Navarro H, Marimon JM, Freire J, Salcines-Cuevas D, Carmen Fariñas M, Onzalez-Rico C, Marradi M, Garcia I, et al. GNP-GAPDH1-22 nanovaccines prevent neonatal listeriosis by blocking microglial apoptosis and bacterial dissemination.. Oncotarget. 2017;8(33):53916–53934. doi:10.18632/oncotarget.19405.
- Chandra D, Selvanesan BC, Yuan Z, Libutti SK, Koba W, Beck A, Zhu K, Casadevall A, Dadachova E, Gravekamp C. 32-Phosphorus selectively delivered by listeria to pancreatic cancer demonstrates a strong therapeutic effect. Oncotarget. 2017;8(13):20729–20740. doi:10.18632/oncotarget.15117.
- Kim SH, Castro F, Paterson Y, Gravekamp C. High efficacy of a Listeria-based vaccine against metastatic breast cancer reveals a dual mode of action. Cancer Res. 2009;69:5860–5866. doi:10.1158/0008-5472.CAN-08-3660.
- Calderon-Gonzalez R, Teran-Navarro H, Marimon JM, González-Rico C, Calvo-Montes J, Frande-Cabanes E, Alkorta-Gurrutxaga M, Fariñas MC, Martínez-Martínez L, Perez-Trallero E, et al. Biomarker tools to design clinical vaccines determined from a study of annual listeriosis incidence in northern Spain. Front Immunol. 2016;7:541. doi:10.3389/fimmu.2016.00541.
- European Food Safety Authority (EFSA). The European Union Summary report on trends and sources of zoonoses, zoonotic agents and food-borne outbreaks in 2016. EFSA Journal. 2017;15(12):5077.
- Akiyama Y, Tanosaki R, Inoue N, Shimada M, Hotate Y, Yamamoto A, Yamazaki N, Kawashima I, Nukaya I, Takesako K, et al. Clinical response in Japanese metastatic melanoma patients treated with peptide cocktail-pulsed dendritic cells. J Trans Med. 2005;3:1–10. doi:10.1186/1479-5876-3-4.
- Oshita C, Wu M-N, Guo X-F, Zhang S-H, Miao Q-F, Zhen Y-S. Dendritic cell-based vaccination in metastatic melanoma patients: phase II clinical trial. Oncol Rep. 2012;28:1131–1138. doi:10.3892/or.2012.1910.
- de Rosa F, Ridolfi L, Ridolfi R, Gentili G, Valmorri L, Nanni O, Petrini M, Fiammenghi L, Granato A, Ancarani V, et al. Vaccination with autologous dendritic cells loaded with autologous tumor lysate or homogenate combined with immunomodulating radiotherapy and/or preleukapheresis IFN-α in patients with metastatic melanoma: a randomised “proof-of-principle” phase II study. J Transl Med. 2014;12:209. doi:10.1186/1479-5876-12-209.
- Van Lint S, Wilgenhof S, Heirman C, Corthals J, Breckpot K, Bonehill A, Neyns B, Thielemans K. Optimized dendritic cell-based immunotherapy for melanoma: the TriMix formula. Cancer Immunol Immunother. 2014;63(9):959–967. doi:10.1007/s00262-014-1558-3.
- Ochoa MC, Fioravanti J, Rodriguez I, Hervas-Stubbs S, Azpilikueta A, Mazzolini G, Gurpide A, Prieto J, Pardo J, Berraondo P, et al. Antitumor immunotherapeutic and toxic properties of an HDL-conjugated chimeric IL-15 fusion protein. Cancer Res. 2013;73:139–149. doi:10.1158/0008-5472.CAN-12-2660.
- Edelson BT, Bradstreet TR, Hildner K, Carrero JA, Frederick KE, Wumesh KC, Belizaire R, Aoshi T, Schreiber RD, Miller MJ, et al. CD8α(+) dendritic cells are an obligate cellular entry point for productive infection by Listeria monocytogenes. Immunity. 2011;35(2):236–248. doi:10.1016/j.immuni.2011.06.012.
- Diamont MS, Kinder M, Matsushita H, Mashayekhi M, Dunn GP, Archambault JM, Lee H, Arthur CD, White JM, Kalinke U, et al. Type I interferon is selectively required by dendritic cells for immune rejection of tumours. J Exp Med. 2011;208:1989–2003. doi:10.1084/jem.20101158.
- Hildner K, Edelson BT, Purtha WE, Diamond M, Matsushita H, Kohyama M, Calderon B, Schraml BU, Unanue ER, Diamond MS, et al. Batf3 deficiency reveals a critical role for CD8alpha+ dendritic cells in cytotoxic T cell immunity. Science. 2008;322:1097–1100. doi:10.1126/science.1164206.
- Lauerova L, Dusek L, Simickova M, Kocák I, Vagundová M, Zaloudík J, Kovarík J. Malignant melanoma associates with Th1/Th2 imbalance that coincides with disease progression and immunotherapy response. Neoplasma. 2002;49(3):159–166.
- Galluzzi L, Vitale I, Aaronson SA, Abrams JM, Adam D, Agostinis P, Alnemri ES, Altucci L, Amelio I, Andrews DW, et al. Molecular mechanisms of cell death: recommendations of the nomenclature committee on cell death 2018. Cell Death Differ. 2018;25:486–541. doi:10.1038/s41418-017-0012-4.
- Morales-Kastresana A, Sanmamed MF, Rodriguez I, Palazon A, Martinez-Forero I, Labiano S, Hervas-Stubbs S, Sangro B, Ochoa C, Rouzaut A, et al. Combined immunostimulatory monoclonal antibodies extend survival in an aggressive transgenic hepatocellular carcinoma mouse model. Clin Cancer Res. 2013;19:6151–6162. doi:10.1158/1078-0432.CCR-13-1189.
- Budczies J, Bockmayr M, Klauschen F, Endris V, Fröhling S, Schirmacher P, Denkert C, Stenzinger A. Mutation patterns in genes encoding interferon signaling and antigen presentation: A pan-cancer survey with implications for the use of immune checkpoint inhibitors. Genes Chromosomes Cancer. 2017 Aug;56(8):651–659. doi:10.1002/gcc.22468.
- Homet-Moreno B, Zaretsky JM, Garcia-Diaz A, Tsoi J, Parisi G, Robert L, Meeth K, Ndoye A, Bosenberg M, Weeraratna AT, et al. Response to programmed cell death-1 blockade in a murine melanoma syngenic model requires costimulation, CD4, and CD8 T cells. Cancer Immunol Res. 2016;4(10):845–857. doi:10.1158/2326-6066.CIR-16-0060.
- Blank CU, Haanen JB, Ribas A, Schumacher TN. Cancer Immunology. The “cancer immunogram”. Science. 2016;352(6286):658–660. doi:10.1126/science.aaf2834.
- Dubensky TW, Kanne DB, Leong ML. Rationale, progress and development of vaccines utilizing STING-activating cyclic dinucleotide adjuvants. Ther Adv Vaccines. 2013;1:131–143. doi:10.1177/2051013613501988.
- Fu J, Kanne DB, Leong M, Glickman LH, McWhirter SM, Lemmens E, Mechette K, Leong JJ, Lauer P, Liu W. STING agonist formulated cancer vaccines can cure established tumors resistant to PD-1 blockage. Sci Transl Med. 2015;7(283):283ra52. doi:10.1126/scitranslmed.aad3106.
- Aris M, Bravo AI, Barrio MM, Mordoh J. Inoculation site from a cutaneous melanoma patient treated with an allogenic therapeutic vaccine: a case report. Front Immunol. 2015;6:144. doi:10.3389/fimmu.2015.00144.
- Aris M, Barrio MM. Combining immunotherapy with oncogene-targeted therapy: a new road for melanoma treatment. Front Immunol. 2015;6:46. doi:10.3389/fimmu.2015.00046.