ABSTRACT
The treatment of breast cancer largely depends on the utilization of immunogenic chemotherapeutics, which, as a common leitmotif, stimulate the exposure of calreticulin (CALR) on the surface of cancer cells, thereby facilitating their recognition by dendritic cells for the uptake of tumor-associated antigens and subsequent antigen cross-presentation to cytotoxic T cells. Breast cancer cells also express the calreticulin antagonist CD47, which inhibits tumor cell phagocytosis and consequently subverts anticancer immune responses. Here, we treated carcinogen-induced or transplantable mouse models of cancer by a CD47 blocking antibody that was at least as efficient as chemotherapy and that could be favorably combined with the anthracycline mitoxantrone in the context of carcinogen-induced orthotopic breast cancers. Monotherapy by CD47 blockade led to a reduction in tumor growth and an increase in overall survival. Of note, this treatment lead to a moderate depletion of M2 macrophages as well as close-to-complete elimination of regulatory T cells from the tumor bed, suggesting a strong favorable impact of CD47 blockade on the tumor microenvironment.
Introduction
Effective anticancer agents mediate their long-term effects in vivo through a dual action, namely direct anticancer effects due to their cytostatic or cytotoxic action on cancer cells plus indirect effects that are mediated by the immune system.Citation1,Citation2 Such indirect effects can be explained by the capacity of some anticancer agents to kill tumor cells in a fashion that makes the dying tumor cells recognizable to the immune system. This “immunogenic cell death” (ICD) stimulates an anticancer immune response that allows the host to control residual tumor cells.Citation3–Citation6 Tumor cell death triggered by immunogenic chemotherapeutic such as anthracyclines, cyclophosphamide and taxanes is characterized by a specific combination of cellular alterations that together form an ICD signature.Citation7–Citation10 The characteristics of ICD involve, beyond the classical hallmarks of apoptotic cell death, the premortem exposure of calreticulin (CALR) on the cell surface. CALR translocates from the endoplasmic reticulum lumen (its orthotopic location) to the surface of the plasma membrane (its ectopic location) where it serves as a de novo “eat-me” signal for dendritic cells (DC) of the immune system.Citation11,Citation12 On the contrary, another surface protein, CD47, transmits an inhibitory “don’t eat me” signal upon ligation with its receptor signal regulatory protein α (SIRPα), which is expressed primarily on phagocytic cells, including DC.Citation13 Up-regulation of CD47 may occur in breast cancer cells in a hypoxia-inducible factor 1 (HIF1)-dependent or tumor necrosis factor-NFκB-dependent fashion and potentially plays an important role in tumor cell evasion.Citation14–Citation17 Orthotopic mouse breast tumor treated with anti-mouse CD47 antibodies were reduced according to one report,Citation18 which however was not replicated in another study.Citation19
It may be speculated that ICD inducers that trigger the exposure of the “eat-me” signal CALR might be advantageously combined with an anti-CD47 monoclonal antibody that abolishes the corresponding “don’t eat me” signaling to enhance tumor antigen uptake by DC and to trigger therapeutic anticancer immune responses.Citation20 For this reason, we analyzed the effect of anti-CD47 monoclonal antibody in different breast cancer models in immunocompetent mice, including transplantable AT3 tumors and primary carcinogen-induced mammary carcinomas.
Results and discussion
CD47 blockade retards the growth of carcinogen-induced and spontaneous breast cancer
As a first approximation to explore the potential of CD47 blockade for the therapy of breast cancer, we took advantage of a hormone/carcinogen induced breast cancer model triggered by continuous stimulation of progesterone receptors by medroxyprogesterone acetate (MPA) and repeated gavage with the DNA-damaging agent dimethylbenzantracene (DMBA). This MPA/DMBA model of breast cancer is modulated by the immune system, as indicated by the fact that the mammary carcinomas that develop can be successfully treated by anthracycline-based chemotherapy unless CD8+ T lymphocytes are depleted or the formyl peptide receptor-1 (FPR1), which is important for the recruitment of DC into the tumor, is blocked.Citation21–Citation23 Importantly, such MPA/DMBA-induced breast cancers developing in immunocompetent C57Bl/6 mice express significant levels of CD47 on their cell surface as indicated by immunofluorescence staining () suggesting that they might respond to therapeutic CD47 blockade. We decided to treat recently diagnosed (palpable) MPA/DMBA-induced cancer either transiently by four systemic CD47 antibody injections (schedule I, ) or five times per week throughout the duration of the experiment (schedule II, ) to discover that both schedules tend to reduce tumor growth () and to significantly prolong the survival of mice (). As an internal control, we confirmed that both schedules caused an increase in splenic size as a mechanism-related side effect ((h–i)) because CD47 blockade leads to an enhanced sequestration of erythrocytes by the reticuloendothelial system, hence favoring splenomegaly.Citation24,Citation25 Of note, we observed that CD47 blockade and immunogenic chemotherapy with the anthracycline mitoxantrone (MTX) could be favorably combined against DMBA/MPA-induced cancers. Thus, the combination of MTX (administered once upon diagnosis of the tumors) and CD47 blockade (administered continuously every other day) led to a more pronounced tumor growth reduction than either of the two treatments alone (Fig. S1).
Figure 1. CD47 antibody retards the growth of spontaneous tumors. Medroxyprogesterone acetate (MPA) pellets (50 mg, 90-day release) were implanted subcutaneously into the interscapular area of immunocompetent C57BL/6 mice. Then the animals received 1 mg dimethylbenzantracene (DMBA) administered by oral gavage 6 x during 7 weeks. When spontaneous tumors became palpable, primary tumors (PT) were either excised and used for the flowcytometric detection of CD47 surface expression (a) or mice were randomly assigned to receive 10 mg/kg anti-CD47 antibody or isotype control that was administered intraperitoneally (i.p.). The antibodies were administered 4 times a week and then discontinued (Schedule I, b,d,f) or continuously given throughout the experiment (Schedule II, c,e,g). The tumor area was measured using a caliper until ethical endpoints were reached and animals had to be sacrificed (d,e). Experiments were performed with 12 animals per group and isotype controls from schedules I and II were pooled for tumor growth and survival analyses. Data were analyzed with TumGrowth (https://github.com/kroemerlab) to calculate significances and overall survival (f,g). The spleen from representative tumor-bearing C57BL/6 that were treated with the indicated antibodies were resected at the end of the experiment and photos were taken to document the increase in organ size (h,i).
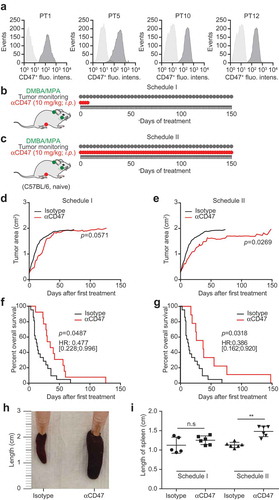
With respect to the monotherapy with anti-CD47 blockade, very similar data were obtained in a second transplantable model of breast cancer, in which AT3, which express CD47 (similar to another breast cancer cell line 4T1) ()), were injected subcutaneously into immunocompetent C57BL/6 mice. In this model, we again performed CD47 blockade when tumors became palpable, by injecting CD47-blocking antibodies either for 4 days, followed by therapy discontinuation (schedule I, ), Fig. S2A) or throughout the duration of the experiment (schedule II, ), Fig. S2B). Schedule II turned out be more efficient in reducing tumor growth and in extending life span (–g)), although both schedules were similarly efficient in inducing splenomegaly (,i).
Figure 2. CD47 antibody decreases the growth of transplanted tumors. CD47 surface expression was detected in murine breast cancer 4T1 and AT3 cells by flow cytometry (a). One million murine breast cancer AT3 cells were inoculated subcutaneously into immunocompetent syngeneic C57BL/6 mice. Palpable tumors were injected intraperitoneally (i.p.) with 10 mg/kg anti-CD47 antibody or isotype control. Antibody or isotype control was administered 4 times a week for one week and then discontinued (Schedule I, b,d,f) or continuously (5 times a week) injected until the end of the experiment (Schedule II, c,e,g). The tumor area was measured using a caliper until ethical endpoints were reached when animals had to be sacrificed. Mean values of 8 animal per group are depicted (d,e). Data were analyzed with TumGrowth (https://github.com/kroemerlab) to calculate statistical differences and overall survival (f,g). The spleens from representative tumor-bearing C57BL/6 that were treated with the indicated antibodies were resected at the end of the experiment, and photos of the organs were taken to document morphological changes (h,i).
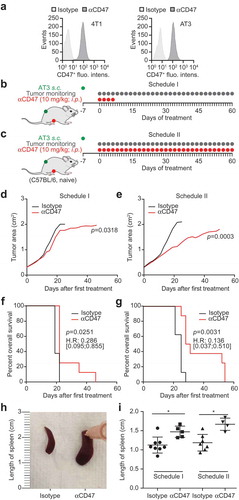
T cell dependent effects of CD47-antibody immunotherapy
In a next round of experiments, we attempted to combine continuous CD47-directed immunotherapy with MTX-based chemotherapy (). The latter was administered at a dose that is efficient against many transplantable mouse cancers.Citation10,Citation23,Citation26 However, the combination treatment did not further increase the anti-CD47-induced control of tumor growth (–f) or overall survival (). Thus, at difference with the carcinogen-induced model of breast cancer (Fig. S1), we did not find an additive interaction between chemotherapy and CD47 blockade on the growth of AT3 cancers. Importantly, we found that depletion of CD4+ and CD8+ lymphocytes by periodic injection of suitable antibodies () was able to eliminate tumor growth reduction by the combination of CD47 blockade and MTX (), supporting that these effects entirely depend on T cells.
Figure 3. CD47 antibody-mediated tumor growth suppression is T cell-dependent. One million murine breast cancer AT3 cells were inoculated subcutaneously in immunocompetent syngeneic C57BL/6 mice. Palpable tumors were treated with 10 mg/kg anti-CD47 antibody or isotype control administered intraperitoneally (i.p.) together with or without mitoxantrone (MTX)-based chemotherapy (5.17 mg/kg). Antibody or isotype control was administered 5 times a week, while chemotherapy was administered once only. For T cell depletion, CD4 and CD8-specific antibodies (0.4 mg/mouse each) were injected together with chemotherapy and then once per week (a). The tumor area was measured using a caliper until ethical endpoints were reached. Tumor growth curves are individually depicted (b–e) and summarized as mean values (f). Data were analyzed with TumGrowth (https://github.com/kroemerlab) to calculate significances and overall survival (G).
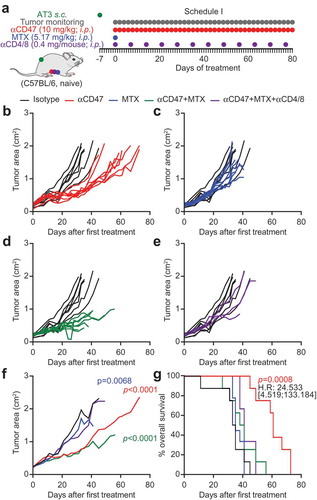
Stimulated by these results, we decided to investigate the effects of CD47 blockade on the composition of the immune infiltrate of AT3 tumors. For this, mice bearing established AT3 cancers were systemically treated with the CD47-blocking antibody for 10 days () and the immune infiltrate was determined by immunofluorescence analysis of monocellular suspensions obtained from the excised tumors (for gating strategies see Fig. S3). The absolute density of regulatory T cells (Tregs defined as CD3+CD4+CD25+FoxP3+) in the tumor tissue was strongly reduced (), and the proportion of Tregs among CD3+CD4+ cells also declined (), while the ratio of CD3+CD8+ T cells (which include bona fide cytotoxic T lymphocytes) over CD3+CD4+ cells () or Tregs () was increased upon CD47 blockade. Similarly, the absolute density of M2 macrophages (defined as CD45+CD11b+CD11clowF4/80+CD80−MHCclass II+) declined upon CD47 blockade (), commensurate with an increase in the M1/M2 ratio (). Altogether, these results indicate that CD47 blockade leads to the elimination of immunosuppressive cells (such as Tregs and M2 macrophages) from the tumor microenvironment, hence favoring anticancer immune responses at this level.
Figure 4. Immunotherapy with CD47 antibody induces changes in tumor immune infiltrate. Mouse breast cancer AT3 tumors were established subcutaneously and mice received 10 mg/kg anti CD47 antibody daily (a). At day 10 tumors were excised and dissociated followed by staining with exclusion dye and the immune detection of surface markers and intranuclear Foxp3 by flow cytometry. Data was analyzed by GraphPad and subjected to outlier exclusion by means of the Grubbs’ test. The absolute number of regulatory T cells (Tregs) per tumor mass and the percent of Tregs decreased in response to CD47 antibody immunotherapy (b,c), whereas the ratio of CD8+ over CD4+ and ratio of CD8+ over Tregs increased (d,e). The number of M2 macrophages decreased and the ratio of M1 over M2 macrophages increased (f,g). Results were subjected to unpaired Student t tests (*p < 0.05; **p < 0.01; ***p < 0.001).
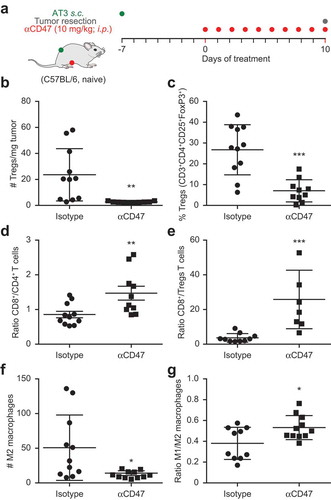
Concluding remarks
The data presented in this paper strongly indicate that CD47 blockade has a favorable impact on the evolution of carcinogen-induced orthotopic or transplantable breast cancers that express CD47. Indeed, repeated injections of a CD47-blocking antibody used as a stand-alone therapeutic agent were strongly effective in reducing tumor growth. This effect was reversed by the depletion of T lymphocytes, suggesting that it is immune-dependent, and was indeed accompanied by a major remodeling of the tumor microenvironment with a partial reduction of M2 macrophages and a close-to-complete elimination of immunosuppressive Tregs. From these results, we conclude that CD47 blockade might constitute a valuable strategy for treating breast cancer. Recently the expression of MHC class I component β2-microglobulin (β2M) by cancer cells was identified as yet another “don’t eat me” signal and future studies on its therapeutic value are urgently awaited.Citation27
It remains to be determined which molecular subtypes of breast cancer would be amenable to such a therapeutic avenue. In speculative terms, distinct breast cancer types might differ in CD47 expression, knowing that circulating metastasis-initiating cells tend to be CD47+.Citation17 Moreover, distinct breast cancer subtypes are largely heterogeneous in their immune infiltrate,Citation28 and breast cancer cells are not uniform in their capacity to activate the molecular pathway leading to CALR exposure that depends on the phosphorylation of eukaryotic initiation factor 2α (eIF2α).Citation29–Citation31 Hence, it will be important to understand which particular fraction of breast cancer patients would be amenable to successful CD47 blockade.
Materials and methods
Cell culture and treatment
Anti-CD47 (murine IgG2b, that aside from rodent CD47 also recognizes human CD47) was obtained from Tioma Therapeutics (Brisbane, CA, US) and isotype control (ISO, mIgG2a; clone C1.18.4) came from BioXCell (West Lebanon, NH, US). Culture media were from Gibco-Invitrogen (Carlsbad, CA, US). AT3 (ATCC® CRL-2375) cells were obtained from the European branch of the American Type Culture Collection (ATCC) and cultured under standard cell culture conditions (5% CO2; 37°C in a water saturated atmosphere within a cell culture incubator (HeraCell, Heraeus, Germany)).
In vivo procedure
Animal experiments were conducted in compliance with the EU Directive 63/2010 and the procedures (2016_069_5580) were approved by the Ethical Committee of the Gustave Roussy Campus Cancer (CEEA IRCIV/IGR no. 26, registered at the French Ministry of Research). C57BL/6 mice (Envigo, Gannat, France) were housed in a temperature-controlled environment with 12 h light/dark cycles and received food and water ad libitum. One million murine breast cancer AT3 cells were inoculated s.c. into the flank of C57BL/6 mice and tumor growth was routinely monitored thereafter by means of a standard caliper. When tumor surface reached 35–50 mm2, mice (n = 8 per group) were randomly assigned to receive either 10 mg/kg anti-CD47 antibody on the same day as chemotherapy (mitoxantrone, MTX, 5.17 mg/kg) or with a delay of 3 days. Then, the animal received anti-CD47 antibody or the same amount of isotype control (10 mg/kg) 5 times a week. A total of 0.5 mg of anti-CD8 (clon 2.43 BioXCell BE0061) and anti-CD4 (clon GK1.5 BioXCell BE0003-1) i.p. injections were repeated every 7 days to assure the complete depletion of both T cell populations during the whole experiment.
Six-week-old female C57BL/6 mice (n = 12 per group) underwent surgical implantation of slow-release medroxyprogesterone acetate (MPA) pellets (50 mg, 90-day release; Innovative Research of America, Sarasota, Fl, US) s.c. in the right flank. 200 μL of 5 mg/mL dimethylbenzantracene (DMBA, Sigma Aldrich, St. Louis, MO, US) dissolved in corn oil was administered by oral gavage 6 x during 7 weeks.
Flow cytometric detection of CD47 expression
Primary tumor or cell culture cells were harvested and separated by standard procedures before incubation of samples with the therapeutic anti-CD47 antibody or isotype control. The samples were repeatedly washed and then incubated with species-matched fluorochrome-coupled secondary antibody before flowcytometric assessment.
Tumor immune infiltrate phenotyping
Mouse breast cancer AT3 tumors were established subcutaneously and mice received 10 mg/kg anti-CD47 daily. At day 10, tumors were excised and collected in gentleMACS C Tubes (Miltenyi Biotech; Bergisch Gladbach, Germany) containing cell culture medium. The samples were dissociated using the gentleMACS dissociator in combination with a mouse tumor dissociation kit (Miltenyi Biotech) according to the manufacturer’s recommendations. The tumor cell suspension was resuspended in cell culture media and further dissociated by means of 70 μm and 40 μm nylon cell strainers (Miltenyi Biotec) before washing with cold PBS. Prior to the immune detection of surface markers, samples were incubated with LIVE/DEAD® Fixable Yellow Dead Cell dye (Invitrogen) to discriminate viable from damaged/dead cells. Then, Fc receptors were blocked through incubation with anti-CD16/CD32 (BD Biosciences) and the cells were subjected to immunodetection of surface markers with a panel of fluorescence-conjugated antibodies before the permeabilization and fixation by means of the FoxP3/Transcription Factor Staining Buffer Set (eBioscience, San Diego, CA, US) for the staining of intranuclear FoxP3. Data were acquired by means of a BD LSRII flow cytometer (BD Biosciences) and analyzed with the FlowJo software (FlowJo LLC; Ashland, OR, US). Tumor-infiltrating lymphocytes were enumerated taking in account harvested tumor mass and volume of the dissociated tumor cell suspension as well as the proportion of the cell suspension that has been actually stained and ran through the flow cytometer.
Statistical procedures
Unless otherwise specified, experiments were performed with 8–12 animals per group. Tumor immune infiltrate data was analyzed with GraphPad and outliers were excluded by means of the Grubbs’ test. In vivo data were analyzed with the open access application TumGrowth (https://github.com/kroemerlab) which automatically applies outlier exclusion and statistical tests. Longitudinal analyses of segments of tumor growth curves are subjected to automatic analyses of breakpoints in the tumor growth characteristics and outlier detection on the basis of Bonferroni-corrected p-values calculated from the residuals (if required). Tumor growth curves were subjected to type II ANOVA and pairwise comparisons across groups. Cross-sectional analyses for the comparison of tumor sizes at one single time point were subjected to outlier detection on the basis of Bonferroni-corrected p-values calculated from the residuals (if required), followed by comparison of groups using the Wilcoxon rank sum test adjusted by the Holm method. Time-to-endpoint data were visualized as Kaplan Meier plots and subjected to statistical comparisons by means of the Cox regression model, likelihood ratios, Wald and score tests.
Abbreviations
CALR | = | calreticulin |
DC | = | dendritic cell |
ICD | = | immunogenic cell death |
ip | = | intraperitoneal |
Conflicts of interest
This work was partially funded by Tioma Therapeutics.
Supplemental Material
Download Zip (269.8 KB)Acknowledgments
GK is supported by the Ligue contre le Cancer (équipe labellisée); Agence National de la Recherche (ANR) – Projets blancs; Association pour la recherche sur le cancer (ARC); Cancéropôle Ile-de-France; Chancelerie des universités de Paris (Legs Poix); Fondation pour la Recherche Médicale (FRM); the European Research Council (ERC); Institut National du Cancer (INCa); Inserm (HTE); Institut Universitaire de France; the LabEx Immuno-Oncology; the RHU Torino Lumière; the SIRIC Stratified Oncology Cell DNA Repair and Tumor Immune Elimination (SOCRATE); the SIRIC Cancer Research and Personalized Medicine (CARPEM), as well as Vasculox Ltd. LZ was supported by Ligue contre le Cancer (équipe labellisée), RHU TORINO-LUMIERE, Swiss Bridge Foundation, ARC and LabEx Immuno-Oncology.
Supplementary Material
Supplemental data for this article can be accessed here.
References
- Galluzzi L, Buque A, Kepp O, Zitvogel L, Kroemer G. Immunological effects of conventional chemotherapy and targeted anticancer agents. Cancer Cell. 2015;28:690–714. doi:10.1016/j.ccell.2015.10.012.
- Galluzzi L, Senovilla L, Zitvogel L, Kroemer G. The secret ally: immunostimulation by anticancer drugs. Nat Rev Drug Discov. 2012;11:215–233. doi:10.1038/nrd3626.
- Bezu L, Sauvat A, Humeau J, Leduc M, Kepp O, Kroemer G. eIF2alpha phosphorylation: A hallmark of immunogenic cell death. Oncoimmunology. 2018;7:e1431089. doi:10.1080/2162402X.2018.1431089.
- Garg AD, More S, Rufo N, Mece O, Sassano ML, Agostinis P, Zitvogel L, Kroemer G, Galluzzi L. Trial watch: immunogenic cell death induction by anticancer chemotherapeutics. Oncoimmunology. 2017;6:e1386829. doi:10.1080/2162402X.2017.1386829.
- Lund A, Tjolsen A, Hole K. Desipramine in small doses induces antinociception in the increasing temperature hot-plate test, but not in the tail-flick test. Neuropharmacology. 1989;28:1169–1173.
- Yang M, Li C, Zhu S, Cao L, Kroemer G, Zeh H, Tang D, Kang R. TFAM is a novel mediator of immunogenic cancer cell death. Oncoimmunology. 2018;7:e1431086. doi:10.1080/2162402X.2018.1431086.
- Galluzzi L, Buque A, Kepp O, Zitvogel L, Kroemer G. Immunogenic cell death in cancer and infectious disease. Nat Rev Immunol. 2017;17:97–111. doi:10.1038/nri.2016.107.
- Kroemer G, Galluzzi L, Kepp O, Zitvogel L. Immunogenic cell death in cancer therapy. Annu Rev Immunol. 2013;31:51–72. doi:10.1146/annurev-immunol-032712-100008.
- Pfirschke C, Engblom C, Rickelt S, Cortez-Retamozo V, Garris C, Pucci F, Yamazaki T, Poirier-Colame V, Newton A, Redouane Y, et al. Immunogenic chemotherapy sensitizes tumors to checkpoint blockade therapy. Immunity. 2016;44:343–354. doi:10.1016/j.immuni.2015.11.024.
- Senovilla L, Vitale I, Martins I, Tailler M, Pailleret C, Michaud M, Galluzzi L, Adjemian S, Kepp O, Niso-Santano M, et al. An immunosurveillance mechanism controls cancer cell ploidy. Science. 2012;337:1678–1684. doi:10.1126/science.1224922.
- Obeid M, Tesniere A, Ghiringhelli F, Fimia GM, Apetoh L, Perfettini JL, Castedo M, Mignot G, Panaretakis T, Casares N, et al. Calreticulin exposure dictates the immunogenicity of cancer cell death. Nat Med. 2007;13:54–61. doi:10.1038/nm1523.
- Obeid M, Panaretakis T, Joza N, Tufi R, Tesniere A, van Endert P, Zitvogel L, Kroemer G. Calreticulin exposure is required for the immunogenicity of gamma-irradiation and UVC light-induced apoptosis. Cell Death Differ. 2007;14:1848–1850. doi:10.1038/sj.cdd.4402201.
- Chao MP, Jaiswal S, Weissman-Tsukamoto R, Alizadeh AA, Gentles AJ, Volkmer J, Weiskopf K, Willingham SB, Raveh T, Park CY, et al. Calreticulin is the dominant pro-phagocytic signal on multiple human cancers and is counterbalanced by CD47. Sci Transl Med. 2010;2:63ra94. doi:10.1126/scitranslmed.3001375.
- Zhang H, Lu H, Xiang L, Bullen JW, Zhang C, Samanta D, Gilkes DM, He J, Semenza GL. HIF-1 regulates CD47 expression in breast cancer cells to promote evasion of phagocytosis and maintenance of cancer stem cells. Proc Natl Acad Sci U S A. 2015;112:E6215–6223. doi:10.1073/pnas.1520032112.
- Samanta D, Park Y, Ni X, Li H, Zahnow CA, Gabrielson E, Pan F, Semenza GL. Chemotherapy induces enrichment of CD47(+)/CD73(+)/PDL1(+) immune evasive triple-negative breast cancer cells. Proc Natl Acad Sci U S A. 2018;115:E1239–E1248. doi:10.1073/pnas.1718197115.
- Betancur PA, Abraham BJ, Yiu YY, Willingham SB, Khameneh F, Zarnegar M, Kuo AH, McKenna K, Kojima Y, Leeper NJ, et al. A CD47-associated super-enhancer links pro-inflammatory signalling to CD47 upregulation in breast cancer. Nat Commun. 2017;8:14802. doi:10.1038/ncomms14802.
- Baccelli I, Schneeweiss A, Riethdorf S, Stenzinger A, Schillert A, Vogel V, Klein C, Saini M, Bauerle T, Wallwiener M, et al. Identification of a population of blood circulating tumor cells from breast cancer patients that initiates metastasis in a xenograft assay. Nat Biotechnol. 2013;31:539–544. doi:10.1038/nbt.2576.
- Willingham SB, Volkmer JP, Gentles AJ, Sahoo D, Dalerba P, Mitra SS, Wang J, Contreras-Trujillo H, Martin R, Cohen JD, et al. The CD47-signal regulatory protein alpha (SIRPa) interaction is a therapeutic target for human solid tumors. Proc Natl Acad Sci U S A. 2012;109:6662–6667. doi:10.1073/pnas.1121623109.
- Horrigan SK. Reproducibility project: cancer B. Replication Study: the CD47-signal regulatory protein alpha (SIRPa) interaction is a therapeutic target for human solid tumors. Elife. 2017:6. doi:10.7554/eLife.18173.
- Feliz-Mosquea YR, Christensen AA, Wilson AS, Westwood B, Varagic J, Melendez GC, Schwartz AL, Chen QR, Mathews Griner L, Guha R, et al. Combination of anthracyclines and anti-CD47 therapy inhibit invasive breast cancer growth while preventing cardiac toxicity by regulation of autophagy. Breast Cancer Res Treat. 2018. doi:10.1007/s10549-018-4884-x.
- Pietrocola F, Pol J, Vacchelli E, Rao S, Enot DP, Baracco EE, Levesque S, Castoldi F, Jacquelot N, Yamazaki T, et al. Caloric restriction mimetics enhance anticancer immunosurveillance. Cancer Cell. 2016;30:147–160. doi:10.1016/j.ccell.2016.05.016.
- Baracco EE, Pietrocola F, Buque A, Bloy N, Senovilla L, Zitvogel L, Vacchelli E, Kroemer G. Inhibition of formyl peptide receptor 1 reduces the efficacy of anticancer chemotherapy against carcinogen-induced breast cancer. Oncoimmunology. 2016;5:e1139275. doi:10.1080/2162402X.2016.1139275.
- Vacchelli E, Ma Y, Baracco EE, Sistigu A, Enot DP, Pietrocola F, Yang H, Adjemian S, Chaba K, Semeraro M, et al. Chemotherapy-induced antitumor immunity requires formyl peptide receptor 1. Science. 2015;350:972–978. doi:10.1126/science.aad0779.
- Bian Z, Shi L, Guo YL, Lv Z, Tang C, Niu S, Tremblay A, Venkataramani M, Culpepper C, Li L, et al. Cd47-Sirpalpha interaction and IL-10 constrain inflammation-induced macrophage phagocytosis of healthy self-cells. Proc Natl Acad Sci U S A. 2016;113:E5434–5443. doi:10.1073/pnas.1521069113.
- Gardai SJ, McPhillips KA, Frasch SC, Janssen WJ, Starefeldt A, Murphy-Ullrich JE, Bratton DL, Oldenborg PA, Michalak M, Henson PM. Cell-surface calreticulin initiates clearance of viable or apoptotic cells through trans-activation of LRP on the phagocyte. Cell. 2005;123:321–334. doi:10.1016/j.cell.2005.08.032.
- Casares N, Pequignot MO, Tesniere A, Ghiringhelli F, Roux S, Chaput N, Schmitt E, Hamai A, Hervas-Stubbs S, Obeid M, et al. Caspase-dependent immunogenicity of doxorubicin-induced tumor cell death. J Exp Med. 2005;202:1691–1701. doi:10.1084/jem.20050915.
- Barkal AA, Weiskopf K, Kao KS, Gordon SR, Rosental B, Yiu YY, George BM, Markovic M, Ring NG, Tsai JM, et al. Engagement of MHC class I by the inhibitory receptor LILRB1 suppresses macrophages and is a target of cancer immunotherapy. Nat Immunol. 2018;19:76–84. doi:10.1038/s41590-017-0004-z.
- Kroemer G, Senovilla L, Galluzzi L, Andre F, Zitvogel L. Natural and therapy-induced immunosurveillance in breast cancer. Nat Med. 2015;21:1128–1138. doi:10.1038/nm.3944.
- Bezu L, Sauvat A, Humeau J, Gomes-da-Silva LC, Iribarren K, Forveille S, Garcia P, Zhao L, Liu P, Zitvogel L, et al. eIF2alpha phosphorylation is pathognomonic for immunogenic cell death. Cell Death Differ. 2018. doi:10.1038/s41418-017-0044-9.
- Semeraro M, Adam J, Stoll G, Louvet E, Chaba K, Poirier-Colame V, Sauvat A, Senovilla L, Vacchelli E, Bloy N, et al. The ratio of CD8(+)/FOXP3 T lymphocytes infiltrating breast tissues predicts the relapse of ductal carcinoma in situ. Oncoimmunology. 2016;5:e1218106. doi:10.1080/2162402X.2016.1218106.
- Zitvogel L, Kepp O, Senovilla L, Menger L, Chaput N, Kroemer G. Immunogenic tumor cell death for optimal anticancer therapy: the calreticulin exposure pathway. Clin Cancer Res. 2010;16:3100–3104. doi:10.1158/1078-0432.CCR-09-2891.