ABSTRACT
Dexamethasone is a synthetic glucocorticoid commonly used for the prevention and management of side effects in cancer patients undergoing chemotherapy. While it is effective as an anti-emetic and in preventing hypersensitivity reactions, dexamethasone depletes peripheral blood lymphocytes and impacts immune responses. The effect of dexamethasone on the number and quality of tumour-infiltrating leukocytes has not been reported. To address this, we calibrated the dose in two different strains of mice to achieve the same extent of peripheral blood lymphocyte depletion observed in patients with cancer. Doses that caused analogous depletion of T and B lymphocytes and NK cells from the peripheral blood, elicited no change in these populations within the tumour. The expression of immune checkpoint molecules PD-1, OX40, GITR and TIM3 on tumour-infiltrating lymphocytes was not altered. We found that dexamethasone had a small but significant deleterious impact on weakly efficacious chemoimmunotherapy but had no effect when the protocol was highly efficacious. Based on these results, we predict that dexamethasone will have a modest negative influence on the overall effectiveness of chemoimmunotherapy treatment.
Introduction
Glucocorticoids are used to prevent and reduce side effects in patients receiving chemotherapy, including nausea, vomiting and allergic/hypersensitivity reactions.Citation1 While there are many classes of synthetic glucocorticoids, dexamethasone is the most widely used adjunct for systemic chemotherapy. It has a longer half-life and a higher binding affinity for glucocorticoid receptors than either cortisol or other synthetic corticosteroid variants and is known to be immunosuppressive. Specifically, dendritic cell (DC) numbers are reduced and maturation is inhibited via downregulation of MHC class II, CD1a, CD80/86 and reduced cytokine synthesis including IL-12 and TNFα.Citation2 Direct suppression can occur via inhibition of AP-1, NF-κB and nuclear factor of activated T cells (NFAT), all of which are involved in T cell receptor signalling.Citation3 Dexamethasone also induces apoptosis of circulating CD4+ and CD8+ T lymphocytes while increasing both ICOS and Ki67 expression on regulatory T cells (Tregs), indicating increases in Treg activation and proliferation status, contributing to their immune suppressive activity.Citation4
The combination of chemotherapy with immune checkpoint blockade is being widely tested in clinical trials,Citation5–Citation7 with at least part of the rationale being that chemotherapy can induce several immunostimulatory effects.Citation8–Citation10 These include immunogenic cell death, increased cross-presentation of tumour antigens, DC activation, depletion of suppressor cells and enhanced tumour sensitivity to cytotoxic T lymphocyte (CTL) killingCitation8,Citation11 While systematic reviews have not found a significant effect of glucocorticoids on clinical outcome in patients treated with immune checkpoint blocking antibodies, these analyses used retrospective data, which could be subject to selection bias.Citation12 Given the chance of severe toxicity when administering chemotherapy to patients in the absence of dexamethasone, a clinical trial testing its influence on the therapeutic response would not be feasible. Here, we therefore assessed the effects of clinically relevant dosages of dexamethasone on immune cell populations, and immune checkpoint expression in the tumour in preclinical tumour models. We focused on the effect of dexamethasone on tumour-infiltrating immune cell subsets and the clinical response to combination treatment with chemotherapy and checkpoint blockade, using a dosing regime that phenocopies the peripheral lymphodepletion occurring in patients.
Results
Dexamethasone treatment results in substantial lymphodepletion in peripheral blood in patients and mice
We aimed to quantify the extent of lymphodepletion following dexamethasone treatment in patients in the context of standard chemotherapy. We found a mean decrease of approximately 450 CD3 + T lymphocytes per uL of blood after dexamethasone treatment ()), which represents a 44% change from baseline. To replicate the extent of lymphodepletion in mice, we dosed BALB/c and C57BL/6 mice with different concentrations of dexamethasone for four days taking blood samples before dosing and 24 hours after the final dose. Both mouse strains showed a dose-dependent depletion of peripheral blood lymphocytes. The lowest dose that provided a significant depletion was 1.25 mg/kg, and the dose that gave a depletion of approximately 50% in both models was 5 mg/kg. These dosages were therefore used for subsequent experiments.
Figure 1. Dexamethasone induces peripheral blood lymphodepletion in BALB/c and C57BL/6 mice similar to patients. (a), Treatment schedules for mesothelioma patients and (b), mice receiving dexamethasone. (c), Absolute CD3+ T lymphocyte numbers in peripheral blood of patients before and after dexamethasone administration. (d), Percentage change from baseline of lymphocytes in BALB/c and (e), C57BL/6J mice at different dosages of dexamethasone as measured on the Hemavet blood analyser. Depicted are mean percentages with SD, n = 6 for all groups; *p < .05, **p < .01, ***p < .001,****p < .0001 (comparison with PBS).
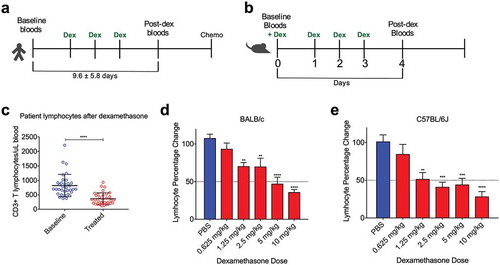
Dexamethasone causes lymphodepletion in blood but not tumour
To characterise the effects of dexamethasone on individual immune cell population numbers and activation/proliferation state in blood and tumour, we used flow cytometry. Using both the automated signature stratification algorithm CITRUSCitation13 (Supplementary Fig 1) and standard manual gating of the flow cytometry data (), we found distinct patterns between blood and tumour samples from the same mice. In blood, there was a significant decrease in CD4+ and CD8+ T cells, Tregs, B cells and NK cells in a dose dependent manner ()). Neutrophils were significantly elevated in blood, reaching almost 60% of circulating CD45+ leucocytes ()). In patients, similar proportional increases in granulocytes are observed following dexamethasone, thus validating the mouse models as a surrogate for patients.Citation14
Figure 2. Dexamethasone causes lymphodepletion in blood, but not in tumours. Blood and tumour samples were collected from mice that received either saline, 1.25 mg/kg or 5 mg/kg dexamethasone and analysed via flow cytometry. Percentage changes of (a), lymphoid and (b), myeloid immune subsets in blood and tumour from BALB/cArc mice treated with saline, 1.25 mg/kg dexamethasone or 5 mg/kg dexamethasone. (c), Analysis of activation marker ICOS and (d), proliferation marker Ki67 expression of CD4+ and CD8+ T lymphocytes and Tregs in blood and tumour. Depicted are mean percentages with SEM, n=8 for all groups; *p < .05, **p < .01, ***p < .001 (comparison with saline controls).
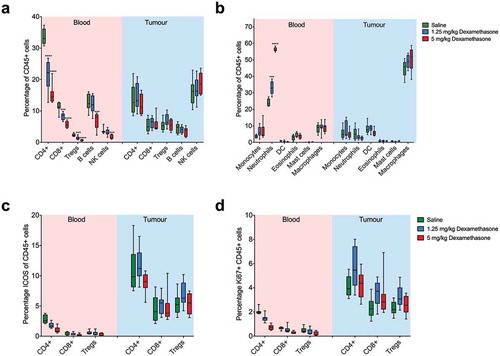
In contrast, in the tumour we observed no significant differences in any of the immune subsets at either dose of dexamethasone compared to untreated mice. T cell activation as measured by ICOS expression, was not significantly altered after dexamethasone ()). Similarly, the proliferative capacity of tumour-infiltrating T cells as measured by KI67 expression, was not affected by dexamethasone treatment ()). Together, these data show that lymphodepletion occurs in peripheral blood but is not apparent in the tumour. Importantly, T cell activation and proliferation in the tumour microenvironment are not affected by dexamethasone.
Immune checkpoint molecule expression on intratumoural T lymphocytes remains largely unaltered after dexamethasone administration
Since dexamethasone is part of the standard supportive care of patients treated with chemotherapy in combination with immune checkpoint blocking antibodies,Citation5,Citation6 we analysed the level of expression of several immune checkpoints on tumour-infiltrating lymphocytes following dexamethasone. We found that the expression of most immune checkpoints was unaltered even following the highest dose of dexamethasone. As expected, we observed an increase of GITR (glucocorticoid-induced TNFR family related gene), as well as PD-1 on CD4+ T lymphocytes ()), but this was not the case for CD8+ T cells or Tregs. In fact, no change in checkpoint molecule expression was detected on CD8+ T lymphocytes ()). Dexamethasone did not induce changes to CD3- cells within the tumour (Supplementary Fig 4).
Figure 3. Checkpoint molecule expression on tumour-infiltrating lymphocytes is not affected by dexamethasone. Flow cytometry analysis of tumour samples after dexamethasone treatment showing expression of immune checkpoint molecules GITR, OX40, PD-1 and TIM-3 on tumour-infiltrating T cells. (a), Foxp3−/CD4+ T helper cells. (b), FoxP3+ Tregs. (c), and CD8+ cytotoxic T cells. Depicted are mean percentages with SEM of the parent population. n = 5 for all groups; *p < .05, **p < .01.
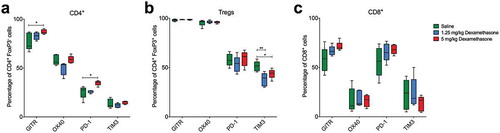
Differential effects of dexamethasone on the in vivo efficacy of chemoimmunotherapy treatment combinations
Given the limited effects of dexamethasone on the quantity and phenotype of tumour-infiltrating lymphocytes, we tested the effect of co-administration of dexamethasone with chemotherapy/checkpoint blockade on tumour control. Dexamethasone was administered to mice that received anti-CTLA4 and anti-PD-L1 antibodies in combination with either cisplatin or 5-FU (). These models display different levels of sensitivity to immune checkpoint blockade/chemotherapy combination treatment, with AE17 being relatively resistant, AB1 showing some additive efficacy for combination over single treatment with cisplatin, and synergistic efficacy for combination of 5-FU/checkpoint blockade over either treatment alone. We found that dexamethasone did not have a significant effect on the limited anti-tumour response in AE17 ()). However, the response to cisplatin/checkpoint combination therapy was significantly diminished with dexamethasone treatment in AB1 ()), reducing the overall response rate from 50% to 20%. In contrast, there was no effect on response with the 5-FU combination ()), with a cure rate of 100%, regardless of whether dexamethasone was administered or not. Since these differences in effect on the anti-tumour response could be due to the fact that we used two different chemotherapeutics, we also compared the effects of dexamethasone on checkpoint blockade alone in AB1, which is relatively sensitive to this therapy.Citation15 Also in this setting, although we still observed complete responders, we did observe that the clinical efficacy of anti-CTLA4/anti-PD-L1 checkpoint blockade was slightly diminished by co-treatment with dexamethasone even though this did not result in a statistically significant survival difference (), Supplementary Figure 2). Combined, these data indicate that dexamethasone, when given at a dose that causes lymphodepletion to a similar level as seen in patients, can have a modest inhibitory effect on the efficacy of checkpoint blockade/chemotherapy. Dexamethasone did not alter the response to either chemotherapy alone (Supplementary Figure 3).
Figure 4. Dexamethasone has a differential effect on the in vivo therapeutic response of chemotherapy/checkpoint blockade combination therapy. Survival plot of s.c. AE17 mesothelioma-bearing C57BL/6 mice (a), n = 10 per group) or AB1 mesothelioma-bearing BALB/c mice (b), n = 15 per group) treated with cisplatin with or without anti-CTLA4/anti-PD-L1, with or without dexamethasone. (c), Survival plot of s.c. AB1 mesothelioma-bearing BALB/c mice treated with 5-FU with or without anti-CTLA4/anti-PD-L1, with or without dexamethasone (n = 10 per group). (d), Mean growth curves of AB1 tumours in BALB/c mice treated with anti-CTLA4/anti-PD-L1 checkpoint blockade (starting day 12) with or without 1.25 mg/kg dexamethasone (days 12–15). Log rank (Mantel-Cox) analysis performed on survival curves and a mixed model analysis of variance on tumour growth (n = 15 per group).
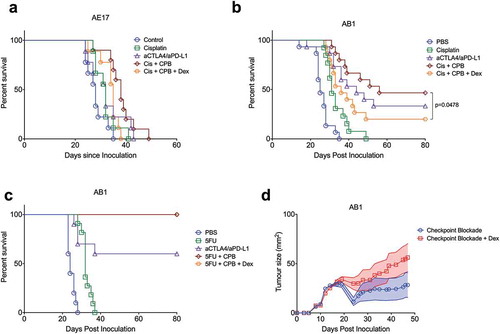
Discussion
Patients undergoing systemic chemotherapy with or without checkpoint blockade routinely receive supportive care to manage side effects. For those receiving platinum-based chemotherapeutics, toxicity can be quite severe and includes nausea, vomiting and loss of appetite.Citation1 For other chemotherapeutics, such as pemetrexed, dexamethasone needs to be co-administered to prevent hypersensitivity drug reactions.Citation16 Dexamethasone is a common synthetic glucocorticoid agonist that is largely successful in limiting these side effects. However, due to its wide ranging immunosuppressive properties,Citation3 we wanted to test whether dexamethasone might inhibit the positive immunostimulatory effects of checkpoint blocking antibodies. We previously found significant decreases in some T lymphocyte subsets following dexamethasone treatment that was independent of chemotherapy.Citation4 While pre-clinical studies have presented both pro- and anti-tumorigenic effects of dexamethasone,Citation17 this has not been done in the context of combination chemoimmunotherapy. Most pre-clinical work has analysed the effect of glucocorticoid activity in vitro using cell lines. The effects of glucocorticoids on in vivo anti-tumour responses after treatment have not been characterised in detail.
Clinical trials investigating the potential of chemoimmunotherapy combinations have shown both positiveCitation5 and nullCitation7 effects, however, the use of glucocorticoids including dexamethasone has not been examined in these trials. It remains a possibility that positive results could have been better, if glucocorticoids were inhibiting immune activation. Usually patients receiving chronic glucocorticoid treatment above adrenal replacement doses are excluded from checkpoint blockade treatment due to the assumption that immune function will be inhibited.Citation12 While there are data suggesting that glucocorticoids do not alter the efficacy of immune checkpoint blockade;Citation18–Citation22 these analyses were ad hoc and not a primary endpoint of the trial. In addition, these were retrospective analyses investigating whether treatment of dexamethasone for toxicity induced by immune checkpoint blockade would not influence the outcome compared to patients who were not treated with dexamethasone. These retrospective analyses cannot exclude selection bias. Lastly, none of these analyses were in the context of chemotherapy-checkpoint antibody combination therapy, where patients were treated with dexamethasone right from the beginning as part of their supportive care. Indeed, there are also retrospective analyses that do show a deleterious effect of glucocorticoids.Citation23 A recent systematic review of the literature could not identify any clinical trial that investigated the interaction of glucocorticoids and checkpoint blockade as a primary endpoint, nor any studies that investigated the effect of dexamethasone on intratumoural leukocytes,Citation12 and illustrates the need for more targeted investigation. Ideally, this would be investigated in a prospective randomized trial, but given the potential for severe chemotherapy-induced toxicity in the absence of dexamethasone co-treatment, such a trial would pose ethical problems. For this reason, we considered animal studies as the next best alternative to investigate any dexamethasone effect on immune checkpoint blockade efficacy.
We optimized dexamethasone treatments in mice to mimic the depletion of lymphocyte populations including CD4+ and CD8+ T cells in the peripheral blood of patients.Citation4 The dose required to induce significant lymphodepletion in mice is analogous to that in humans when calculated relative to body surface area.Citation24 However, at these dosages, there was no change in any of the immune subtypes in tumours, indicating that while dexamethasone may have a systemic lymphodepleting effect, this is not necessarily reflected in the tumour microenvironment. Neutrophilia, characterised by an average increase of circulating neutrophils by 32%, was observed in peripheral blood, presumably due to inhibition of spontaneous neutrophil apoptosis which also mimics patient data.Citation25 Again, this was not observed within the tumour. Checkpoint molecule expression within tumours did not change markedly after dexamethasone treatment in vivo. However, it has been shown that dexamethasone enhances the level of PD-1 expression in vitro when cultured with mouse or human T cells, which did correlate with our findings.Citation26
There are several preclinical studies investigating the effect of dexamethasone on tumour cells, either in vitro or in vivo.Citation17 However, these primarily investigated glucocorticoids as a single agent, not in combination with other treatments and present contradictory results, with the majority of studies being conducted in vitro. In the context of chemotherapy treatment, glucocorticoids can induce resistance to therapy in several mouse cancer models.Citation27 Our study specifically focussed on the effect of dexamethasone co-medication on the efficacy of cancer chemoimmunotherapy treatment. 5-FU, an antimetabolite, can enhance anti-tumour immune responses via depletion of myeloid-derived suppressor cellsCitation28-Citation30 while cisplatin can sensitize tumour cells to granzyme B-mediated T cell cytotoxicityCitation31,Citation32 and enhance antigen presentation by dendritic cells.Citation33,Citation34 Cisplatin, which is the standard first-line therapy in mesothelioma in combination with pemetrexed, gave an additive effect over checkpoint blockade alone, while the combination of 5-FU and checkpoint blockade induced a profound anti-tumour immune response. This allowed us to test the effects of dexamethasone on the anti-tumour response when either a moderately additive or a synergistic drug combination was used. In the AB1 model, the robust therapeutic response induced by 5-FU/checkpoint blockade was not hampered by dexamethasone. However, when cisplatin was used, which gave only a modest additive effect on top of the immune checkpoint blockade response, dexamethasone significantly decreased the therapeutic response. There was no stimulatory or inhibitory effect seen in the AE17 model used in C57BL/6J mice, although this model has a lower response to all therapies and so does not provide a substantial effect that could be altered by the addition of dexamethasone. It therefore appears that the negative effect of dexamethasone may depend on the magnitude of the therapeutic effect induced by the chemotherapy, but we cannot exclude the possibility that the effect depends on the chemotherapeutic used. The fact that we also saw a minor decrease in complete responses with checkpoint blockade alone when dexamethasone was added, does hint at the possibility that these responses are affected by dexamethasone. Given that different corticosteroids have different immunological effects,Citation3 it will be of interest to see whether other corticosteroids have similar effects on the anti-tumour response, while maintaining an appropriate anti-emetic effect.
Our study does have several limitations. Firstly, we used mesothelioma models only. We chose this cancer type because the standard treatment for mesothelioma is platinum-based chemotherapy with dexamethasone as mandatory supportive care, and because mesothelioma is a representative example of a cancer that is amenable to chemo-immunotherapy.Citation35 Although responsiveness to checkpoint blockade between murine and human cancer types do not overlap well,Citation36,Citation37 and although we did test the effects of dexamethasone in two different mouse strains excluding strain-specific effects, we cannot exclude the possibility that other cancer types will respond differently. Secondly, while our study forms a first investigation on the effect of dexamethasone on tumour-infiltrating immune cells, an avenue that has not been explored yet in solid tumours,Citation17 further validation in tumour biopsies from patients after glucocorticoid treatment are needed. This could include gene expression studies, as has been done in the context of dexamethasone treatment without chemo-immunotherapy,Citation38 or with immune checkpoint blockade alone.Citation15 Pharmacokinetic analysis of dexamethasone infiltration into solid tumours may also provide insight into its immunomodulatory effects. Thirdly, we investigated the effects of dexamethasone alone on tumour-infiltrating immune cell populations, prior to chemotherapy. It is possible that the combination of dexamethasone and chemotherapy further alters their composition and phenotype. Future studies would ideally include studies investigating the dynamic events following dexamethasone and chemo-immunotherapy.Citation39 Lastly, prospective clinical studies specifically designed to investigate the effect of corticosteroids on the therapeutic immune response will need to be done before firm conclusions can be drawn.
Materials and methods
Patient samples
Blood samples were prospectively obtained from patients enrolled in two phase 1b chemoimmunotherapy clinical trials at Sir Gairdner Hospital (Perth, WA, Australia). Eligible patients had a confirmed diagnosis of malignant pleural mesothelioma (MPM) and were planned to have first-line treatment of cisplatin plus pemetrexed chemotherapy plus either low-dose cyclophosphamide or anti-CD40 agonistic antibody, depending on the trial they were enrolled in. Clinical trial registration numbers with the Australia New Zealand Clinical Trial Registry are ACTRN12609000294257 and ACTRN12609000260224 and all involvement of human subjects complied with the Declaration of Helsinki. Patients received dexamethasone prior to and during chemotherapy as anti-emetic and to prevent allergic reaction to pemetrexed. Dexamethasone was given as 2 × 4 mg oral doses the day before and 1 × 4 mg dose the day of chemotherapy administration. Blood samples were taken before and after dosing of dexamethasone but prior to receiving chemotherapy.Citation4 Blood was collected into BD K2EDTA Vacutainers (BD Diagnostics). We previously reported the results of this cohort with regard to lymphocyte subpopulations following dexamethasone,Citation4 here we focused on the number of CD3+ T cells only. Whole blood was stained and analysed via flow cytometry to determine the absolute cell number of CD3+ T lymphocytes. Samples were stained then fixation and red cell lysis performed using BD FACS lysing buffer. Data was collected using a Millipore Guava and Guava ExpressPro Software.
Mice
Female BALB/cArc and C57BL/6J mice were purchased from the Animal Resource Centre (Murdoch, Western Australia) and housed under specific pathogen free (SPF) conditions at the Harry Perkins Institute of Medical Research Bioresources Centre (QEII Medical Centre, Nedlands, Western Australia). Mice were between 8 to 10 weeks of age at the start of all experiments. Experiments were conducted in accordance with the code of conduct of the National Health and Medical Research Council (NHMRC) of Australia and protocols were approved by the Harry Perkins Institute for Medical Research Animal Ethics Committee (protocols AE029 and AE0100). Mice were fed rat and mouse cubes (Speciality Feeds, Perth, WA Australia) and housed on aspenchipsAB3 bedding (Datesand, Manchester UK). The animal facility temperature was kept between 21°C and 22°C. Drugs were dosed between 9:00 am and 11:00 am. For tumour flow cytometry and therapeutic studies, BALB/cArc and C57BL/6J mice were inoculated s.c. with 5 × 105 AB1 or AE17 mesothelioma tumour cells, respectively. Mice were randomised to treatment groups prior to treatment, several days after tumour inoculation, when tumours were palpable. The researcher measuring tumour size (D.E.H.) was blinded for treatment allocation, which was administered by another researcher (W.J.A.). Blood and tumour samples were collected 24 hours after the final dexamethasone dose into a 1.5 ml Eppendorf tube with 15 μl heparin (Pfizer, Bentley WA Australia) or 2% FCS/PBS respectively.
Cell lines
The mesothelioma cell line AB1 (RRID: CVCL_4403) for BALB/cArc (RRID: IMSR_ARC:BC) mice and AE17 (RRID: CVCL_4408) for C57BL/6J (RRID: IMSR_ARC:B6) mice were used for all flow cytometry and treatment response experiments. These cell lines have been authenticated by CellBank Australia and grown in RPMI 1640 (ThermoFisher Scientific, Scoresby VIC Australia) supplemented with 20 mM HEPES, 0.05 mM 2-mercaptoethanol, 100 units/ml penicillin (CSL, Melbourne VIC, Australia), 50 μg/ml gentamicin (David Bull Labs, Kewdale, Australia), and 10% NCS (ThermoFisher Scientific, Scoresby VIC Australia). Cells were inoculated into mice after 4 passages from being thawed. These cell lines were regularly tested for Mycoplasma spp every 3 months by PCR and found to be negative.
Treatment schedules for in vivo experiments
Dexamethasone (DBL dexamethasone sodium phosphate, Hospira, Mulgrave VIC Australia), cisplatin (DBL cisplatin, Hospira, Mulgrave VIC Australia), 5-fluorouracil (Fluorouracil Ebewe, Sandoz PTY LTD, Pyrmont NSW Australia), and the checkpoint antibodies anti-CTLA4 (clone 9H10) and anti-PD-L1 (clone MIH5) were diluted in sterile 0.9% sodium chloride before use. Mice were injected with the maximum-tolerated dose of cisplatin (6 mg/kg) or 5-FU (75 mg/kg)Citation40 when tumours were ~20 mm2. Anti-CTLA4 was dosed at 100 μg/mouse and anti-PD-L1 was dosed 3 times with 2-day intervals at 100 μg/mouse. Dexamethasone was given daily for four days at varying dosages beginning the day preceding chemotherapy/checkpoint blockade treatment. As body surface area can be used to accurately assess comparative dosages, an average body surface area of 1.62 m2 for humans and 0.007 m2 for mice was used.Citation24 A dose of 10 mg in humans would be equivalent to a dose of 6.17 mg/m2. This falls between our selected dose range of 1.25 mg/kg (3.75 mg/m2) and 5 mg/kg (15 mg/m2) for flow cytometry and tumour response experiments.Citation24 All treatments were administered intraperitoneally (i.p.) except 5-FU, which was administered intravenously (i.v.) via tail vein injection. Mice were euthanised when tumours reached a size of > 100 mm2, or in case of complete regression, when mice had remained tumour-free for more than 4 weeks.
Hemavet and flow cytometry analysis
Blood and tumour samples were collected and tumour samples dissociated on the gentleMACS octo dissociator using the mouse tumour dissociation kit and protocol (Miltenyi Biotec, Sydney NSW, Australia). For Hemavet analysis, 20 μl blood was run on the Hemavet HV950FS blood analyser (Drew Scientific) to determine absolute number of peripheral blood lymphocytes. For flow cytometry, red blood cell lysis was performed on blood samples using BD FACS lysing buffer (BD Biosciences) before staining. Samples were incubated with Fc block (eBioscience) for 10 minutes, washed and viability staining done using the Zombie UV fixable viability kit (BioLegend). All samples were then stained for surface markers, permeabilised using the Transcription Factor Staining Buffer Set (Life Technologies, Scoresby VIC Australia) and stained for intracellular markers. Samples were fixed using BD Stabilising fixative and run on a BD LSR Fortessa using BD FACSDiva software (BD Biosciences). Data was analysed using FlowJo V10 (Tree Star Inc) and CITRUS (cluster identification, characterisation and regression) (Cytobank Inc). CITRUS is an algorithmic tool that clusters cells into phenotypically similar populations using unsupervised analysis, identifying clusters associated with an experimental or clincal endpoint of interest, independently of manual gating.Citation13 A total of 3 flow cytometry panels were run; one for lymphoid markers, one for myeloid markers and one for immune checkpoints (supplementary Table 1).
Statistical analysis
For Hemavet and flow cytometry experiments, statistical analysis was performed using One-way ANOVA with Dunnett’s multiple comparisons test to compare between control and treated samples. Benjamini-Hochberg correction was used to correct for multiple comparisons using a false discovery rate (FDR) of 0.05.
For depletion experiments we wanted to be able to detect a difference of 50% in absolute lymphocyte numbers. Assuming a power of 0.8 and an alpha of 0.05, we estimated that 6 mice per group would allow us the detection of a difference in lymphocyte counts of 20% (untreated) compared to 10% for dexamethasone-treated mice (SD 5%).
For in vivo response experiments, we wanted to be able to detect a change in response rate from 65% in control groups to 10% in dexamethasone treated groups, for which a group size of 14 was required, assuming a power of 0.8 and an alpha of 0.05, using a chi-square test.
Disclosure of Potential Conflicts of Interest
The authors declare no conflict of interest.
Supplemental Material
Download PDF (1.2 MB)Acknowledgments
We thank Ms Amy Prosser for assistance with setting up the flow cytometry panels and Dr Wallace Langdon for assistance with the Hemavet analysis. This work was funded by project grant 1067113 from the National Health and Medical Research Council (NHMRC). W.J.L is funded by an NHMRC RD Wright Fellowship and a Fellowship from the Cancer Council of Western Australia. The authors acknowledge the facilities, and the scientific and technical assistance of the Australian Microscopy & Microanalysis Research Facility at the Centre for Microscopy, Characterisation & Analysis, The University of Western Australia, a facility funded by the University, State and Commonwealth Governments. The National Centre for Asbestos Related Diseases is funded by a NHMRC Centre for Research Excellence grant 1107043.
Supplementary data
Supplemental data for this article can be accessed on the publisher’s website.
Additional information
Funding
References
- Grunberg SM. Antiemetic activity of corticosteroids in patients receiving cancer chemotherapy: dosing, efficacy, and tolerability analysis. Ann Oncol. 2007 Feb;18(2):1–8. PubMed PMID: 17108149. Epub 2006/ 11/17.eng. doi:10.1093/annonc/mdl347.
- Szatmari I, Nagy L. Nuclear receptor signalling in dendritic cells connects lipids, the genome and immune function. Embo J. 2008;27(18):2353–2362. PubMed PMID: 18716631. Pubmed Central PMCID: PMC2525841. Epub 2008/08/22.eng. doi:10.1038/emboj.2008.160.
- Cain DW, Cidlowski JA. Immune regulation by glucocorticoids. Nat Rev Immunol. 2017 Apr;17(4):233–247. PubMed PMID: 28192415. Epub 2017/02/14.eng. doi:10.1038/nri.2017.1.
- Cook AM, McDonnell AM, Lake RA, Nowak AK. Dexamethasone co-medication in cancer patients undergoing chemotherapy causes substantial immunomodulatory effects with implications for chemo-immunotherapy strategies. OncoImmunology. 2015;5(3):16.
- Paz-Ares L, Luft A, Vicente D, Tafreshi A, Gumus M, Mazieres J, Hermes B, Çay Şenler F, Csőszi T, Fülöp A, et al. Pembrolizumab plus chemotherapy for squamous non-small-cell lung cancer. N Engl J Med. 2018 Sep 25. PubMed PMID: 30280635. Epub 2018/10/04.eng. doi:10.1056/NEJMoa1810865.
- Gandhi L, Rodriguez-Abreu D, Gadgeel S, Esteban E, Felip E, De Angelis F, Domine M, Clingan P, Hochmair MJ, Powell SF, et al. Pembrolizumab plus chemotherapy in metastatic non-small-cell lung cancer. N Engl J Med. 2018 Apr 16. PubMed PMID: 29658856. Epub 2018/04/17.eng. doi:10.1056/NEJMoa1801005.
- Reck M, Luft A, Szczesna A, Havel L, Kim SW, Akerley W, Pietanza MC, Wu Y-L, Zielinski C, Thomas M, et al. Phase III randomized trial of ipilimumab plus etoposide and platinum versus placebo plus etoposide and platinum in extensive-stage small-cell lung cancer. J Clin Oncol. 2016;34(31):3740–3748. PubMed PMID: 27458307. Epub 2016/07/28.eng. doi:10.1200/JCO.2016.67.6601.
- Aston WJ, Fisher SA, Khong A, Mok C, Nowak AK, Lake RA, Lesterhuis WJ. Combining chemotherapy and checkpoint blockade in thoracic cancer: how to proceed? Lung Cancer Manag. 2014;3(6):443–457. doi:10.2217/lmt.14.37.
- McDonnell AM, Lesterhuis WJ, Khong A, Nowak AK, Lake RA, Currie AJ, Robinson BWS. Tumor-infiltrating dendritic cells exhibit defective cross-presentation of tumor antigens, but is reversed by chemotherapy. Eur J Immunol. 2015 Jan;45(1):49–59. PubMed PMID: 25316312. Epub 2014/ 10/16.eng. doi:10.1002/eji.201444722.
- Lesterhuis WJ, Salmons J, Nowak AK, Rozali EN, Khong A, Dick IM, Harken JA, Robinson BW, Lake RA, de Mello RA. Synergistic effect of CTLA-4 blockade and cancer chemotherapy in the induction of anti-tumor immunity. PLoS One. 2013;8(4):e61895. PubMed PMID: 23626745. Pubmed Central PMCID: PMC3633941. Epub 2013/04/30.eng. doi:10.1371/journal.pone.0061895.
- Cook AM, Lesterhuis WJ, Nowak AK, Lake RA. Chemotherapy and immunotherapy: mapping the road ahead. Curr Opin Immunol. 2016 4;39:23–29. doi:10.1016/j.coi.2015.12.003.
- Garant A, Guilbault C, Ekmekjian T, Greenwald Z, Murgoi P, Vuong T. Concomitant use of corticosteroids and immune checkpoint inhibitors in patients with hematologic or solid neoplasms: A systematic review. Crit Rev Oncol Hematol. 2017 Dec;120:86–92. PubMed PMID: 29198341. Epub 2017/ 12/05.eng. doi:10.1016/j.critrevonc.2017.10.009.
- Bruggner RV, Bodenmiller B, Dill DL, Tibshirani RJ, Nolan GP. Automated identification of stratifying signatures in cellular subpopulations. Proc Natl Acad Sci U S A. 2014;111(26):E2770–7. PubMed PMID: 24979804. Pubmed Central PMCID: PMC4084463. Epub 2014/07/01. eng. doi:10.1073/pnas.1408792111.
- Mishler JM, Emerson PM. Development of neutrophilia by serially increasing doses of dexamethasone. Br J Haematol. 1977 Jun;36(2):249–257. PubMed PMID: 871436. Epub 1977/06/01.eng. doi:10.1111/j.1365-2141.1977.tb00646.x.
- Lesterhuis WJ, Rinaldi C, Jones A, Rozali EN, Dick IM, Khong A, Boon L, Robinson BW, Nowak AK, Bosco A, et al. Network analysis of immunotherapy-induced regressing tumours identifies novel synergistic drug combinations. Sci Rep. 2015;5:12298. PubMed PMID: 26193793. eng. doi:10.1038/srep12298.
- Elsoueidi R, Lander MJ, Richa EM, Adane ED. Single-dose dexamethasone for the prevention of pemetrexed associated cutaneous adverse reactions. J Oncol Pharm Pract. 2016 Apr;22(2):271–274. PubMed PMID: 25908647. Epub 2015/ 04/25.eng. doi:10.1177/1078155215583523.
- Maurice-Dror C, Perets R, Bar-Sela G. Glucocorticoids as an adjunct to oncologic treatment in solid malignancies - not an innocent bystander. Crit Rev Oncol Hematol. 2018 Jun;126:37–44. PubMed PMID: 29759565. Epub 2018/05/16.eng. doi:10.1016/j.critrevonc.2018.03.015.
- Amin A, DePril V, Hamid O, Wolchock J, Maio M, Neyns B, Ibrahim R, Hoos A, O'Day S.Evaluation of the effect of systemic corticosteroids for the treatment of immune-related adverse events (irAEs) on the development or maintenance of ipilimumab clinical activity. J Clin Oncol. 2009;27(15S):9037.
- Harmankaya K, Erasim C, Koelblinger C, Ibrahim R, Hoos A, Pehamberger H, Binder M. Continuous systemic corticosteroids do not affect the ongoing regression of metastatic melanoma for more than two years following ipilimumab therapy. Med Oncol. 2011 Dec;28(4):1140–1144. PubMed PMID: 20593249. Epub 2010/ 07/02.eng. doi:10.1007/s12032-010-9606-0.
- Downey SG, Klapper JA, Smith FO, Yang JC, Sherry RM, Royal RE, Kammula US, Hughes MS, Allen TE, Levy CL, et al. Prognostic factors related to clinical response in patients with metastatic melanoma treated by CTL-associated antigen-4 blockade. Clin Cancer Res. 2007;13(22 Pt 1):6681–6688. PubMed PMID: 17982122. Pubmed Central PMCID: PMC2147083. Epub 2007/11/06.eng. doi:10.1158/1078-0432.CCR-07-0187.
- Horvat TZ, Adel NG, Dang TO, Momtaz P, Postow MA, Callahan MK, Carvajal RD, Dickson MA, D’Angelo SP, Woo KM, et al. Immune-related adverse events, need for systemic immunosuppression, and effects on survival and time to treatment failure in patients with melanoma treated with ipilimumab at memorial sloan kettering cancer center. J Clin Oncol. 2015;33(28):3193–3198. PubMed PMID: 26282644. Pubmed Central PMCID: PMC5087335 online at www.jco.org. Author contributions are found at the end of this article. Epub 2015/08/19.eng. doi:10.1200/JCO.2015.60.8448.
- Weber JS, Hodi FS, Wolchok JD, Topalian SL, Schadendorf D, Larkin J, Sznol M, Long GV, Li H, Waxman IM, et al. Safety profile of nivolumab monotherapy: A pooled analysis of patients with advanced melanoma. J Clin Oncol. 2017 Mar;35(7):785–792. PubMed PMID: 28068177. Epub 2017/ 01/10.eng. doi:10.1200/JCO.2015.66.1389.
- Arbour KC, Mezquita L, Long N, Rizvi H, Auclin E, Ni A, Martinez Bernal G, Chaft JE, Ferrara R, Lai W-CV, et al. Deleterious effect of baseline steroids on efficacy of PD-(L)1 blockade in patients with NSCLC. J Clin Oncol. 2018;36(15_suppl):9003. doi:10.1200/JCO.2018.36.15_suppl.9003.
- Nair AB, Jacob S. A simple practice guide for dose conversion between animals and human. J Basic Clin Pharm. 2016 Mar;7(2):27–31. PubMed PMID: 27057123. eng. doi:10.4103/0976-0105.177703.
- Saffar AS, Ashdown H, Gounni AS. The molecular mechanisms of glucocorticoids-mediated neutrophil survival. Curr Drug Targets. 2011 Apr;12(4):556–562. PubMed PMID: 21504070. Pubmed Central PMCID: PMC3267167. Epub 2011/04/20.eng.
- Xing K, Gu B, Zhang P, Wu X. Dexamethasone enhances programmed cell death 1 (PD-1) expression during T cell activation: an insight into the optimum application of glucocorticoids in anti-cancer therapy. BMC Immunol. 2015;16:39. PubMed PMID: 26112261. Pubmed Central PMCID: PMC4480888. Epub 2015/ 06/27.eng. doi:10.1186/s12865-015-0103-2.
- Herr I, Ucur E, Herzer K, Okouoyo S, Ridder R, Krammer PH, von Knebel Doeberitz M, Debatin K-M. Glucocorticoid cotreatment induces apoptosis resistance toward cancer therapy in carcinomas. Cancer Res. 2003 Jun 15;63(12):3112–3120. PubMed PMID: 12810637. Epub 2003/06/18.eng.
- Vincent J, Mignot G, Chalmin F, Ladoire S, Bruchard M, Chevriaux A, Martin F, Apetoh L, Rébé C, Ghiringhelli F. 5-Fluorouracil selectively kills tumor-associated myeloid-derived suppressor cells resulting in enhanced T cell-dependent antitumor immunity. Cancer Res. 2010;70(8):3052–3061. PubMed PMID: 20388795. Epub 2010/04/15.eng. doi:10.1158/0008-5472.CAN-09-3690.
- Wang Z, Till B, Gao Q. Chemotherapeutic agent-mediated elimination of myeloid-derived suppressor cells. Oncoimmunology. 2017;6(7):e1331807. PubMed PMID: 28811975. Pubmed Central PMCID: PMC5543863. Epub 2017/08/16.eng. doi:10.1080/2162402X.2017.1331807.
- Klement JD, Paschall AV, Savage NM, Nayak-Kapoor A, Liu K. 5- Fluorouracil regulation of myeloid-derived suppressor cell differentiation in vitro and in vivo. J Immunol. 2017;198:205.5.
- Hato SV, Khong A, de Vries IJ, Lesterhuis WJ. Molecular pathways: the immunogenic effects of platinum-based chemotherapeutics. Clin Cancer Res. 2014;20(11):2831–2837. PubMed PMID: 24879823. Epub 2014/ 06/01. eng. doi:10.1158/1078-0432.CCR-13-3141.
- Ramakrishnan R, Assudani D, Nagaraj S, Hunter T, Cho HI, Antonia S, Altiok S, Celis E, Gabrilovich DI. Chemotherapy enhances tumor cell susceptibility to CTL-mediated killing during cancer immunotherapy in mice. J Clin Invest. 2010 Apr;120(4):1111–1124. PubMed PMID: 20234093. Pubmed Central PMCID: PMC2846048. Epub 2010/03/18.eng. doi:10.1172/JCI40269.
- Lesterhuis WJ, Punt CJ, Hato SV, Eleveld-Trancikova D, Jansen BJ, Nierkens S, Schreibelt G, de Boer A, Van Herpen CML, Kaanders JH, et al. Platinum-based drugs disrupt STAT6-mediated suppression of immune responses against cancer in humans and mice. J Clin Invest. 2011 Aug;121(8):3100–3108. PubMed PMID: 21765211. Pubmed Central PMCID: PMC3148725. Epub 2011/07/19.eng. doi:10.1172/JCI43656.
- Hato SV, Figdor CG, Takahashi S, Pen AE, Halilovic A, Bol KF, Vasaturo A, Inoue Y, de Haas N, Verweij D, et al. Direct inhibition of STAT signaling by platinum drugs contributes to their anti-cancer activity. Oncotarget. 2017;8(33):54434–54443. PubMed PMID: 28903353. Pubmed Central PMCID: PMC5589592. Epub 2017/09/15.eng. doi:10.18632/oncotarget.17661.
- Nowak A, Kok P, Lesterhuis W, Hughes B, Brown C, Kao S, Karikios D, John T, Pavlakis N, O’Byrne K, et al. OA08.02 DREAM - A phase 2 trial of durvalumab with first line chemotherapy in mesothelioma: final result. J Thoracic Oncol. 2018;13(10):S338–S9. doi:10.1016/j.jtho.2018.08.276.
- Grosso JF, Jure-Kunkel MN. CTLA-4 blockade in tumor models: an overview of preclinical and translational research. Cancer Immun. 2013;13:5. PubMed PMID: 23390376. eng.
- Mosely SI, Prime JE, Sainson RC, Koopmann JO, Wang DY, Greenawalt DM, Ahdesmaki MJ, Leyland R, Mullins S, Pacelli L, et al. Rational selection of syngeneic preclinical tumor models for immunotherapeutic drug discovery. Cancer Immunol Res. 2017 Jan;5(1):29–41. PubMed PMID: 27923825. Epub 2016/ 12/08.eng. doi:10.1158/2326-6066.CIR-16-0114.
- Davies S, Dai D, Pickett G, Leslie KK. Gene regulation profiles by progesterone and dexamethasone in human endometrial cancer Ishikawa H cells. Gynecol Oncol. 2006 Apr;101(1):62–70. PubMed PMID: 16289307. Epub 2005/ 11/18.eng. doi:10.1016/j.ygyno.2005.09.054.
- Lesterhuis WJ, Bosco A, Millward MJ, Small M, Nowak AK, Lake RA. Dynamic versus static biomarkers in cancer immune checkpoint blockade: unravelling complexity. Nat Rev Drug Discov. 2017 Apr;16(4):264–272. PubMed PMID: 28057932. Epub 2017/ 01/07.eng. doi:10.1038/nrd.2016.233.
- Aston WJ, Hope DE, Nowak AK, Robinson BW, Lake RA, Lesterhuis WJ. A systematic investigation of the maximum tolerated dose of cytotoxic chemotherapy with and without supportive care in mice. BMC Cancer. 2017 October 2016. 12 Julyreceived 10 August accepted;17:684. PubMed PMID: PMC5644108. doi:10.1186/s12885-017-3677-7.