ABSTRACT
Caspase 3 (CASP3) has a key role in the execution of apoptosis, and many cancer cells are believed to disable CASP3 as a mechanism of resistance to cytotoxic therapeutics. Alongside, CASP3 regulates stress-responsive immunomodulatory pathways, including secretion of type I interferon (IFN). Here, we report that mouse mammary carcinoma TSA cells lacking Casp3 or subjected to chemical caspase inhibition were as sensitive to the cytostatic and cytotoxic effects of radiation therapy (RT) in vitro as their control counterparts, yet secreted increased levels of type I IFN. This effect originated from the accrued accumulation of irradiated cells with cytosolic DNA, likely reflecting the delayed breakdown of cells experiencing mitochondrial permeabilization in the absence of CASP3. Casp3-/- TSA cells growing in immunocompetent syngeneic mice were more sensitive to RT than their CASP3-proficient counterparts, and superior at generating bona fide abscopal responses in the presence of an immune checkpoint blocker. Finally, multiple genetic signatures of apoptotic proficiency were unexpectedly found to have robust negative (rather than positive) prognostic significance in a public cohort of breast cancer patients. However, these latter findings were not consistent with genetic signatures of defective type I IFN signaling, which were rather associated with improved prognosis. Differential gene expression analysis on patient subgroups with divergent prognosis (as stratified by independent signatures of apoptotic proficiency) identified SLC7A2 as a new biomarker with independent prognostic value in breast cancer patients. With the caveats associated with the retrospective investigation of heterogeneous, public databases, our data suggest that apoptotic caspases may influence the survival of breast cancer patients (or at least some subsets thereof) via mechanisms not necessarily related to type I IFN signaling as they identify a novel independent prognostic biomarker that awaits prospective validation.
Introduction
Apoptosis is a variant of regulated cell death (RCD) that relies on the sequential activation of two or more cysteine-dependent aspartate-directed proteases commonly known as caspases.Citation1 Apoptosis not only provides a major contribution to the fully physiological waves of cellular demise that underlie both (post-)embryonic development and adult tissue homeostasis,Citation2 but is also responsible for the death of malignant cells exposed to adverse microenvironmental conditionsCitation3 or exposed to cytotoxic therapeutic regimens, including chemotherapy and radiation therapy (RT).Citation4 In line with this notion, the ability of progressing tumors to acquire genetic or epigenetic alterations to circumvent stress-driven apoptosis has been recognized as a common hallmark of malignancy as early as two decades ago, well before the establishment of modern immunotherapy.Citation5 Now, it is clear that tumors form, progress and respond to therapy in the context of a complex, bidirectional interaction with the host immune system,Citation6 and that such a crosstalk constitutes a major actionable item from a therapeutic perspective.Citation7 Alongside, it has become accepted that most, if not all, durable clinical responses to conventional chemotherapy, targeted anticancer agents and RT rely on the activation of tumor-targeting immune response.Citation8 Moreover, resistance to cytotoxic therapeutics appears to involve immunological mechanisms way more often than originally suspected. Thus, great attention has been attracted by the links between the activation of stress-responsive pathways by cancer cells, be they successful (i.e., culminating with cell survival) or not (i.e., culminating with cell death), and the configuration of the tumor microenvironment.Citation9,Citation10 In this setting, apoptotic caspases – namely, CASP3, CASP6, CASP7, CASP8, CASP9 and CASP10 – turned out to occupy a key position in the control of signals whereby stress (cancer) cells communicate with the immunological microenvironment.Citation11
CASP3 (the main executor of both intrinsic and extrinsic variants of apoptosis)Citation1 stands out as a major contributor to the establishment of peripheral tolerance against dying cells, at least in part reflecting the need for suppressed immunity in the context of physiological cell death.Citation2,Citation12 Indeed, CASP3 activation drives the exposure of phosphatidylserine (PS) on the outer leaflet of dying cells, which favors the phagocytic activity of macrophages in the context of immunosuppressive signaling,Citation13–Citation16 stimulates the secretion of prostaglandin E2 (PGE2), which actively inhibits immune effector cells,Citation17,Citation18 and that of lysophosphatidylcholine (LPC), which recruits phagocytes to sites of cell death to favor removal of cell corpses in the absence of inflammation.Citation19 Moreover, activation of CASP3 downstream of mitochondrial outer membrane permeabilization (MOMP) and consequent CASP9 activation inhibits the secretion of type I interferon (IFN) driven by the ability of cyclic GMP-AMP synthase (CGAS) to detect mitochondrial DNA (mtDNA) accumulating in the cytoplasm.Citation20–Citation23
CGAS signaling and consequent secretion of type I IFN by irradiated cells are paramount for abscopal responses (i.e., the immunological control of out-of-field malignant lesions following the irradiation of an anatomically distant tumor).Citation24 We therefore set to elucidate the impact of apoptotic caspase activation on type I IFN secretion and responses to RT in a transplantable model of mammary breast carcinoma (TSA cells). Our preclinical findings demonstrate that CASP3 inhibits abscopal responses by accelerating the breakdown of cells that accumulate cytosolic DNA in response to RT, pointing to CASP3 as an actionable target to ameliorate the clinical efficacy of RT. However, bioinformatic analyses on publicly available datasets suggest that type I IFN signaling has a negative impact on the survival of breast cancer patients. Moreover, differential gene expression studies on patient subgroups exhibiting divergent prognosis (as stratified by independent signatures of apoptotic proficiency) identified 11 common genes, 3 of which had never been attributed an impact on survival in this setting. Of these 3 genes, solute carrier family 7 (cationic amino acid transporter, y+ system), member 2 (SLC7A2) had independent prognostic value on multivariate Cox regression analyses. With the caveats linked to retrospective bioinformatic studies of heterogeneous, publicly available databases, our data suggest that apoptotic caspases may influence the survival of breast cancer patients (or at least some subsets thereof) via mechanisms not necessarily related to type I IFN signaling, as they identify a novel, independent prognostic biomarker that awaits prospective validation.
Materials and methods
Chemicals and cell culture
Unless otherwise specified, chemicals have been acquired from Sigma-Aldrich, reagents for cell culture from Gibco™-Thermo Fischer, and plasticware from Corning. Wild-type mouse breast carcinoma TSA cells, as well as control and Casp3-/- TSA cell clones were maintained in DMEM supplemented with 10% fetal bovine serum (FBS), as per ATCC recommendations. Cells between passage 2 and 10 were employed for experimental determinations. Control and Casp3-/- TSA clones were generated via the CRISPR/Cas9 technology based on commercial non-targeting and CASP3-specific guide RNAs from Sigma-Aldrich. Plasmid transfection and clone selection were performed as per the manufacturer’s recommendations.
Flow cytometry
Flow cytometry was employed to determine MOMP, PS exposure and plasma membrane rupture as per standard protocols.Citation25 In brief, MOMP was monitored with the mitochondrial transmembrane potential-sensitive dye 3,3′-dihexyloxacarbocyanine iodide (DiOC6(3), from Invitrogen™-Thermo Fischer) (40 nM), PS exposure with FITC-conjugated annexin V (Biolegend), and plasma membrane rupture with propidium iodide (PI, from Sigma-Aldrich) (0.5 µg/mL). Stained samples were acquired on a MACSQuant® Analyzer 10 (Miltenyi Biotech) and data were analyzed with FlowJo v. 10.6 (FlowJo LLC).
Immunoblotting
Immunoblotting was performed as previously reported,Citation26 with primary antibodies specific for CASP3 (#14220 from Cell Signaling Technology), MAPLC3B (#2775, from Cell Signaling Technology), SQSTM1 (#5114S, from Cell Signaling Technology), or actin, beta (ACTB, #8H10D10, from Cell Signaling Technology), which was employed as loading control. Upon wash and incubation with species-specific horseradish peroxidase (HRP)-conjugated secondary antibodies (from GE Healthcare Life Sciences), revelation was performed with ECL Select Detection Reagent (from GE Healthcare Life Sciences) on a C600 Gel Doc & Western Imaging System (Azure Biosystems).
Immunofluorescence
For immunofluorescence, cells growing on glass coverslip were fixed and permeabilized with 0.1% Tween20 and 0.01% Triton X-100 in PBS, followed by incubation with primary antibodies specific for double-stranded DNA (dsDNA, #ab27156, from Abcam), wash and incubation with species-specific secondary antibodies conjugated to Alexa Fluor® 594 (ab150120, from Abcam).Citation27 Samples were mounted on slides with 4′,6-diamidino-2-phenylindole (DAPI)-containing ProLong™ Diamond Antifade Mountant (from Thermo Fischer), and images were acquired with an Evos® FL Imaging System (Thermo Fischer). dsDNA quantification was performed on 8–10 randomly selected fields per sample, with ImageJ (NIH).
RT-PCR
IFNB1 levels were quantified relative to RPL19 levels, according to standard RT-PCR procedures based on commercial primer sets from Bio-Rad. Amplifications were run on a 7500 RT-PCR system (Applied Biosystems) as per thermal protocols provided by the primer manufacturer, and data were analyzed with the ΔΔCt method.
ELISA
Type I IFN levels in the culture supernatants were quantified with the VERIKINE-HS™ IFN Beta Serum ELISA kit (#42410, from PBL Assay Science), as per the recommendations of the manufacturer.
In vivo studies
Female 4–9-weeks old BALB/c were employed as host for single neoplastic lesions driven by 0.25 × 106 wild-type TSA cells implanted s.c. in one flank, or double neoplastic lesions driven by 0.25 × 106 control or Casp3-/- TSA cell clones implanted s.c. in one flank and 0.25 × 106 control TSA cells implanted s.c. contralaterally. Mice were monitored for tumor growth on the primary (in both experimental settings) and abscopal site (in the double lesion model only) routinely by means of common caliper, and once primary tumors reached a surface area of 15–25 mm2 (day 0), mice were allocated to either of the following treatment groups: (1) control, no treatment or 100 µL vehicle i.p. on days 2, 5 and 8; (2) a single RT fraction of 20 Gy on day 0; (3) 3 RT fractions of 8 Gy each on days 0, 1 and 2; and (4) 3 RT fractions of 8 Gy each on days 0, 1 and 2, combined with a cytotoxic T lymphocyte associated protein 4 (CTLA4)-targeting antibody (#9H10, from Bio X Cell), 200 µg/mouse in 100 µL i.p., on days 2, 5 and 8. RT was delivered with the Small Animal Radiation Research Platform (SARRP, from Xstrahl). Response to treatment and potential toxicities were monitored routinely. Mice experiencing complete tumor regression were re-challenged with wild-type TSA cells
Bioinformatic analyses
All statistical analyses and graphics were performed in the statistical programming language R (version 3.5.2). Gene expression levels and clinicopathological parameters of 1422 breast cancer patients (METABRIC database) for which cancer-specific overall survival was available were acquired from cBioportal (http://www.cbioportal.org/). Survival analysis was performed using the Survival and Survminer R packages, The Kaplan–Meier method was used to estimate patient overall survival rates upon patient stratification into 2 groups based on median mRNA levels of individual genes (AGR3, ANKRD30A, APAF1, BMPR1B, CALML5, CASP3, CASP9, CLIC6, CYP4X1, CXCL9, LTB, SLC7A2, STC2, SUSD3) or the following signatures, calculated as geometric mean of respective genes: BCLs (BCL2, BCL2L1, MCL1), type I IFN signaling (ADAR, B2M, BATF2, BST2, C1S, CASP1, CASP8, CCRL2, CD47, CD74, CMPK2, CNP, CSF1, CXCL10, CXCL11, DDX60, DHX58, EIF2AK2, ELF1, EPSTI1, FAM125A, FAM46A, FTSJD2, GBP2, GBP4, GMPR, HERC6, HLA-C, IFI27, IFI30, IFI35, IFI44, IFI44L, IFIH1, IFIT2, IFIT3, IFITM1, IFITM2, IFITM3, IL15, IL4R, IL7, IRF1, IRF2, IRF7, IRF9, ISG15, ISG20, LAMP3, LAP3, LGALS3BP, LPAR6, LY6E, MOV10, MX1, NCOA7, NMI, NUB1, OAS1, OASL, OGFR, PARP12, PARP14, PARP9, PLSCR1, PNPT1, PRIC285, PROCR, PSMA3, PSMB8, PSMB9, PSME1, PSME2, RIPK2, RNF31, RSAD2, RTP4, SAMD9, SAMD9L, SELL, SLC25A28, SP110, STAT2, TAP1, TDRD7, TMEM140, TRAFD1, TRIM14, TRIM21, TRIM25, TRIM26, TRIM5, TXNIP, UBA7, UBE2L6, USP18, WARS) and interferon gamma (IFNG) signaling (ADAR, APOL6, ARID5B, ARL4A, AUTS2, B2M, BANK1, BATF2, BPGM, BST2, BTG1, C1R, C1S, CASP1, CASP3, CASP4, CASP7, CASP8, CCL2, CCL5, CCL7, CD274, CD38, CD40, CD69, CD74, CD86, CDKN1A, CFB, CFH, CIITA, CMKLR1, CMPK2, CSF2RB, CXCL10, CXCL11, CXCL9, DDX58, DDX60, DHX58, EIF2AK2, EIF4E3, EPSTI1, FAS, FCGR1A, FGL2, FPR1, FTSJD2, GBP4, GBP6, GCH1, GPR18, GZMA, HERC6, HIF1A, HLA-A, HLA-B, HLA-DMA, HLA-DQA1, HLA-DRB1, HLA-G, ICAM1, IDO1, IFI27, IFI30, IFI35, IFI44, IFI44L, IFIH1, IFIT1, IFIT2, IFIT3, IFITM2, IFITM3, IFNAR2, IL10RA, IL15, IL15RA, IL18BP, IL2RB, IL4R, IL6, IL7, IRF1, IRF2, IRF4, IRF5, IRF7, IRF8, IRF9, ISG15, ISG20, ISOC1, ITGB7, JAK2, KLRK1, LAP3, LATS2, LCP2, LGALS3BP, LY6E, LYSMD2, MARCH1, METTL7B, MT2A, MTHFD2, MVP, MX1, MX2, MYD88, NAMPT, NCOA3, NFKB1, NFKBIA, NLRC5, NMI, NOD1, NUP93, OAS2, OAS3, OASL, OGFR, P2RY14, PARP12, PARP14, PDE4B, PELI1, PFKP, PIM1, PLA2G4A, PLSCR1, PML, PNP, PNPT1, PRIC285, PSMA2, PSMA3, PSMB10, PSMB2, PSMB8, PSMB9, PSME1, PSME2, PTGS2, PTPN1, PTPN2, PTPN6, RAPGEF6, RBCK1, RIPK1, RIPK2, RNF213, RNF31, RSAD2, RTP4, SAMD9L, SAMHD1, SECTM1, SELP, SERPING1, SLAMF7, SLC25A28, SOCS1, SOCS3, SOD2, SP110, SPPL2A, SRI, SSPN, ST3GAL5, ST8SIA4, STAT1, STAT2, STAT3, STAT4, TAP1, TAPBP, TDRD7, TNFAIP2, TNFAIP3, TNFAIP6, TNFSF10, TOR1B, TRAFD1, TRIM14, TRIM21, TRIM25, TRIM26, TXNIP, UBE2L6, UPP1, USP18, VAMP5, VAMP8, VCAM1, WARS, XAF1, XCL1, ZBP1, ZNFX1). Log-rank p values <.05 were considered to be statistically significant. Cox proportional hazard analysis was performed to assess the association of clinicopathological parameters or gene expression levels with survival. In multivariate COX regression, the stepwise selection approach was used. Differentially expressed genes (DEGs) were determined using the LIMMA R package.Citation28 Hierarchical clustering analysis was conducted using the ComplexHeatmap package,Citation29 based on the Pearson distance and average clustering method. Pearson correlation analysis among gene expression levels was performed using the Hmisc package and correlation matrix was generated using the Corrplot package. Gene set enrichment analysis (GSEA) was used to investigate the enriched functions of significantly changed genes. The significantly expressed gene list was ranked according to fold changes and compared with sets of genes that were classified according to hallmark gene sets (MsigDB v6.2).
Results
Inhibition of apoptotic caspases does not compromise the therapeutic potential of RT
To investigate the impact of apoptotic caspases on cell death signaling driven by RT, we subjected wild-type TSA cells to a single RT fraction of 8 Gy or 20 Gy, in the absence or presence of the pan-caspase inhibitor Z-Val-Ala-Asp fluoromethyl ketone (Z-VAD-fmk) and assessed several biomarkers of apoptosis, including MOMP and PI uptake (a marker of plasma membrane rupture) 24 and 48 hours post-irradiation. As expected, Z-VAD-fmk robustly inhibited MOMP and PI uptake driven at 24 hours by the prototypic apoptosis inducer staurosporine (STS) (,b)). Consistent with this, short-term exposure of TSA cells to STS for 24 hours resulted in the full proteolytic activation of CASP3 (i.e., generation of the p17 fragment), which could be inhibited by Z-VAD-fmk ()). Irradiated TSA cells begun to acquire biochemical markers of apoptosis 48 hours after treatment (,e)). Accordingly, the Z-VAD-fmk-inhibitable generation of the CASP3 p17 fragment became manifest in TSA cells only 48 hours after irradiation ()). However, Z-VAD-fmk did not confer robust cytoprotection in this setting (,e)), and it failed to affect the clonogenic survival of TSA cells exposed to a single RT fraction of 1 Gy or 3 Gy (,h)).
Figure 1. Chemical caspase inhibition fails to impact on the cytotoxic effects of RT.
(a,b) Percentage of TSA cells manifesting apoptosis-associated mitochondrial outer membrane permeabilization and plasma membrane breakdown, as assessed by flow cytometry upon staining with the mitochondrial transmembrane potential-sensitive dye DiOC6(3) and the vital dye propidium iodide (PI), following 24 hours of culture in control conditions or in the presence of 0.25 μM staurosporine (STS), 20 μM Z-VAD-fmk, or their combination. Representative dotplots (a) and quantitative results (b) are reported. Numbers indicate the percentage of cells in each quadrant. Results are means ± SEM, n = 2–3 independent experiments, ***p < .001 (One-way ANOVA, as compared to untreated cells); ###p < 0.001 (One-way ANOVA, as compared to cells treated with STS). C. Proteolytic CASP3 activation in TSA cells cultured as in (a,b). Actin, beta (ACTB) levels were monitored to ensure equal loading. D,E. Percentage of TSA cells manifesting biochemical markers of apoptosis, as assessed by flow cytometry upon staining with DiOC6(3) and PI 48 hours after γ irradiation with the indicated dose, alone or in presence of 20 μM Z-VAD-fmk. Representative dotplots (d) and quantitative results (e) are reported. Numbers indicate the percentage of cells in each quadrant. Results are means ± SEM, n = 2–3 independent experiments, ***p < .001 (One-way ANOVA, as compared to untreated cells); n.s., not significant (One-way ANOVA, as compared to cells treated with the same dose of radiation only). F. Proteolytic CASP3 activation in TSA cells cultured as in (d,e). ACTB levels were monitored to ensure equal loading. (g,h). Residual clonogenic potential of TSA cells receiving γ irradiation at the indicated dose, alone or in presence of 20 μM Z-VAD-fmk and allowed to generate colonies for 14 days. Representative images (g) and quantitative results (h) are reported. Results are means ± SEM, n = 2–3 independent experiments, ***p < .001 (One-way ANOVA, as compared to untreated cells); n.s., not significant (One-way ANOVA, as compared to cells treated with the same dose of radiation only). SF, survival fraction.
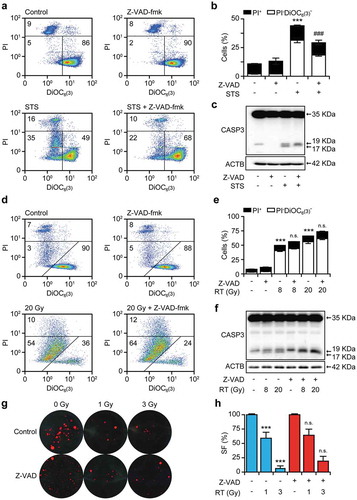
Since Z-VAD-fmk is poorly specific (it inhibits multiple caspases as well as other proteases) and rather unstable,Citation25 we decided to harness the CRISPR/Cas technology to generate TSA cell clones lacking the Casp3 gene. Our focus on CASP3 (over other caspases involved in the execution of apoptosis, like CASP6 and CASP7) was dictated by its multipronged immunosuppressive functions, which are not consistently shared by CASP6 and CASP7.Citation11 Recapitulating data with Z-VAD-fmk, Casp3-/- TSA cells were protected from STS-driven rapid apoptosis as compared to their control counterparts (TSA cells subjected to CRISPR/Cas with a non-targeting guide RNA) (–c)). The Casp3-/- genotype also conferred cytoprotection to TSA cells driven into acute cell death by RT in vitro (–f)). However, Casp3-/- TSA cells did not differ from their control counterparts in residual clonogenic survival upon irradiation with 1 or 3 Gy (,h)). Moreover, Casp3-/- TSA clones growing in immunocompetent syngeneic BALB/c mice were more (not less) sensitive to focal irradiation with a single fraction of 20 Gy as compared their control counterparts, as manifested by improved disease control and increased incidence of tumor eradication (2/10 in control lesions, 4/6 in Casp3-/- lesions) ()). Altogether, these data demonstrate that CASP3 activation influences the early kinetics of RT-driven cell death (i.e., the speed at which irradiated cells die), but fails to impact on its actual occurrence (i.e., the fraction of cells ultimately succumbing to irradiation), in vitro. Moreover, they indicate that the absence of CASP3 enables superior therapeutic responses to RT in vivo, in immunocompetent syngeneic settings. These findings led us to hypothesize that CASP3 may mediate immunosuppressive effects that limit RT efficacy in vivo.
Figure 2. CASP3 is dispensable for the cytotoxic effects of RT in vitro and in vivo.
(a,b) Percentage of control (SCR) and Casp3-/- TSA cells manifesting apoptosis-associated phosphatidylserine exposure and plasma membrane breakdown, as assessed by flow cytometry upon staining with fluorescent AnnexinV (AnnV) and the vital dye propidium iodide (PI), following 24 hours of culture in control conditions or in the presence of 0.1 or 0.25 μM staurosporine (STS). Representative dotplots (a) and quantitative results (b) are reported. Numbers indicate the percentage of cells in each quadrant. Results are means ± SEM, n = 2–3 independent experiments, ***p < .001 (One-way ANOVA, as compared to untreated SCR cells); ###p < .001 (One-way ANOVA, as compared to SCR cells treated with STS). C. Proteolytic CASP3 activation in SCR and Casp3-/- TSA cells cultured as in A,B. Actin, beta (ACTB) levels were monitored to ensure equal loading. D,E. Percentage of SCR and Casp3-/- TSA cells manifesting biochemical markers of apoptosis, as assessed by flow cytometry upon staining with fluorescent AnnV and PI 48 hours after γ irradiation with the indicated dose. Representative dotplots (d) and quantitative results (e) are reported. Numbers indicate the percentage of cells in each quadrant. Results are means ± SEM, n = 2–3 independent experiments, ***p < .001 (One-way ANOVA, as compared to untreated SCR cells); n.s., not significant (One-way ANOVA, as compared to SCR cells treated with the same dose of radiation only). (f) Proteolytic CASP3 activation in SCR and Casp3-/- TSA cells cultured as in (d,e). ACTB levels were monitored to ensure equal loading. (g,h). Residual clonogenic potential of SCR and Casp3-/- TSA cells receiving γ irradiation at the indicated dose, and allowed to generate colonies for 14 days. Representative images (g) and quantitative results (h) are reported. Results are means ± SEM, n = 2–3 independent experiments, ***p < .001 (One-way ANOVA, as compared to untreated SCR cells); n.s., not significant (One-way ANOVA, as compared to SCR cells treated with the same dose of radiation only). SF, survival fraction. (i) Growth of SCR and Casp3-/- TSA cells grafted in immunocompetent syngeneic BALB/c mice and optionally subjected to focal γ irradiation with a single fraction of 20 Gy. Results are means ± SEM, n = 6–10 mice/group from 2 independent experiments. Rate of complete disease eradication is reported. ***p < .001 (Two-way ANOVA, as compared to untreated SCR lesions), ###p < .001 (Two-way ANOVA, as compared to irradiated SCR lesions).
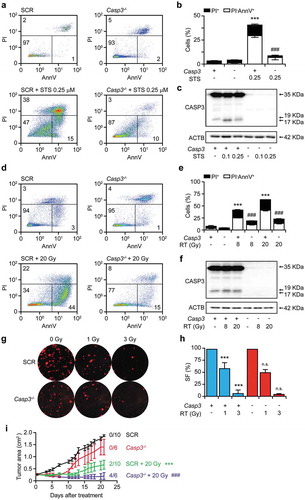
Inhibition of apoptotic caspases exacerbates type I IFN secretion by irradiated cells as it delays the breakdown of cells with cytosolic dsDNA
We next set to investigate the impact of caspase inhibition on type I IFN release by TSA cells exposed to RT in vitro. Recapitulating published data from the Demaria group,Citation24 TSA cells exposed to a single RT fraction of 8 Gy exhibited increased transcription from the IFNB1 locus, which could be further enhanced by the absence of the Casp3 gene ()), reminiscent of previous findings with MOMP-inducing chemical agents from the Vaux and Flavell laboratories.Citation21–Citation23 Similar results were obtained by measuring the amount of type I IFN secreted in culture supernatants by ELISA ()). Surprisingly, Casp3-/- TSA cells also exhibited increased IFNB1 transcription and type I IFN secretion at baseline (,b)). Type I IFN signaling driven by RT in TSA cells depends on the sensing of cytosolic double-stranded DNA (dsDNA) species by CGAS.Citation24 To test whether accrued type I IFN responses enabled by apoptotic caspase inhibition rely on the same mechanism, we quantified cytosolic dsDNA in caspase-proficient versus caspase-deficient TSA cells maintained in control conditions or exposed to a single RT fraction of 8 Gy. In line with published data,Citation24 RT with 8 Gy efficiently caused the accumulation of dsDNA in the cytosol of irradiated cells ()). The absence of Casp3 not only caused a significant increase in ability of RT to cause the accumulation of dsDNA in the cytosol of TSA cells, but also enabled dsDNA accumulation in non-irradiated cells ()), reflecting the ability of Casp3-/- TSA cells to produce type I IFN at baseline (,b)). We interpreted these findings to indicate that apoptotic caspase inhibition promotes the accumulation of cells with cytosolic dsDNA. Consistent with this interpretation, the population of living (PI−) Casp3-/- TSA cells detectable 24 hours after RT exhibited lower mitochondrial transmembrane potential as compared to their caspase-proficient counterparts ()). Of note, this could not be attributed to a general defect in autophagy, which normally ensures the disposal of permeabilized mitochondria.Citation30 Indeed, the starvation-driven lipidation of microtubule associated protein 1 light chain 3 beta (MAP1LC3B) and degradation of sequestosome 1 (SQSTM1, best known as p62), two biochemical markers of autophagy, were not altered by the presence of Z-VAD-fmk and in the absence of Casp3 (Suppl. Figure 1). Altogether, these data suggest that apoptotic caspases inhibit type I IFN secretion by irradiated cells by facilitating the breakdown (and hence complete functional inactivation) of cells undergoing RT-driven MOMP.
Figure 3. CASP3 inhibits type I IFN secretion in vitro and abscopal responses to RT in vivo.
(a). IFNB1 levels in control (SCR) or Casp3-/- TSA cells optionally exposed to γ irradiation (8 Gy) and cultured in control conditions for 48 hours. Results are means ± SEM, n = 2 independent experiments. ***p < .001 (One-way ANOVA, as compared to untreated SCR cells); ###p < .001 (One-way ANOVA, as compared to irradiated SCR cells). (b) Type I IFN levels in the supernatant of SCR or Casp3-/- TSA cells optionally exposed to γ irradiation (8 Gy) and cultured in control conditions for 48 hours. Results are means ± SEM, n = 2 independent experiments. ***p < .001 (One-way ANOVA, as compared to untreated SCR cells); ###p < .001 (One-way ANOVA, as compared to irradiated SCR cells). (c) Cytosolic DNA accumulation in SCR or Casp3-/- TSA cells optionally exposed to γ irradiation (8 Gy) and cultured in control conditions for 24 hours, as assessed by immunofluorescence with a double-stranded DNA-specific antibody. Representative images (scale bar = 10 μm) and quantitative data are reported. Results are means ± SEM and individual data points, n = 2 independent experiments. ***p < .001 (One-way ANOVA, as compared to untreated SCR cells); ###p < .001 (One-way ANOVA, as compared to irradiated SCR cells). D. Mitochondrial transmembrane potential of control (SCR) or Casp3-/- TSA cells optionally exposed to γ irradiation (8 Gy) and cultured in control conditions for 24 hours, as assessed by flow cytometry upon staining with the mitochondrial transmembrane potential-sensitive dye DiOC6(3) and the exclusion dye propidium iodide (PI). PI+ cells (dead cells) are excluded from the analysis. Representative histograms and quantitative data are reported. Results are means ± SEM, n = 2 independent experiments. ###p < .001 (One-way ANOVA, as compared to irradiated SCR cells). (e) Experimental plans for the assessment of abscopal responses. (f) Rejection rate of SCR TSA lesions implanted in syngeneic, immunocompetent BALB/c mice contralaterally to SCR TSA lesions or TSA lesions from 2 distinct Casp3-/- TSA clones (C1 and C2) established 2 days earlier (as per the original schedule depicted in E). Results are from one experiment. (g) Growth of primary (SCR or Casp3-/-, as indicated) and abscopal (SCR) TSA lesions established in syngeneic, immunocompetent BALB/c mice randomly allocated to receive no treatment, focal γ irradiation to the primary lesion in 3 fractions of 8 Gy each, or focal γ irradiation to the primary lesion according to the same schedule plus a CTLA4 blocking antibody (9H10) i.p., as per the revised schedule depicted in (e) Results are means ± SEM, n = 2–10 mice/group from 2 independent experiments. Rate of complete disease eradication at each disease site is reported. ***p < .001, **p < .01, n.s., not significant (Two-way ANOVA, as compared to untreated lesions at the same site).
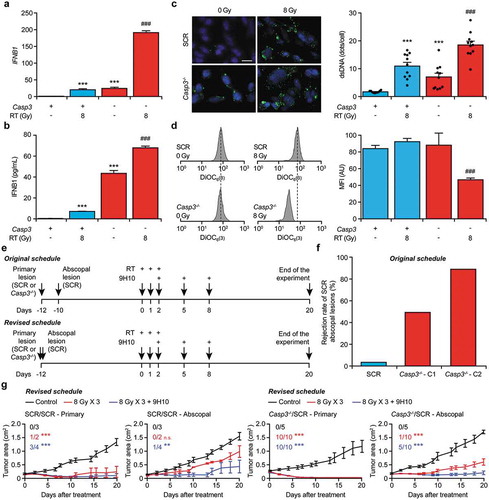
Inhibition of apoptotic caspases exacerbate abscopal responses to RT
To investigate the impact of apoptotic caspases on abscopal responses, we set to employ control or Casp3-/- TSA cell clones to establish primary tumors in the left flank of immunocompetent, syngeneic BALB/c mice, and control TSA clones to generate slightly asynchronous secondary lesions in the right flank. The therapeutic plan was to treat primary tumors of surface area 15–40 mm2 (day 0) with 3 focal RT fractions of 8 Gy each, alone or in combination with systemic inhibition of cytotoxic T lymphocyte associated protein 4 (CTLA4) ()), which per se is known to have no therapeutic activity in this model.Citation24 However, control TSA clones were highly inefficient at generating secondary lesions when contralaterally implanted two days after Casp3-/- (but not control) clones ()), which we interpreted as a sign of the ability of Casp3-/- cells to generate some degree of protective immunity, most likely reflecting their capacity to secrete type I IFN in non-stimulated conditions (–c)). We therefore switched to an alternative experimental plan involving the synchronous establishment of primary and secondary tumors. In this setting, control TSA clones were perfectly able to generate secondary lesions, irrespective of the genotype of contralateral lesions ()). More importantly, the ability of RT combined with systemic CTLA4 inhibition to control the growth of a non-irradiated, distant lesion was greatly ameliorated by the absence of Casp3 ()). Moreover, irradiation of Casp3-/- (but not control) lesions enabled some degree of abscopal control even in the absence of CTLA4 blockage ()). Thus, CASP3 inhibits abscopal responses driven by RT in mice as it limits type I IFN secretion by irradiated cells.
Signatures of apoptotic proficiency convey negative prognostic value in breast cancer
To test the translational value of our findings, we took advantage of METABRIC public database, which provides well-annotated transcriptional data on approximately 2,000 breast tumors,Citation31 and performed survival analyses based on the expression levels of key regulators of apoptosis (–d)) or broader transcriptional signatures of apoptotic proficiency. Consistent with our preclinical findings, stratifying patients with breast cancer from the METABRIC cohort based on median CASP3 expression levels identified a robust cancer-specific survival advantage for individuals with CASP3Lo (as compared to CASP3Hi) lesions (p = .0016) ()). In line with the importance of limited CASP3 activation for superior disease outcome in setting, a similar survival advantage could be documented for patients with breast cancers expressing low (as compared to high) levels of apoptotic peptidase activating factor 1 (APAF1), one of the component of the CASP3-activating platform commonly known as apoptosome (p = .0012) ()),, as well as for patients with breast tumors expressing high levels of BCL2 apoptosis regulator (BCL2), BCL2 like 1 (BCL2L1, best known as BCL-XL) and MCL1 apoptosis regulator, BCL2 family member (MCL1), three core inhibitors of MOMP, which we called BCLsHi patients (p < .0001) ()). Conversely, patients with CASP9Hi breast cancers, which express high levels of another main component of the apoptosome, had superior cancer-specific survival as compared to their CASP9Lo counterparts (p = .0038) ()), potentially pointing to a positive influence of CASP9 on disease outcome independent of its role in the CASP3 activation pathway. Univariate Cox regression analyses on patients from the METABRIC database for whom cancer-specific survival data are available revealed a prognostic value of CASP3 (p < .001), BCLs (p < .001), and CASP9 (p = .011), but not APAF1 (p = .1) (), which was not confirmed on multivariate Cox regressions ().
Table 1. Univariate Cox proportional-hazard regression analysis.
Table 2. Multivariate Cox proportional-hazard regression analysis.
Figure 4. Signatures of apoptotic proficiency are linked to poor survival in breast cancer patients from the METABRIC database.
(a–e) Cancer-specific overall survival (OS) of 1422 breast cancer patients from the METABRIC database upon median stratification based on the expression levels of CASP3 (a), APAF1 (b), geometrically meaned BCL2-BCL2L1-MCL1 (c, BCLs), CASP9 (d), or a gene signature of type I IFN signaling (e). N° at risk and p values are reported.
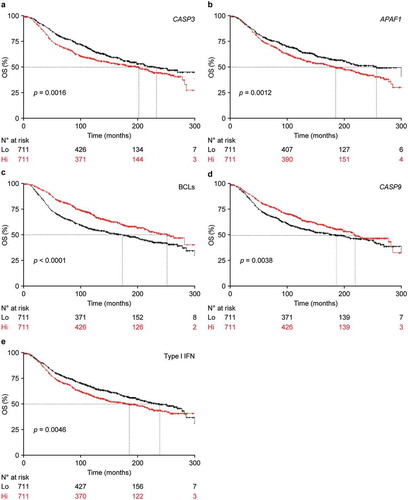
Contrarily to our hypothesis, however, patients in the METABRIC database exhibiting a signature of type I IFN signaling (Type I IFNHi) displayed poor survival as compared to their Type I IFNLo counterparts ()), which is in line with previous observation from others and point to a detrimental role for indolent, chronic (as opposed to robust, acute) type I IFN signaling in the tumor microenvironment.Citation32,Citation33 Consistent with this notion, differential gene expression analysis comparing CASP3Hi, APAF1Hi and BCLsLo patients (the subgroups with inferior survival) to their CASP3Lo, APAF1Lo and BCLsHi counterparts (the subgroups with superior survival), followed by gene set enrichment analysis (GSEA) invariably identified a statistically significant overrepresentation of genes from the Hallmark signatures „interferon alpha response“, „interferon gamma response“, and „inflammatory response“ (–c) and Suppl. Table 1,2). Of note, the reverse was true for CASP9Hi versus CASP9Lo patients () and Suppl. Table 1,2). Together with the overall poor correlation between CASP3 levels, APAF1 levels, BCL2 status, CASP9 levels and signatures of type I IFN or IFNG signaling (Suppl. Figure 2), these findings suggest that general apoptotic proficiency in breast cancer patients may influence survival independent of type I IFN signaling and consequent activation of an IFNG-driven immune response. Of note, the top 2 clusters of breast cancer patients identified by the unsupervised clustering of genes differentially expressed by patients subgroups with different apoptotic proficiency, i.e., CASP3Lo versus CASP3Hi, APAF1Lo versus APAF1Hi, BCLsHi versus BCLsLo, and CASP9Hi versus CASP9Lo, exhibited some (but not complete) overlap with this stratification (–d)) but invariably had increased prognostic significance (Suppl. Figure 3A-D). Intriguingly, patient clusters defined by differential gene expression based on CASP3, BCLs and CASP9 levels were largely overlapping (Suppl. Figure 3E,F), even though CASP3, BCLs and CASP9 levels exhibited very limited correlation (Suppl. Figure 2).
Figure 5. Differential gene expression in breast cancer patients with divergent apoptotic proficiency.
(a–d) Unsupervised hierarchical clustering of differentially expressed genes (DEGs) in 1422 breast cancer patients from the METABRIC database for whom cancer-specific overall survival data are available, subdivided in 2 groups by median expression levels of CASP3 (a), APAF1 (b), geometrically meaned BCL2-BCL2L1-MCL1 (c, BCLs) or CASP9 (d). Gene set enrichment analysis (GSEA) for the Hallmarks terms “interferon alpha response”, “interferon gamma response” is reported. FDR, false discovery rate; NER, normalized enrichment score. See also Supplemental Tables 1 and 2.
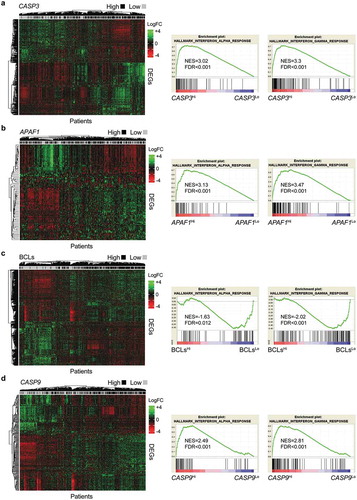
Driven by these findings, we checked for overlaps in the genes differentially expressed by patients with good versus bad prognosis in the METABRIC database as defined by our four independent signatures of apoptotic proficiency, identifying the following 11 genes: cytochrome P450, family 4, subfamily x, polypeptide 1 (CYP4X1), sushi domain containing 3 (SUSD3), anterior gradient 3, protein disulfide isomerase family member (AGR3), ankyrin repeat domain 30A (ANKRD30A), bone morphogenetic protein receptor, type 1B (BMPR1B), chloride intracellular channel 6 (CLIC6), solute carrier family 7 (cationic amino acid transporter, y+ system), member 2 (SLC7A2) and stanniocalcin 2 (STC2), which were all enriched in patients with good prognosis, as well as C-X-C motif chemokine ligand 9 (CXCL9), calmodulin like 5 (CALML5), and lymphotoxin B (LTB), which were all enriched in patients with poor prognosis (,b) and Suppl. Table 3). Strikingly, 7 of these 11 genes have previously been attributed an impact on breast cancer progression and patient survival that is in line with our findings. These genes are AGR3,Citation34 ANKRD30A,Citation35 BMPR1B,Citation36,Citation37 STC2Citation38,Citation39 and SUSD3,Citation40 which are all indicators of early disease or good prognosis, as well as CXCL9,Citation41,Citation42 and CALML5,Citation43 both of which have previously been linked to disease progression or poor prognosis. Conversely, CYP4X1 (which is our hands was enriched in patients with good survival) has previously been positively correlated with tumor grade.Citation44
Figure 6. Signatures of apoptotic proficiency identify novel genes with prognostic value for breast cancer patients.
(a,b) Overlaps among differentially expressed genes (DEGs) enriched in 1422 breast cancer patients from the METABRIC database that exhibit superior (a) or inferior (b) survival upon median stratification based on expression levels of CASP3, APAF1, geometrically meaned BCL2-BCL2L1-MCL1 (BCLs), or CASP9. Genes common to all patients subgroups are indicated. See also Supplemental Table 3. C-H. Cancer-specific overall survival (OS) of 1422 breast cancer patients from the METABRIC database upon median stratification based on the expression levels of AGR3 (c), CALML5 (d), CLIC6 (e), SLC7A2 (f), STC2 (g) or SUSD3 (h). N° at risk and p values are reported.
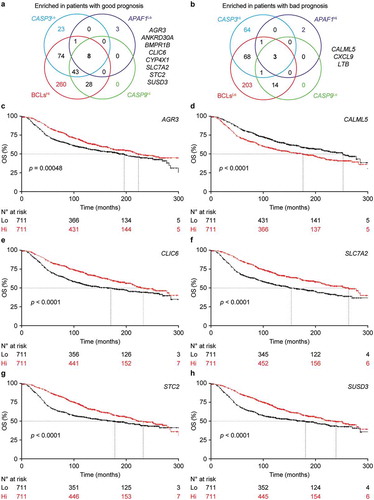
We therefore harnessed the METABRIC database to test the prognostic value of each of these genes taken individually. As per previous reports, patients with AGR3Hi (p = .0048), ANKRD30AHi (p = .034), BMPR1BHi (p = .046), CALML5Lo (p < .00001), CXCL9Lo (p = .029), STC2Hi (p < .0001), and SUSD3Hi (p < .0001) lesion had improved survival as compared to their Lo or Hi counterparts (–h) and data not shown). Alongside, we were unable to attribute to CYP4X1 (p = .062) or LTB (p = .74) any prognostic value in this setting. Conversely, we identified a robust survival advantage for both CLIC6Hi (p < .00001) and SLC7A2Hi (p < .00001) patients (–h)), and neither of these genes had previously been linked to differential survival in patients with breast cancer. Univariate Cox regression analysis confirmed a statistically significant on prognosis for AGR3 (p < .001), ANKRD30A (p = .045), BMPR1B (p = .002), CALML5 (p < .001), CLIC6 (p < .001), SLC7A2 (p < .001), STC2 (p < .001), SUSD3 (p < .001), but not for CXCL9 (p = .21), as it suggested a potential effect for CYP4X1 (p = .032) (). However, prognostic value was corroborated on multivariate COX regression analysis only for CALML5 (p < .0043), SLC7A2 (p < .033), and SUSD3 (p < .012) (). Thus, SLC7A2 stands out as a novel independent prognostic biomarker in this cohort of breast cancer patients.
Discussion
In the late 1990s and early 2000s, caspases have attracted considerable attention as targets for the development of therapeutic interventions against various diseases characterized by the unwarranted loss of post-mitotic cells, including (but not limited to) myocardial infarction and cerebral stroke.Citation45,Citation46 Unfortunately, such efforts failed to culminate with the approval of cardioprotective or neuroprotective agents based on caspase inhibition for use in humans by American or European regulatory agencies. These rather dismal results mainly reflect (1) the complex architecture of cell death signaling in mammalian cells, whereby the inhibition of single signal transducers generally alters the phenotypic, biological and immunological manifestations of RCD, but fails to convey true cytoprotection;Citation47 and (2) the previously unappreciated multifactorial etiology of similar conditions (which generally involve a large immunological component).Citation46
Here, we demonstrate that the inhibition of CASP3 does not compromise the ability of RT to mediate cytostatic and cytotoxic effects against mouse mammary cancer cells in vitro and rather sensitize them to RT in vivo, in immunocompetent syngeneic settings ( and ). Moreover, CASP3 inhibition exacerbates the capacity of irradiated mammary cancer cells to secrete type I IFN as a consequence of decelerated breakdown of cells with permeabilized mitochondria, and hence favors therapeutically relevant cancer-specific immune responses (). To which extent the ability of CASP3 to limit type I IFN secretion in our experimental setting reflected the proteolytic inactivation of CGASCitation48 remains to be elucidated.
Of note, multiple signatures of apoptotic proficiency (rather than deficiency) turned out to be associated with poor disease outcome in patients with breast cancer from the METABRIC database (). However, that was not a reflection of defective type I IFN signaling in the tumor microenvironment, as a genetic signature of type I IFN signaling was associated with inferior (not superior) survival (). As a possibility, these apparently contrasting observations reflect the opposed activity of robust, acute versus indolent, chronic type I IFN signaling in the breast microenvironment.Citation49
Differential gene expression analysis of patient subgroups with good versus bad survival (based on 4 independent signatures of apoptotic proficiency) identified 11 genes similarly enriched in either of these groups, 8 of which had previously been attributed an impact on breast cancer progression or patient survival ( and ).Citation34,Citation37–Citation43 The expression levels of these genes also conveyed prognostic value in patients from the METABRIC dataset ( and and ), with the potential exception of CYP4X1. Of the remaining three genes, LTB failed to impact cancer-specific overall survival in the METABRIC cohort ( and ), while both CLIC6 and SLC7A2 were strongly associated with improved disease outcome ( and and ), although only SLC7A2 as an independent prognostic biomarker (). The molecular mechanisms whereby CLIC6 expression may positively impact on survival remain obscure. Conversely, SLC7A2 (which was enriched in patients with good prognosis) is known for its anti-inflammatory effects,Citation50 at least in part linked to its key role in the immunosuppressive activity of myeloid-derived suppressor cells.Citation51 These observations lend further support to the notion that chronic, indolent inflammation in the breast microenvironment may support disease progression.
With the unavoidable limitations imposed by retrospective in silico studies on large (and hence heterogeneous) public databases, our findings may suggest that apoptotic proficiency influences the survival of breast cancer patients via pathways that are not necessarily linked to type I IFN signaling. Thus, caspase inhibitors may constitute a promising partner for RT for breast cancer patients or subsets thereof. Although no caspase inhibitor is currently available for clinical use, the investigational agent emricasan has been granted Fast Track designation by the US Food and Drug Administration (FDA) in early 2016 for the treatment of liver cirrhosis caused by nonalcoholic steatohepatitis,Citation52 and recent data from randomized trials confirm preliminary observations indicating that emricasan is safe and well tolerated, at least in this non-oncological setting.Citation53,Citation54 Whether emricasan can be safely and efficiently combined with RT for the treatment of breast cancer remains an unexplored possibility.
Disclosures
LG provides remunerated consulting to OmniSEQ (Buffalo, NY, USA), Astra Zeneca (Gaithersburg, MD, USA), Inzen (New York, NY, USA) and the Luke Heller TECPR2 Foundation (Boston, MA, USA), and he is member of the Scientific Advisory Committee of OmniSEQ (Buffalo, NY, USA).
Supplemental Material
Download Zip (5.6 MB)Supplementary material
Supplemental data for this article can be accessed on the publisher’s website.
Additional information
Funding
References
- Galluzzi L, Vitale I, Aaronson SA, Abrams JM, Adam D, Agostinis P, Alnemri ES, Altucci L, Amelio I, Andrews DW, et al. Molecular mechanisms of cell death: recommendations of the nomenclature committee on cell death 2018. Cell Death Differ. 2018;25:486–541. doi:10.1038/s41418-017-0012-4.
- Fuchs Y, Steller H. Live to die another way: modes of programmed cell death and the signals emanating from dying cells. Nat Rev Mol Cell Biol. 2015;16:329–344. doi:10.1038/nrm3999.
- Green DR, Galluzzi L, Kroemer G. Cell biology. Metabolic control of cell death. Science. 2014;345:1250256.
- Tait SW, Green DR. Mitochondria and cell death: outer membrane permeabilization and beyond. Nat Rev Mol Cell Biol. 2010;11:621–632. doi:10.1038/nrm2952.
- Hanahan D, Weinberg RA. The hallmarks of cancer. Cell. 2000;100:57–70. doi:10.1016/s0092-8674(00)81683-9.
- Hanahan D, Weinberg RA. Hallmarks of cancer: the next generation. Cell. 2011;144:646–674. doi:10.1016/j.cell.2011.02.013.
- Galluzzi L, Chan TA, Kroemer G, Wolchok JD, Lopez-Soto A. The hallmarks of successful anticancer immunotherapy. Sci Transl Med. 2018;10. doi:10.1126/scitranslmed.aao4496.
- Galluzzi L, Buque A, Kepp O, Zitvogel L, Kroemer G. Immunological effects of conventional chemotherapy and targeted anticancer agents. Cancer Cell. 2015;28:690–714. doi:10.1016/j.ccell.2015.10.012.
- Ichim G, Tait SW. A fate worse than death: apoptosis as an oncogenic process. Nat Rev Cancer. 2016;16:539–548. doi:10.1038/nrc.2016.58.
- Galluzzi L, Yamazaki T, Kroemer G. Linking cellular stress responses to systemic homeostasis. Nat Rev Mol Cell Biol. 2018;19:731–745. doi:10.1038/s41580-018-0068-0.
- Galluzzi L, Lopez-Soto A, Kumar S, Kroemer G. Caspases connect cell-death signaling to organismal homeostasis. Immunity. 2016;44:221–231. doi:10.1016/j.immuni.2016.01.020.
- Yatim N, Cullen S, Albert ML. Dying cells actively regulate adaptive immune responses. Nat Rev Immunol. 2017;17:262–275. doi:10.1038/nri.2017.9.
- Kiss RS, Elliott MR, Ma Z, Marcel YL, Ravichandran KS. Apoptotic cells induce a phosphatidylserine-dependent homeostatic response from phagocytes. Curr Biol. 2006;16:2252–2258. doi:10.1016/j.cub.2006.09.043.
- Li MO, Sarkisian MR, Mehal WZ, Rakic P, Flavell RA. Phosphatidylserine receptor is required for clearance of apoptotic cells. Science. 2003;302:1560–1563. doi:10.1126/science.1087621.
- Segawa K, Kurata S, Yanagihashi Y, Brummelkamp TR, Matsuda F, Nagata S. Caspase-mediated cleavage of phospholipid flippase for apoptotic phosphatidylserine exposure. Science. 2014;344:1164–1168. doi:10.1126/science.1252809.
- Suzuki J, Denning DP, Imanishi E, Horvitz HR, Nagata S. Xk-related protein 8 and CED-8 promote phosphatidylserine exposure in apoptotic cells. Science. 2013;341:403–406. doi:10.1126/science.1236758.
- Huang Q, Li F, Liu X, Li W, Shi W, Liu FF, O'Sullivan B, He Z, Peng Y, Tan AC, et al. Caspase 3-mediated stimulation of tumor cell repopulation during cancer radiotherapy. Nat Med. 2011;17:860–866. doi:10.1038/nm.2385.
- Zelenay S, van der Veen AG, Bottcher JP, Snelgrove KJ, Rogers N, Acton SE, Chakravarty P, Girotti MR, Marais R, Quezada SA, et al. Cyclooxygenase-dependent tumor growth through evasion of immunity. Cell. 2015;162:1257–1270. doi:10.1016/j.cell.2015.08.015.
- Lauber K, Bohn E, Krober SM, Xiao YJ, Blumenthal SG, Lindemann RK, Marini P, Wiedig C, Zobywalski A, Baksh S, et al. Apoptotic cells induce migration of phagocytes via caspase-3-mediated release of a lipid attraction signal. Cell. 2003;113:717–730. doi:10.1016/s0092-8674(03)00422-7.
- Galluzzi L, Vanpouille-Box C, Bakhoum SF, Demaria S. SnapShot: CGAS-STING signaling. Cell. 2018;173:276–276 e271. doi:10.1016/j.cell.2018.03.015.
- Rongvaux A, Jackson R, Harman CC, Li T, West AP, de Zoete MR, Wu Y, Yordy B, Lakhani SA, Kuan CY, et al. Apoptotic caspases prevent the induction of type I interferons by mitochondrial DNA. Cell. 2014;159:1563–1577. doi:10.1016/j.cell.2014.11.037.
- White MJ, McArthur K, Metcalf D, Lane RM, Cambier JC, Herold MJ, van Delft MF, Bedoui S, Lessene G, Ritchie ME, et al. Apoptotic caspases suppress mtDNA-induced STING-mediated type I IFN production. Cell. 2014;159:1549–1562. doi:10.1016/j.cell.2014.11.036.
- McArthur K, Whitehead LW, Heddleston JM, Li L, Padman BS, Oorschot V, Geoghegan ND, Chappaz S, Davidson S, San Chin H, et al. BAK/BAX macropores facilitate mitochondrial herniation and mtDNA efflux during apoptosis. Science. 2018;359. doi:10.1126/science.aao6047.
- Vanpouille-Box C, Alard A, Aryankalayil MJ, Sarfraz Y, Diamond JM, Schneider RJ, Inghirami G, Coleman CN, Formenti SC, Demaria S. DNA exonuclease Trex1 regulates radiotherapy-induced tumour immunogenicity. Nat Commun. 2017;8:15618. doi:10.1038/ncomms15618.
- Galluzzi L, Aaronson SA, Abrams J, Alnemri ES, Andrews DW, Baehrecke EH, Bazan NG, Blagosklonny MV, Blomgren K, Borner C, et al. Guidelines for the use and interpretation of assays for monitoring cell death in higher eukaryotes. Cell Death Differ. 2009;16:1093–1107. doi:10.1038/cdd.2009.44.
- Morselli E, Shen S, Ruckenstuhl C, Bauer MA, Marino G, Galluzzi L, Criollo A, Michaud M, Maiuri MC, Chano T, et al. p53 inhibits autophagy by interacting with the human ortholog of yeast Atg17, RB1CC1/FIP200. Cell Cycle. 2011;10:2763–2769. doi:10.4161/cc.10.16.16868.
- Michels J, Vitale I, Galluzzi L, Adam J, Olaussen KA, Kepp O, Senovilla L, Talhaoui I, Guegan J, Enot DP, et al. Cisplatin resistance associated with PARP hyperactivation. Cancer Res. 2013;73:2271–2280. doi:10.1158/0008-5472.CAN-12-3000.
- Ritchie ME, Phipson B, Wu D, Hu Y, Law CW, Shi W, Smyth GK. limma powers differential expression analyses for RNA-sequencing and microarray studies. Nucleic Acids Res. 2015;43:e47. doi:10.1093/nar/gkv007.
- Gu Z, Eils R, Schlesner M. Complex heatmaps reveal patterns and correlations in multidimensional genomic data. Bioinformatics. 2016;32:2847–2849. doi:10.1093/bioinformatics/btw313.
- Galluzzi L, Baehrecke H, Ballabio A, Boya P, Bravo-San Pedro JM, Cecconi F, Choi AM, Chu CT, Codogno P, Colombo MI, et al. Molecular definitions of autophagy and related processes. Embo J. 2017;36:1811–1836. doi:10.15252/embj.201796697.
- Curtis C, Shah SP, Chin SF, Turashvili G, Rueda OM, Dunning MJ, Speed D, Lynch AG, Samarajiwa S, Yuan Y, et al. The genomic and transcriptomic architecture of 2,000 breast tumours reveals novel subgroups. Nature. 2012;486:346–352. doi:10.1038/nature10983.
- Weichselbaum RR, Ishwaran H, Yoon T, Nuyten DS, Baker SW, Khodarev N, Su AW, Shaikh AY, Roach P, Kreike B, et al. An interferon-related gene signature for DNA damage resistance is a predictive marker for chemotherapy and radiation for breast cancer. Proc Natl Acad Sci U S A. 2008;105:18490–18495. doi:10.1073/pnas.0809242105.
- Erdal E, Haider S, Rehwinkel J, Harris AL, McHugh PJ. A prosurvival DNA damage-induced cytoplasmic interferon response is mediated by end resection factors and is limited by Trex1. Genes Dev. 2017;31:353–369. doi:10.1101/gad.289769.116.
- Obacz J, Brychtova V, Podhorec J, Fabian P, Dobes P, Vojtesek B, Hrstka R. Anterior gradient protein 3 is associated with less aggressive tumors and better outcome of breast cancer patients. Onco Targets Ther. 2015;8:1523–1532. doi:10.2147/OTT.S82235.
- Chen C, Qin F, Zhang H, Liu X, Guo C, Zhang M, Gu F, Fu L, Ma Y. Microarray expression profiling of dysregulated long non-coding RNAs in triple-negative breast cancer. Cancer Biol Ther. 2015;16:856–865. doi:10.1080/15384047.2015.1040957.
- Bokobza SM, Ye L, Kynaston HE, Mansel RE, Jiang WG. Reduced expression of BMPR-IB correlates with poor prognosis and increased proliferation of breast cancer cells. Cancer Genomics Proteomics. 2009;6:101–108.
- Dai K, Qin F, Zhang H, Liu X, Guo C, Zhang M, Gu F, Fu L, Ma Y. Low expression of BMPRIB indicates poor prognosis of breast cancer and is insensitive to taxane-anthracycline chemotherapy. Oncotarget. 2016;7:4770–4784. doi:10.18632/oncotarget.6613.
- Esseghir S, Kennedy A, Seedhar P, Nerurkar A, Poulsom R, Reis-Filho JS, Isacke CM. Identification of NTN4, TRA1, and STC2 as prognostic markers in breast cancer in a screen for signal sequence encoding proteins. Clin Cancer Res. 2007;13:3164–3173. doi:10.1158/1078-0432.CCR-07-0224.
- Coulson-Gilmer C, Humphries MP, Sundara Rajan S, Droop A, Jackson S, Condon A, Cserni G, Jordan LB, Jones LJ, Kanthan R, et al. Stanniocalcin 2 expression is associated with a favourable outcome in male breast cancer. J Pathol Clin Res. 2018;4:241–249. doi:10.1002/cjp2.106.
- Aushev VN, Lee E, Zhu J, Gopalakrishnan K, Li Q, Teitelbaum SL, Wetmur J, Degli Esposti D, Hernandez-Vargas H, Herceg Z, et al. Novel predictors of breast cancer survival derived from miRNA activity analysis. Clin Cancer Res. 2018;24:581–591. doi:10.1158/1078-0432.CCR-17-0996.
- Franzen B, Alexeyenko A, Kamali-Moghaddam M, Hatschek T, Kanter L, Ramqvist T, Kierkegaard J, Masucci G, Auer G, Landegren U, et al. Protein profiling of fine-needle aspirates reveals subtype-associated immune signatures and involvement of chemokines in breast cancer. Mol Oncol. 2019;13:376–391. doi:10.1002/1878-0261.12410.
- Ma X, Norsworthy K, Kundu N, Rodgers WH, Gimotty PA, Goloubeva O, Lipsky M, Li Y, Holt D, Fulton A. CXCR3 expression is associated with poor survival in breast cancer and promotes metastasis in a murine model. Mol Cancer Ther. 2009;8:490–498. doi:10.1158/1535-7163.MCT-08-0485.
- Debald M, Schildberg FA, Linke A, Walgenbach K, Kuhn W, Hartmann G, Walgenbach-Brunagel G. Specific expression of k63-linked ubiquitination of calmodulin-like protein 5 in breast cancer of premenopausal patients. J Cancer Res Clin Oncol. 2013;139:2125–2132. doi:10.1007/s00432-013-1541-y.
- Murray GI, Patimalla S, Stewart KN, Miller ID, Heys SD. Profiling the expression of cytochrome P450 in breast cancer. Histopathology. 2010;57:202–211. doi:10.1111/j.1365-2559.2010.03606.x.
- Hyman BT, Yuan J. Apoptotic and non-apoptotic roles of caspases in neuronal physiology and pathophysiology. Nat Rev Neurosci. 2012;13:395–406. doi:10.1038/nrn3228.
- Bonora M, Wieckowski MR, Sinclair DA, Kroemer G, Pinton P, Galluzzi L. Targeting mitochondria for cardiovascular disorders: therapeutic potential and obstacles. Nat Rev Cardiol. 2019;16:33–55. doi:10.1038/s41569-018-0074-0.
- Galluzzi L, Bravo-San Pedro JM, Vitale I, Aaronson SA, Abrams JM, Adam D, Alnemri ES, Altucci L, Andrews D, Annicchiarico-Petruzzelli M, et al. Essential versus accessory aspects of cell death: recommendations of the NCCD 2015. Cell Death Differ. 2015;22:58–73. doi:10.1038/cdd.2014.137.
- Ning X, Wang Y, Jing M, Sha M, Lv M, Gao P, Zhang R, Huang X, Feng JM, Jiang Z. Apoptotic Caspases suppress Type I interferon production via the cleavage of cGAS, MAVS, and IRF3. Mol Cell. 2019;74:19–31 e17. doi:10.1016/j.molcel.2019.02.013.
- Vanpouille-Box C, Demaria S, Formenti SC, Galluzzi L. Cytosolic DNA sensing in organismal tumor control. Cancer Cell. 2018;34:361–378. doi:10.1016/j.ccell.2018.05.013.
- Coburn LA, Singh K, Asim M, Barry DP, Allaman MM, Al-Greene NT, Hardbower DM, Polosukhina D, Williams CS, Delgado AG, et al. Loss of solute carrier family 7 member 2 exacerbates inflammation-associated colon tumorigenesis. Oncogene. 2019;38:1067–1079.
- Cimen Bozkus C, Elzey BD, Crist SA, Ellies LG, Ratliff TL. Expression of cationic amino acid transporter 2 is required for myeloid-derived suppressor cell-mediated control of T cell immunity. J Immunol. 2015;195:5237–5250. doi:10.4049/jimmunol.1500959.
- Rotman Y, Sanyal AJ. Current and upcoming pharmacotherapy for non-alcoholic fatty liver disease. Gut. 2017;66:180–190. doi:10.1136/gutjnl-2016-312431.
- Shiffman M, Freilich B, Vuppalanchi R, Watt K, Chan JL, Spada A, Hagerty DT, Schiff E. Randomised clinical trial: emricasan versus placebo significantly decreases ALT and caspase 3/7 activation in subjects with non-alcoholic fatty liver disease. Aliment Pharmacol Ther. 2019;49:64–73. doi:10.1111/apt.15030.
- Mehta G, Rousell S, Burgess G, Morris M, Wright G, McPherson S, Frenette C, Cave M, Hagerty DT, Spada A, et al. A placebo-controlled, multicenter, double-blind, phase 2 randomized trial of the pan-caspase inhibitor emricasan in patients with acutely decompensated cirrhosis. J Clin Exp Hepatol. 2018;8:224–234. doi:10.1016/j.jceh.2017.11.006.