ABSTRACT
Cancer cells exploit CD47 overexpression to inhibit phagocytic elimination and neoantigen processing via the myeloid CD47-SIRPα axis and thereby indirectly evade adaptive T cell immunity. Here, we report on a hitherto unrecognized direct immunoinhibitory feature of cancer cell-expressed CD47. We uncovered that in response to IFNγ released during cognate T cell immune attack, cancer cells dynamically enhance CD47 cell surface expression, which coincides with acquiring adaptive immune resistance toward pro-apoptotic effector T cell mechanisms. Indeed, CRISPR/Cas9-mediated CD47-knockout rendered cancer cells more sensitive to cognate T cell immune attack. Subsequently, we developed a cancer-directed strategy to selectively overcome CD47-mediated adaptive immune resistance using bispecific antibody (bsAb) CD47xEGFR-IgG2s that was engineered to induce rapid and prolonged cancer cell surface displacement of CD47 by internalization. Treatment of CD47pos cancer cells with bsAb CD47xEGFR-IgG2s potently enhanced susceptibility to cognate CD8pos T cells. Targeting CD47-mediated adaptive immune resistance may open up new avenues in cancer immunotherapy.
Introduction
CD47 is a pleiotropic glycoprotein that is broadly expressed in normal cells and that is best known for its regulatory ‘don’t-eat-me’ function in cell homeostasis. In this process, cell surface-expressed CD47 interacts with the inhibitory signal regulatory protein α (SIRPα) that is expressed in various types of phagocytes and antigen-presenting cells, including macrophages and dendritic cells. A broad variety of hematologic and solid malignancies overexpress CD47 to inhibit phagocytic elimination.Citation1–3 Moreover, CD47 overexpression inhibits neoantigen processing via the myeloid CD47-SIRPα axis, thereby protecting cancer cells against elimination by adaptive T cell immunity, albeit in an indirect manner.Citation4,Citation5 Previously, it was demonstrated that CD47-blocking antibodies restore phagocytic elimination of CD47-overexpressing cancer cells and promote the subsequent induction of antitumor adaptive T cell-mediated immunity.Citation4
These observations have led to the current prevailing paradigm that cancer cells overexpress CD47 to indirectly prevent elimination by anticancer T cell immunity. However, recent reports have suggested that cancer cells may also exploit CD47 overexpression to somehow directly affect their susceptibility to the anticancer activity of cytotoxic T cells.Citation6,Citation7 Unfortunately, it proved cumbersome to provide compelling evidence for this suggestion in vivo using immunocompetent (humanized) mouse tumor models.
To address this issue, we applied an in vitro model system using a novel T cell engager, designated Retarg-1, that allows to selectively ‘decorate’ EpCAM-expressing cancer cells with an HLA-B7/CMV-peptide complex. Subsequently, Retarg-1-decorated cancer cells were subjected to immune attack by cognate CMVpp65-specific CD8pos T cells and analyzed for acquisition of adaptive resistance to T cell-mediated cytotoxicity.
Here, we demonstrate for the first time that CD47-overexpressing cancer cells under T cell immune attack respond by dynamic upregulation of CD47 cell surface expression levels, which coincides with acquiring enhanced resistance to the pro-apoptotic T cell effector molecules TRAIL and FASL. Moreover, we demonstrate that CRISPR/Cas9-mediated knockout (KO) and siRNA-mediated knockdown (KD) of CD47 expression by cancer cells significantly increased their susceptibility to TRAIL- and FASL-mediated cell death.
Consequently, we propose to use bispecific antibody (bsAb) CD47xEGFR-IgG2s with potent capacity to simultaneously target and internalize EGFR and CD47, as a potential therapeutic agent to selectively enhance the intrinsic susceptibility of EGFRpos/CD47pos cancer cells to the pro-apoptotic effector mechanisms of anticancer T cells.
Results
Cancer cells under T cell immune attack upregulate cell surface CD47
CD47 expression levels by cancer cells under immune attack of cognate CD8pos T cell were evaluated using a model system in which EpCAMpos PC-3 cells were rendered recognizable to the potent cytotoxic activity of CMVpp65-specific T cells by decorating them with Retarg-1, a soluble EpCAM-directed HLA-B*07:02 fragment genetically equipped with pp65 peptide TPRVTGGGAM. In this process, we noted a viable (Annexin-Vneg) subpopulation of Retarg-1-decorated PC-3 cells that appeared to have evaded from elimination by CMVpp65-specific T cells and which showed an up to fourfold enhanced overexpression of CD47 (). Of note, these PC-3.CD47high cells also showed a > 10-fold increase in PD-L1 expression, a feature which is known to incapacitate anticancer T cells (). Similarly, enhanced CD47 expression was observed when treating EpCAMpos (solid) cancer cell lines PC-3, NCI-H292, OvCAR-3 and FaDu ().
Figure 1. Cancer cells under T cell immune attack upregulate cell surface CD47
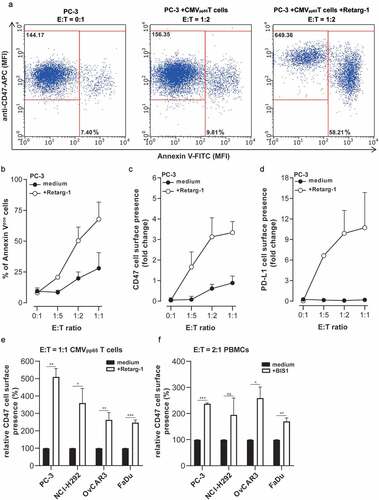
Analogously, treatment of EpCAMpos cancer cells with CD3pos T cells redirected with the EpCAM-directed CD3-agonistic bsAb (BIS1), resulted in a ~twofold increase in CD47 expression by cancer cells that had managed to escape from elimination (). Importantly, flow cytometric analysis indicated that treatments with Retarg-1 or BIS1 are not cytotoxic to the respective effector T cells (data not shown).
Of note, Retarg-1 exclusively redirects cognate HLA-matched and ready-to-go CMVpp65-specific T cells to kill EpCAM-expressing PC-3 cells, whereas BIS1 acts by activating essentially all CD3pos T cells present and then redirecting them to eliminate PC-3 cells in an HLA-independent manner irrespective of their intrinsic TCR specificity. Moreover, CD3-based bispecific T cell engagers like BIS1 are known to induce massive release of proinflammatory cytokines, whereas engagement of HLA-matched cognate CD8pos T cells by Retarg-1 is accompanied by a more physiologically relevant cytokine release. Nevertheless, the data presented in demonstrated that the immune attacks induced by these very different types of T cell-engaging agents clearly modulated the level of cancer cell-expressed CD47.
T cell-produced IFNγ induces upregulation of CD47 on cancer cells
Incubation of cancer cells using medium conditioned by anti-CD2/-CD3/-CD28 bead-activated T cells resulted in a >twofold upregulation of CD47 expression compared to medium conditioned by resting T cells (Suppl. Figure S1a). CD47 upregulation was largely abrogated in the presence of excess amounts of a neutralizing anti-IFNγ antibody. Treatment of NCI-H292 cells with recombinant IFNγ for 48 h resulted in a threefold upregulation of CD47 and eightfold upregulation of PD-L1, respectively (Suppl. Figure S1b).
IFNγ is main inducer for upregulation of CD47 expression by solid cancer types
A strong positive Spearman correlation could be calculated between mRNA expression of the CD47 gene and that of genes involved in the signaling pathways of type I and II IFN for various solid cancer types which suggested that interferons are involved in the upregulation of CD47 expression (Suppl. Figure S2a). To validate this in silico correlation in vitro, flow cytometry was used to assess the respective capacities of IFNα, IFNβ, IFNω and IFNγ to enhance CD47 expression in a panel of solid cancer cell lines, including FaDu, NCI-H292, 92–1 and A172. Treatment with recombinant IFNγ resulted in an >twofold upregulation of CD47 in all cell lines tested, while treatment with type I interferons only marginally enhanced CD47 expression (Suppl. Figure 2Sb).
Next, the respective capacities of IFNγ and TNFα to upregulate CD47 expression by solid and non-solid cancer cell lines were analyzed by flow cytometry. Treatment with IFNγ resulted in an approximate twofold upregulation of CD47 in solid cancer cell lines, whereas treatment with TNFα only showed marginal capacity to upregulate CD47 in MCF-7 and OvCAR-3 cells (Suppl. Figure S2c). Identical treatment regime of non-solid cancer cell types essentially failed to upregulate CD47. Treatment of solid cancer cell lines A172, NCI-H292, PC-3, 92–1, GIST882 and FaDu, with IFNγ and TNFα only marginally enhanced CD47 expression, whereas PD-L1 expression was enhanced up to 10-fold (Suppl. Figures S2D and E).
Importantly, treatment of primary patient-derived (solid) tumor samples with IFNγ resulted in the upregulation of CD47 in 6 out of 6 samples, whereas treatment with TNFα only marginally induced the upregulation of CD47 in one patient sample (Suppl. Figure S2c).
Upregulation of CD47 expression enhances resistance of cancer cells to T cell-mediated cytotoxicity
Co-treatment of PC-3 cells with IFNγ and TNFα resulted in a ~threefold upregulation of CD47 expression (Suppl. Figure S2d). A similar enhancement of CD47 expression level was reached when PC-3 cells were subjected to medium conditioned by bead-activated T cells (Suppl. Figure S1a). Treatment of Retarg-1-decorated PC-3 cells with IFNγ and TNFα significantly (p < .001) enhanced resistance to the anticancer activity by HLA-matched CMVpp65-specific T cells (), since cancer cell death dropped from ~75% to ~50%. Identical treatment using Retarg-1-decorated PC-3.CD47KO cells failed to enhance their resistance toward T cell-mediated cytotoxicity (). Of note, PC-3 and PC-3.CD47KO cells were confirmed to have similar EpCAM cell surface expression levels (Suppl. Figure S3a).
Figure 2. Upregulation of CD47 expression enhances resistance of cancer cells to T cell-mediated cytotoxicity
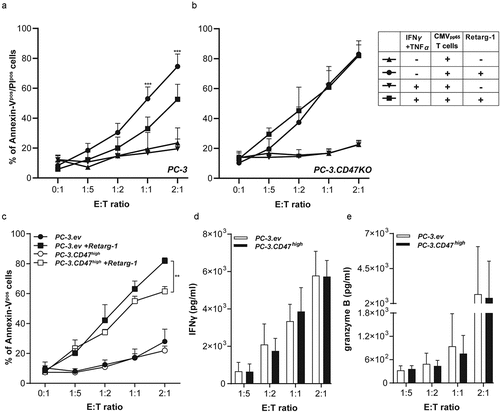
Ectopic overexpression of CD47 enhances resistance of cancer cells to T cell-mediated cytotoxicity
CD47-encoding lentiviral particles were titrated to generate PC-3.CD47high cells with a ~fourfold enhanced CD47 expression level compared to PC-3 cells transduced with empty vector (ev)-based lentiviral particles (PC-3.ev, Suppl. Figure S3b).
Treatment of Retarg-1-decorated PC-3.CD47high cells with CMVpp65-specific T cells (E:T cell ratio = 2:1) resulted in cancer cell death of 60%, whereas identical treatment of Retarg-1-decorated PC-3.ev cells induced up to 80% cancer cell death (p < .001) ().
This indicated that PC-3.CD47high cells are significantly more resistant to T cell-mediated cytotoxicity than PC-3.ev cells. Of note, in both situations, the cognate cytotoxic T cells excreted similar levels of IFNγ and granzyme B, indicating that the observed results are not attributable to intra-assay variation in cytotoxic activity ().
CD47 knockout enhances susceptibility of cancer cells to T cell-mediated cytotoxicity
Compared to the parental PC-3 cells, treatment of PC-3.CD47KO cells with PBMCs in the presence of BIS1 resulted in enhanced cell death, which reached significance at E:T cell ratio 2:1 (, Suppl. Figure S5). Next, we evaluated whether knocking-out CD47 in the CTL-resistant squamous cell carcinoma cell line, FaDu would sensitize these cells to T cell-mediated cell death. Compared to FaDu parental cells, FaDu.CD47KO showed a ~twofold increase in cancer cell death at all E:T cell ratios evaluated (). Subsequently, the coculture of BIS1-redirected PBMCs with FaDu or FaDu.CD47KO cells was selected as particularly useful model system for further investigation. After 48 h, co-cultures of BIS1-redirected PBMCs with FaDu or FaDu.CD47KO cells contained similar percentages of CD69pos/CD3pos T cells () and similar levels of IFNγ and granzyme B (), indicating that enhanced killing of FaDu.CD47KO cells was not attributable to differences in cytotoxic capacity of the respective immune effector cells. Moreover, in contrast to parental FaDu cells, vitality of FaDu.CD47KO cancer cells was dramatically reduced upon treatment with PBMCs redirected by T cell-engaging bsAb BIS1 in RTCA cell viability analysis (). Of note, treatment conditions in which cancer cells were incubated with BIS1 or Retarg-1 in the absence of effector cells showed no effect on growth characteristics (data not shown).
Figure 3. CD47 knockout enhances susceptibility of cancer cells to T cell-mediated cytotoxicity
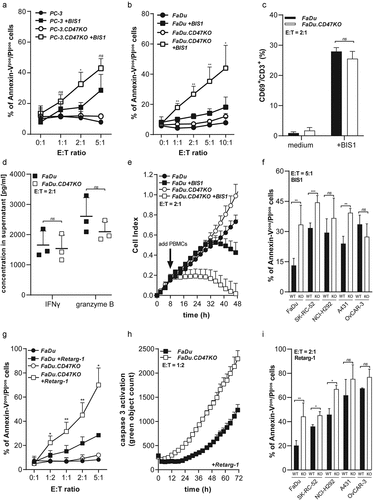
Similarly, treatment of EpCAMpos cell lines (FaDu, SK-RC-52, NCI-H292, A431 and OvCAR-3) versus the corresponding CD47-KO versions thereof with BIS1-redirected PBMCs resulted in the induction of a significantly enhanced percentage of Annexin-Vpos/PIpos cells for target cell lines FaDu.CD47KO, SK-RC-52.CD47KO and A431.CD47KO ().
CD47 knockout enhances susceptibility of cancer cells to Retarg-1-induced T cell cytotoxicity
Treatment of Retarg-1-decorated FaDu.CD47KO cells with CMVpp65-specific T cells (E:T cell ratio = 5:1 for 48 h) resulted in threefold (p < .05) higher cancer cell death compared to parental FaDu cells (). Of note, the apparent differences in susceptibility of CD47-KO cancer cells to treatment with T cells redirected by BIS1 versus Retarg-1, as shown in vs. g, could be attributable to differences in the respective immunologic effector conditions involved. In particular, BIS1 activates and redirects essentially all CD3pos T cells in an HLA-independent manner irrespective of their TCR specificity, whereas Retarg-1 exclusively redirects cognate HLA-matched and ready-to-go CMVpp65-specific T cells toward EpCAMpos target cancer cells. Despite these differences, the data demonstrate that respective immune attacks mediated by both types of T cell-engaging agents are modulated by the cell surface presence of CD47 on the targeted cancer cell types.
Additionally, results from corroborated the significant increase in caspase 3-mediated cancer cell death (Live-Cell Analysis) in FaDu.CD47KO cells compared to FaDu parental cells (). Similar results were obtained for CD47KO cell lines SK-RC-52.CD47KO and NCI-H292.CD47KO when compared to the respective parental cell line. Of note, in this assay, both OvCAR-3 and A431 cancer cells showed no significant difference in susceptibility to T cell cytotoxicity compared to their corresponding CD47-KO counterparts ().
CD47 knockout enhances susceptibility of cancer cells to TRAIL- and FAS-mediated cell death
Next, we investigated whether the enhanced susceptibility to T cell cytotoxicity of CD47.KO cell lines is attributable to a change in intrinsic sensitivity toward the pro-apoptotic activity of T cell effector molecules TRAIL and FASL. Indeed, CD47.KO cell lines were proved to be more sensitive to TRAIL- (4 out of 5, ) and FAS-mediated (3 out of 5, ) cell death. Reversely, elimination of Retarg-1-decorated FaDu.CD47KO cells by CMVpp65-specific T cells was significantly reduced when treatment was performed in the presence of TRAIL- or FASL-neutralizing antibodies (p < .05 and p < .01, respectively, ).
Figure 4. CD47 knockout enhances susceptibility of cancer cells to TRAIL- and FAS-mediated cell death
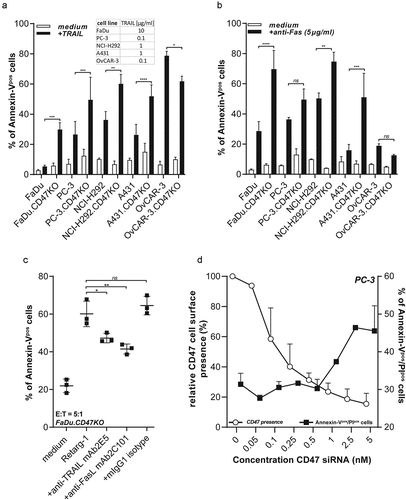
Progressive knockdown of endogenous CD47 by siRNA suggests ‘threshold’ for TRAIL susceptibility
PC-3 cells in which CD47 expression was dose-dependently knocked down by CD47-specific siRNA were subjected to TRAIL-mediated cell death. The results indicated that PC-3 cells required a ~ 75% knockdown of the endogenous CD47 expression levels in order to overcome the intrinsic resistance of these cancer cells to TRAIL-mediated apoptosis ().
Treatment with bsAb CD47xEGFR-IgG2s induces EGFR-assisted internalization and subsequent reduction of cancer cell surface-expressed CD47
We previously reported that the capacity of bsAb CD47xEGFR-IgG2s to internalize CD47 dose-dependently relied on the cell surface expression levels of EGFR of the respective cancer cell lines.Citation8 In particular, bsAb CD47xEGFR-IgG2s showed only low capacity for CD47 internalization when treating EGFRlow cell lines like OvCAR-3 and PC-3, but prominent capacity to do so when treating EGFRhigh cell lines like NCI-H292, FaDu, A431 and SK-RC-52 cells as is shown in Suppl. Figure S4A-C. The capacity of bsAb CD47xEGFR-IgG2s to induce prolonged displacement of cancer cell surface-expressed EGFR and CD47 was further investigated using A431.CD47KO and SK-RC-52.CD47KO cancer cells that were transfected with cDNA encoding CD47 C-terminally fused to GFP (CD47-GFP). Internalization and subsequent reduction of CD47-GFP, evident from decrease of its fluorescent activity, were evaluated by flow cytometry. Treatment of CD47-GFP-expressing A431.CD47KO and SK-RC-52.CD47KO cells with bsAb CD47xEGFR-IgG2s resulted in an up to 50% reduction in the amount of CD47-GFPpos cells. CD47-blocking antibodies B6H12, CC-90002 and CD47-blocking fusion protein SIRPα-Fc failed to co-internalize CD47 and EGFR (). Of note, ‘piggybacking’ of the Hum-ZAP toxin on bsAb CD47xEGFR-IgG2s confirms enhanced internalization antigen/bsAb-complexes from the cell surface compared to CD47-blocking agents (Suppl. Figure S4d).
Figure 5. BsAb CD47xEGFR-IgG2s-mediated internalization of CD47 enhances susceptibility of cancer cells to TRAIL-mediated cell death
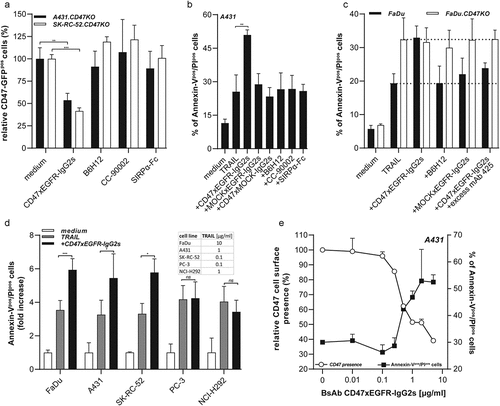
Consequently, we selected EGFRhigh cell lines NCI-H292, FaDu, A431 and SK-RC-52 as particularly suitable indicator cell lines to investigate the capacity of bsAb CD47xEGFR-IgG2s to enhance susceptibility to TRAIL-mediated apoptosis () and to anti-CMVpp65 T cell-mediated cytotoxicity ().
Figure 6. BsAb CD47xEGFR-IgG2s-mediated internalization of CD47 enhances susceptibility of cancer cells to T cell-induced cytotoxicity
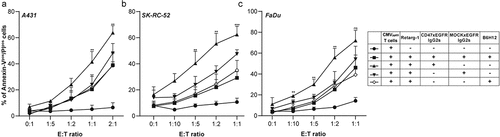
Conventional CD47-blocking agents fail to enhance susceptibility of cancer cells to TRAIL-mediated cell death
Various clinically relevant/frequently used CD47-blocking agents were evaluated for capacity to enhance susceptibility of cancer cells to TRAIL-mediated apoptosis. To this end, A431 cells were treated with mAb B6H12, CC-90002 or fusion protein SIRPα-Fc. Only treatment with bsAb CD47xEGFR-IgG2s resulted in an enhanced susceptibility of cancer cells to TRAIL-mediated cell death (). Importantly, treatment of FaDu cells with bsAb CD47xEGFR-IgG2s enhanced susceptibility to TRAIL-mediated cell death to a level similar to that observed in FaDu.CD47KO cells (). Of note, treatment of FaDu cells with bsAb CD47xEGFR-IgG2s in the presence of excess amounts of EGFR-blocking antibody mAb 425 prevented enhanced susceptibility to TRAIL-mediated cell death.
Treatment with bsAb CD47xEGFR-IgG2s enhances susceptibility of cancer cells to TRAIL-mediated cell death
Treatment of FaDu, A431 or SK-RC-52 cells, but not PC-3 or NCI-H292 cells, with bsAb CD47xEGFR-IgG2s and TRAIL resulted in a significant increase in cell death compared to treatment with TRAIL alone (p < .001, p < .05 and p < .05, respectively, ). Treatment with bsAb CD47xEGFR-IgG2s dose-dependently displaced CD47 (and EGFR) from the cancer cell surface, which coincided with enhancement of susceptibility to TRAIL-mediated cell death. In particular, pretreatment of A431 cells with bsAb CD47xEGFR-IgG2s (5 μg/ml) for 24 h followed by the treatment with TRAIL (1 µg/ml) induced an up to >40% displacement of CD47 from cancer cell surface, which coincided with a ~twofold increase in cancer cell death ().
BsAb CD47xEGFR-IgG2s-mediated internalization of CD47 enhances susceptibility of cancer cells to T cell-induced cytotoxicity
We investigated whether treatment with bsAb CD47xEGFR-IgG2s enhances susceptibility of cancer cells to T cell-induced cytotoxicity. Indeed, treatment of Retarg-1-decorated A431 cells with bsAb CD47xEGFR-IgG2s resulted in a twofold enhanced susceptibility to pro-apoptotic elimination by CMVpp65-specific T cells (), whereas treatment with CD47-blocking mAb B6H12 or control bsAb MOCKxEGFR-IgG2s failed to do so. Similar results were obtained using FaDu and SK-RC-52 cancer cells ( and ). Moreover, treatment with antibody CC-90002 or fusion protein SIRPα-Fc failed to enhance susceptibility of cancer cells to T cell-induced cytotoxicity (data not shown).
Discussion
Currently, the dominant paradigm advocates that cancer cells exploit overexpression of CD47 to inhibit phagocytic elimination and prevent neoantigen processing via the CD47-SIRPα axis, thereby only indirectly evading T cell-mediated adaptive immunity. Previously, selected reports have suggested that cancer cells may also exploit CD47 expression to directly inhibit elimination by cytotoxic T cells.Citation6,Citation7 However, thus far these suggestions have remained unsupported by compelling evidence.
To address this issue, we applied an in vitro model system in which EpCAMpos cancer cell types were selectively decorated with Retarg-1, a soluble EpCAM-directed HLA-B*07:02 fragment genetically equipped with pp65 peptide TPRVTGGGAM. Retarg-1 redirects the potent cytotoxic activity of CMVpp65-specific CD8pos T cells to EpCAMpos cancer cells, which resulted in their rapid elimination. However, we noted that a small viable subpopulation of Retarg-1-decorated cancer cells had managed to evade elimination by CMVpp65-specific T cells. As expected, the surviving cancer cells showed a prominent (>10-fold) increase in PD-L1 expression, a feature known to incapacitate cytotoxic T cells. Intriguingly, this upregulation of PD-L1 coincided with a fourfold increase in CD47 expression by the same cancer cells. Similarly, treatment of EpCAMpos cancer cells with CD3pos T cells redirected with an EpCAM-directed CD3-agonistic bsAb (BIS1) resulted in a ~twofold increase in CD47 expression by cancer cells that had escaped from elimination. Of note, the difference in dynamic range of IFNγ-mediated upregulation between CD47 and PD-L1 expression is related to the fact that in the absence of activated immune cells most cancer cell types already overexpress CD47, but show minimal or no expression of PD-L1.
We identified T cell-secreted IFNγ as main inducer for CD47 upregulation, both for solid cancer cell lines and primary patient-derived cancer cells. This result is in line with IFNγ-mediated upregulation of CD47 mRNA reported for uveal melanoma cells.Citation9 Moreover, our analysis of genomic data from cBioportal database for Cancer Genomics revealed that mRNA expression of CD47 in various cancer types positively correlates with that of IFNGR1/2 and various genes involved in the IFN signaling pathway. Recently, it was reported that MCF-7 cancer cells upregulate CD47 expression in response to TNFα treatment.Citation10 However, our data indicate that for most solid cancer cell lines IFNγ is the main inducer for CD47 upregulation.
Importantly, we uncovered that both IFNγ-mediated and lentivirus-mediated enhancement of CD47 cell surface expression significantly increased the intrinsic resistance of PC-3 cancer cells to cytotoxic T cells. Conversely, CD47-knockout cancer cells showed increased susceptibility to cytotoxic T cells. In the latter experiments, cytotoxic T cells expressed similar levels of activation markers and excreted similar levels of IFNγ and granzyme B.
Intriguingly, siRNA-mediated knockdown of CD47 to ~25% of the endogenous expression level appeared to act as a ‘threshold’ below which PC-3 cancer cells became significantly more susceptible to TRAIL. This CD47 expression level-associated threshold appears to be cancer cell type dependent. This in turn suggested to us that targeted down-modulation of cancer cell surface-expressed CD47 may be therapeutically exploitable to enhance the susceptibility of cancer cells to the pro-apoptotic effector mechanisms of anticancer T cells.
Consequently, we evaluated whether a bsAb with dual targeting capacity for CD47 and EGFR could be used for this purpose. In this respect, we previously reported on bsAb CD47xEGFR-IgG1 which has potent capacity to selectively direct CD47-blockade toward EGFR-overexpressing cancer cells.Citation8 BsAb CD47xEGFR-IgG1 has a unique mode-of-action in that treatment of CD47pos/EGFRpos cancer cells resulted in rapid co-internalization of EGFR and CD47, followed by prolonged displacement of both EGFR and CD47 from the cell surface. For the current study, we constructed variant bsAb CD47xEGFR-IgG2s which was equipped with an immune effector function nullified Fc domain.Citation8,Citation11 Indeed, treatment of CD47pos/EGFRpos cancer cells with bsAb CD47xEGFR-IgG2s resulted in prolonged cell surface displacement of both EGFR and CD47 and a marked sensitization of these cancer cells to TRAIL- and T cell-induced cytotoxicity.
Previously, it was demonstrated that EGFR internalization represents a crucial step for its signal termination.Citation12 Therefore, the capacity of bsAb CD47xEGFR-IgG2s to co-internalize CD47 and EGFR may potentiate its therapeutic efficacy as this inhibits EGFR-associated oncogenic features and concurrently enhances the susceptibility of the targeted cancer cells to pro-apoptotic activity of CTLs.
The mechanism by which cancer cell-expressed CD47 regulates sensitivity to the pro-apoptotic activity of cognate T cells remains to be elucidated. In this respect, it is noteworthy that the intrinsic apoptosis pathway is primarily controlled by the BCL-2 family of proteins. This can be divided into pro-survival/anti-apoptotic (BCL-2, BCL-xL, MCL-1, BCL-W, BFL1), effector (BAK, BAX, BOK), BH3-only activator (BIM, BID, PUMA) and sensitizer (NOXA, BAD, BMF, BIK, Hrk) proteins.Citation13 The decision between cancer cell survival and death appears to depend on the precise balance of these anti- and pro-apoptotic proteins. Previously, it was reported that certain anti-CD47 antibodies, such as CD47-blocking mAbs CC2C6 and MABL, induce cell death in selected cancer types by ligation of CD47 in a caspase-independent manner.Citation14–16 In this process, mAb CC2C6 was found to stimulate increases in MCL-1 and NOXA levels. When cancer cells were co-treated with mAb CC2C6 and a caspase-activating agent, the MCL-1:NOXA ratio was further changed in favor of NOXA, resulting in enhanced apoptotic cancer cell death. Of note, we applied the CD47-blocking scFv antibody fragment derived from mAb MABL and the EGFR-inhibiting scFv from matuzumab to construct bsAb CD47xEGFR-IgG2s.
Moreover, it has been reported that combining MCL-1 and EGFR inhibitors synergistically reduced cancer cell viability, induced apoptosis and prevented the development of drug-tolerance. This combination also reduced subcutaneous tumors in xenograft models with EGFR-mutated cell lines.Citation17 Although not formally investigated here, it is tentative to speculate that the dual-blocking capacity of bsAb CD47xEGFR-IgG2s enhances the susceptibility of cancer cells to pro-apoptotic immune effector molecules by selectively tipping the MCL-1:NOXA ratio in favor of pro-apoptotic NOXA.
In conclusion, we demonstrate for the first time that cancer cells under immune attack dynamically upregulate CD47 cell surface expression, which is associated with a directly enhanced resistance to pro-apoptotic effector molecules TRAIL and FASL of cytotoxic T cells, fully independent of modulation of the CD47-SIRPα axis. Therefore, bsAb CD47xEGFR-IgG2s may be of therapeutic value to selectively enhance the intrinsic susceptibility of CD47pos/EGFRpos cancer cells to the pro-apoptotic activity of anticancer T cells. This approach may open up alternate avenues for CD47-blocking approaches in cancer immunotherapy with potentially enhanced efficacy and reduced off-target side effects.
Materials and methods
Antibodies and reagents
The following primary fluorescently labeled murine mAbs directed against human antigens were used: FITC/APC-labeled CD47-blocking antibody (clone B6H12, eBioscience), PE-labeled anti-SIRPα antibody (clone 15–414, ThermoFisher), FITC-labeled non-blocking anti-CD47 antibody (clone 2D3, eBioscience), FITC/APC-labeled anti-EGFR antibody (clone 528, Santa Cruz Biotechnology), PerCP-Cy5.5-labeled anti-CD3 antibody (clone OKT-3, eBioscience), APC-labeled anti-CD69 antibody (clone FN50, Immunotools), APC-labeled anti-CD8 antibody (clone RPA-T8, eBioscience), APC-labeled anti-PD-L1 antibody (clone 29E.2A3, BioLegend) and FITC/APC-labeled IgG1 isotype control antibody (clone 1 F8, Immunotools).
The following unconjugated antibodies directed against human antigens were used: anti-SIRPγ antibody (clone LSB2.20, Santa Cruz Biotechnology), anti-CD47 antibody (B6H12, ThermoFisher), antagonistic anti-TRAIL antibody (clone 2E5, Abcam), antagonistic anti-FASL antibody (clone 2 C101, Enzo Life Sciences), agonistic anti-FAS antibody (clone 7C11, Immunotech), antagonistic anti-IFNγ antibody (clone B27, Immunotools), antagonistic anti-TNFα antibody (clone D2E7, Enzo Life Sciences), EGFR-blocking antibody (clone 425, Merck). SIRPα-Fc (TTI-621, Trillium Therapeutics) and anti-human CD47-IgG4 antibody (CC-90002, Inhibrix) were generated by commercial gene synthesis service (GenScript). Fluorescently labeled secondary antibody goat anti-human Ig PE and goat anti-mouse IgG APC were both from Southern Biotech.
The following reagents were used: recombinant human IFNα, IFNβ, IFNω, IFNγ, TNFα, IL-2 and FITC/APC-labeled Annexin-V (all Immunotools) and propidium iodide (PI) (Invitrogen). A recombinant human hexa-ligand form of TRAIL (TRAIL:Fc-fusion, AB Biosciences) was used in TRAIL-mediated cytotoxicity experiments. BIS1 and Retarg-1 were produced in-house.Citation18 Secretion of IFNγ and granzyme B was measured using appropriate ELISA kits (IFNγ from eBioscience and granzyme B from Mabtech).
Cell lines and primary patient-derived solid cancer cells
Cell lines HEK293T, PC-3, OvCAR-3, NCI-H292, FaDu, A431, MDA-MB-231, A172, MCF-7, A375m, SEM, K562, Jurkat and U937 cells were obtained from the American Type Culture Collection (ATCC). Cell line SK-RC-52 was a kind gift from Dr. Otto Boerman (department of nuclear medicine, Radboud UMC, Nijmegen). Cell line GIST882 was a kind gift from Dr. Steven de Jong (Department of Medical Oncology, UMCG, Groningen). Cell line 92–2 was a kind gift from Dr. Martine Jager (Department of Ophthalmology, LUMC, Leiden). Cancer cells were cultured in RPMI-1640 or DMEM (Lonza) as indicated, supplemented with 10% fetal calf serum (FCS, ThermoFisher) at 37°C in a humidified 5% CO2 atmosphere. Peripheral blood mononuclear cells (PBMCs) were cultured in X–VIVO15 medium (Lonza). Primary patient-derived tumor cells were collected during surgical resection. This study was carried out in The Netherlands in accordance with International Ethical and Professional Guidelines (the Declaration of Helsinki and the International Conference on Harmonization Guidelines for Good Clinical Practice). The use of anonymous rest material is regulated under the code for good clinical practice in the Netherlands. Informed consent was waived in accordance with Dutch regulations.
PC-3, OvCAR-3, NCI-H292, FaDu, SK-RC-52 and A431 cancer cell lines knockout for CD47 (Suppl. Figures S3C and S3D) were generated by transfection with pSpCas9 BB-2A-GFP (PX458) plasmid (Origene) encoding sgRNA 5ʹ-ATCGAGCTAAAATATCGTGT-3ʹ and subsequent single-cell or bulk sorting at the UMCG Flow Cytometry facility.Citation19
PBMC isolation
In short, PBMCs were isolated from healthy donors obtained by standard density gradient centrifugation (Lymphoprep, Stemcell technologies), according to manufacturer’s recommendations. In short, two parts of whole blood (1:1 diluted with PBS) was put on top of one part Lymphoprep and centrifuged at 1000xg for 20 min at RT. PBMCs were harvested, washed twice with PBS and resuspended in RPMI supplemented with 10% FCS.
Immunoblot analysis
Immunoblot analysis was used to confirm CRISPR/Cas9-mediated knockout (KO) of CD47 expression (Suppl. Figure S3e). To this end, lysates of PC-3, PC-3.CD47KO, FaDu and FaDu.CD47KO cells (each containing 20 µg protein) were dissolved in Laemmli sample buffer containing 2.5% β-mercaptoethanol, incubated at room temperature for 30 min and then separated by SDS-PAGE (10% AA). Of note, per instruction of the manufacturer of the sheep anti-human CD47 polyclonal antibody, heating of the samples by boiling was omitted to prevent the reported formation of high-molecular weight CD47 aggregates that cannot enter the running gel. Subsequently, proteins were electroblotted from the separation gel onto a nitrocellulose (NC) membrane. Before immunologic probing, the membrane was blocked using TBS (Tris-buffered saline) supplemented with 5% BSA. Subsequently, the NC membrane was probed with 1 µg/mL of sheep anti-human CD47 (antigen affinity-purified) polyclonal antibody (R&D systems, cat# AF4670) followed by HRP-conjugated anti-sheep IgG secondary antibody (R&D systems, cat# HAF016) and detection by standard chemiluminescence technology.
Lentiviral overexpression of CD47 in cancer cells
Lentiviral particles for overexpression of CD47 in cells were produced by transient transfection of HEK293T cells with eGFP.hCD47 plasmid (a kind gift from Dr. Luigi Naldini, Hospitale San Raffaele, Italy) using lentiviral transfection kit (Origene) according to manufacturer's protocol. In short, PC-3 cancer cells (2 × 105) were plated in a 6-well plate in complete media with 8 μg/ml polybrene (Sigma-Aldrich) and transduced with 0.5 ml lentiviral particles containing eGFP.CD47 or empty vector (ev) eGFP. Next, transduced PC-3 cells were bulk-sorted for eGFP expression at the UMCG Flow Cytometry facility. Lentiviral overexpression of CD47 in PC-3 cells (PC-3.CD47high) was verified by flow cytometry using APC-labeled CD47 antibody B6H12 (see also Suppl. Figure S3b).
Construction, production and evaluation of bsAb CD47xEGFR-IgG2s
A detailed evaluation of bsAb CD47xEGFR-IgG, including its construction and production, was recently published by our group.Citation8 In short, DNA fragments encoding scFv MABL and scFv 425 were generated by commercial gene synthesis service (GenScript) based on published VH and VL sequence data.Citation20,Citation21Citation22 For construction and production of bsAb CD47xEGFR-IgG2s, we used eukaryotic expression plasmid pEE14-bsAb, which contains three multiple cloning sites (MCS) for directional and in-frame insertion of DNA fragments: scFv MABL, scFv 425 and IgG2s-Fc.Citation11 BsAb CD47xEGFR-IgG2s was produced using the Expi293 expression system (ThermoFisher). Expi293 supernatant, containing bsAb CD47xEGFR-IgG2s, was purified using a HiTrap protein A HP column connected to an ÄKTA Start chromatography system (GE Healthcare Life Sciences).
CMV-based model system for antigen-specific T cell responses
The CMVpp65-based model system has been described in detail elsewhere.Citation8 In short, PBMCs were freshly isolated from an HLA-B*07:02 CMV-positive healthy donor and stimulated with 5–10 μl of recombinant CMVpp65 protein stock solution (Miltenyi Biotec) per ml of cell suspension for 2–3 d. Next, PBMCs were repeatedly treated with 500–1000 U/ml IL-2 and/or cultured on a feeder layer of OvCAR-3.pp65 cells (HLA-B*07:02pos). Flow cytometry indicated that this stimulation protocol yielded >60% CMVpp65-specific T cells (data not shown). Construction, production and evaluation of Retarg-1 will be published elsewhere (I. Britsch et al., manuscript in preparation).
Assessment of CD47 upregulation by cancer cells under T cell immune attack
Upregulation of CD47 in cancer cells was assessed by flow cytometry using anti-CD47 antibody B6H12. The obtained mean fluorescent intensity (MFI) data were corrected for background fluorescence and presented as fold increase compared to untreated cancer cells. In short, ex vivo expanded CMVpp65-specific T cells or PBMCs were co-cultured with cancer cells at indicated E:T cell ratios in the presence of Retarg-1 (0.3 μg/ml) or BIS1 (0.15 μg/ml), respectively, for 48 h. Next, cancer cells were evaluated for CD47 or PD-L1 expression by flow cytometry. Annexin-Vpos cancer cells were excluded from the analysis.
For flow cytometric analysis of coculture experiments, effector cells (PBMCs or anti-CMVpp65 T cells) were discerned from target cancer cells using their respective differential forward scatter (FSC) and side scatter (SCC) profiles. This gating strategy was confirmed by staining cocultures with fluorescently labeled antibodies directed against CD3 (identifying T cells) and EpCAM (identifying carcinoma cells) (data not shown).
Upregulation of CD47 expression enhances resistance of PC-3 cells to T cell-mediated cytotoxicity
Cancer cells (PC-3, PC-3.CD47KO, PC-3.ev or PC-3.CD47high) were plated in a 48-well plate and pretreated (or not) with IFNγ and TNFα (100 ng/ml each) for 48 h. Next, cells were washed and CMVpp65-specific T cells were added at indicated E:T cell ratios in the presence or absence of Retarg-1 (0.3 μg/ml) for 24 h, after which cell death in PC-3 cells was evaluated. Supernatants of co-cultured CMVpp65-specific T cells and cancer cells were harvested after 24 h and frozen at −20°C prior to IFNγ and granzyme B ELISA.
CD47 knockout enhances susceptibility of cancer cells to T cell-mediated cytotoxicity
Cancer cell susceptibility to T cell-mediated cytotoxicity was assessed by flow cytometry using Annexin-V/PI staining. In short, CMVpp65-specific T cells or PBMCs were co-cultured with cancer cells at indicated E:T cell ratios in the presence or absence of Retarg-1 (0.3 μg/ml) or BIS1 (0.15 μg/ml), respectively, for 48 h. Next, cells were harvested, and killing of cancer cells was assessed. In addition, BIS1-activated PBMCs that were co-cultured with FaDu or FaDu.CD47KO cells in an E:T cell ratio of 2:1 were stained with anti-CD3 antibody and anti-CD69 antibody to identify activated T cells. Supernatant derived from a 48 h co-culture of PBMCs with FaDu cells was analyzed for IFNγ and granzyme B content by ELISA.
Additionally, cancer cell susceptibility to T cell-mediated cytotoxicity was assessed using the xCELLigence RTCA instrument (ACEA Biosciences) and Live-Cell Imaging (Incucyte). In short, FaDu or FaDu.CD47KO cells (8 × 103/well) were seeded into an E-plate 16 (ACEA Biosciences) and treated (or not) with BIS1 (0.15 μg/ml). After 8 h, PBMCs were added to an E:T cell ratio of 2:1. Next, cancer cell viability (indicated as cell index) was monitored for 48 h using the xCELLigence RTCA instrument. Caspase-3 activation in FaDu or FaDu.CD47KO cells was assessed using the Incucyte live-cell imaging system. In this assay, FaDu or FaDu.CD47KO cells (3 × 103/well) were plated in a 96-well plate and incubated with 1 μM fluorogenic caspase-3 substrate (NucView 488 caspase-3 assay kit, Biotium). Next, CMVpp65-specific T cells and Retarg-1 (0.3 μg/ml) were added to the plate in an E:T cell ratio of 1:2, after which caspase-3 activation in target cells was monitored for 72 h.
Assessment of cancer cell susceptibility to TRAIL or FAS-mediated cell death
Cancer cell susceptibility to TRAIL or FAS-mediated cytotoxicity was assessed by flow cytometry using Annexin-V. In short, cancer cells (1–2 × 104/well) were seeded in a 48-well plate overnight and then treated with indicated concentrations of TRAIL or anti-FAS antibody (5 μg/ml) for 24 h. Next, cancer cells were harvested and the percentage of TRAIL or FAS-induced cancer cell death was assessed. In addition, FaDu.CD47KO cells were co-cultured with CMVpp65-specific T cells in an E:T cell ratio of 5:1 in the presence of Retarg-1 and antagonistic anti-TRAIL antibody mAb 2E5 or antagonistic anti-FASL antibody mAb 2 C101 or mouse IgG1 control antibody. After 16 h, cancer cell death was evaluated by flow cytometry.
Assessment of PC-3 cell susceptibility to TRAIL-mediated cell death following siRNA-mediated knockdown of CD47
Cancer cell susceptibility to TRAIL-mediated cytotoxicity was assessed by flow cytometry using Annexin-V/PI staining. In short, PC-3 cells were transfected with indicated concentrations of CD47-specific siRNA (cat. 4392421, Invitrogen) or control-siRNA (cat. 4390843, Invitrogen) using ExpiFectamine293 transfection kit (ThermoFisher) (Suppl. Figure S3d). After 72 h, transfected-PC-3 cancer cells were seeded in a 48-well plate and treated with 0.1 μg/ml TRAIL for 4 h, after which cancer cell death was evaluated. Of note, CD47 cell surface levels on cancer cells were evaluated ~72 h after transfection with CD47-specific siRNA. Residual CD47 cell-surface presence was assessed using the non-competing anti-CD47 mAb 2D3. CD47 surface levels in mock-transfected or untreated cells were normalized to 100%.
Assessment of internalization of antibody/antigen complexes by bsAb CD47xEGFR-IgG2s
Antibody-induced internalization of CD47 was assessed using CD47-KO cancer cells transfected with cDNA encoding CD47 tagged with GFP. Upon internalization of CD47-GFP the percentage of leftover GFPpos cancer cells after 48 h treatment with bsAb CD47xEGFR-IgG2s or control antibodies (5 μg/ml) was evaluated by flow cytometry and indicated as percentage of medium.
Assessment of cancer cell susceptibility to TRAIL-mediated cell death following treatment with bsAb CD47xEGFR-IgG2s
Cancer cell susceptibility to TRAIL-mediated cytotoxicity was assessed by flow cytometry using Annexin-V/PI staining. In short, various cancer cell lines were plated in a 48-well plate (1–2 × 104/well) and treated with indicated concentrations of bsAb CD47xEGFR-IgG2s or control antibodies for 24 h to promote internalization of cell-surface CD47. Subsequently, these antibody-treated cancer cells were subjected to treatment with indicated concentrations of TRAIL for 24 h. Cancer cell death was evaluated and indicated as percentage Annexin-Vpos/PIpos events or as fold increase in cell death compared to untreated cells.
Assessment of A431 cancer cell susceptibility to TRAIL-mediated cell death following bsAb CD47xEGFR-IgG2s-mediated internalization of CD47
A431 cancer cell susceptibility to TRAIL-mediated cytotoxicity was assessed by flow cytometry using Annexin-V/PI staining. In short, A431 cells were treated with indicated concentrations bsAb CD47xEGFR-IgG2s for 24 h. Next, A431 cells were additionally treated with 1 μg/ml TRAIL for 24 h, after which cancer cell death was evaluated. Of note, CD47 cell surface levels on cancer cells were evaluated 24 h after incubation with bsAb CD47xEGFR-IgG2s.
Assessment of cancer cell susceptibility to T cell-induced cytotoxicity following treatment bsAb CD47xEGFR-IgG2s
Cancer cell susceptibility to T cell-induced cytotoxicity was assessed by flow cytometry using Annexin-V/PI staining. In short, cancer cells (A431, FaDu or SK-RC-52) were treated with bsAb CD47xEGFR-IgG2s or control antibodies (2 μg/ml) for 24 h. Next, cells were washed and thereafter co-cultured with CMVpp65-specific T cells at the indicated E:T cell ratios in the presence of Retarg-1 (0.3 μg/ml) for 24 h. Cancer cell death was assessed and indicated as percentage Annexin-Vpos/PIpos events.
Statistics
Statistical analysis was performed using GraphPad Prism 8 (GraphPad Software). Graphs show mean ± standard deviation (SD) from at least three independent experiments, unless stated otherwise. Means were assessed for differences using an unpaired two-tailed Student’s t-test or one-way ANOVA followed by multiple comparisons test, where appropriate. P-values < 0.05 were considered to be significant and are indicated by asterisks as follows: *p < .05; **p < .01; ***p < .001; ****p < .0001.
Supplemental Material
Download ()Disclosure statement
No potential conflict of interest was reported by the author(s).
Supplementary material
Supplemental data for this article can be accessed on the publisher’s website.
Additional information
Funding
References
- Jaiswal S, Jamieson CHM, Pang WW, Park CY, Chao MP, Majeti R, Traver D, van Rooijen N, Weissman IL. Cd47 is upregulated on circulating hematopoietic stem cells and leukemia cells to avoid phagocytosis. Cell. 2009;138(2):271–14. doi:10.1016/j.cell.2009.05.046.
- Chao MP, Alizadeh AA, Tang C, Jan M, Weissman-Tsukamoto R, Zhao F, Park CY, Weissman IL, Majeti R. Therapeutic antibody targeting of cd47 eliminates human acute lymphoblastic leukemia. Cancer Res. 2011;71(4):1374–1384. doi:10.1158/0008-5472.CAN-10-2238.
- Willingham SB, Volkmer JP, Gentles AJ, Sahoo D, Dalerba P, Mitra SS, Wang J, Contreras-Trujillo H, Martin R, Cohen JD. The CD47-signal regulatory protein alpha (Sirpa) interaction is a therapeutic target for human solid tumors. Proc Natl Acad Sci U.S.A. 2012;109(17):6662–6667. doi:10.1073/pnas.1121623109.
- Tseng D, Volkmer JP, Willingham SB, Contreras-Trujillo , H, Fathman, JW, Fernhoff, NB, Seita, J, Inlay, MA, Weiskopf, K, Miyanishi, M, and Weissman, IL, et al. Anti-CD47 antibody–mediated phagocytosis of cancer by macrophages primes an effective antitumor T-cell response. PNAS. 2013;110(27):11103–11108. doi:10.1073/pnas.1305569110.
- Liu X, Pu Y, Cron K, Deng, L, Kline, J, Frazier, WA, Xu, H, Peng, H, Fu, Y, and Xu, MM, et al. CD47 blockade triggers T cell–mediated destruction of immunogenic tumors. Nat Med. 2015;21(10):1209–1215. doi:10.1038/nm.3931.
- Soto-Pantoja DR, Terabe M, Ghosh A, Ridnour LA, DeGraff WG, Wink DA, Berzofsky JA, Roberts DD. Cd47 in the tumor microenvironment limits cooperation between antitumor t-cell immunity and radiotherapy. Cancer Res. 2014;74(23):6771–6783. doi:10.1158/0008-5472.CAN-14-0037-T.
- Logtenberg MEW, Scheeren FA, Schumacher TN. The cd47-sirpα immune checkpoint. Immunity. 2020;52(5):742–752. doi:10.1016/j.immuni.2020.04.011.
- Hendriks MAJM, Ploeg EM, Koopmans I, Britsch I, Ke X, Samplonius DF, Helfrich W. Bispecific antibody approach for EGFR-directed blockade of the CD47-SIRPα “don’t eat me” immune checkpoint promotes neutrophil-mediated trogoptosis and enhances antigen cross-presentation. OncoImmunology. 2020;9(1):1824323. doi:10.1080/2162402X.2020.1824323.
- Basile MS, Mazzon E, Russo A, Mammana, S, Longo, A, Bonfiglio, V, Fallico, M, Caltabiano, R, Fagone , P, Nicoletti, F, Avitabile, T, and Reibaldi, M, et al. Differential modulation and prognostic values of immune-escape genes in uveal melanoma. PLoS One. 2019;14:1.
- Betancur PA, Abraham BJ, Yiu YY, Willingham SB, Khameneh F, Zarnegar M, Kuo AH, McKenna K, Kojima Y, Leeper NJ, et al. A CD47-associated super-enhancer links pro-inflammatory signalling to CD47 upregulation in breast cancer. Nat Commun. 2017;8(1):14802. doi:10.1038/ncomms14802.
- Vafa O, Gilliland GL, Brezski RJ, Strake B, Wilkinson T, Lacy ER, Scallon B, Teplyakov A, Malia TJ, Strohl WR, et al. An engineered Fc variant of an IgG eliminates all immune effector functions via structural perturbations. Methods. 2014;65(1):114–126. doi:10.1016/j.ymeth.2013.06.035.
- Al-Akhrass H, Naves T, Vincent F, Magnaudeix A, Durand K, Bertin F, Melloni B, Jauberteau M-O, Lalloué F. Sortilin limits EGFR signaling by promoting its internalization in lung cancer. Nat Commun. 2017;8(1):1182. doi:10.1038/s41467-017-01172-5.
- Singh R, Letai A, Sarosiek K. Regulation of apoptosis in health and disease: the balancing act of BCL-2 family proteins. Nat Rev Mol Cell Biol. 2019;20(3):175–193. doi:10.1038/s41580-018-0089-8.
- Leclair P, Liu -C-C, Monajemi M, Reid GS, Sly LM, Lim CJ. CD47-ligation induced cell death in T-acute lymphoblastic leukemia. Cell Death Dis. 2018;9(5):1–14. doi:10.1038/s41419-018-0601-2.
- Uno S, Kinoshita Y, Azuma Y, Tsunenari T, Yoshimura Y, Iida S, Kikuchi Y, Yamada-Okabe H, Fukushima N. Antitumor activity of a monoclonal antibody against CD47 in xenograft models of human leukemia. Oncol Rep. 2007;17:1189–1194.
- Sagawa M, Shimizu T, Fukushima N, Kinoshita Y, Ohizumi I, Uno S, Kikuchi Y, Ikeda Y, Yamada-Okabe H, Kizaki M, et al. A new disulfide-linked dimer of a single-chain antibody fragment against human CD47 induces apoptosis in lymphoid malignant cells via the hypoxia inducible factor-1α pathway. Cancer Sci. 2011;102(6):1208–1215. doi:10.1111/j.1349-7006.2011.01925.x.
- Song KA, Hosono Y, Turner C, Jacob S, Lochmann TL, Murakami Y, Patel NU, Ham J, Hu B, Powell KM, et al. Increased synthesis of MCL-1 protein underlies initial survival of EGFR -mutant lung cancer to EGFR inhibitors and provides a novel drug target. Clin Cancer Res. 2018;24(22):5658–5672. doi:10.1158/1078-0432.CCR-18-0304.
- Kroesen BJ, Ter Haar A, Spakman H, Willemse P, Sleijfer DT, De Vries EGE, Mulder NH, Berendsen HH, Limburg PC, The TH, et al. Local antitumour treatment in carcinoma patients with bispecific-monoclonal-antibody-redirected T cells. Cancer Immunol Immunother. 1993;37(6):400–407. doi:10.1007/BF01526797.
- Sanjana NE, Shalem O, Zhang F. Improved vectors and genome-wide libraries for CRISPR screening. Nat Methods. 2014;11(8):783–784. doi:10.1038/nmeth.3047.
- Kikuchi Y, Uno S, Yoshimura Y, Otabe K, Iida S-I, Oheda M, Fukushima N, Tsuchiya M. A bivalent single-chain Fv fragment against CD47 induces apoptosis for leukemic cells. Biochem Biophys Res Commun. 2004;315(4):912–918. doi:10.1016/j.bbrc.2004.01.128.
- Wiersma VR, He Y, Samplonius DF, Van Ginkel RJ, Gerssen J, Eggleton P, Zhou J, Bremer E, Helfrich W. A CD47-blocking TRAIL fusion protein with dual pro-phagocytic and pro-apoptotic anticancer activity. Br J Haematol. 2014;164(2):304–307. doi:10.1111/bjh.12617.
- Cerami E, Gao J, Dogrusoz U, Gross BE, Sumer SO, Aksoy BA, Jacobsen A, Byrne CJ, Heuer ML, Larsson E, et al. The cbio cancer genomics portal: an open platform for exploring multidimensional cancer genomics data. Cancer Discov. 2012;2(5):401–404. doi:10.1158/2159-8290.CD-12-0095.