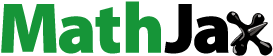
ABSTRACT
Gut microbiota impacts responses to immune checkpoint inhibitors (ICI). A high level of Faecalibacterium prausnitzii have been associated with a positive response to ICI in multiple cancer types. Here, based on fecal shotgun metagenomics data, we show in two independent cohorts of patients with non-small cell lung cancer and advanced melanoma that a high level of F. prausnitzii at baseline is positively associated with a better clinical response to ICI. In MCA205 tumor-bearing mice, administration of F. prausnitzii strain EXL01, already in clinical development for Inflammatory Bowel Disease, restores the anti-tumor response to ICI in the context of antibiotic-induced microbiota perturbation at clinical and tumor transcriptomics level. In vitro, EXL01 strain enhances T cell activation in the presence of ICI. Interestingly, oral administration of EXL01 strain did not induce any change in fecal microbiota diversity or composition, suggesting a direct effect on immune cells in the small intestine. F. prausnitzii strain EXL01 will be evaluated as an adjuvant to ICI in multiple cancers in the near future.
Introduction
Immunotherapy has revolutionized the oncology landscape by releasing the “breaks” on patient’s T cells. Immune checkpoint inhibitors (ICI) such as antibodies targeting CTLA-4, PD-1 and its ligand (PD-L1), are proven effective in several solid and hematologic cancers.Citation1 The gut microbiota plays a key role in shaping the immune response, and is a major tumor-extrinsic regulator of responses to immunotherapy. Early studies reported that antibiotics (ABX) impair the therapeutic effect of ICI (anti-PD-1 alone or combined with anti-CTLA-4 antibodies) in sarcoma and melanoma-bearing mice with increased tumor size and reduced overall survival (OS).Citation2 In humans, early ABX exposure (prior to or early during ICI therapy) has been associated with decreased immunotherapy efficacy in multiple cancers types.Citation2,Citation3 Logically, the next step was to identify gut microbiota features at baseline associated with response to ICI. Multiple studies have reported significant differences in microbial diversity and composition between responding and non-responding melanoma, non-small cell lung cancer (NSCLC) and renal cell carcinoma (RCC) patients treated with anti-PD-1 alone or in combination with anti-CTLA-4 agents (ipilimumab).Citation2,Citation4-8 Enrichment of specific commensal bacteria such as Bifidobacteriaceae spp., Ruminococcaceae spp., and more specifically F. prausnitzii,Citation4,Citation6,Citation9 Akkermansia muciniphilaCitation2 or bothCitation10 have been correlated with improved progression-free survival (PFS) and OS to both anti-CTLA-4 and anti-PD-L1 agents in patients with metastatic melanoma, RCC, and NSCLC. A first study of 26 patients with metastatic melanoma treated with anti-CTLA-4 agents suggested that patients with baseline gut microbiota enriched with Faecalibacterium species (and other members of the Firmicutes phylum) had improved PFS and OS compared to patients who were not enriched.Citation4 Simultaneously, a second study on 43 patients with metastatic melanoma treated with anti-PD-1 agents showed the same results, with high F. prausnitzii relative abundance reported as the strongest signal of response to ICI.Citation6 These two cohorts were then further expanded with 38 patients and the results were confirmed in 293 additional melanoma patients.Citation9,Citation11 A causality link was then established by showing that mice colonized with microbiota from human patients that responded to ICI had a better response to ICI in a melanoma model than their counterparts colonized with the microbiota from non-responders.Citation6 Interestingly, the positive response to ICI in this context was also associated with higher F. prausnitzii levels.Citation6 Several other studies also identified that high F. prausnitzii level was associated with better response to ICI in multiple cancer types. A meta-analysis found that high abundance of Faecalibacterium genus was the strongest signal associated with response to ICI, using either anti-PD-1 or anti-CTLA-4 antibodies.Citation5–7,Citation12,Citation13 Baseline enrichment of F. prausnitzii in stools was found as a predictor of response to ICI in hepatocellular carcinomaCitation14 and in NSCLC.Citation15 Another study performed deep shotgun metagenomics sequencing in 2 cohorts of patients with diverse rare cancers treated with combination ICI.Citation16 Comforted by a meta-analysis of gut metagenomes from 6 additional comparable studies, they confirmed the cross-cancer (and cross-country) validity of strain – response signatures which were dependent on the ICI treatment rather than on the cancer type.Citation16 Recently, a clinical trial assessed the influence of microbiota metabolic signature on clinical outcome in patients with melanoma and treated with another type of immunotherapy, based on the injection of dendritic cells (DC).Citation17 They show that different microbes were associated with prognosis, with F. prausnitzii standing out as the main beneficial taxon for no recurrence at 2 year in the patients receiving the DC treatment.Citation17 In addition, intervention studies in humans have reinforced the hypothesis of a causative role of gut microbiome alterations in the response to ICI. Two small open-label uncontrolled studies reported that fecal microbiota transplantation (FMT) using responders to ICI as donors were able to rescue the failure of ICI in a subpopulation of patients with metastatic melanoma.Citation18,Citation19 Interestingly, some link was found between FMT efficacy and F. prausnitzii level.Citation19
There is thus increasing evidence to suggest that the abundance of F. prausnitzii in the gut may impact clinical response to ICI across multiple cancers. F. prausnitzii, a member of the Firmicutes phylum, is the most abundant species in the human intestinal microbiota of healthy adults, representing around 5% of the total fecal microbiota.Citation20–22 The abundance and ubiquity of F. prausnitzii suggest that it is a major contributor to microbiota functions in healthy individuals. Indeed, F. prausnitzii exhibits immunomodulatory effects, and a high level of F. prausnitzii is associated with health, whereas low level is associated with disease, notably Inflammatory Bowel Disease (IBD)Citation22,Citation23 and cancer.Citation24 F. prausnitzii is thus identified as a strong candidate for the development of live biotherapeutic products (LBP), a new type of biological product containing live microorganisms and applicable to the prevention or treatment of human diseases.
In the current study, we reanalyzed two large datasets of patients treated with ICI for NSCLCCitation10 or advanced melanomaCitation25 and showed in these two independent cohorts that a high abundance of F. prausnitzii at baseline was predictive of a positive response to ICI therapy. We then took advantage of F. prausnitzii EXL01 strain, currently in clinical development for IBD (NCT05542355), to evaluate its effect on the activity of ICI in tumor-bearing mice. We showed that F. prausnitzii EXL01 strain restores the anti-tumor response to anti-PD-L1 treatment in mice with ABX‑induced gut dysbiosis bearing MCA205 sarcomas. Strikingly, the effect was not related to any change in either fecal microbiota diversity or composition, suggesting a direct interaction between F. prausnitzii EXL01 strain and host immune cells in the small intestine. This positive effect of F. prausnitzii EXL01 strain was associated with the restoration of an anti-tumor response to ICI at the tumor transcriptomics level. Finally, we demonstrate that F. prausnitzii E×L01 strain boosts T cell activation in the presence of ICI in vitro.
Material and methods
Overall survival
F. prausnitzii relative abundance from MetaPhlAn 4Citation26 profiles from the Derosa et al.Citation10 and Lee et al.Citation27 cohorts were used to calculate survival curves. Patients were grouped according to the abundance of the bacteria, and survival curves were estimated using the Kaplan – Meier method and compared with the log-rank test. Patient microbiomes from Lee et al.Citation27 were stratified into community types using Dirichlet multinomial mixture.Citation28 Clustering was performed with the DirichletMultinomial package in R (version 1.32.0). Cox regression models were performed to determine hazard ratios and 95% confidence intervals for overall survival, adjusting for other clinical data using the R Survival package (version 3.2–10). Multivariable association between microbial community abundance and community type was examined with MaAsLin2 (version 1.4.0)Citation29 taking into account potential confounding factors (age, gender, BMI, treatment with ABX and disease progression).
Preparation of Faecalibacterium prausnitzii EXL01 strain
F. prausnitzii EXL01 strain was grown at 37°C. The test material was prepared to be ready-to-use in PBS/glycerol, characterized by enumerating the number of morphologically intact bacteria i.e. the Total Cell Count (TCC).
MCA205 tumor-bearing mouse model
All animal studies were carried out under protocols approved by the Institutional Animal Care and Use Committee at University of Bordeaux (France). Four week-old female C57Bl/6J mice obtained from Charles River Laboratories (France) were acclimated for 3 weeks at the Bordeaux University animal facility A2 under specific pathogen-free conditions before study. Based on the model by Routy et al.Citation2 mice were randomized to receive a broad-spectrum ABX combination from Day 14 to 30 (Ampicillin (1 mg/mL, Sigma), Streptomycin (5 mg/mL, Sigma) and Colistin (1 mg/mL, Carbosynth) and 1% sucrose (Sigma, added to compensate the bitter taste of the ABX cocktail and enable the mice to drink normal amount ox ABX-supplemented water throughout the experiments), prepared weekly in drinking water). Sarcoma MCA205 tumor cell line was cultured according to the provider’s specification (Merck) and inoculated subcutaneously at 9 weeks of age (Day 0, 500 000 cells/100 μL/mouse). On Day 5 post-tumor inoculation, mice were randomized based on tumor volume and allocated to vehicle and anti-PD-L1 ±EXL01-treated groups. EXL01 strain was prepared in PBS/Glycerol in ready-to-use vials stored at −80°C. Starting from Day 6, EXL01-strain was administered by oral gavage (200 μL/mouse/d, 1010 TCC per dose). On Days 6, 9, 12, and 15 after tumor cell inoculation, mice were treated with or without intraperitoneal anti-PD-L1 antibody (BioXCell, clone 10F.9G2, 5 mg/kg). Animals were monitored 3 times/week (tumor size, body weight, survival) until Day 30. Tumor volumes were obtained according to the following formula “V = Length * WidthCitation2/2”. Blood and feces were collected on Day −14 (before ABX treatment), Day 1 (before anti-PD-L1 treatment), Day 5 (before EXL01 strain treatments), Day 13 (1 d after the third anti-PD-L1 treatment), and Day 30 post tumor-inoculation (end of experiment). On Day 13, tumor biopsies were also collected and snap-frozen until analysis.
Mixed lymphocyte reaction (MLR) in vitro assay
The co-culture of human HLA-mismatched mDC and CD4+ T cells was performed using PBMCs from three independent healthy donor pairs, with mDC and CD4+ T cells of each pair isolated from different donors. Monocytes were isolated and differentiated into DCs upon GM-CSF and IL4 treatment (1000UI/mL, each) for 6 d and then matured with LPS (3 µg/mL) for 1 d. During this 1-d maturation period, mDCs were treated with/without E×L01 strain at 3 doses (MOI 1, 10 and 100). At the end of maturation, viable mDCs were assessed by flow cytometry using Novocyte Quanteon® for the following expression markers: CD209, CD1a, CD80, CD83, CD86 and PD-L1. mDCs were then cocultured with HLA-mismatched CD4 T cells (prestained with an immunofluorescent probe for cellular replication), in the presence of Nivolumab (1 μg/mL), at two stimulator:responder ratios (1:5 and 1:10) and treated with/without EXL01 strain at the three different doses. Cytokine quantification in the supernatant was performed using specific HTRF-based detection kits (Cisbio, France) at 24 h post-culture for IL-2 and 72 h post-culture for IFN. Proliferation of CD4+ T cells was analyzed using total viable CD4 T cell count and the CellTrace Violet probe at 120 h post-co-culture/treatment. As control conditions, CD4+ T cells were treated with anti-CD3/anti-CD28 Dynabeads (bead-to-cell ratio of 1/1, Thermofisher) and Nivolumab (1 µg/mL) in the absence or presence of EXL01 at the three doses. An additional control condition included the treatment of mDCs alone with Nivolumab (1 µg/mL) in the absence or presence of EXL01.
Results
Fecal levels of Faecalibacterium prausnitzii predict clinical response to PD-1 blockade in patients with advanced non-small-cell lung cancer
Derosa et al.Citation10 showed that levels of fecal Akkermansia muciniphila correlated with ICI clinical success in patients NSCLC treated with ICI. Using this cohort of patients with advanced NSCLC, we studied the potential role of F. prausnitzii in the clinical response to ICI. The 337 patients were separated into three groups according to their baseline relative abundance of F. prausnitzii (low, medium and high tertile) in stools. Higher F. prausnitzii abundance was associated with better OS, with a dose effect (p = .008, ). Upon analysis based on ABX exposure, the predictive effect of the abundance of F. prausnitzii was lost in a subgroup of patients exposed to ABX within 2 months prior to starting ICI treatment (p = .83, ), in contrast to patients who did not receive ABX (p = .031, ). The loss of the predictive effect of F. prausnitzii on OS in patients exposed to ABX-induced microbiota perturbations suggests that the microbiota directly influences the activity of ICI. Patients exposed to ABX had a lower relative abundance of F. prausnitzii, and a less rich and diverse microbiota (Supp. Figure S1a,b). Furthermore, we also observed that high F. prausnitzii abundance was predictive of a higher OS when we applied the thresholds defining F. prausnitzii abundance tertile from the non-ABX-exposed subgroup to the ABX-exposed one (Supp. Figure S1c) suggesting that despite ABX-induced microbiota perturbations, patients harboring high F. prausnitzii levels exhibit a positive response to ICI. To evaluate the potential interaction with PD-L1 expression, which is predictive of a positive response to ICI in NSCLC,Citation30 we further separated the cohort into three tertiles according to PD-L1 expression levels (0, 1–49% and ≥50%). The predictive effect of F. prausnitzii on OS was observed in patients with low (<50%) or no PD-L1 expression (, Supp. Figure S1e).
Figure 1. F. prausnitzii level predicts clinical response to PD-L1 in patients with advanced non-small-cell lung cancer.
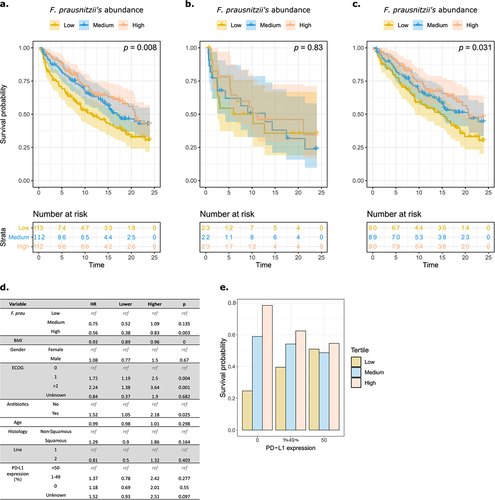
Fecal levels of Faecalibacterium prausnitzii predict clinical response to ICI in a dominant group of patients with advanced melanoma
Using a cross-cohort study evaluating the association between the gut microbiome and response to ICI in advanced melanoma,Citation27 we pooled the 165 patients from the 5 cohorts and separated them according to their relative abundance of F. prausnitzii. Unlike the previous cohort, best results were obtained with two groups (low abundance tertile vs pool of medium & high abundance tertiles). Six patients were negative for F. prausnitzii and were classified in the low abundance tertile. The OS analyses on the whole population did not reveal any significant differences (the same results were observed when using the cut-offs of ); however, there was a trend toward a higher OS in patients with higher relative abundance of F. prausnitzii (). As the original study comprised samples from five distinct cohorts, a Dirichlet Multinomial Mixtures approach was used to identify underlying microbiota structure and different community types. Using the Laplace approximation, we determined that the optimum number of clusters was two (). The multivariate analysis in shows the most significant differences in terms of composition between the two community types. Of note, the clustering was not only dependent on the original five cohorts as patients from several cohorts (Manchester, Leeds, PRIMM.NL, PRIMM.UK) were distributed in the two community clusters (Supp. Figure S2a). Also, there were no differences in the microbiota richness and diversity between the two communities (Supp. Figure S2b) nor in the abundance of F. prausnitzii (Supp. Figure S2c). In the first community type (comprising two-thirds of the global cohort), patients with higher levels of F. prausnitzii had a better OS than those with a low F. prausnitzii level. However, no such signal was observed in the second community type (). Multivariate Cox regression analysis confirmed the independent association between F. prausnitzii level and OS in the first (HR = 0.44; 95% CI = 0.21–0.94; p = .03) but not in the second (HR = 0.63; 95% CI = 0.19–2.02; p = .46) community type ().
Figure 2. F. prausnitzii level predicts clinical response to ICI in a dominant group of patients with advanced melanoma.
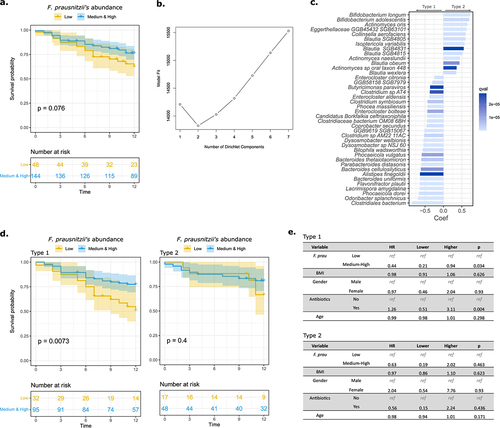
F. prausnitzii EXL01 strain restores response to ICI in presence of antibiotic-induced gut microbiota perturbation
These new results in two independent cohorts, together with previous studies,Citation4,Citation6,Citation9,Citation11,Citation12,Citation14,Citation15 confirm that a high abundance of F. prausnitzii in stools at baseline is predictive of a positive response to ICI in multiple cancer types. We thus sought to determine whether oral supplementation of F. prausnitzii can impact responses to ICI. For this purpose, we took advantage of the EXL01 strain, currently being evaluated in a Phase 1 clinical study as a maintenance treatment for Crohn’s disease (NCT05542355). MCA205 tumor-bearing mice were treated with or without ABX (ampicillin, streptomycin and colistin) to induce gut microbiota perturbation and four intraperitoneal anti-PD-L1 injections. In the experimental groups, EXL01 strain was administered daily by oral gavage starting Day 6 post-tumor inoculation (). As expected, in non-ABX-treated mice, anti-PD-L1 treatment significantly reduced tumor volume and increased survival compared to the vehicle group (). In accordance with previously published data,Citation2 ABX treatment significantly reduced the activity of anti-PD-L1, as illustrated by a greater tumor growth and reduced survival, highlighting the microbiota-dependent efficacy of ICI (). Of note, the chosen ABX cocktail alters the mouse microbiota structure without eliminating it completely (discussed in ), thus resembling the type of microbiota alteration cancer patients may experience in clinical practice. In ABX-treated mice, oral administration of EXL01 strain restored the efficacy of anti-PD-L1 treatment as demonstrated by a greater reduction in mean tumor volume and increased survival compared to anti-PD-L1 alone (). EXL01 strain alone is thus able to restore the anti-tumor effect of anti-PD-L1 treatment in the context of gut microbiota perturbation.
Figure 3. F. prausnitzii EXL01strain restores anti-tumor response to ICI in presence of ABX-induced gut microbiota perturbation.
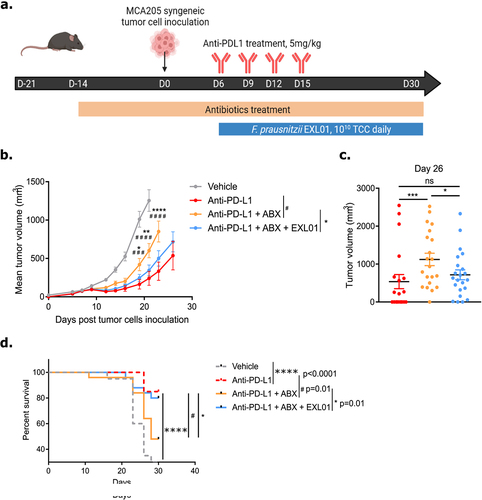
Figure 4. F. prausnitzii EXL01 strain administration does not impact fecal microbiota composition and diversity.
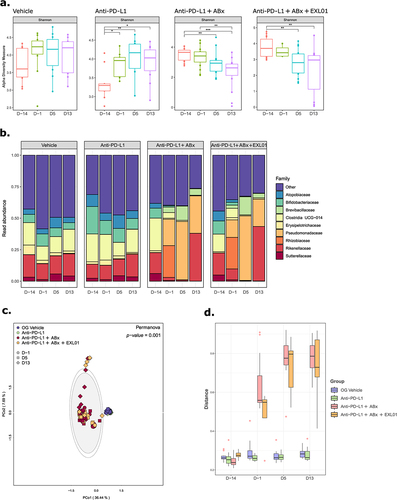
F. prausnitzii EXL01 strain administration does not impact fecal microbiota composition and diversity
To better understand the mechanisms by which F. prausnitzii E×L01 strain can modulate responses to ICI, the fecal microbiota of the mice were analyzed using 16S rRNA gene sequencing at different time points during the treatment course. In mice treated with ABX alone, alpha diversity decreased over time, whereas it increased in the group treated with anti-PD-L1 alone (). In the ABX-treated groups, the relative abundance of many bacterial families increased, while others decreased (, Supp. Figure S3). Compared to the effect of ABX, there were no major changes in the bacterial communities over time in the group treated with anti-PD-L1 alone, indicating that the anti-PD-L1 treatment did not have significant effect on fecal microbiota composition (, Supp. Figure S3). Likewise, the administration of E×L01 strain in combination with ABX and anti-PD-L1 treatment did not shift the microbial communities, with almost no taxonomic difference between the groups (, Supp. Figure S3). Importantly, F. prausnitzii was detected neither at baseline, nor at any other timepoints in the mice feces in any of the studied groups. These analyses demonstrated that changes in fecal microbiota composition over time were primarily due to the addition of ABX rather than anti-PD-L1 and E×L01 strain treatment, suggesting that E×L01 strain modulation of ICI activity is not a result of changes in the composition or diversity of the fecal microbiome.
F. prausnitzii EXL01 strain administration restores normal response to ICI at the tumor gene expression level in gut microbiota perturbation context
To investigate the effects of EXL01 strain at the tumor level, we conducted RNA sequencing analysis on the tumors biopsies collected 13 d post tumor inoculation. Principal component analysis (PCA) showed a clear clustering according to treatment groups (, PERMANOVA: F = 3.2484, df = 3, p = .001). Our analysis revealed that 3,835 genes were differentially expressed between the groups treated by vehicle and by anti-PD-L1 alone (padj <0.05, ). Treatment with anti-PD-L1 led to an upregulation of genes associated with immune response and a downregulation of genes involved in cell proliferation (). A comparison between the group treated with anti-PD-L1 alone and the group treated with both ABX and anti-PD-L1 revealed that 3,313 genes were differentially expressed (padj <0.05, ). Genes involved in cell proliferation-related pathways were upregulated in ABX-treated mice, suggesting that ABX blocked the ability of anti-PD-L1 to modulate cancer cell proliferation (). In mice that received oral EXL01 strain, gene expression was restored, as demonstrated by 4,468 differentially expressed genes between anti-PD-L1 and ABX treatment group and the group that received anti-PD-L1, ABX and EXL01 strain (padj <0.05, ). This finding was further confirmed by comparing the group treated with anti-PD-L1 alone and the group treated with anti-PD-L1, ABX and EXL01 strain, where almost no genes and no functional pathway were differentially expressed (). Consistent with this result, PCA analysis showed that mice treated with anti-PD-L1 alone and by anti-PD-L1, ABX and EXL01 strain clustered together (, Pairwise PERMANOVA: F = 1.1252, df = 1, p = .281) providing further evidence that EXL01 strain restored the anti-tumor transcriptomic response to ICI.
Figure 5. F. prausnitzii EXL01strain administration restores normal response to ICI at the tumor transcriptomics level in gut microbiota perturbation context.
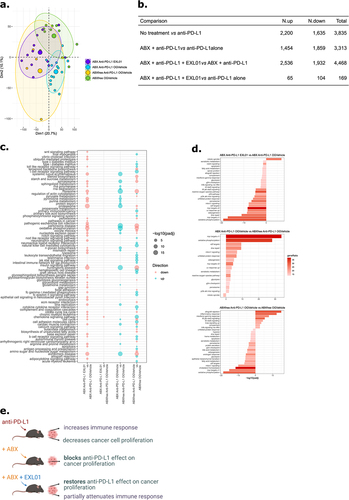
F. prausnitzii EXL01 strain activates both dendritic cells and T cells in the presence of anti-PD-L1
To gain further insight into the potential effect of EXL01 strain on immune cells in the ICI treatment setting, we first co-incubated EXL01 strain with CD4+ T cells and monocyte-derived dendritic cells (mDC) separately, all prepared from PBMCs originating from healthy human donors. In the presence of anti-PD-L1, EXL01 strain increased the production of the anti-tumorigenic cytokine IFN by CD4+ T cells in a dose-dependent manner (). Similar results were observed for IL-2 (Suppl Figure S4a). Stimulation of mDC by E×L01 strain increased the surface expression of the immune-checkpoint protein PD-L1 () and of the activation markers CD80 (CD28 ligand, ) and CD1a (Suppl ) in a dose-dependent manner. No induction of the maturation markers CD83 (Suppl ) and CD86 (Suppl ) was observed, most probably as mDC were matured by LPS before stimulation with EXL01 strain. Interestingly, EXL01 strain reduced the expression of the regulatory marker CD209 (DC-SIGN, ). To explore the potential of EXL01 strain to modulate T cell activation mediated by mDC, we performed a mixed lymphocyte reaction (MLR) assay from three independent healthy donor pairs and evaluated the effect of EXL01 strain on mDC expression profile and T cell activation (). In this co-stimulation setting, EXL01 strain strongly increased the production of IFN
(, Suppl Figure S4e) and, to a lesser extent, the proliferation of CD4+ T cells in percentage and count (, Suppl Figure S4f). Thus, EXL01 strain enhances ICI activity by activating both dendritic cells (DC) and T cells in the presence of anti-PD-L1.
Figure 6. F. prausnitzii EXL01 boost activation of dendritic cells and T cells in the presence of anti-PD-L1.
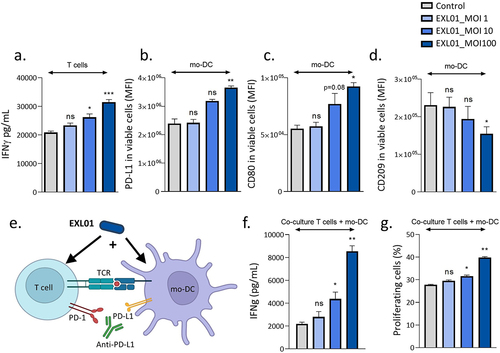
Discussion
The intestinal microbiota is essential to the host physiology and is now established as a key modulator in immune responses.Citation31 In this context, the gut microbiota has been involved in response to cancer treatments, and in particular to immunotherapy.Citation2,Citation6,Citation7,Citation32 Targeting the gut microbiota appears as an attractive strategy to improve response to cancer therapy. Recently, clinical trials suggested that FMT can rescue the efficacy of ICI in non-responders, notably in patients with advanced melanoma refractory to treatment with PD-1 inhibitors, with a response rate of 30–40%.Citation18,Citation19 Although FMT is generally recognized as safe, with no or limited side effects,Citation33 unexpected toxicity has been reported, e.g., extended-spectrum beta-lactamase (ESBL)-producing Escherichia coli bacteremia in two patients who received FMT.Citation34 In addition, FMT reproducibility, scalability and costs limit its broad implementation in chronic and frequent conditions. In this context, there is growing interest on identifying bacterial strains that provide a health benefit and to develop more controlled and specific microbiota-modulating therapies.Citation35 A. muciniphila and F. prausnitzii are among the few human microbiota-derived species that have been positively associated with health and therapeutic potential, notably in metabolic syndrome and inflammatory bowel diseases, and are thus strong candidates for the development of LBP.Citation36,Citation37 In patients with cancer, specific taxa, including F. prausnitzii, A. muciniphila and Bifidobacteria, have been associated with improved clinical responses to ICI.Citation2,Citation6,Citation7 A. muciniphila is positively associated with anti-PD-1 antibodies response in NSCLC patients,Citation2,Citation38 and its baseline level in stool has been shown to predict this enhanced response.Citation10 However, higher A. muciniphila level (above 4.8% abundance) in NSCLC patients is surprisingly associated with poor survival rates.Citation10 Such a high relative abundance of A. muciniphila coincided with ABX use (20% of cases) and presence of the genus Clostridium, both associated with resistance to ICI.Citation10 Further investigation is necessary to explore the impacts and safety of A. muciniphila supplementation in the context of ICI treated patients. Recently, a small size randomized clinical trial suggested that supplementation of the Bifidobacterium spp. CBM588 significantly improved PFS when added to a dual CTLA-4 and PD-1 inhibition treatment in patients with metastatic renal cell carcinoma.Citation39 Interestingly, Bifidobacterium spp. increased with CBM588 therapy, specifically in responders.Citation39 However, half of the patients, supplemented or not with CBM588, developed toxicity that was associated with an increased abundance of E. coli and Klebsiella spp.Citation39 Another study linked toxicity with dual CTLA-4 and PD-1 inhibition to an increased abundance of Bacteroides intestinalis in the stool of patients with melanoma.Citation40
In the current study, we showed that high levels of F. prausnitzii in the intestinal microbiota were positively correlated with a better response to ICI in two independent cohorts of non-small cell lung cancerCitation10 or advanced melanoma.Citation25 In a mouse preclinical model of ICI treatment, we demonstrated that F. prausnitzii EXL01 strain restores the response to ICI in the context of ABX-induced gut microbiota perturbation at both clinical and tumor transcriptomic levels. Interestingly, F. prausnitzii colonization has also been shown to abrogate ICI-induced colitis and improve ICI antitumor activity in two mouse cancer models.Citation41 In vitro, we demonstrate direct interactions between F. prausnitzii EXL01 strain and host immune cells, through activation of both DC and CD4+ T cells in the presence of ICI. Multiple studies explored the mechanisms underlying the therapeutic effect of F. prausnitzii in the context of intestinal inflammation. However, those involved in boosting the response to ICI remain unknown. Interestingly, F. prausnitzii EXL01 strain supplementation was not associated with a change in fecal microbiota composition or diversity. F. prausnitzii EXL01 is an extremely oxygen-sensitive (EOS) bacterium. Even brief oxygen exposure can hinder its growth and even its regrowth when returned to strictly anaerobic conditions. In both in vitro and in vivo experiments, oxygen exposure leads to the loss of EXL01 culturability. However, despite this, the bacterium still exhibits potent biological effects, suggesting that the biological activity of EXL01 does not necessarily depend on metabolically active or culturable bacteria. Similar results were observed with the supplementation of a bifidogenic live bacterial product in renal carcinoma patients.Citation39 These results suggest that F. prausnitzii EXL01 strain might act through direct interactions with host immune cells in the small intestine, although the precise mechanisms remain to be elucidated. Thus, the F. prausnitzii EXL01 strain, already in clinical development stage for IBD (NCT05542355), is an ideal candidate strain for the development of LBP in immune-oncology indications, as it is well characterized, with immune-modulatory properties and a striking ability to potentiate immunotherapy in preclinical studies. It will be evaluated as an adjuvant to ICI in multiple cancers in the near future starting with metastatic gastric cancer (NCT06253611).
Author contributions
M.B., C.D., H.S. designed the study, performed the analyses, interpreted the results and drafted the manuscript. H.P.P. performed the RNA-seq analysis; LD, BR, LZ and NS provided clinical and shotgun metagenomics data. AB performed experiments; L.C., LB and I.A. provided technical help. All authors provided intellectual input, reviewed and approved the manuscript.
Ethics approval
Animals were housed in animal facility “Animalerie A2 Université de Bordeaux” which received approval B33-063-916 from the DDPP (Direction Départementale de Protection des Populations). Animal experiments were performed under ethical protocol 19,664–201903071145699.
Sup_Fig_1.jpg
Download JPEG Image (1.3 MB)Sup_Fig_3.jpg
Download JPEG Image (3.6 MB)Sup_Fig_4.jpg
Download JPEG Image (1.2 MB)Sup_Fig_2.jpg
Download JPEG Image (688.9 KB)Supplementary_material.docx
Download MS Word (18.4 KB)Acknowledgments
LZ and LD were supported by SEERAVE Foundation, European Union Horizon 2020, Project Number 825410 and Project Acronym ONCOBIOME, Institut National du Cancer (INCa), ANR Ileobiome – 19-CE15-0029-01, ANR RHU5 “ANR-21-RHUS-0017” IMMUNOLIFE”, MAdCAM INCA_16698, ANR-IHU Méditerranée-Infections, Ligue contre le cancer. European Union’s Horizon 2020 research and innovation programme under grant agreement number: 825410, Project Acronym: ONCOBIOME, Project title: Gut OncoMicrobiome Signatures (GOMS) associated with cancer incidence, prognosis and prediction of treatment response.
Disclosure statement
HS report lecture fee, board membership, or consultancy from Amgen, Fresenius, IPSEN, Actial, Astellas, Danone, THAC, Biose, BiomX, Eligo, Immusmol, Adare, Nestle, Ferring, MSD, Bledina, Pfizer, Biocodex, BMS, Bromatech, Gilead, Janssen, Mayoli, Roche, Sanofi, Servier, Takeda, Abbvie, has stocks from Enterome bioscience and is co-founder of Exeliom Biosciences. PL report lecture fee, board membership, or consultancy from Biose, Biostime, Boiron, Bonduelle, BMS, Bromatech, IPSEN, iTaK, Lallemand, Lesaffre, L’Oréal, Mayoli, Merck, Procter and Gamble, Second Genome, Therascience and URGO and is co-founder of Exeliom Biosciences. PR is an employee of Exeliom Biosciences. LD was supported by Philantropia Fondation. BR reports grants from Davoltera outside the submitted work, as well as consulting fees from BMS, AstraZeneca, Merck and Davolterra and he is the co-founder of Curebiota. ABC reports lecture fee, board membership, or consultancy from Amgen, Astra-Zeneca, Novartis, Merck KGaA, Pfizer, Roche, Janssen, Abbvie, Sanofi, Takeda, MSD, BMS, InhaTarget and Exeliom. The other authors declare that they have no competing interests.
Data availability statement
Raw sequence RNAseq data are accessible in Gene Expression Omnibus (accession number GSE234818), and 16S data are accessible in the European Nucleotide Archive (accession number PRJNA975093).
Supplementary material
Supplemental data for this article can be accessed online at https://doi.org/10.1080/2162402X.2024.2374954
Correction Statement
This article has been republished with minor changes. These changes do not impact the academic content of the article.
Additional information
Funding
References
- Ribas A, Wolchok JD. Cancer immunotherapy using checkpoint blockade. Science. 2018;359(6382):1350–12. doi:10.1126/science.aar4060.
- Routy B, Le Chatelier E, Derosa L, Duong CPM, Alou MT, Daillère R, Fluckiger A, Messaoudene M, Rauber C, Roberti MP, et al. Gut microbiome influences efficacy of PD-1–based immunotherapy against epithelial tumors. Science. 2018;359(6371):91–97. doi:10.1126/science.aan3706.
- Derosa L, Hellmann MD, Spaziano M, Halpenny D, Fidelle M, Rizvi H, Long N, Plodkowski AJ, Arbour KC, Chaft JE, et al. Negative association of antibiotics on clinical activity of immune checkpoint inhibitors in patients with advanced renal cell and non-small-cell lung cancer. Annals of Oncology. 2018;29(6):1437–1444. doi:10.1093/annonc/mdy103.
- Chaput N, Lepage P, Coutzac C, Soularue E, Le Roux K, Monot C, Boselli L, Routier E, Cassard L, Collins M, et al. Baseline gut microbiota predicts clinical response and colitis in metastatic melanoma patients treated with ipilimumab. Ann Oncol. 2017;28(6):1368–1379. doi:10.1093/annonc/mdx108.
- Frankel AE, Coughlin LA, Kim J, Froehlich TW, Xie Y, Frenkel EP, Koh AY. Metagenomic shotgun sequencing and unbiased metabolomic profiling identify specific human gut microbiota and metabolites associated with immune checkpoint therapy efficacy in melanoma patients. Neoplasia. 2017;19(10):848–855. doi:10.1016/j.neo.2017.08.004.
- Gopalakrishnan V, Spencer CN, Nezi L, Reuben A, Andrews MC, Karpinets TV, Prieto PA, Vicente D, Hoffman K, Wei SC, et al. Gut microbiome modulates response to anti–PD-1 immunotherapy in melanoma patients. Science. 2018;359(6371):97–103. doi:10.1126/science.aan4236.
- Matson V, Fessler J, Bao R, Chongsuwat T, Zha Y, Alegre M-L, Luke JJ, Gajewski TF. The commensal microbiome is associated with anti–PD-1 efficacy in metastatic melanoma patients. Science. 2018;359(6371):104–108. doi:10.1126/science.aao3290.
- Derosa L, Routy B, Fidelle M, Iebba V, Alla L, Pasolli E, Segata N, Desnoyer A, Pietrantonio F, Ferrere G, et al. Gut bacteria composition drives primary resistance to cancer immunotherapy in renal cell carcinoma patients. Eur Urol. 2020;78(2):195–206. doi:10.1016/j.eururo.2020.04.044.
- Spencer CN, McQuade JL, Gopalakrishnan V, McCulloch JA, Vetizou M, Cogdill AP, Khan MAW, Zhang X, White MG, Peterson CB, et al. Dietary fiber and probiotics influence the gut microbiome and melanoma immunotherapy response. Science. 2021;374(6575):1632–1640. doi:10.1126/science.aaz7015.
- Derosa L, Routy B, Thomas AM, Iebba V, Zalcman G, Friard S, Mazieres J, Audigier-Valette C, Moro-Sibilot D, Goldwasser F, et al. Intestinal Akkermansia muciniphila predicts clinical response to PD-1 blockade in patients with advanced non-small-cell lung cancer. Nat Med. 2022;28(2):315–324. doi:10.1038/s41591-021-01655-5.
- Coutzac C, Jouniaux J-M, Paci A, Schmidt J, Mallardo D, Seck A, Asvatourian V, Cassard L, Saulnier P, Lacroix L, et al. Systemic short chain fatty acids limit antitumor effect of CTLA-4 blockade in hosts with cancer. Nat Commun. 2020;11(1):2168. doi:10.1038/s41467-020-16079-x.
- Limeta A, Ji B, Levin M, Gatto F, Nielsen J. Meta-analysis of the gut microbiota in predicting response to cancer immunotherapy in metastatic melanoma. JCI Insight. 2020;5(23):e140940. doi:10.1172/jci.insight.140940.
- Peters BA, Wilson M, Moran U, Pavlick A, Izsak A, Wechter T, Weber JS, Osman I, Ahn J. Relating the gut metagenome and metatranscriptome to immunotherapy responses in melanoma patients. Genome Med. 2019;11(1):61. doi:10.1186/s13073-019-0672-4.
- Li L, Ye J. Characterization of gut microbiota in patients with primary hepatocellular carcinoma received immune checkpoint inhibitors: a Chinese population-based study. Medicine (Baltimore). 2020;99(37):e21788. doi:10.1097/MD.0000000000021788.
- Newsome RC, Gharaibeh RZ, Pierce CM, da Silva WV, Paul S, Hogue SR, Yu Q, Antonia S, Conejo-Garcia JR, Robinson LA, et al. Interaction of bacterial genera associated with therapeutic response to immune checkpoint PD-1 blockade in a United States cohort. Genome Med. 2022;14(1):35. doi:10.1186/s13073-022-01037-7.
- Gunjur A, Shao Y, Rozday T, Klein O, Mu A, Haak BW, Markman B, Kee D, Carlino MS, Underhill C, et al. A gut microbial signature for combination immune checkpoint blockade across cancer types. Nat Med. 2024 Mar 1;30(3):797–809. doi:10.1038/s41591-024-02823-z.
- Alves Costa Silva C, Piccinno G, Suissa D, Bourgin M, Schreibelt G, Durand S, Birebent R, Fidelle M, Sow C, Aprahamian F, et al. Influence of microbiota-associated metabolic reprogramming on clinical outcome in patients with melanoma from the randomized adjuvant dendritic cell-based MIND-DC trial. Nat Commun. 2024;15(1):1633. doi:10.1038/s41467-024-45357-1.
- Baruch EN, Youngster I, Ben-Betzalel G, Ortenberg R, Lahat A, Katz L, Adler K, Dick-Necula D, Raskin S, Bloch N, et al. Fecal microbiota transplant promotes response in immunotherapy-refractory melanoma patients. Science. 2021;371(6529):602–609. doi:10.1126/science.abb5920.
- Davar D, Dzutsev AK, McCulloch JA, Rodrigues RR, Chauvin J-M, Morrison RM, Deblasio RN, Menna C, Ding Q, Pagliano O, et al. Fecal microbiota transplant overcomes resistance to anti–PD-1 therapy in melanoma patients. Science. 2021;371(6529):595–602. doi:10.1126/science.abf3363.
- Hold GL, Schwiertz A, Aminov RI, Blaut M, Flint HJ. Oligonucleotide probes that detect quantitatively significant groups of butyrate-producing bacteria in human feces. Appl Environ Microbiol. 2003;69(7):4320–4324. doi:10.1128/AEM.69.7.4320-4324.2003.
- Lopez-Siles M, Martinez-Medina M, Surís-Valls R, Aldeguer X, Sabat-Mir M, Duncan SH, Flint HJ, Garcia-Gil LJ. Changes in the abundance of Faecalibacterium prausnitzii phylogroups I and II in the intestinal mucosa of inflammatory bowel disease and patients with colorectal cancer. Inflamm Bowel Dis. 2016;22(1):28–41. doi:10.1097/MIB.0000000000000590.
- Sokol H, Pigneur B, Watterlot L, Lakhdari O, Bermúdez-Humarán LG, Gratadoux J-J, Blugeon S, Bridonneau C, Furet J-P, Corthier G, et al. Faecalibacterium prausnitzii is an anti-inflammatory commensal bacterium identified by gut microbiota analysis of Crohn disease patients. Proc Natl Acad Sci USA. 2008;105(43):16731–16736. doi:10.1073/pnas.0804812105.
- Touch S, Godefroy E, Rolhion N, Danne C, Oeuvray C, Straube M, Galbert C, Brot L, Alonso Salgueiro I, Chadi S, et al. Human CD4+CD8α+ Tregs induced by Faecalibacterium prausnitzii protect against intestinal inflammation. JCI Insight. 2022;7(12):e154722. doi:10.1172/jci.insight.154722.
- Dikeocha IJ, Al-Kabsi AM, Chiu H-T, Alshawsh MA. Faecalibacterium prausnitzii Ameliorates Colorectal Tumorigenesis and Suppresses Proliferation of HCT116 Colorectal Cancer Cells. Biomedicines. 2022;10(5):1128. doi:10.3390/biomedicines10051128.
- Lee N, Zakka LR, Mihm MC, Schatton T. Tumour-infiltrating lymphocytes in melanoma prognosis and cancer immunotherapy. Pathology. 2016;48(2):177–187. doi:10.1016/j.pathol.2015.12.006.
- Blanco-Miguez A, Beghini F, Cumbo F, McIver LJ, Thompson KN, Zolfo M, Manghi P, Dubois L, Huang KD, Thomas AM, et al. Extending and improving metagenomic taxonomic profiling with uncharacterized species with MetaPhlAn 4. Bioinformatics. 2022;41(11):1633–1644.
- Lee KA, Thomas AM, Bolte LA, Björk JR, de Ruijter LK, Armanini F, Asnicar F, Blanco-Miguez A, Board R, Calbet-Llopart N, et al. Cross-cohort gut microbiome associations with immune checkpoint inhibitor response in advanced melanoma. Nat Med. 2022;28(3):535–544. doi:10.1038/s41591-022-01695-5.
- Holmes I, Harris K, Quince C, Gilbert JA. Dirichlet multinomial mixtures: generative models for microbial metagenomics gilbert JA, editor. PLOS ONE. 2012;7(2):e30126. doi:10.1371/journal.pone.0030126.
- Mallick H, Rahnavard A, McIver LJ, Ma S, Zhang Y, Nguyen LH, Tickle TL, Weingart G, Ren B, Schwager EH, et al. Multivariable association discovery in population-scale meta-omics studies Coelho LP, editor. PLOS Comput Biol. 2021;17(11):e1009442. doi:10.1371/journal.pcbi.1009442.
- Topalian SL, Hodi FS, Brahmer JR, Gettinger SN, Smith DC, McDermott DF, Powderly JD, Carvajal RD, Sosman JA, Atkins MB, et al. Safety, activity, and immune correlates of anti–PD-1 antibody in cancer. N Engl J Med. 2012;366(26):2443–2454. doi:10.1056/NEJMoa1200690.
- Zheng D, Liwinski T, Elinav E. Interaction between microbiota and immunity in health and disease. Cell Res. 2020;30(6):492–506. doi:10.1038/s41422-020-0332-7.
- Salgia NJ, Bergerot PG, Maia MC, Dizman N, Hsu J, Gillece JD, Folkerts M, Reining L, Trent J, Highlander SK, et al. Stool microbiome profiling of patients with metastatic renal cell carcinoma receiving anti–PD-1 immune checkpoint inhibitors. Eur Urol. 2020;78(4):498–502. doi:10.1016/j.eururo.2020.07.011.
- Merrick B, Allen L, Masirah M, Zain N, Forbes B, Shawcross DL, Goldenberg SD. Regulation, risk and safety of faecal microbiota transplant. Infect Prev Pract. 2020;2(3):100069. doi:10.1016/j.infpip.2020.100069.
- DeFilipp Z, Bloom PP, Torres Soto M, Mansour MK, Sater MRA, Huntley MH, Turbett S, Chung RT, Chen Y-B, Hohmann EL. Drug-resistant E. coli bacteremia transmitted by fecal microbiota transplant. N Engl J Med. 2019;381(21):2043–2050. doi:10.1056/NEJMoa1910437.
- Erdmann J. How gut bacteria could boost cancer treatments. Nature. 2022;607(7919):436–439. doi:10.1038/d41586-022-01959-7.
- O’Toole PW, Marchesi JR, Hill C. Next-generation probiotics: the spectrum from probiotics to live biotherapeutics. Nat Microbiol. 2017;2(5):17057. doi:10.1038/nmicrobiol.2017.57.
- Martín R, Langella P. Emerging health concepts in the probiotics field: streamlining the definitions. Front Microbiol. 2019;10:1047. doi:10.3389/fmicb.2019.01047.
- Jin Y, Dong H, Xia L, Yang Y, Zhu Y, Shen Y, Zheng H, Yao C, Wang Y, Lu S. The diversity of gut microbiome is associated with favorable responses to anti–programmed death 1 immunotherapy in Chinese patients with NSCLC. J Thorac Oncol. 2019;14(8):1378–1389. doi:10.1016/j.jtho.2019.04.007.
- Dizman N, Meza L, Bergerot P, Alcantara M, Dorff T, Lyou Y, Frankel P, Cui Y, Mira V, Llamas M, et al. Nivolumab plus ipilimumab with or without live bacterial supplementation in metastatic renal cell carcinoma: a randomized phase 1 trial. Nat Med. 2022;28(4):704–712. doi:10.1038/s41591-022-01694-6.
- Andrews MC, Duong CPM, Gopalakrishnan V, Iebba V, Chen W-S, Derosa L, Khan MAW, Cogdill AP, White MG, Wong MC, et al. Gut microbiota signatures are associated with toxicity to combined CTLA-4 and PD-1 blockade. Nat Med. 2021;27(8):1432–1441. doi:10.1038/s41591-021-01406-6.
- Gao Y, Xu P, Sun D, Jiang Y, Lin X-L, Han T, Yu J, Sheng C, Chen H, Hong J, et al. Faecalibacterium prausnitzii abrogates intestinal toxicity and promotes tumor immunity to increase the efficacy of dual CTLA4 and PD-1 checkpoint blockade. Cancer Res. 2023;83(22):3710–3725. doi:10.1158/0008-5472.CAN-23-0605.