ABSTRACT
The long story of NK cells started about 50 y ago with the first demonstration of a natural cytotoxic activity within an undefined subset of circulating leukocytes, has involved an ever-growing number of researchers, fascinated by the apparently easy-to-reach aim of getting a “universal anti-tumor immune tool”. In fact, in spite of the impressive progress obtained in the first decades, these cells proved far more complex than expected and, paradoxically, the accumulating findings have continuously moved forward the attainment of a complete control of their function for immunotherapy. The refined studies of these latter years have indicated that NK cells can epigenetically calibrate their functional potential, in response to specific environmental contexts, giving rise to extraordinarily variegated subpopulations, comprehensive of memory-like cells, tissue-resident cells, or cells in various differentiation stages, or distinct functional states. In addition, NK cells can adapt their activity in response to a complex body of signals, spanning from the interaction with either suppressive or stimulating cells (myeloid-derived suppressor cells or dendritic cells, respectively) to the engagement of various receptors (specific for immune checkpoints, cytokines, tumor/viral ligands, or mediating antibody-dependent cell-mediated cytotoxicity). According to this picture, the idea of an easy and generalized exploitation of NK cells is changing, and the way is opening toward new carefully designed, combined and personalized therapeutic strategies, also based on the use of genetically modified NK cells and stimuli capable of strengthening and redirecting their effector functions against cancer.
Introduction
Different effector cells are involved in anti-tumor activity, including αβ and γδ T lymphocytes, natural killer (NK) cells, and M1-polarized macrophages. In addition, dendritic cells (DC) instruct T lymphocytes by presenting tumor peptides, while phagocytes may participate in eliminating apoptotic and necrotic tumor cells. Despite the potential effectiveness of all these cellular mechanisms, tumors can counteract and escape the immune response, primarily by creating a hostile environment, both by directly impairing immune effector cells or by instructing different immune and nonimmune cells to become immunosuppressive.Citation1 These effects lead to a suppressive tumor microenvironment (TME), which may subvert the potential anti-tumor effect. Among defensive cells, NK lymphocytes play a major role in anti-tumor activity. They were discovered in the mid ‘70s, but only in the late ‘80s, the inspiring “missing self hypothesis”Citation2 led to the identification in the early ‘90s of the Human Leukocyte Antigen (HLA)-specific killer Ig-like receptors (KIRs), CD94/NKG2A, and of activating receptors.Citation3–7 These latter receptors allow the detection and killing of tumor cells, including the Natural Cytotoxicity Receptors (NCRs: NKp46, NKp44, NKp30), NKG2D, and DNAM-1.Citation8–10
This contribution intends to briefly review the main interactions occurring between NK cells and tumor cells, the effect of suppressive activity of TME, and the immunotherapeutic approaches which may restore and/or potentiate the anti-tumor activity of NK cells. Some essential notions of NK cells and their receptors will be discussed to allow a deeper understanding of the NK/tumor cell interactions.
NK cell receptors and subsets
NK cells contribute to a first line of innate defenses: they are naturally equipped with a lytic machinery which allows the killing of some pathological target cells, including tumor and virus-infected cells. While innate immunity can frequently stop infections at a subclinical level, in more severe cases, it can contain infection allowing the intervention of the more efficient and specific, adaptive immunity, which involves T lymphocytes and, subsequently, B-cell-derived antibodies.Citation11
The molecular mechanisms which allow NK cells to discriminate between healthy and abnormal cells remained a mystery for a long time. The main questions were as follows: 1) which receptors do allow NK cells to selectively hit tumor and virally infected cells? 2) what makes these target cells susceptible to NK cells? Capitalizing on the development of a sophisticated technique, allowing high cloning efficiency of T, and, subsequently, NK cells, Alessandro Moretta and coworkers discovered the prototypes and most important inhibitory receptors, i.e., KIRs.Citation3 These receptors, recognizing groups of HLA class I (HLA-I) allotypes, inhibit NK cell functions, avoiding killing healthy self cells. The major families of inhibitory receptors for HLA-I are the KIRs and the CD94/NKG2A heterodimer. In particular, KIR2DL1 and KIR2DL2/3 recognize HLA-C molecules carrying lysine and asparagine at position 80, respectively. KIR3DL1 binds to HLA-B and -A allotypes sharing the Bw4 epitope, and KIR3DL2 recognizes HLA-A *03 and -A *11. Differently, CD94/NKG2A is specific to the non-classical HLA-E molecule. The discovery of KIRs attracted the attention of immunogenetics researchers to study the KIR gene family and allowed the identification of two different haplotypes (termed A and B) differing in gene content (i.e., number and type of KIR genes).Citation12–14 The evidence of an “off” signal implies the existence of an “on” signal occurring when NK cells interact with abnormal target cells. This led to the discovery, by the same research group, of activating receptors involved in the recognition of abnormal cells and in the induction of the functional program of NK cells, i.e., cytotoxicity and cytokine production (primarily IFN-γ and TNF-α).Citation8–10,Citation15 Importantly, the molecular structures recognized by activating NK receptors on target cells are either absent or expressed at low levels on healthy cells, while they become overexpressed in “stressed” cells. While also healthy cells can express such ligands for activating NK receptors upon “stress” induced by cytokines and cell proliferation/activation, they do express normal, or even increased levels of HLA-I molecules. The latters, upon interaction with KIR or CD94/NKG2A inhibitory receptors, inactivate NK cells, thus protecting them from NK-mediated attack. In contrast, tumor cells, as well as virally infected cells, may lose HLA expression or display an altered structure of HLA-I.
Some basic concepts regarding NK cell development and subsets are mentioned below.
Traditionally, two main NK cell subsets have been distinguished in peripheral blood (PB) based on the cell surface density of CD56 and CD16 molecules, termed CD56brightCD16dim/− and CD56dimCD16+, with the former believed to be less mature but capable of producing high levels of cytokines (e.g., IFN-γ and TNF-α) and the latter more mature and cytotoxic.Citation16,Citation17 Furthermore, CD56dim NK cells can mediate antibody-dependent cell-mediated cytotoxicity (ADCC) through the engagement of CD16, the low-affinity receptor for the Fc fragment of immunoglobulin G (FcγRIIIA, CD16A).Citation18 PB CD56brightCD16− NK cells are uniformly positive for the HLA-E-specific inhibitory receptor CD94/NKG2A but lack KIRs. Differently, PB CD56dimCD16+ NK cells show variable expression of CD94/NKG2A and KIRs. Further distinctions were made within the CD56dimCD16+ NK cell subset which led to the description of a fully differentiated NK cell subset, characterized by the expression of CD57 and the absence of CD94/NKG2A, and a subset of mature NK cells, expressing the HLA-E-specific CD94/NKG2C activating receptor and displaying “adaptive” immune features in the context of CMV infection (called adaptive NK cells).Citation19,Citation20 Indeed, under certain conditions NK cells undergo antigen-specific clonal expansion and become long-lived memory cells, features considered hallmarks of adaptive immunity lymphocytes.
Recent studies have also characterized NK cells in different tissues, each with distinct phenotypic and functional profiles. Indeed, the presence of tissue-resident NK cells has been shown in certain tissues, where they are retained thanks to the expression of the CD103 (αE integrin) and CD49a (α1 integrin) tissue residency markers.Citation17,Citation21 In addition, the development of advanced single-cell technologies, such as scRNA-seq and CITE (Cellular Indexing of Transcriptomes and Epitopes)-seq, has accelerated the dissection of NK cell subset heterogeneity, revealing an intricate and nuanced NK cell landscape as well as different trajectories in their differentiation from hemopoietic precursors. Nevertheless, data based on these technologies still require a careful comparison with established markers and, more in general, with the proteins that are actually expressed. Another important concept related to NK cell maturation is the process of NK cell licensing or education, during which only NK cells expressing KIRs and/or CD94/NKG2A recognizing self HLA-I alleles become fully functional.Citation22–24
Tumor escape mechanisms in the tumor microenvironment
To understand the potential efficacy of NK cell-mediated anti-tumor activity, the accessibility of NK cells into the tumor and the role of TME have to be taken into consideration .Citation25–28
Figure 1. Inhibition of NK cell-mediated anti-tumor response by tumor microenvironment.
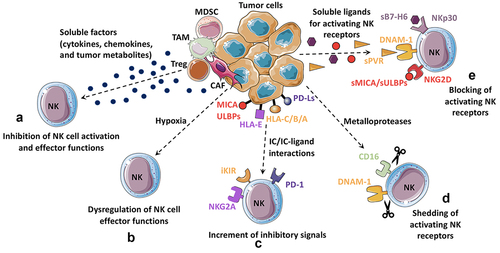
NK chemokine receptors and tissue chemoattractants
NK cell migration into inflamed tissues is controlled by adhesion molecules, chemokine receptors, and chemokine gradients. While CD56dim NK cells express CXCR1, CX3CR1, CXCR2, and low levels of CXCR3, CD56bright NK cells express L-selectin (CD62L), CCR7, CCR5, and CXCR3.Citation29 Therefore, these two NK cell subsets show different migration capabilities. Chemokine receptor expression differences account for the differential migration of CD56dim cells and CD56bright NK cells into inflamed tissues and secondary lymphoid organs, respectively. However, so far, it is not known whether CD56bright and CD56dim NK cell subsets are differentially recruited to the tumor because of their diverse chemokine receptor repertoire.Citation30,Citation31 At least in some tumors, it has been described that the presence of high NK cell infiltration is related to a more favorable outcome.Citation32 It is conceivable that NK cell recruitment into the tumor bed might be regulated by CXCR3 and CX3CR1 chemokine receptors. Experimental cancer models have shown that gene therapy with CX3CL1/fractalkine and CCL2 can stimulate tumor rejection by boosting NK cell infiltration and activation.Citation33,Citation34 In addition, it has been suggested that NK cells exposed to IL-15 and glucocorticoids express a high level of CXCR3, thereby increasing their potential to infiltrate CXCL10-positive melanomas. Thus, in humans, CD56bright could be preferentially recruited to the tumor due to their surface expression of CXCR3.Citation35,Citation36 Transforming Growth Factor beta (TGF-β) promotes the deregulation of the CX3CL1–CX3CR1 axis thus inducing the recruitment of CD56bright NK cells at the expense of the CD56dim subset. On the same line, increased levels of CCL19, CXCL9, and CXCL10 and decreased levels of CXCL2 in lung tumors foster the recruitment of CD16− NK cells, to the detriment of the more cytotoxic CD16+ NK cells.Citation37 Also, CD56dim NK cells have been found in human lung tumors, colorectal cancer, and lymph node melanoma metastases.Citation38–40 In addition to chemokines, other factors could boost the recruitment of NK cells in tumors. In a melanoma model, chemerin, an important chemoattractant, enhances the infiltration of immune cells expressing the chemerin receptor CMKLR1 (i.e., NK cells, T cells, and DCs) into tumors.Citation41 In addition, our group has demonstrated that NK cells, upon interaction with melanoma cells, can release a form of high mobility group box-1 protein able to chemoattract activated NK cells.Citation42 Moreover, NK cells can be recruited into the tumor DC capable of prime T cell-mediated immunity. In human cancers, intratumoral CCL5, XCL1, and XCL2 transcripts closely correlate with gene signatures of both NK cells and conventional type 1 DC and are associated with increased overall patient survival in several cancer types.Citation43 In addition, these NK cells, through secretion of FLT3L, control the abundance of intratumoral stimulatory DC and further the responsiveness of melanoma patients receiving anti-PD-1 therapy.Citation44
On the other hand, it has been shown that tumor cells may also affect the ability of NK cells to enter a solid tumor site. In this context, it has been shown that neuroblastoma-derived TGF-β could skew the NK cell chemokine-receptor repertoire.Citation45
Tumor-infiltrating cells
In the TME, different immune and stromal cells are present, as well as a heterogeneous array of soluble factors endowed with an immune suppressive activity.Citation46,Citation47 Several tumor-infiltrating cell types can shape the TME and affect NK cell functions through different mechanisms. In the TME, the major cellular components are represented by myeloid cells, namely tumor-associated macrophages (TAM), myeloid-derived suppressor cells (MDSC), and tumor-associated neutrophils (TAN) ().Citation48–50 In particular, the subset of MDSC of polymorphonuclear lineage (PMN-MDSC) has recently been found not only in TME but also in PB in tumor patients. Importantly, their frequency in PB directly correlated with the severity and the prognosis of lung carcinoma patients. Notably, NK cells in these patients were significantly impaired in their functional activities.Citation51 Thus, it is conceivable that targeting or inactivating PMN-MDSC may represent a promising therapeutic tool. In mouse, it has been described that TAN can impair the cytotoxicity and infiltration capability of NK cells by CCR1 downregulation. Moreover, neutrophils can decrease the responsiveness of the NKp46 and NKG2D activating receptors. Enhanced expression of PD-L1 on neutrophils and of PD-1 on NK cells, and subsequent PD-L1/PD-1 interactions were the main mechanisms determining the neutrophil-mediated suppression of NK cell immunity.Citation52 Other cells can contribute to the development and maintenance of an immune suppressive microenvironment, such as regulatory T lymphocytes (Tregs), frequently expanded in tumor patients and found both in PB and at the tumor site. Their presence in tumor infiltrates correlates with impaired immune function and bad prognosis. In Gastrointestinal Stromal Tumor (GIST) and Hepatocellular Carcinoma (HCC) patients, low NK cell functional capabilities correlate with high numbers of Tregs. Along this line, Tregs isolated from GIST patients inhibit NK cells through membrane-bound TGF-β.Citation53 In addition, since Treg cells express the high-affinity IL-2 receptor alpha (CD25, IL-2 rα), they could also interfere with NK cell activation by competing for IL-2 availability.
Interestingly, the presence of tumor-associated adaptive NK cells with tissue-resident traits has recently been evaluated in some solid tumors, but the available data are still few and partially conflicting. In particular, tumor-associated NKG2C+ inhibitory self-KIR+ NK cells, expressing the tissue residency marker CD94a, have been described to be present in lung cancer patients but only in CD56bright CD16− NK cells and not in CD56dim CD16+ NK cells as occurring in PB. In addition, these cells were hyperresponsive toward K562 cells in terms of both cytokine release and CD107a degranulation.Citation54 On the other hand, CD56dim FCεRγ− adaptive NK cells have been detected with increased frequencies in HCMV+ HBV-associated hepatocellular carcinoma patients. These cells exhibited low expression of tissue-resident markers (including CD49a, CXCR6, and CD69) and limited anti-tumoral activity toward liver cancer cells, suggesting that TME can influence their anti-tumor capabilities.Citation55
Among tumor-associated stromal cells, activated fibroblasts, often termed cancer-associated fibroblasts (CAF), are considered to play a role in mediating suppressive activity toward NK cells in the TME.Citation25 CAF have been shown to display distinct phenotypes and features with respect to fibroblasts residing in healthy tissues.Citation56,Citation57 For example, CAF derived from melanoma, HCC, and colorectal carcinomas were shown to inhibit NK cell activity through cell-to-cell contact and through secretion of Prostaglandin E2 (PGE2), which could abrogate IL-2-induced upregulation of NKp44, DNAM-1, and NKp30 activating receptors.Citation58–60 In addition, in acute myeloid leukemias (AML), aberrant bone marrow (BM) mesenchymal stromal cells (α-SMA+ MSC) and hypoxia may contribute to generate a pro-tumoral BM niche that can affect NK cell differentiation and lead to an impairment of NK cell cytolytic activity against neoplastic cells.Citation61
Suppressive soluble factors at TME
Tumor-associated cells in the TME and tumor cells themselves can inhibit NK cell anti-tumor effector functions both by establishing cell-to-cell contacts and through the release of several cytokines, inflammatory mediators, reactive oxygen species, and other soluble molecules ().
Among soluble factors, TGF-β plays a pivotal role in tumor-mediated immune suppression. It is produced by different cell types in the TME, including tumor cells themselves, and is exposed on the surface of regulatory immune cells; it can also be delivered to extracellular vesicles or stored as an inactive component inside the extracellular matrix and released through the action of some metalloproteases.Citation62,Citation63 Products of tumor cell metabolism can also be released. For example, both in solid tumors and in leukemias, enhanced tryptophan catabolism leads to an immunosuppressive environment. Thus, overexpression of the indoleamine 2,3-dioxygenase (IDO) enzyme catalyzes tryptophan degradation by producing l-kynurenine, which can directly affect NK cells. Our group showed that melanoma-derived IDO and/or PGE2 could inhibit NK cell surface expression of NKp30, NKp44, and NKG2D that are required for target cell recognition and killing.Citation64 Besides TGF-β, also IL-4, macrophage migration inhibitory factor (MIF), MUC-16, and adenosine can affect NK cell function.Citation65 In particular, it has been described that TGF-β is able to down-regulate NKG2D and NKp30 activating receptor expression, while IL-4 alters the capability of NK cells to produce cytokines.Citation66,Citation67 Ovarian tumor cells can release/express both MIF and MUC-16 glycoprotein: MIF can down-regulate NKG2D expression, while MUC-16 can interfere with the formation of immunological synapses between NK and tumor cells.Citation68,Citation69
The chronic exposure to soluble factors and cell-to-cell contacts in the TME can deeply modify NK cells, inducing an “exhausted” phenotype. Exhausted tumor-associated (TA)-NK cells exhibit upregulation of inhibitory receptors such as PD-1, TIM-3, TIGIT (T cell immunoreceptor with Ig, and Immunoreceptor tyrosine-based inhibition Motif (ITIM) domains), LAG-3, and NKG2A, while they display a decreased expression of activating receptors (such as NKG2D)Citation70,Citation71 and of Eomesodermin and T-bet transcription factors.Citation72,Citation73 Exhausted TA-NK cells exhibit lower ability to proliferate, degranulate, and produce cytokines. In the TME, several metabolic stressed conditions such as nutrient depletion, low oxygen, and low pH can also impair NK cell functions, thus favoring exhaustion.Citation74 It has been shown that NK cell activity, proliferation, and survival rely on either oxidative phosphorylation (at steady-state) or glycolysis (upon activation).Citation75 Along this line, it has been described that during lung cancer progression, the presence of TGF-β in TME-induced inhibition of glycolysis results in a decreased cytotoxicity and viability of NK cells.Citation76 Altogether, these features explain the reduced anti-tumor effector functions frequently displayed by NK cells in the TME.Citation77–84 Although exhausted NK cells do not necessarily display alterations in the content of cytotoxic molecules, the impairment of NK cytotoxic function can also occur through the modulation of the effector molecules normally stored inside lytic granules. In different solid and hematologic tumors, patients’ NK cells have been shown to display alterations in perforin and/or granzyme B expression, mainly mediated by TGF-β.Citation85 In addition, perforin and granzyme expression can be modulated by microRNA (miRNA) carried to NK cells via tumor-derived exosomes. Interestingly, tumor-derived exosomes can deliver several miRNAs with immune-modulating effects, such as miR-544, inhibiting NKp46 expression or miR-146a, down-regulating IFN-γ and TNF-α.Citation86,Citation87
Tumor-NK cell interactions
As described above, tumor cell recognition takes place through multiple NK receptor–ligand interactions dictating whether target cells will be spared or killed by NK cells. Tumor escape mechanisms targeting these interactions represent an effective strategy to limit tumor cell recognition and subsequent cytotoxic activity by NK cells. On the NK cell side, the expression of the main activating NK receptors responsible for tumor cell killing can be reduced by different factors found in the TME. NCRs, NKG2D, and DNAM-1 surface expression can be variably affected by TGF-β, PGE2 or L-kynurenine produced by the IDO enzymeCitation64,Citation66,Citation88,Citation89 In addition, hypoxia, which often characterizes the tumor and its microenvironment, can be responsible for the modulation of activating NK receptors ().Citation90–92 Interestingly, tumor cells undergoing epithelial–mesenchymal transition (EMT), a process favored by hypoxia and other factors present in TME, display an increased ability to induce the down modulation of activating receptors on NK cells.Citation93 NK receptor modulation can also occur following persistent stimulation of NK cells with ligands expressed by cancer cells; in this case, receptor engagement by the corresponding ligand(s) induces receptor endocytosis, resulting in a decreased ability of NK cells to recognize and kill tumor cells.Citation94,Citation95 Receptor modulation has been demonstrated for NCRs, NKG2D, and DNAM-1.Citation96–100 An additional mechanism by which activating NK receptors can be down-regulated involves the activity of metalloproteases, which mediate the shedding of the receptor from the cell surface through proteolytic cleavage. In particular, enzymes belonging to the matrix metalloproteinases (MMP) and ADAM (a disintegrin with metallopeptidase domain) families have been implicated in this process, for example, in the case of CD16 and DNAM-1 receptors ().Citation101–106 In this context, the use of MMP and/or ADAM inhibitors could improve the ADCC function and increase the efficacy of therapeutic monoclonal antibodies (mAbs).Citation107,Citation108 On the other hand, it has also been reported that the cleavage of CD16 mediated by ADAM17 could increase the efficiency of NK cell response, since CD16 shedding favors NK cell detachment from the target cell, which in turn improves serial killing.Citation109
Regardless the mechanisms involved, it is of note that NK cells displaying a decreased surface expression of activating receptors have been detected in patients affected by different types of both solid and hematological cancers.Citation39,Citation96,Citation110–112
Dysregulation of tumor-NK cell interactions can also be achieved through the upregulation of receptors delivering inhibitory signals, i.e., receptors for immune checkpoints (IC), including PD-1, TIM3, TIGIT, and CD96 ().Citation113 Indeed, several studies demonstrated that in cancer patients these receptors can be upregulated in both PB-derived and tumor-infiltrating NK cells.Citation78–83 In addition, NK cells express a still unknown receptor able to recognize B7-H3, a member of the B7 family behaving as an immune checkpoint, given its broad expression in different tumor types and its ability to inhibit NK cell function following receptor ligation.Citation114 It has also to be considered that cancer cells can frequently display high levels of classical and non-classical HLA class I molecules, as a result of IFN-γ stimulation, further favoring the inhibitory signals conveyed to NK cells through inhibitory KIRs and CD94/NKG2A upon ligand engagement.Citation115,Citation116 In addition, IFN-γ is also responsible for the upregulation of PD-L1/-L2 expression on tumor cells via the JAK/STAT pathway.Citation117
Indeed, focusing on target cells, the alteration of ligands expressed on tumor cells is an effective strategy to limit recognition and killing by NK cells. Regarding this issue, ligands for NKG2D receptor represent a good paradigm, as their modulation from tumor cell surface can occur via different mechanisms. NKG2D ligands, namely MICA/B and ULPBs, are generally overexpressed or de novo expressed upon cellular stress, neoplastic transformation or viral infection.Citation104,Citation118,Citation119 Their expression can be diminished following the release of soluble ligands in exosomes, as a result of proteolytic shedding or translation of alternatively spliced transcripts. Interestingly, the inhibition of MICA/B cleavage can be obtained with a mAb that binds MICA/B in the α3 domain including the site of proteolytic shedding. This mAb has been shown to inhibit tumor growth in multiple fully immunocompetent mouse models and reduce human melanoma metastases in a humanized mouse model.Citation120 In addition, a Phase 1 dose-escalation clinical trial is showing promising results with a humanized anti-MICA/B mAb (cln-619), both as monotherapy and in combination with pembrolizumab in patients with solid tumors (NCT05117476). Alternatively, the disappearance of NKG2D ligands from the cell surface can be due to intracellular retention or to internalization and subsequent proteasomal degradation ().Citation121–125 Ligand expression can also be regulated at the transcriptional level; for example, tumor cells can overexpress several miRNAs targeting MICA.Citation126–130 Regarding DNAM-1 ligands, soluble poliovirus receptor (PVR or CD155) can be obtained by alternative splicing, while post-translational modifications affecting PVR or Nectin-2 (CD112) may induce intracellular retention and protein degradation.Citation106,Citation131–133 Although the knowledge of NCR ligands is still incomplete, several tumor escape strategies targeting molecules recognized by NCRs have been described so far. In particular, NKp30 ligands, represented by B7-H6 transmembrane molecule and by BAT3/BAG6 nuclear protein,Citation134,Citation135 can be released in soluble form or through exosomes;Citation136–138 in addition, B7-H6 can undergo proteolytic cleavage by ADAM-10 and -17 proteases.Citation139 On the other hand, NKp44-mediated NK cell activation can be impaired by tumor cells, thanks to the overexpression of proliferating cell nuclear antigen, delivering an inhibitory signal upon NKp44 recognition or through the release of Nidogen-1, an extracellular matrix protein most likely acting as a decoy ligand.Citation140,Citation141 Remarkably, soluble forms of different NK receptor ligands are under investigation as predictive biomarkers, since they can be detected in sera of tumor patients, and their levels are frequently associated with disease progression and poor prognosis.Citation136,Citation139,Citation142–144
A peculiar, and still poorly explored, mechanism by which the tumor can become more resistant to NK-mediated lysis involves cytoskeletal changes within cancer cells, deeply affecting the formation of the lytic immunological synapse. In this context, tumor escape from NK-mediated cytotoxicity driven by actin cytoskeleton remodeling has been clearly demonstrated in breast cancer.Citation145
Other tumor-associated intrinsic and extrinsic factors can induce an increased resistance to NK cells. For instance, it has been shown that NK cells can promote EMT in tumor cells, while EMT can favor the escape from NK cell attack.Citation93 In particular, lung cancer cells undergoing an intermediate EMT state have been recently shown to avoid NK cell attacks by both reducing chemokine production and inhibiting NK cell cytotoxic response.Citation146 In the case of pancreatic adenocarcinoma (PA), it has been demonstrated that PA cell lines deriving from primary or metastatic tumors display a different susceptibility to NK cells, with metastatic ones displaying EMT-related gene expression and phenotype and an increased resistance to NK cell-mediated lysis.Citation147
Therapeutic approaches exploiting NK cells
In spite of the many tumor-related mechanisms of immune suppression and escape, the generation of NK-based immunotherapeutic tools remains a fascinating and promising option for the research of new, more effective, treatments of malignancies.Citation148 However, the grade of NK cell infiltration into solid tumors is crucial when considering the use of NK cells for cancer immunotherapy, as NK cells generally have a low ability to infiltrate. Poor NK cell infiltration into the TME may be attributed to the interference with NK chemotactic signaling and activation, and to properties of the tumor bed (i.e., vasculature density and ECM structure).Citation73 Strategies to increase NK homing and infiltration into tumors would be essential to improve NK anti-tumor efficacy and prevent resistance and relapse. In these latter years, several groups have been conducting preclinical and clinical studies trying to optimize the anti-tumor effectiveness of NK cells over the barriers of the KIR/HLA-I and NKG2A/HLA-E inhibitory interactions, the suppressive and poorly accessible TME, and the limited persistency of NK cells after transfer. To overcome these limitations, several strategies are under study, including the evaluation of enhancers of NK cell activity (e.g., cytokines, IC blockers, and specific NK cell engagers), the generation of NK cells engineered to express specific chimeric activating receptors (i.e. CAR-NK cells), and the study of the most effective NK cell subset in hemopoietic stem cell transplantation (HSCT).
Cytokine-activated NK cells and CIML NK cells
Several cytokines have been evaluated to expand in vitro NK cells with enhanced anti-tumor properties and to sustain persistency and activation of adoptively transferred NK cells.Citation149
The first cytokine used was IL-2, which, however, has shown negative side effects at the bed side. Specifically, the induction of Tregs, the activation-induced cell death on NK cells, and the possible contribution of IL-2 to the vascular leak syndrome,Citation150–152 represented important issues. Moreover, the adoptive transfer of Lymphokine Activated Killer cells (LAK) (induced in vitro by IL-2 stimulation) showed limited efficacy.Citation153,Citation154 Therefore, the use of IL-2 in therapy required careful revision of doses and protocols, and stimulated the search for new activating means. In this context, engineered IL-2 molecules (superkines) have been developed to selectively enhance the therapeutic effects of IL-2. IL-2 superkines induced prolonged and intense NK cell activation, expansion of cytotoxic T cells, rather than Tregs, and improved anti-tumor responses in mouse models.Citation155–158 Another option to stimulate NK cells in therapeutic settings is represented by IL-15, which shares two of its receptor subunits with the IL-2 receptor complex, showing similar NK cell activation properties. At variance with IL-2, however, IL-15 does not stimulate Tregs and, for this reason, it has been considered a promising alternative to IL-2.Citation159 As this cytokine shows optimal effect when it is associated with the IL-15 Rα and trans-presented by IL-15 Rα+ cells,Citation160,Citation161 a super-agonistic molecular complex comprising the trans-presenting IL-15 Rα sushi domain and IgG1-Fc has been generated (N-803/ALT-803) and demonstrated to be effective in animal models and well tolerated in patients.Citation162–164 On the other hand, it has also been recently discussed how IL-15 or N-803 could be less effective than IL-2 in supporting the persistence of transferred allogeneic NK cells in AML patients, due to its propensity to activate host cytotoxic T lymphocytes (CTLs) and CTL-mediated rejection of transferred cells.Citation165,Citation166 It is also important to consider that long-term exposure to IL-15 can lead to NK cell dysfunction through metabolic and epigenetic reprogramming.Citation167,Citation168
Another interesting cytokine for NK cells is represented by IL-18, but the anti-tumor activity of this cytokine is limited by IL-18BP, a high-affinity IL-18 decoy receptor frequently upregulated in diverse human and mouse tumors. To maintain the signaling potential of IL-18, an IL-18 variant (‘decoy-resistant’ IL-18, DR-18) resistant to the inhibition mediated by IL-18BP has been developed. DR-18 has been shown to exert potent anti-tumor effects in mouse tumor models by enhancing the activity and maturation of NK cells, which can be exploited to effectively treat tumors that are resistant to anti-PD-1 therapy and have lost surface expression of MHC class I molecules.Citation169
The use of the IL-12/15/18 cytokine cocktail was initially studied, more than 10 y ago, for its ability to activate NK cells that could persist in vivo and recall the initial stimulus.Citation170,Citation171 Such, so-called cytokine-induced memory-like (CIML) NK cells have been extensively analyzed for their epigenetic signature and functional characteristics, and their potential therapeutic properties are under investigation for both hematologic and solid tumors.Citation172–175 In particular, in two recent studies, PB NK cells from haploidentical donors have been exposed for 12–16 h to the IL-12/15/18 cytokine cocktail and then transferred to AML patients directly after lymphodepletion or as supportive immunotherapy after donor-hematopoietic cell transplantation. In both cases, transferred cells have been demonstrated to proliferate and differentiate to memory-like phenotype in the patients and support anti-tumor activity.Citation176,Citation177
CAR-NK cells
Chimeric antigen receptor-engineered T cell (CAR-T) therapy has revolutionized the treatment of several types of cancer.Citation178 Despite this success, there are still major limitations and obstacles to the broad application of this therapy, including manufacturing complexity, high cost, and treatment-associated toxicities.Citation179 Furthermore, CAR-T cell therapy has good success in the treatment of hematological malignancies, but response rates are much lower in patients with solid tumors.Citation180
CAR-NK cell therapy is emerging as a promising alternative to modified T cells and can represent a simpler and cheaper “off-the-shelf” treatment compared to CAR-T cells. In addition, unlike CAR-T cells, CAR-NK cells do not cause cytokine release syndrome, immune effector cell-associated neurotoxicity syndrome and graft-versus-host disease (GvHD). They do not require HLA compatibility, are associated with minimal side-effects, can be produced on a large scale from several sources, can be applied in an allogeneic setting, and, different from what should be required for CAR-T, do not need gene editing to avoid TCR engagement. CAR-NK cell administration has been shown to be safe and effective for patients with multiple myeloma, AML, and CD19+ lymphoid tumors, thus supporting further investigation in a wide range of tumors.Citation181,Citation182
Many of the initial studies used NK cell lines, instead of NK cells, due to their superior proliferative capacity. Nevertheless, NK cell lines have significant limitations in their clinical applications.Citation183,Citation184 Indeed, since NK cell lines derive from lymphomas, they must be irradiated or inactivated before the infusion into the patient, thus limiting their in vivo persistence and the effectiveness of the therapy.Citation185 Thus, recent interesting alternative sources are represented by NK cells isolated from PB or differentiated from cord/PB CD34+ stem cells, CIML-NK cells, or induced pluripotent stem cell-derived NK cells.Citation186–191 Furthermore, several preclinical studies have been conducted to increase the clinical efficacy of CAR-NK cells through different approaches: i) prolonging the in vivo persistence by modifying CAR-NK cells to ectopically express cytokines such as IL-15; ii) promoting homing and trafficking to the tumor site (crucial point for the efficacy of cell therapy against solid tumors) by engineering cells to express peculiar chemokine receptors; iii) overcoming the immunosuppressive action of TGF-β in the TME (e.g., by deleting the TGF-β receptor) thanks to the use of inducible promoters, which become active upon recognition of a tumor-associated antigen, metabolite, or drug; and finally iv) metabolically optimizing NK cells. However, the long-term persistence of CAR-NK cells may not be necessary for a long-lasting anti-tumor response. Indeed, a standardized CAR-NK therapy that uses multiple infusions could be hypothesized to maintain enough circulating cells for the control of the ongoing disease.Citation182 Moreover, CAR-NK cells, in addition to CAR-mediated antigen recognition, take advantage of their innate killing capabilities and the possibility to trigger ADCC without further manipulation.Citation149,Citation181
NK cells in the context of hematopoietic stem cell transplantation
The capability of NK cells to recognize and eliminate transformed cells led to the investigation of the anti-tumor activity of these lymphocytes in the setting of HSCT. To take advantage of donor NK anti-leukemic effect (graft versus leukemia, GvL), the knowledge of the interactions between NK receptors and ligands expressed on tumor cells is crucial. The interaction between leukemic blasts and NK cells occurs in both directions. Indeed, malignant cells may impair their NK-mediated recognition by producing soluble ligands of NK activating receptors (e.g., MICA) or expressing ligands of inhibitory checkpoints, leading to NK cell exhaustion, as well as by modifying patient NK cell cytolytic capabilities by decreasing the expression levels of activating receptors (i.e., NCRs, NKG2D, and DNAM-1)Citation96,Citation111,Citation192–196 or increasing the expression of ICs (namely, TIGIT, PD-1, TIM3, and LAG3)Citation149,Citation197,Citation198 The relevance of NK/leukemic cell interactions is well documented in AML patients, in whom the anti-leukemic NKmediated activity was suppressed at diagnosis and relapse but restored when they achieved complete remission.Citation199 Moreover, many studies have demonstrated that early NK cell reconstitution following HSCT and higher NK cell numbers in the stem cell graft resulted in improved patient outcome by increasing the overall survival and reducing the incidence of relapse, GvHD, and viral post-transplant infections.Citation200
Being donor-derived NK cells significant effectors of anti-tumor and anti-infective responses in the recipients, the relevance of both NK receptor and ligand polymorphisms was investigated to design donor selection algorithms and stratify patients with worse post-transplant complications. One of the mechanisms involved in the GvL NK-mediated effect is driven by inhibitory KIRs. The impact of KIR/KIR-ligand mismatch was first examined in a cohort of adult AML patients receiving haploidentical HSCT (haplo-HSCT) with grafts composed of megadoses of highly purified CD34pos hematopoietic precursors. In this pioneering study, the authors reported a better outcome in the patients receiving a transplant from a donor characterized by an “alloreactive” NK subset.Citation201 NK alloreactivity occurs when the donor NK cells express only an inhibitory KIR recognizing a self-KIR ligand (thus allowing NK cell education), which is lacking in the patient (thus providing NK-mediated allorecognition).Citation202–204 Moreover, the alloreactive NK subset decreases the incidence of GvHD by reducing patient antigen-presenting cells and prevents graft rejection by eliminating host T cells.Citation201 More recently, novel methods of graft manipulations, based on ex vivo or in vivo T cell depletion (i.e., T cell-depleted and T cell-repleted graft, respectively) were developed, and the effects of KIR/KIR-ligand mismatch have been examined in different HSCT settings. ,Citation205–208
Evidence supporting the preferential selection of donors characterized by KIR B/x genotypes was produced for patients with AML or myeloid malignancies when unrelated donors are the stem cell source, leading to the KIR B content scoring model.Citation209–212 This algorithm stratifies the different KIR genotypes by analyzing the number of centromeric and telomeric regions containing B haplotype-defining genes. A positive effect of donor KIR haplotype B was also observed in pediatric haplo-HSCT.Citation207 More recently, the evaluation of donor KIR genotype in a cohort of transplanted children with high-risk acute lymphoblastic leukemia suggested that a different centromeric presence and telomeric absence of KIR B motifs were associated with reduced relapse risk.Citation213
Thus, several studies indicate that the donor KIR gene repertoire analysis may influence patient outcome, although with variable impacts among diverse HSCT platforms, suggesting that it should be introduced in donor selection searches when alternative donors are available. Many groups have attempted to apply an algorithm to quantify the complex interactions of activating and/or inhibitory KIR in the context of their ligands. Despite this, currently, there is no algorithm that can be applied to select the best donors in all haploidentical transplant platforms.Citation214 This lack is probably due to differences among transplantation settings, including graft manipulation approaches, conditioning regimens, and post-transplant immunosuppressive therapies. Nevertheless, KIR repertoire analysis and assignment of the presence of A and/or B haplotypes based on KIR gene content has been recently included in the British Society for Histocompatibility and Immunogenetics guideline among the donor selection in HSCT, especially for C2/C2 group recipients having worse outcome in all HSCT settings.Citation215
The post-transplant infusion of donor NK cells may result in an improved GvL effect. This advantage was demonstrated in the haplo-HSCT with post-transplant cyclophosphamide platform, in which the administration of high doses of in vitro expanded donor NK cells in early post-transplant time decreased the relapse rate, improving patient survival without any increase of GvHD.Citation216
Biologicals triggering NK cell-mediated tumor recognition
Tumor recognition by NK cells can be triggered through the use of mAbs targeting IC–IC ligand interactions or by engagers bridging NK cell receptors to tumor-expressed surface proteins.
Immunotherapy with blocking monoclonal antibodies
As mentioned above, NK cells express IC which may compromise their anti-tumor activity upon interaction with their ligands on tumor cells. Although studies have been primarily focused on IC+ T lymphocytes, also IC+ NK cells are frequently present in the TME, and may be drastically impaired in their anti-tumor activity.
A promising therapeutic approach to treat HLA-I+ solid tumors is represented by the use of monoclonal antibodies (mAbs) capable of disrupting the interactions between the HLA-specific inhibitory receptors (expressed on NK cells) and their ligands (expressed on tumor cells). In this context, the use of monalizumab, a first-in-class immune checkpoint inhibitor (ICI) targeting NKG2A and blocking the interaction between NKG2A and HLA-E, is achieving encouraging results in clinical studies in solid tumors, especially when combined with other checkpoint inhibitors, such as durvalumab (anti-PD-L1) in a variety of solid tumors (NCT02671435), or when used together with mAbs inducing ADCC, such as cetuximab (an EGFR-targeting antibody) in recurrent or metastatic head and neck squamous cell carcinoma (NCT04590963), and trastuzumab for HER2-positive breast cancer (NCT04307329). In non-small cell lung cancer (NSCLC), durvalumab plus monalizumab treatment has been associated with enhanced effector immune infiltration of tumors, interferon responses and markers of tertiary lymphoid structure formation, and systemic functional immune cell activation, as compared to durvalumab alone.Citation217
However, the recovery of NK cell function appears crucial, particularly in tumors that have lost (e.g., neuroblastoma) or express low levels of HLA-I molecules (e.g., melanoma). These tumors are “invisible” to classical αβ T cells, while they may be susceptible to NK cells. For this reason, we felt important to briefly discuss therapies based on the use of ICI.
In recent years, molecules that block the PD-1/PD-L1 pathway have been developed to re-activate the immune system’s response to cancer cells. This breakthrough led to the creation of several mAbs targeting PD-1 (like pembrolizumab and nivolumab) and PD-L1 (such as atezolizumab, avelumab, and durvalumab). These drugs have become foundational to contemporary cancer immunotherapy, serving as primary treatments for various tumor types and frequently outperforming traditional chemotherapy ().Citation218 Identifying patients most likely to benefit from anti-PD-1/PD-L1 therapies is challenging, as many patients exhibit no response to these treatments. This has spurred efforts to develop predictive biomarkers, with PD-L1 expression emerging as the most commonly employed indicator.Citation219,Citation220 PD-L1 expression, detected via immunohistochemistry on tumor or immune cells, is critical for therapy selection. The advent of various assays for measuring PD-L1 expression, each using distinct antibodies, has introduced complexity and inconsistencies in treatment eligibility determinations.Citation221
Figure 2. PD-L1 immunohistochemical expression in lung adenocarcinoma and contribution of NK cells in the anti-tumor response against HLA-Ineg tumor cells.
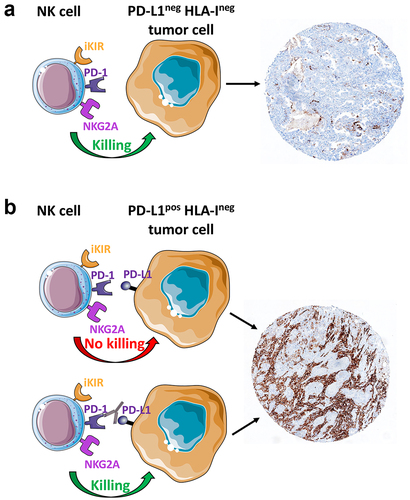
The FDA has designated several assays as companion diagnostics for the safe and effective application of their corresponding drugs. These include assays for atezolizumab in urothelial carcinoma and NSCLC, as well as for nivolumab in NSCLC and pembrolizumab across a range of solid tumors. Other assays are categorized as complementary diagnostics, helpful but not mandatory for drug administration.Citation222 The commercial availability of diverse assays for clinical decision-making highlights a significant challenge: integrating comprehensive testing into routine clinical practice. Efforts to harmonize results from different antibodies have shown some analytical performance similarity among certain assays, suggesting potential interchangeability.Citation223 However, discrepancies persist, particularly in identifying positive cases at clinically relevant cutoffs, emphasizing the complexity of PD-L1 testing and accurate biomarker assessment.Citation224,Citation225
To overcome resistance to immune checkpoint inhibitors (ICI), numerous trials are exploring combination treatment approaches, following the initial success of nivolumab and ipilimumab in melanoma and renal cell carcinoma.Citation226 Novel combination strategies are rapidly developing, with promising results from dual-checkpoint inhibition of PD-L1 and LAG-3 or TIGIT.Citation227 The potential for digital pathology and artificial intelligence to enhance patient selection for immunotherapy is promising.Citation228 These technologies could offer a more detailed evaluation of the immune context, potentially leading to robust predictive models. However, integrating such advanced methodologies into clinical practice requires substantial infrastructure updates and rigorous validation efforts.
NK cell engagers
The NK cell engagers are represented by engineered multivalent soluble molecules. Bi- or tri-specific killer engagers (BiKE or TriKE) consist of two associated single-chain fragment variables, targeting, respectively, a given tumor-specific antigen, and an activating NK receptor (usually CD16), and (in the case of TriKEs) an additional domain generally engaging cytokine receptors on NK cells. This field of research has received great impulse in these last years, and different platforms have been set up to generate ever more sophisticated molecules. These molecules, identified with different acronyms, depending on the originating lab and platform, are being evaluated in preclinical and clinical studies.Citation229,Citation230 Thus, for example, TriKEs combining IL-15 and anti-CD16 fragment and targeting HER2 or mesothelin have been recently tested against ovarian and lung cancer cells, respectively, and an anti-B7-H3 TriKE has been evaluated against different tumor cell types.Citation231 In this latter case, as B7-H3 is an inhibitory ligand for NK cells, theoretically, this engager has the double effect of targeting tumor cells and unleashing NK cell activity by the impairment of an inhibitory interaction. Other engagers, presently under study in phase I/II clinical trials, have been set up to simultaneously trigger two different activating receptors such as CD16 and NKp46, or CD16 and NKG2D, and targeting given tumor-associated antigens.Citation230,Citation232–234
Conclusions
There is no doubt that tumor immunotherapy has made major progress during the past 10 y. Two game-changing approaches have been the use of ICI and CAR-engineered immune effector cells (CAR-T and CAR-NK cells). However, there are still many unanswered questions for which we have major expectations of substantial improvements. For example, why can disruptive PD-1/PD-L1 interactions lead to a significant restoration of anti-tumor responses despite the presence of other mechanisms of immune evasion? An explanation might be the existence of still poorly explored connections among different inhibitory mechanisms operating at the tumor site. A better understanding of the prevalent mechanisms in a given tumor and their connections may lead to the design of combined strategies to improve responses to ICI. In spite of more recent development, CAR-NK cells may offer advantages over CAR-T, especially considering the additional difficulties in supporting effective anti-tumor activity in solid tumors. As discussed above, the tumor microenvironment may result hostile to any effector cells. First, tumor-specific surface antigens are extremely rare. Second, the homing of CAR-T cells to tumor tissues requires the release of appropriate chemotactic factors which attract effector cells at the tumor site. In addition, engineering and expanding autologous T cells (which may also be compromised) from individual patients is time-consuming, particularly expensive, and uncertain in terms of cell numbers obtained in each procedure. Therefore, it is not possible to establish reliable and consistent protocols. Importantly, NK cells do not cause GvHD and, thereby, can be obtained from allogeneic healthy donors and stored in large numbers. Thus, they represent ideal off-the-shelf products, immediately available for treating tumor patients. Indeed, major expectations are also based on CAR-NK cells because of their strong cytolytic activity and homing ability. Thus, obtaining good expansions of cytotoxic NK cells under good manufacturing practice conditions is an important issue to improve the use of NK cells in clinical practice, both as unmodified and genetically modified cells.
On the whole, the recent advances in the knowledge of NK cell biology and their manipulation are unveiling the considerable previously unknown therapeutic potential of these cells, which, we do believe, will be crucial for a future breakthrough in the fight against cancer.
Abbreviations
ADCC | = | Antibody-Dependent Cell-mediated Cytotoxicity |
AML | = | Acute Myeloid Leukemias |
BiKE | = | Bi-specific Killer Engagers |
BM | = | Bone Marrow |
CAF | = | Cancer-Associated Fibroblasts |
CAR | = | Chimeric Activating Receptor |
CIML | = | Cytokine-Induced Memory-Like |
DC | = | Dendritic Cells |
EMT | = | Epithelial-Mesenchymal Transition |
FDA | = | Food and Drug Administration |
GIST | = | Gastrointestinal Stromal Tumor |
GvHD | = | Graft versus Host Disease |
GvL | = | Graft versus Leukemia |
haplo-HSCT | = | Haploidentical HSCT |
HCC | = | Hepatocellular Carcinoma |
HLA | = | Human Leukocyte Antigen |
HLA-I | = | HLA class I |
HSCT | = | Hemopoietic Stem Cell Transplantation |
IC | = | Immune Checkpoints |
ICI | = | IC Inhibitors |
IDO | = | Indoleamine 2,3-Dioxygenase |
KIR | = | Killer Ig-like Receptor |
MMP | = | Matrix Metalloproteinases |
MIF | = | Migration Inhibitory Factor |
miRNA | = | microRNA |
MDSC | = | Myeloid-Derived Suppressor Cells |
NCRs | = | Natural Cytotoxicity Receptors |
NID1 | = | Nidogen-1 |
NSCLC | = | Non-Small Cell Lung Cancer |
NK | = | Natural Killer |
PA | = | Pancreatic Adenocarcinoma |
PB | = | Peripheral Blood |
PGE2 | = | Prostaglandin E2 |
PVR | = | Poliovirus Receptor |
TAM | = | Tumor-Associated Macrophages |
TAN | = | Tumor-Associated Neutrophils |
TGF-β | = | Transforming Growth Factor beta |
TME | = | Tumor Microenvironment |
Tregs | = | Regulatory T lymphocytes |
TriKE | = | Tri-specific Killer Engagers |
Acknowledgments
This Review is dedicated to the memory of Alessandro Moretta who sadly left us on February 17th 2018. His seminal discoveries of the HLA-I-specific inhibitory NK receptors and of the prototypes of the activating receptors responsible for tumor cell recognition and killing allowed to unravel the molecular mechanisms involved in NK cell function. These and many other Alex groundbreaking discoveries left a big footprint in Immunology and in Medicine. We greatly miss his scientific insight and, even more, his humanity and his unforgettable irony and smile.
Disclosure statement
No potential conflict of interest was reported by the author(s).
Additional information
Funding
References
- Binnewies M, Roberts EW, Kersten K, Chan V, Fearon DF, Merad M, Coussens LM, Gabrilovich DI, Ostrand-Rosenberg S, Hedrick CC. et al. Understanding the tumor immune microenvironment (TIME) for effective therapy. Nat Med. 2018;24(5):541–18. doi: 10.1038/s41591-018-0014-x.
- Ljunggren HG, Karre K. In search of the ‘missing self’: MHC molecules and NK cell recognition. Immunol Today. 1990;11:237–244. doi: 10.1016/0167-5699(90)90097-s.
- Moretta A, Bottino C, Vitale M, Pende D, Biassoni R, Mingari MC, Moretta L. Receptors for HLA class-I molecules in human natural killer cells. Annu Rev Immunol. 1996;14(1):619–648. doi: 10.1146/annurev.immunol.14.1.619.
- Long EO, Burshtyn DN, Clark WP, Peruzzi M, Rajagopalan S, Rojo S, Wagtmann N, Winter CC. Killer cell inhibitory receptors: diversity, specificity, and function. Immunol Rev. 1997;155(1):135–144. doi: 10.1111/j.1600-065x.1997.tb00946.x.
- Lanier LL. Natural killer cells: from no receptors to too many. Immunity. 1997;6(4):371–378. doi: 10.1016/s1074-7613(00)80280-0.
- Colonna M. Specificity and function of immunoglobulin superfamily NK cell inhibitory and stimulatory receptors. Immunol Rev. 1997;155(1):127–133. doi: 10.1111/j.1600-065x.1997.tb00945.x.
- Parham P. MHC class I molecules and KIRs in human history, health and survival. Nat Rev Immunol. 2005;5(3):201–214. doi: 10.1038/nri1570.
- Tomasello E, Blery M, Vely F, Vivier E. Signaling pathways engaged by NK cell receptors: double concerto for activating receptors, inhibitory receptors and NK cells. Semin Immunol. 2000;12(2):139–147. doi: 10.1006/smim.2000.0216.
- Moretta A, Bottino C, Vitale M, Pende D, Cantoni C, Mingari MC, Biassoni R, Moretta L. Activating receptors and coreceptors involved in human natural killer cell-mediated cytolysis. Annu Rev Immunol. 2001;19(1):197–223. doi: 10.1146/annurev.immunol.19.1.197.
- Lanier LL. On guard—activating NK cell receptors. Nat Immunol. 2001;2(1):23–27. doi: 10.1038/83130.
- Vivier E, Raulet DH, Moretta A, Caligiuri MA, Zitvogel L, Lanier LL, Yokoyama WM, Ugolini S. Innate or adaptive immunity? The example of natural killer cells. Science. 2011;331(6013):44–49. doi: 10.1126/science.1198687.
- Uhrberg M, Valiante NM, Shum BP, Shilling HG, Lienert-Weidenbach K, Corliss B, Tyan D, Lanier LL, Parham P. Human diversity in killer cell inhibitory receptor genes. Immunity. 1997;7(6):753–763. doi: 10.1016/s1074-7613(00)80394-5.
- Trowsdale J, Barten R, Haude A, Stewart CA, Beck S, Wilson MJ. The genomic context of natural killer receptor extended gene families. Immunol Rev. 2001;181(1):20–38. doi: 10.1034/j.1600-065x.2001.1810102.x.
- Vilches C, Parham P. KIR: diverse, rapidly evolving receptors of innate and adaptive immunity. Annu Rev Immunol. 2002;20(1):217–251. doi: 10.1146/annurev.immunol.20.092501.134942.
- Vitale M, Cantoni C, Della Chiesa M, Ferlazzo G, Carlomagno S, Pende D, Falco M, Pessino A, Muccio L, De Maria A. et al. An historical overview: the discovery of how NK cells can kill enemies, recruit defense troops, and more. Front Immunol. 2019;10:1415. doi: 10.3389/fimmu.2019.01415.
- Bjorkstrom NK, Ljunggren HG, Michaelsson J. Emerging insights into natural killer cells in human peripheral tissues. Nat Rev Immunol. 2016;16(5):310–320. doi: 10.1038/nri.2016.34.
- Freud AG, Mundy-Bosse BL, Yu J, Caligiuri MA. The broad spectrum of human natural killer cell diversity. Immunity. 2017;47(5):820–833. doi: 10.1016/j.immuni.2017.10.008.
- Ochoa MC, Minute L, Rodriguez I, Garasa S, Perez‐Ruiz E, Inogés S, Melero I, Berraondo P. Antibody-dependent cell cytotoxicity: immunotherapy strategies enhancing effector NK cells. Immunol Cell Biol. 2017;95(4):347–355. doi: 10.1038/icb.2017.6.
- Cerwenka A, Lanier LL. Natural killer cell memory in infection, inflammation and cancer. Nat Rev Immunol. 2016;16(2):112–123. doi: 10.1038/nri.2015.9.
- Lopez-Botet M, De Maria A, Muntasell A, Della Chiesa M, Vilches C. Adaptive NK cell response to human cytomegalovirus: Facts and open issues. Semin Immunol. 2023;65:101706. doi: 10.1016/j.smim.2022.101706.
- Tang F, Li J, Qi L, Liu D, Bo Y, Qin S, Miao Y, Yu K, Hou W, Li J. et al. A pan-cancer single-cell panorama of human natural killer cells. Cell. 2023;186(19):4235–4251 e4220. doi: 10.1016/j.cell.2023.07.034.
- Anfossi N, André P, Guia S, Falk CS, Roetynck S, Stewart CA, Breso V, Frassati C, Reviron D, Middleton D. et al. Human NK cell education by inhibitory receptors for MHC class I. Immunity. 2006;25(2):331–342. doi: 10.1016/j.immuni.2006.06.013.
- Orr MT, Lanier LL. Natural killer cell education and tolerance. Cell. 2010;142(6):847–856. doi: 10.1016/j.cell.2010.08.031.
- Boudreau JE, Hsu KC. Natural killer cell education in human health and disease. Curr Opin Immunol. 2018;50:102–111. doi: 10.1016/j.coi.2017.11.003.
- Vitale M, Cantoni C, Pietra G, Mingari MC, Moretta L. Effect of tumor cells and tumor microenvironment on NK-cell function. Eur J Immunol. 2014;44(6):1582–1592. doi: 10.1002/eji.201344272.
- Anderson NM, Simon MC. The tumor microenvironment. Curr Biol. 2020;30(16):R921–R925. doi: 10.1016/j.cub.2020.06.081.
- Balzasch BM, Cerwenka A. Microenvironmental signals shaping NK-cell reactivity in cancer. Eur J Immunol. 2023;53(12):e2250103. doi: 10.1002/eji.202250103.
- de Visser KE, Joyce JA. The evolving tumor microenvironment: From cancer initiation to metastatic outgrowth. Cancer Cell. 2023;41(3):374–403. doi: 10.1016/j.ccell.2023.02.016.
- Castriconi R, Carrega P, Dondero A, Bellora F, Casu B, Regis S, Ferlazzo G, Bottino C. Molecular mechanisms directing migration and retention of natural killer cells in human tissues. Front Immunol. 2018;9:2324. doi: 10.3389/fimmu.2018.02324.
- Cooper MA, Fehniger TA, Caligiuri MA. The biology of human natural killer-cell subsets. Trends Immunol. 2001;22(11):633–640. doi: 10.1016/s1471-4906(01)02060-9.
- Caligiuri MA. Human natural killer cells. Blood. 2008;112(3):461–469. doi: 10.1182/blood-2007-09-077438.
- Burke S, Lakshmikanth T, Colucci F, Carbone E. New views on natural killer cell-based immunotherapy for melanoma treatment. Trends Immunol. 2010;31(9):339–345. doi: 10.1016/j.it.2010.06.003.
- Lavergne E, Combadière B, Bonduelle O, Iga M, Gao J-L, Maho M, Boissonnas A, Murphy PM, Debré P, Combadière C. Fractalkine mediates natural killer-dependent antitumor responses in vivo. Cancer Res. 2003;63(21):7468–7474.
- Tsuchiyama T, Nakamoto Y, Sakai Y, Marukawa Y, Kitahara M, Mukaida N, Kaneko S. Prolonged, NK cell-mediated antitumor effects of suicide gene therapy combined with monocyte chemoattractant protein-1 against hepatocellular carcinoma. J Immunol. 2007;178(1):574–583. doi: 10.4049/jimmunol.178.1.574.
- Mamessier E, Sylvain A, Thibult M-L, Houvenaeghel G, Jacquemier J, Castellano R, Gonçalves A, André P, Romagné F, Thibault G. et al. Human breast cancer cells enhance self tolerance by promoting evasion from NK cell antitumor immunity. J Clin Invest. 2011;121(9):3609–3622. doi: 10.1172/JCI45816.
- Carrega P, Bonaccorsi I, Di Carlo E, Morandi B, Paul P, Rizzello V, Cipollone G, Navarra G, Mingari MC, Moretta L. et al. CD56(bright)perforin(low) noncytotoxic human NK cells are abundant in both healthy and neoplastic solid tissues and recirculate to secondary lymphoid organs via afferent lymph. J Immunol. 2014;192(8):3805–3815. doi: 10.4049/jimmunol.1301889.
- Portale F, Di Mitri D. NK cells in cancer: mechanisms of dysfunction and therapeutic potential. Int J Mol Sci. 2023;24(11):9521. doi: 10.3390/ijms24119521.
- Halama N, Braun M, Kahlert C, Spille A, Quack C, Rahbari N, Koch M, Weitz J, Kloor M, Zoernig I. et al. Natural killer cells are scarce in colorectal carcinoma tissue despite high levels of chemokines and cytokines. Clin Cancer Res. 2011;17(4):678–689. doi: 10.1158/1078-0432.CCR-10-2173.
- Platonova S, Cherfils-Vicini J, Damotte D, Crozet L, Vieillard V, Validire P, André P, Dieu-Nosjean M-C, Alifano M, Régnard J-F. et al. Profound coordinated alterations of intratumoral NK cell phenotype and function in lung carcinoma. Cancer Res. 2011;71(16):5412–5422. doi: 10.1158/0008-5472.CAN-10-4179.
- Ali TH, Pisanti S, Ciaglia E, Mortarini R, Anichini A, Garofalo C, Tallerico R, Santinami M, Gulletta E, Ietto C. et al. Enrichment of CD56(dim)KIR + CD57 + highly cytotoxic NK cells in tumour-infiltrated lymph nodes of melanoma patients. Nat Commun. 2014;5(1):5639. doi: 10.1038/ncomms6639.
- Pachynski RK, Zabel BA, Kohrt HE, Tejeda NM, Monnier J, Swanson CD, Holzer AK, Gentles AJ, Sperinde GV, Edalati A. et al. The chemoattractant chemerin suppresses melanoma by recruiting natural killer cell antitumor defenses. J Exp Med. 2012;209(8):1427–1435. doi: 10.1084/jem.20112124.
- Parodi M, Pedrazzi M, Cantoni C, Averna M, Patrone M, Cavaletto M, Spertino S, Pende D, Balsamo M, Pietra G. et al. Natural killer (Nk)/melanoma cell interaction induces NK-mediated release of chemotactic High Mobility Group Box-1 (HMGB1) capable of amplifying NK cell recruitment. Oncoimmunology. 2015;4(12):e1052353. doi: 10.1080/2162402X.2015.1052353.
- Bottcher JP, Bonavita E, Chakravarty P, Blees H, Cabeza-Cabrerizo M, Sammicheli S, Rogers NC, Sahai E, Zelenay S, Reis e Sousa C. NK cells stimulate recruitment of cDC1 into the tumor microenvironment promoting cancer immune control. Cell. 2018;172(5):1022–1037 e1014. doi: 10.1016/j.cell.2018.01.004.
- Barry KC, Hsu J, Broz ML, Cueto FJ, Binnewies M, Combes AJ, Nelson AE, Loo K, Kumar R, Rosenblum MD. et al. A natural killer–dendritic cell axis defines checkpoint therapy–responsive tumor microenvironments. Nat Med. 2018;24(8):1178–1191. doi: 10.1038/s41591-018-0085-8.
- Castriconi R, Dondero A, Bellora F, Moretta L, Castellano A, Locatelli F, Corrias MV, Moretta A, Bottino C. Neuroblastoma-derived TGF-β1 modulates the chemokine receptor repertoire of human resting NK cells. J Immunol. 2013;190(10):5321–5328. doi: 10.4049/jimmunol.1202693.
- Balkwill FR, Mantovani A. Cancer-related inflammation: common themes and therapeutic opportunities. Semin Cancer Biol. 2012;22(1):33–40. doi: 10.1016/j.semcancer.2011.12.005.
- Labani-Motlagh A, Ashja-Mahdavi M, Loskog A. The tumor microenvironment: a milieu hindering and obstructing antitumor immune responses. Front Immunol. 2020;11:940. doi: 10.3389/fimmu.2020.00940.
- Gabrilovich DI, Ostrand-Rosenberg S, Bronte V. Coordinated regulation of myeloid cells by tumours. Nat Rev Immunol. 2012;12(4):253–268. doi: 10.1038/nri3175.
- Bonavita E, Galdiero MR, Jaillon S, Mantovani A. Phagocytes as corrupted policemen in cancer-related Inflammation. Adv Cancer Res. 2015;128:141–171. doi: 10.1016/bs.acr.2015.04.013.
- Hedrick CC, Malanchi I. Neutrophils in cancer: heterogeneous and multifaceted. Nat Rev Immunol. 2022;22(3):173–187. doi: 10.1038/s41577-021-00571-6.
- Tumino N, Besi F, Martini S, Di Pace AL, Munari E, Quatrini L, Pelosi A, Fiore PF, Fiscon G, Paci P. et al. Polymorphonuclear myeloid-derived suppressor cells are abundant in peripheral blood of cancer patients and suppress natural killer cell anti-tumor activity. Front Immunol. 2022;12:803014. doi: 10.3389/fimmu.2021.803014.
- Sun R, Xiong Y, Liu H, Gao C, Su L, Weng J, Yuan X, Zhang D, Feng J. Tumor-associated neutrophils suppress antitumor immunity of NK cells through the PD-L1/PD-1 axis. Transl Oncol. 2020;13(10):100825. doi: 10.1016/j.tranon.2020.100825.
- Ghiringhelli F, Ménard C, Terme M, Flament C, Taieb J, Chaput N, Puig PE, Novault S, Escudier B, Vivier E. et al. CD4 + CD25 + regulatory T cells inhibit natural killer cell functions in a transforming growth factor–β–dependent manner. J Exp Med. 2005;202(8):1075–1085. doi: 10.1084/jem.20051511.
- Brownlie D, Scharenberg M, Mold JE, Hård J, Kekäläinen E, Buggert M, Nguyen S, Wilson JN, Al-Ameri M, Ljunggren H-G. et al. Expansions of adaptive-like NK cells with a tissue-resident phenotype in human lung and blood. Proc Natl Acad Sci USA. 2021;118(11). doi: 10.1073/pnas.2016580118.
- Rennert C, Tauber C, Fehrenbach P, Heim K, Bettinger D, Sogukpinar Ö, Schuch A, Zecher BF, Bengsch B, Lang SA. et al. Adaptive subsets limit the anti-tumoral NK-Cell activity in hepatocellular carcinoma. Cells. 2021;10(6):1369. doi: 10.3390/cells10061369.
- An Y, Liu F, Chen Y, Yang Q. Crosstalk between cancer-associated fibroblasts and immune cells in cancer. J Cell Mol Med. 2020;24(1):13–24. doi: 10.1111/jcmm.14745.
- Lavie D, Ben-Shmuel A, Erez N, Scherz-Shouval R. Cancer-associated fibroblasts in the single-cell era. Nat Cancer. 2022;3(7):793–807. doi: 10.1038/s43018-022-00411-z.
- Balsamo M, Scordamaglia F, Pietra G, Manzini C, Cantoni C, Boitano M, Queirolo P, Vermi W, Facchetti F, Moretta A. et al. Melanoma-associated fibroblasts modulate NK cell phenotype and antitumor cytotoxicity. Proc Natl Acad Sci USA. 2009;106(49):20847–20852. doi: 10.1073/pnas.0906481106.
- Li T, Yang Y, Hua X, Wang G, Liu W, Jia C, Tai Y, Zhang Q, Chen G. Hepatocellular carcinoma-associated fibroblasts trigger NK cell dysfunction via PGE2 and IDO. Cancer Lett. 2012;318(2):154–161. doi: 10.1016/j.canlet.2011.12.020.
- Li T, Yi S, Liu W, Jia C, Wang G, Hua X, Tai Y, Zhang Q, Chen G. Colorectal carcinoma-derived fibroblasts modulate natural killer cell phenotype and antitumor cytotoxicity. Med Oncol. 2013;30(3):663. doi: 10.1007/s12032-013-0663-z.
- Vasold J, Wagner M, Drolle H, Deniffel C, Kütt A, Oostendorp R, Sironi S, Rieger C, Fiegl M. The bone marrow microenvironment is a critical player in the NK cell response against acute myeloid leukaemia in vitro. Leuk Res. 2015;39(2):257–262. doi: 10.1016/j.leukres.2014.12.001.
- Shi M, Zhu J, Wang R, Chen X, Mi L, Walz T, Springer TA. Latent TGF-β structure and activation. Nature. 2011;474(7351):343–349. doi: 10.1038/nature10152.
- Travis MA, Sheppard D. TGF-β Activation and Function in Immunity. Annu Rev Immunol. 2014;32(1):51–82. doi: 10.1146/annurev-immunol-032713-120257.
- Pietra G, Manzini C, Rivara S, Vitale M, Cantoni C, Petretto A, Balsamo M, Conte R, Benelli R, Minghelli S. et al. Melanoma cells inhibit natural killer cell function by modulating the expression of activating receptors and cytolytic activity. Cancer Res. 2012;72(6):1407–1415. doi: 10.1158/0008-5472.CAN-11-2544.
- Hoskin DW, Mader JS, Furlong SJ, Conrad DM, Blay J. Inhibition of T cell and natural killer cell function by adenosine and its contribution to immune evasion by tumor cells (Review). Int J Oncol. 2008;32:527–535. doi: 10.3892/ijo.32.3.527.
- Castriconi R, Cantoni C, Della Chiesa M, Vitale M, Marcenaro E, Conte R, Biassoni R, Bottino C, Moretta L, Moretta A. Transforming growth factor β1 inhibits expression of NKp30 and NKG2D receptors: consequences for the NK-mediated killing of dendritic cells. Proc Natl Acad Sci USA. 2003;100(7):4120–4125. doi: 10.1073/pnas.0730640100.
- Marcenaro E, Chiesa MD, Bellora F, Parolini S, Millo R, Moretta L, Moretta A. IL-12 or IL-4 prime human NK cells to mediate functionally divergent interactions with dendritic cells or tumors. J Immunol. 2005;174(7):3992–3998. doi: 10.4049/jimmunol.174.7.3992.
- Krockenberger M, Dombrowski Y, Weidler C, Ossadnik M, Hönig A, Häusler S, Voigt H, Becker JC, Leng L, Steinle A. et al. Macrophage migration inhibitory factor contributes to the immune escape of ovarian cancer by down-regulating NKG2D. J Immunol. 2008;180(11):7338–7348. doi: 10.4049/jimmunol.180.11.7338.
- Gubbels JA, Felder M, Horibata S, Belisle JA, Kapur A, Holden H, Petrie S, Migneault M, Rancourt C, Connor JP. et al. MUC16 provides immune protection by inhibiting synapse formation between NK and ovarian tumor cells. Mol Cancer. 2010;9(1):11. doi: 10.1186/1476-4598-9-11.
- Crane CA, Han SJ, Barry JJ, Ahn BJ, Lanier LL, Parsa AT. TGF- downregulates the activating receptor NKG2D on NK cells and CD8+ T cells in glioma patients. Neuro Oncol. 2010;12(1):7–13. doi: 10.1093/neuonc/nop009.
- Hilpert J, Grosse-Hovest L, Grünebach F, Buechele C, Nuebling T, Raum T, Steinle A, Salih HR. Comprehensive analysis of NKG2D ligand expression and release in leukemia: implications for NKG2D-mediated NK cell responses. J Immunol. 2012;189(3):1360–1371. doi: 10.4049/jimmunol.1200796.
- Gill S, Vasey AE, De Souza A, Baker J, Smith AT, Kohrt HE, Florek M, Gibbs KD, Tate K, Ritchie DS. et al. Rapid development of exhaustion and down-regulation of eomesodermin limit the antitumor activity of adoptively transferred murine natural killer cells. Blood. 2012;119(24):5758–5768. doi: 10.1182/blood-2012-03-415364.
- Ben-Shmuel A, Biber G, Barda-Saad M. Unleashing natural killer cells in the tumor microenvironment-the next generation of immunotherapy? Front Immunol. 2020;11:275. doi: 10.3389/fimmu.2020.00275.
- O’Brien KL, Finlay DK. Immunometabolism and natural killer cell responses. Nat Rev Immunol. 2019;19(5):282–290. doi: 10.1038/s41577-019-0139-2.
- Yang Y, Chen L, Zheng B, Zhou S. Metabolic hallmarks of natural killer cells in the tumor microenvironment and implications in cancer immunotherapy. Oncogene. 2023;42(1):1–10. doi: 10.1038/s41388-022-02562-w.
- Cong J, Wang X, Zheng X, Wang D, Fu B, Sun R, Tian Z, Wei H. Dysfunction of natural killer cells by FBP1-induced inhibition of glycolysis during lung cancer progression. Cell Metab. 2018;28(2):243–255 e245. doi: 10.1016/j.cmet.2018.06.021.
- Benson DM Jr., Bakan CE, Mishra A, Hofmeister CC, Efebera Y, Becknell B, Baiocchi RA, Zhang J, Yu J, Smith MK. et al. The PD-1/PD-L1 axis modulates the natural killer cell versus multiple myeloma effect: a therapeutic target for CT-011, a novel monoclonal anti–PD-1 antibody. Blood. 2010;116(13):2286–2294. doi: 10.1182/blood-2010-02-271874.
- MacFarlane AWT, Jillab M, Plimack ER, Hudes GR, Uzzo RG, Litwin S, Dulaimi E, Al-Saleem T, Campbell KS. PD-1 expression on peripheral blood cells increases with stage in renal cell carcinoma patients and is rapidly reduced after surgical tumor resection. Cancer Immunol Res. 2014;2(4):320–331. doi: 10.1158/2326-6066.CIR-13-0133.
- da Silva IP, Gallois A, Jimenez-Baranda S, Khan S, Anderson AC, Kuchroo VK, Osman I, Bhardwaj N. Reversal of NK-cell exhaustion in advanced melanoma by Tim-3 blockade. Cancer Immunol Res. 2014;2(5):410–422. doi: 10.1158/2326-6066.CIR-13-0171.
- Pesce S, Greppi M, Tabellini G, Rampinelli F, Parolini S, Olive D, Moretta L, Moretta A, Marcenaro E. Identification of a subset of human natural killer cells expressing high levels of programmed death 1: A phenotypic and functional characterization. J Allergy Clin Immunol. 2017;139(1):335–346 e333. doi: 10.1016/j.jaci.2016.04.025.
- Zhang Q, Bi J, Zheng X, Chen Y, Wang H, Wu W, Wang Z, Wu Q, Peng H, Wei H. et al. Blockade of the checkpoint receptor TIGIT prevents NK cell exhaustion and elicits potent anti-tumor immunity. Nat Immunol. 2018;19(7):723–732. doi: 10.1038/s41590-018-0132-0.
- Khan M, Arooj S, Wang H. NK cell-based immune checkpoint Inhibition. Front Immunol. 2020;11:167. doi: 10.3389/fimmu.2020.00167.
- Alteber Z, Kotturi MF, Whelan S, Ganguly S, Weyl E, Pardoll DM, Hunter J, Ophir E. Therapeutic targeting of checkpoint receptors within the dnam1 axis. Cancer Discov. 2021;11(5):1040–1051. doi: 10.1158/2159-8290.CD-20-1248.
- Jia H, Yang H, Xiong H, Luo KQ. NK cell exhaustion in the tumor microenvironment. Front Immunol. 2023;14:1303605. doi: 10.3389/fimmu.2023.1303605.
- Wang Y, Chu J, Yi P, Dong W, Saultz J, Wang Y, Wang H, Scoville S, Zhang J, Wu L-C. et al. SMAD4 promotes TGF-β–independent NK cell homeostasis and maturation and antitumor immunity. J Clin Invest. 2018;128(11):5123–5136. doi: 10.1172/JCI121227.
- Xu D, Han Q, Hou Z, Zhang C, Zhang J. miR-146a negatively regulates NK cell functions via STAT1 signaling. Cell Mol Immunol. 2017;14:712–720. doi: 10.1038/cmi.2015.113.
- Pan C, Xiang L, Pan Z, Wang X, Li J, Zhuge L, Fang P, Xie Q, Hu X. MiR-544 promotes immune escape through downregulation of NCR1/NKp46 via targeting RUNX3 in liver cancer. Cancer Cell Int. 2018;18(1). doi: 10.1186/s12935-018-0542-y.
- Della Chiesa M, Carlomagno S, Frumento G, Balsamo M, Cantoni C, Conte R, Moretta L, Moretta A, Vitale M. The tryptophan catabolite L-kynurenine inhibits the surface expression of NKp46- and NKG2D-activating receptors and regulates NK-cell function. Blood. 2006;108(13):4118–4125. doi: 10.1182/blood-2006-03-006700.
- Wilson EB, El-Jawhari JJ, Neilson AL, Hall GD, Melcher AA, Meade JL, Cook GP. Human tumour immune evasion via TGF-β blocks NK cell activation but not survival allowing therapeutic restoration of anti-tumour activity. PLOS ONE. 2011;6(9):e22842. doi: 10.1371/journal.pone.0022842.
- Balsamo M, Manzini C, Pietra G, Raggi F, Blengio F, Mingari MC, Varesio L, Moretta L, Bosco MC, Vitale M. et al. Hypoxia downregulates the expression of activating receptors involved in NK-cell-mediated target cell killing without affecting ADCC. Eur J Immunol. 2013;43(10):2756–2764. doi: 10.1002/eji.201343448.
- Hasmim M, Messai Y, Ziani L, Thiery J, Bouhris J-H, Noman MZ, Chouaib S. Critical role of tumor microenvironment in shaping NK Cell Functions: implication of hypoxic stress. Front Immunol. 2015;6:482. doi: 10.3389/fimmu.2015.00482.
- Ni J, Wang X, Stojanovic A, Zhang Q, Wincher M, Bühler L, Arnold A, Correia MP, Winkler M, Koch P-S. et al. Single-cell RNA sequencing of tumor-infiltrating nk cells reveals that inhibition of transcription factor HIF-1α unleashes NK cell activity. Immunity. 2020;52(6):1075–1087 e1078. doi: 10.1016/j.immuni.2020.05.001.
- Huergo-Zapico L, Parodi M, Cantoni C, Lavarello C, Fernández-Martínez JL, Petretto A, DeAndrés-Galiana EJ, Balsamo M, López-Soto A, Pietra G. et al. NK-cell editing mediates epithelial-to-mesenchymal transition via phenotypic and proteomic changes in melanoma cell lines. Cancer Res. 2018;78(14):3913–3925. doi: 10.1158/0008-5472.CAN-17-1891.
- Groh V, Wu J, Yee C, Spies T. Tumour-derived soluble MIC ligands impair expression of NKG2D and T-cell activation. Nature. 2002;419(6908):734–738. doi: 10.1038/nature01112.
- Molfetta R, Quatrini L, Zitti B, Capuano C, Galandrini R, Santoni A, Paolini R. Regulation of NKG2D expression and signaling by endocytosis. Trends Immunol. 2016;37(11):790–802. doi: 10.1016/j.it.2016.08.015.
- Costello RT, Sivori S, Marcenaro E, Lafage-Pochitaloff M, Mozziconacci M-J, Reviron D, Gastaut J-A, Pende D, Olive D, Moretta A. Defective expression and function of natural killer cell–triggering receptors in patients with acute myeloid leukemia. Blood. 2002;99(10):3661–3667. doi: 10.1182/blood.v99.10.3661.
- Garcia-Iglesias T, Del Toro-Arreola A, Albarran-Somoza B, Del Toro-Arreola S, Sanchez-Hernandez PE, Ramirez- DueñDueñAs MG, Balderas-Peña LMA, Bravo-Cuellar A, Ortiz-Lazareno PC, Daneri-Navarro A. et al. Low NKp30, NKp46 and NKG2D expression and reduced cytotoxic activity on NK cells in cervical cancer and precursor lesions. BMC Cancer. 2009;9(1):186. doi: 10.1186/1471-2407-9-186.
- Carlsten M, Norell H, Bryceson YT, Poschke I, Schedvins K, Ljunggren H-G, Kiessling R, Malmberg K-J. Primary human tumor cells expressing CD155 impair tumor targeting by down-regulating DNAM-1 on NK cells. J Immunol. 2009;183(8):4921–4930. doi: 10.4049/jimmunol.0901226.
- Sanchez-Correa B, Gayoso I, Bergua JM, Casado JG, Morgado S, Solana R, Tarazona R. Decreased expression of DNAM-1 on NK cells from acute myeloid leukemia patients. Immunol Cell Biol. 2012;90(1):109–115. doi: 10.1038/icb.2011.15.
- Pesce S, Tabellini G, Cantoni C, Patrizi O, Coltrini D, Rampinelli F, Matta J, Vivier E, Moretta A, Parolini S. et al. B7-H6-mediated downregulation of NKp30 in NK cells contributes to ovarian carcinoma immune escape. Oncoimmunology. 2015;4(4):e1001224. doi: 10.1080/2162402X.2014.1001224.
- Xu Z, Zhang T, Zhuang R, Zhang Y, Jia W, Song C, Yang K, Yang A, Jin B. Increased levels of soluble CD226 in sera accompanied by decreased membrane CD226 expression on peripheral blood mononuclear cells from cancer patients. BMC Immunol. 2009;10(1):34. doi: 10.1186/1471-2172-10-34.
- Peruzzi G, Femnou L, Gil-Krzewska A, Borrego F, Weck J, Krzewski K, Coligan JE. Membrane-type 6 matrix metalloproteinase regulates the activation-induced downmodulation of CD16 in human primary NK cells. J Immunol. 2013;191(4):1883–1894. doi: 10.4049/jimmunol.1300313.
- Romee R, Foley B, Lenvik T, Wang Y, Zhang B, Ankarlo D, Luo X, Cooley S, Verneris M, Walcheck B. et al. NK cell CD16 surface expression and function is regulated by a disintegrin and metalloprotease-17 (ADAM17). Blood. 2013;121(18):3599–3608. doi: 10.1182/blood-2012-04-425397.
- Lanier LL. NKG2D receptor and its ligands in host defense. Cancer Immunol Res. 2015;3(6):575–582. doi: 10.1158/2326-6066.CIR-15-0098.
- Kanaya M, Shibuya K, Hirochika R, Kanemoto M, Ohashi K, Okada M, Wagatsuma Y, Cho Y, Kojima H, Teshima T. et al. Soluble DNAM-1, as a predictive biomarker for acute graft-versus-host disease. PLOS ONE. 2016;11(6):e0154173. doi: 10.1371/journal.pone.0154173.
- Molfetta R, Milito ND, Zitti B, Lecce M, Fionda C, Cippitelli M, Santoni A, Paolini R. The Ubiquitin-proteasome pathway regulates Nectin2/CD112 expression and impairs NK cell recognition and killing. Eur J Immunol. 2019;49(6):873–883. doi: 10.1002/eji.201847848.
- Zhou Q, Gil-Krzewska A, Peruzzi G, Borrego F. Matrix metalloproteinases inhibition promotes the polyfunctionality of human natural killer cells in therapeutic antibody-based anti-tumour immunotherapy. Clin Exp Immunol. 2013;173(1):131–139. doi: 10.1111/cei.12095.
- Pham DH, Kim, J.S., Kim, S.K., Shin, D.J., Hyun, H., Yoon, M.S., Kang, S.J., Ryu, Y.J., Cho, J.S., Yoon, J.H., Lee, J.S. Effects of ADAM10 and ADAM17 inhibitors on natural killer cell expansion and antibody-dependent cellular cytotoxicity against breast cancer cells in vitro. Anticancer Res. 2017;37:5507–5513. doi: 10.21873/anticanres.11981.
- Srpan K, Ambrose A, Karampatzakis A, Saeed M, Cartwright ANR, Guldevall K, De Matos GDSC, Önfelt B, Davis DM. Shedding of CD16 disassembles the NK cell immune synapse and boosts serial engagement of target cells. J Cell Biol. 2018;217(9):3267–3283. doi: 10.1083/jcb.201712085.
- Rocca YS, Roberti MP, Arriaga JM, Amat M, Bruno L, Pampena MB, Huertas E, Loria FS, Pairola A, Bianchini M. et al. Altered phenotype in peripheral blood and tumor-associated NK cells from colorectal cancer patients. Innate Immun. 2013;19(1):76–85. doi: 10.1177/1753425912453187.
- Fauriat C, Just-Landi S, Mallet F, Arnoulet C, Sainty D, Olive D, Costello RT. Deficient expression of NCR in NK cells from acute myeloid leukemia: evolution during leukemia treatment and impact of leukemia cells in NCRdull phenotype induction. Blood. 2007;109(1):323–330. doi: 10.1182/blood-2005-08-027979.
- Han B, Mao F-Y, Zhao Y-L, Lv Y-P, Teng Y-S, Duan M, Chen W, Cheng P, Wang T-T, Liang Z-Y. et al. Altered NKp30, NKp46, NKG2D, and DNAM-1 expression on circulating nk cells is associated with tumor progression in human gastric cancer. J Immunol Res. 2018;2018:1–9. doi: 10.1155/2018/6248590.
- Dougall WC, Kurtulus S, Smyth MJ, Anderson AC. TIGIT and CD96: new checkpoint receptor targets for cancer immunotherapy. Immunol Rev. 2017;276(1):112–120. doi: 10.1111/imr.12518.
- Castriconi R, Dondero A, Augugliaro R, Cantoni C, Carnemolla B, Sementa AR, Negri F, Conte R, Corrias MV, Moretta L. et al. Identification of 4Ig-B7-H3 as a neuroblastoma-associated molecule that exerts a protective role from an NK cell-mediated lysis. Proc Natl Acad Sci USA. 2004;101(34):12640–12645. doi: 10.1073/pnas.0405025101.
- Malmberg KJ, Levitsky V, Norell H, Matos CTD, Carlsten M, Schedvins K, Rabbani H, Moretta A, Söderström K, Levitskaya J. et al. IFN-γ protects short-term ovarian carcinoma cell lines from CTL lysis via a CD94/NKG2A-dependent mechanism. J Clin Invest. 2002;110(10):1515–1523. doi: 10.1172/JCI15564.
- Balsamo M, Vermi W, Parodi M, Pietra G, Manzini C, Queirolo P, Lonardi S, Augugliaro R, Moretta A, Facchetti F. et al. Melanoma cells become resistant to NK-cell-mediated killing when exposed to NK-cell numbers compatible with NK-cell infiltration in the tumor. Eur J Immunol. 2012;42(7):1833–1842. doi: 10.1002/eji.201142179.
- Bellucci R, Martin A, Bommarito D, Wang K, Hansen SH, Freeman GJ, Ritz J. Interferon-γ-induced activation of JAK1 and JAK2 suppresses tumor cell susceptibility to NK cells through upregulation of PD-L1 expression. Oncoimmunology. 2015;4(6):e1008824. doi: 10.1080/2162402X.2015.1008824.
- Raulet DH, Gasser S, Gowen BG, Deng W, Jung H. Regulation of ligands for the NKG2D activating receptor. Annu Rev Immunol. 2013;31(1):413–441. doi: 10.1146/annurev-immunol-032712-095951.
- El-Gazzar A, Groh V, Spies T. Immunobiology and conflicting roles of the human NKG2D lymphocyte receptor and its ligands in cancer. J Immunol. 2013;191(4):1509–1515. doi: 10.4049/jimmunol.1301071.
- Ferrari de Andrade L, Tay RE, Pan D, Luoma AM, Ito Y, Badrinath S, Tsoucas D, Franz B, May KF, Harvey CJ. et al. Antibody-mediated inhibition of MICA and MICB shedding promotes NK cell–driven tumor immunity. Science. 2018;359(6383):1537–1542. doi: 10.1126/science.aao0505.
- Waldhauer I, Steinle A. Proteolytic release of soluble UL16-binding protein 2 from tumor cells. Cancer Res. 2006;66(5):2520–2526. doi: 10.1158/0008-5472.CAN-05-2520.
- Fuertes MB, Girart MV, Molinero LL, Domaica CI, Rossi LE, Barrio MM, Mordoh J, Rabinovich GA, Zwirner NW. Intracellular retention of the NKG2D ligand MHC class I chain-related gene a in human melanomas confers immune privilege and prevents NK cell-mediated cytotoxicity. J Immunol. 2008;180(7):4606–4614. doi: 10.4049/jimmunol.180.7.4606.
- Waldhauer I, Goehlsdorf D, Gieseke F, Weinschenk T, Wittenbrink M, Ludwig A, Stevanovic S, Rammensee H-G, Steinle A. Tumor-associated MICA is shed by ADAM proteases. Cancer Res. 2008;68(15):6368–6376. doi: 10.1158/0008-5472.CAN-07-6768.
- Fernandez-Messina L, Reyburn HT, Vales-Gomez M. A short half-life of ULBP1 at the cell surface due to internalization and proteosomal degradation. Immunol Cell Biol. 2016;94(5):479–485. doi: 10.1038/icb.2016.2.
- Labani-Motlagh A, Israelsson P, Ottander U, Lundin E, Nagaev I, Nagaeva O, Dehlin E, Baranov V, Mincheva-Nilsson L. Differential expression of ligands for NKG2D and DNAM-1 receptors by epithelial ovarian cancer-derived exosomes and its influence on NK cell cytotoxicity. Tumour Biol. 2016;37(4):5455–5466. doi: 10.1007/s13277-015-4313-2.
- Stern-Ginossar N, Gur C, Biton M, Horwitz E, Elboim M, Stanietsky N, Mandelboim M, Mandelboim O. Human microRNAs regulate stress-induced immune responses mediated by the receptor NKG2D. Nat Immunol. 2008;9(9):1065–1073. doi: 10.1038/ni.1642.
- Xie J, Liu M, Li Y, Nie Y, Mi Q, Zhao S. Ovarian tumor-associated microRNA-20a decreases natural killer cell cytotoxicity by downregulating MICA/B expression. Cell Mol Immunol. 2014;11(5):495–502. doi: 10.1038/cmi.2014.30.
- Trinh TL, Kandell WM, Donatelli SS, Tu N, Tejera MM, Gilvary DL, Eksioglu EA, Burnette A, Adams WA, Liu J. et al. Immune evasion by TGFβ-induced miR-183 repression of MICA/B expression in human lung tumor cells. Oncoimmunology. 2019;8(4):e1557372. doi: 10.1080/2162402X.2018.1557372.
- Al-Abdallah A, Jahanbani I, Mehdawi H, Ali RH, Al-Brahim N, Mojiminiyi O, Junaid TA. Down-regulation of the human major histocompatibility complex class I chain-related gene a (MICA) and its receptor is mediated by microRNA-146b-5p and is a potential mechanism of immunoediting in papillary thyroid carcinoma. Exp Mol Pathol. 2020;113:104379. doi: 10.1016/j.yexmp.2020.104379.
- Pesce S, Greppi M, Ferretti E, Obino V, Carlomagno S, Rutigliani M, Thoren FB, Sivori S, Castagnola P, Candiani S. et al. miRNAs in NK cell-based immune responses and cancer immunotherapy. Front Cell Dev Biol. 2020;8:119. doi: 10.3389/fcell.2020.00119.
- Baury B, Masson D, McDermott BM, Jarry A, Blottière HM, Blanchardie P, Laboisse CL, Lustenberger P, Racaniello VR, Denis MG. Identification of secreted CD155 isoforms. Biochem Biophys Res Commun. 2003;309(1):175–182. doi: 10.1016/s0006-291x(03)01560-2.
- Zitti B, Molfetta R, Fionda C, Quatrini L, Stabile H, Lecce M, de Turris V, Ricciardi MR, Petrucci MT, Cippitelli M. et al. Innate immune activating ligand SUMOylation affects tumor cell recognition by NK cells. Sci Rep. 2017;7(1):10445. doi: 10.1038/s41598-017-10403-0.
- Okumura G, Iguchi-Manaka A, Murata R, Yamashita-Kanemaru Y, Shibuya A, Shibuya K. Tumor-derived soluble CD155 inhibits DNAM-1–mediated antitumor activity of natural killer cells. J Exp Med. 2020;217(4):1. doi: 10.1084/jem.20191290.
- Pogge von Strandmann E, Simhadri VR, von Tresckow B, Sasse S, Reiners K, Hansen HP, Rothe A, Böll B, Simhadri VL, Borchmann P. et al. Human leukocyte antigen-B-associated transcript 3 is released from tumor cells and engages the NKp30 receptor on natural killer cells. Immunity. 2007;27(6):965–974. doi: 10.1016/j.immuni.2007.10.010.
- Brandt CS, Baratin M, Yi EC, Kennedy J, Gao Z, Fox B, Haldeman B, Ostrander CD, Kaifu T, Chabannon C. et al. The B7 family member B7-H6 is a tumor cell ligand for the activating natural killer cell receptor NKp30 in humans. J Exp Med. 2009;206(7):1495–1503. doi: 10.1084/jem.20090681.
- Reiners KS, Topolar D, Henke A, Simhadri VR, Kessler J, Sauer M, Bessler M, Hansen HP, Tawadros S, Herling M. et al. Soluble ligands for NK cell receptors promote evasion of chronic lymphocytic leukemia cells from NK cell anti-tumor activity. Blood. 2013;121(18):3658–3665. doi: 10.1182/blood-2013-01-476606.
- Matta J, Baratin M, Chiche L, Forel J-M, Cognet C, Thomas G, Farnarier C, Piperoglou C, Papazian L, Chaussabel D. et al. Induction of B7-H6, a ligand for the natural killer cell–activating receptor NKp30, in inflammatory conditions. Blood. 2013;122(3):394–404. doi: 10.1182/blood-2013-01-481705.
- Dassler-Plenker J, Reiners KS, van den Boorn JG, Hansen HP, Putschli B, Barnert S, Schuberth-Wagner C, Schubert R, Tüting T, Hallek M. et al. RIG-I activation induces the release of extracellular vesicles with antitumor activity. Oncoimmunology. 2016;5(10):e1219827. doi: 10.1080/2162402X.2016.1219827.
- Schlecker E, Fiegler N, Arnold A, Altevogt P, Rose-John S, Moldenhauer G, Sucker A, Paschen A, von Strandmann EP, Textor S. et al. Metalloprotease-mediated tumor cell shedding of B7-H6, the ligand of the natural killer cell–activating receptor NKp30. Cancer Res. 2014;74(13):3429–3440. doi: 10.1158/0008-5472.CAN-13-3017.
- Rosental B, Brusilovsky M, Hadad U, Oz D, Appel MY, Afergan F, Yossef R, Rosenberg LA, Aharoni A, Cerwenka A. et al. Proliferating cell nuclear antigen is a novel inhibitory ligand for the natural cytotoxicity receptor NKp44. J Immunol. 2011;187(11):5693–5702. doi: 10.4049/jimmunol.1102267.
- Gaggero S, Bruschi M, Petretto A, Parodi M, Zotto GD, Lavarello C, Prato C, Santucci L, Barbuto A, Bottino C. et al. Nidogen-1 is a novel extracellular ligand for the NKp44 activating receptor. Oncoimmunology. 2018;7(9):e1470730. doi: 10.1080/2162402X.2018.1470730.
- Iguchi-Manaka A, Okumura G, Kojima H, Cho Y, Hirochika R, Bando H, Sato T, Yoshikawa H, Hara H, Shibuya A. et al. Increased soluble CD155 in the serum of cancer patients. PLOS ONE. 2016;11(4):e0152982. doi: 10.1371/journal.pone.0152982.
- Willumsen N, Bager CL, Leeming DJ, Bay-Jensen AC, Karsdal MA. Nidogen-1 degraded by cathepsin s can be quantified in serum and is associated with non–small cell lung cancer. Neoplasia. 2017;19(4):271–278. doi: 10.1016/j.neo.2017.01.008.
- Xing S, Ferrari de Andrade L. NKG2D and MICA/B shedding: a ‘tag game’ between NK cells and malignant cells. Clin Transl Immunol. 2020;9(12):e1230. doi: 10.1002/cti2.1230.
- Al Absi A, Wurzer H, Guerin C, Hoffmann C, Moreau F, Mao X, Brown-Clay J, Petrolli R, Casellas CP, Dieterle M. et al. Actin cytoskeleton remodeling drives breast cancer cell escape from natural killer–mediated cytotoxicity. Cancer Res. 2018;78(19):5631–5643. doi: 10.1158/0008-5472.CAN-18-0441.
- Parodi M, Centonze G, Murianni F, Orecchia P, Andriani F, Roato I, Gardelli C, Balsamo M, Moro M, Taiè G. et al. Hybrid epithelial-mesenchymal status of lung cancer dictates metastatic success through differential interaction with NK cells. J Immunother Cancer. 2024;12(3):e007895. doi: 10.1136/jitc-2023-007895.
- Fiore PF, Di Pace AL, Conti LA, Tumino N, Besi F, Scaglione S, Munari E, Moretta L, Vacca P. Different effects of NK cells and NK-derived soluble factors on cell lines derived from primary or metastatic pancreatic cancers. Cancer Immunol Immunother. 2023;72(6):1417–1428. doi: 10.1007/s00262-022-03340-z.
- Vivier E, Rebuffet L, Narni-Mancinelli E, Cornen S, Igarashi RY, Fantin VR. Natural killer cell therapies. Nature. 2024;626(8000):727–736. doi: 10.1038/s41586-023-06945-1.
- Sivori S, Pende D, Quatrini L, Pietra G, Della Chiesa M, Vacca P, Tumino N, Moretta F, Mingari MC, Locatelli F. et al. NK cells and ILCs in tumor immunotherapy. Mol Aspects Med. 2021;80:100870. doi: 10.1016/j.mam.2020.100870.
- Ahmadzadeh M, Rosenberg SA. IL-2 administration increases CD4+ CD25(hi) Foxp3+ regulatory T cells in cancer patients. Blood. 2006;107(6):2409–2414. doi: 10.1182/blood-2005-06-2399.
- Wei S, Kryczek I, Edwards RP, Zou L, Szeliga W, Banerjee M, Cost M, Cheng P, Chang A, Redman B. et al. Interleukin-2 administration alters the CD4+FOXP3+ T-cell pool and tumor trafficking in patients with ovarian carcinoma. Cancer Res. 2007;67(15):7487–7494. doi: 10.1158/0008-5472.CAN-07-0565.
- Mitra S, Leonard WJ. Biology of IL-2 and its therapeutic modulation: Mechanisms and strategies. J Leukoc Biol. 2018;103(4):643–655. doi: 10.1002/JLB.2RI0717-278R.
- Law TM, Motzer, R.J., Mazumdar, M., Sell, K.W., Walther, P., O’Connell, M., Khan, A., Vlamis, V., Vogelzang, N.J., Bajorin, D.F. Phase III randomized trial of interleukin-2 with or without lymphokine-activated killer cells in the treatment of patients with advanced renal cell carcinoma. Cancer. 1995;76:824–832. doi: 10.1002/1097-0142(19950901)76:5<824:aid-cncr2820760517>3.0.co;2-n.
- Parkhurst MR, Riley JP, Dudley ME, Rosenberg SA. Adoptive transfer of autologous natural killer cells leads to high levels of circulating natural killer cells but does not mediate tumor regression. Clin Cancer Res. 2011;17(19):6287–6297. doi: 10.1158/1078-0432.CCR-11-1347.
- Levin AM, Bates DL, Ring AM, Krieg C, Lin JT, Su L, Moraga I, Raeber ME, Bowman GR, Novick P. et al. Exploiting a natural conformational switch to engineer an interleukin-2 ‘superkine’. Nature. 2012;484(7395):529–533. doi: 10.1038/nature10975.
- Merchant R, Galligan C, Munegowda MA, Pearce LB, Lloyd P, Smith P, Merchant F, To MD. Fine-tuned long-acting interleukin-2 superkine potentiates durable immune responses in mice and non-human primate. J Immunother Cancer. 2022;10(1):e003155. doi: 10.1136/jitc-2021-003155.
- Wolf NK, Blaj C, Picton LK, Snyder G, Zhang L, Nicolai CJ, Ndubaku CO, McWhirter SM, Garcia KC, Raulet DH. Synergy of a STING agonist and an IL-2 superkine in cancer immunotherapy against MHC I–deficient and MHC I + tumors. Proc Natl Acad Sci USA. 2022;119(22):e2200568119. doi: 10.1073/pnas.2200568119.
- Gaggero S, Martinez-Fabregas J, Cozzani A, Fyfe PK, Leprohon M, Yang J, Thomasen FE, Winkelmann H, Magnez R, Conti AG. et al. IL-2 is inactivated by the acidic pH environment of tumors enabling engineering of a pH-selective mutein. Sci Immunol. 2022;7(78):eade5686. doi: 10.1126/sciimmunol.ade5686.
- Zhou Y, Husman T, Cen X, Tsao T, Brown J, Bajpai A, Li M, Zhou K, Yang L. Interleukin 15 in Cell-Based Cancer Immunotherapy. Int J Mol Sci. 2022;23(13):7311. doi: 10.3390/ijms23137311.
- Ferlazzo G, Pack M, Thomas D, Paludan C, Schmid D, Strowig T, Bougras G, Muller WA, Moretta L, Münz C. Distinct roles of IL-12 and IL-15 in human natural killer cell activation by dendritic cells from secondary lymphoid organs. Proc Natl Acad Sci USA. 2004;101(47):16606–16611. doi: 10.1073/pnas.0407522101.
- Anton OM, Peterson ME, Hollander MJ, Dorward DW, Arora G, Traba J, Rajagopalan S, Snapp EL, Garcia KC, Waldmann TA. et al. Trans -endocytosis of intact IL-15Rα–IL-15 complex from presenting cells into NK cells favors signaling for proliferation. Proc Natl Acad Sci USA. 2020;117(1):522–531. doi: 10.1073/pnas.1911678117.
- Han KP, Zhu X, Liu B, Jeng E, Kong L, Yovandich JL, Vyas VV, Marcus WD, Chavaillaz P-A, Romero CA. et al. IL-15: IL-15 receptor alpha superagonist complex: high-level co-expression in recombinant mammalian cells, purification and characterization. Cytokine. 2011;56(3):804–810. doi: 10.1016/j.cyto.2011.09.028.
- Felices M, Chu S, Kodal B, Bendzick L, Ryan C, Lenvik AJ, Boylan KLM, Wong HC, Skubitz APN, Miller JS. et al. IL-15 super-agonist (ALT-803) enhances natural killer (NK) cell function against ovarian cancer. Gynecol Oncol. 2017;145(3):453–461. doi: 10.1016/j.ygyno.2017.02.028.
- Van der Meer JMR, Maas RJA, Guldevall K, Klarenaar K, de Jonge PKJD, Evert JSHV, van der Waart AB, Cany J, Safrit JT, Lee JH. et al. IL-15 superagonist N-803 improves IFNγ production and killing of leukemia and ovarian cancer cells by CD34+ progenitor-derived NK cells. Cancer Immunol Immunother. 2021;70(5):1305–1321. doi: 10.1007/s00262-020-02749-8.
- Berrien-Elliott MM, Becker-Hapak M, Cashen AF, Jacobs M, Wong P, Foster M, McClain E, Desai S, Pence P, Cooley S. et al. Systemic IL-15 promotes allogeneic cell rejection in patients treated with natural killer cell adoptive therapy. Blood. 2022;139(8):1177–1183. doi: 10.1182/blood.2021011532.
- Pende D, Meazza R. N-803: a double-edged sword in haplo-NK therapy. Blood. 2022;139(8):1122–1124. doi: 10.1182/blood.2021014789.
- Felices M, Lenvik AJ, McElmurry R, Chu S, Hinderlie P, Bendzick L, Geller MA, Tolar J, Blazar BR, Miller JS. et al. Continuous treatment with IL-15 exhausts human NK cells via a metabolic defect. JCI Insight. 2018;3(3). doi: 10.1172/jci.insight.96219.
- Merino A, Zhang B, Dougherty P, Luo X, Wang J, Blazar BR, Miller JS, Cichocki F. Chronic stimulation drives human NK cell dysfunction and epigenetic reprograming. J Clin Invest. 2019;129(9):3770–3785. doi: 10.1172/JCI125916.
- Zhou T, Damsky W, Weizman O-E, McGeary MK, Hartmann KP, Rosen CE, Fischer S, Jackson R, Flavell RA, Wang J. et al. IL-18BP is a secreted immune checkpoint and barrier to IL-18 immunotherapy. Nature. 2020;583(7817):609–614. doi: 10.1038/s41586-020-2422-6.
- Cooper MA, Elliott JM, Keyel PA, Yang L, Carrero JA, Yokoyama WM. Cytokine-induced memory-like natural killer cells. Proc Natl Acad Sci USA. 2009;106(6):1915–1919. doi: 10.1073/pnas.0813192106.
- Ni J, Miller M, Stojanovic A, Garbi N, Cerwenka A. Sustained effector function of IL-12/15/18–preactivated NK cells against established tumors. J Exp Med. 2012;209(13):2351–2365. doi: 10.1084/jem.20120944.
- Romee R, Rosario M, Berrien-Elliott MM, Wagner JA, Jewell BA, Schappe T, Leong JW, Abdel-Latif S, Schneider SE, Willey S. et al. Cytokine-induced memory-like natural killer cells exhibit enhanced responses against myeloid leukemia. Sci Transl Med. 2016;8(357):357ra123. doi: 10.1126/scitranslmed.aaf2341.
- Uppendahl LD, Felices M, Bendzick L, Ryan C, Kodal B, Hinderlie P, Boylan KLM, Skubitz APN, Miller JS, Geller MA. Cytokine-induced memory-like natural killer cells have enhanced function, proliferation, and in vivo expansion against ovarian cancer cells. Gynecol Oncol. 2019;153(1):149–157. doi: 10.1016/j.ygyno.2019.01.006.
- Marin ND, Krasnick BA, Becker-Hapak M, Conant L, Goedegebuure SP, Berrien-Elliott MM, Robbins KJ, Foltz JA, Foster M, Wong P. et al. Memory-like differentiation enhances nk cell responses to melanoma. Clin Cancer Res. 2021;27(17):4859–4869. doi: 10.1158/1078-0432.CCR-21-0851.
- Jacobs MT, Wong P, Zhou AY, Becker-Hapak M, Marin ND, Marsala L, Foster M, Foltz JA, Cubitt CC, Tran J. et al. Memory-like differentiation, tumor-targeting mabs, and chimeric antigen receptors enhance natural killer cell responses to head and neck cancer. Clin Cancer Res. 2023;29(20):4196–4208. doi: 10.1158/1078-0432.CCR-23-0156.
- Berrien-Elliott MM, Cashen AF, Cubitt CC, Neal CC, Wong P, Wagner JA, Foster M, Schappe T, Desai S, McClain E. et al. Multidimensional analyses of donor memory-like nk cells reveal new associations with response after adoptive immunotherapy for leukemia. Cancer Discov. 2020;10(12):1854–1871. doi: 10.1158/2159-8290.CD-20-0312.
- Berrien-Elliott MM, Foltz JA, Russler-Germain DA, Neal CC, Tran J, Gang M, Wong P, Fisk B, Cubitt CC, Marin ND. et al. Hematopoietic cell transplantation donor-derived memory-like NK cells functionally persist after transfer into patients with leukemia. Sci Transl Med. 2022;14(633):eabm1375. doi: 10.1126/scitranslmed.abm1375.
- Cappell KM, Kochenderfer JN. Long-term outcomes following CAR T cell therapy: what we know so far. Nat Rev Clin Oncol. 2023;20(6):359–371. doi: 10.1038/s41571-023-00754-1.
- Grigor EJM, Fergusson D, Kekre N, Montroy J, Atkins H, Seftel MD, Daugaard M, Presseau J, Thavorn K, Hutton B. et al. Risks and benefits of chimeric antigen receptor T-Cell (CAR-T) therapy in cancer: a systematic review and meta-analysis. Transfus Med Rev. 2019;33(2):98–110. doi: 10.1016/j.tmrv.2019.01.005.
- Miao L, Zhang Z, Ren Z, Tang F, Li Y. Obstacles and Coping Strategies of CAR-T Cell Immunotherapy in Solid Tumors. Front Immunol. 2021;12:687822. doi: 10.3389/fimmu.2021.687822.
- Rafei H, Daher M, Rezvani K. Chimeric antigen receptor (CAR) natural killer (NK)-cell therapy: leveraging the power of innate immunity. Br J Haematol. 2021;193(2):216–230. doi: 10.1111/bjh.17186.
- Moscarelli J, Zahavi D, Maynard R, Weiner LM. The next generation of cellular immunotherapy: chimeric antigen receptor-natural killer cells. Transpl Cell Ther. 2022;28(10):650–656. doi: 10.1016/j.jtct.2022.06.025.
- Tonn T, Schwabe D, Klingemann HG, Becker S, Esser R, Koehl U, Suttorp M, Seifried E, Ottmann OG, Bug G. et al. Treatment of patients with advanced cancer with the natural killer cell line NK-92. Cytotherapy. 2013;15(12):1563–1570. doi: 10.1016/j.jcyt.2013.06.017.
- Zhang C, Oberoi P, Oelsner S, Waldmann A, Lindner A, Tonn T, Wels WS. Chimeric antigen receptor-engineered nk-92 cells: an off-the-shelf cellular therapeutic for targeted elimination of cancer cells and induction of protective antitumor immunity. Front Immunol. 2017;8:533. doi: 10.3389/fimmu.2017.00533.
- Raftery MJ, Franzén AS, Pecher G. CAR NK Cells: The Future is Now. Annu Rev Cancer Biol. 2023;7(1):229–246. doi: 10.1146/annurev-cancerbio-061521-082320.
- Imai C, Iwamoto S, Campana D. Genetic modification of primary natural killer cells overcomes inhibitory signals and induces specific killing of leukemic cells. Blood. 2005;106(1):376–383. doi: 10.1182/blood-2004-12-4797.
- Li Y, Hermanson DL, Moriarity BS, Kaufman DS. Human iPSC-derived natural killer cells engineered with chimeric antigen receptors enhance anti-tumor activity. Cell STEM Cell. 2018;23(2):181–192 e185. doi: 10.1016/j.stem.2018.06.002.
- Quintarelli C, Sivori S, Caruso S, Carlomagno S, Falco M, Boffa I, Orlando D, Guercio M, Abbaszadeh Z, Sinibaldi M. et al. Efficacy of third-party chimeric antigen receptor modified peripheral blood natural killer cells for adoptive cell therapy of B-cell precursor acute lymphoblastic leukemia. Leukemia. 2020;34(4):1102–1115. doi: 10.1038/s41375-019-0613-7.
- Liu E, Marin D, Banerjee P, Macapinlac HA, Thompson P, Basar R, Nassif Kerbauy L, Overman B, Thall P, Kaplan M. et al. Use of CAR-Transduced Natural Killer Cells in CD19-Positive Lymphoid Tumors. N Engl J Med. 2020;382(6):545–553. doi: 10.1056/NEJMoa1910607.
- Gang M, Marin ND, Wong P, Neal CC, Marsala L, Foster M, Schappe T, Meng W, Tran J, Schaettler M. et al. CAR-modified memory-like NK cells exhibit potent responses to NK-resistant lymphomas. Blood. 2020;136(20):2308–2318. doi: 10.1182/blood.2020006619.
- Marin D, Li Y, Basar R, Rafei H, Daher M, Dou J, Mohanty V, Dede M, Nieto Y, Uprety N. et al. Safety, efficacy and determinants of response of allogeneic CD19-specific CAR-NK cells in CD19(+) B cell tumors: a phase 1/2 trial. Nat Med. 2024;30(3):772–784. doi: 10.1038/s41591-023-02785-8.
- Salih HR, Antropius H, Gieseke F, Lutz SZ, Kanz L, Rammensee H-G, Steinle A. Functional expression and release of ligands for the activating immunoreceptor NKG2D in leukemia. Blood. 2003;102(4):1389–1396. doi: 10.1182/blood-2003-01-0019.
- Carbone E, Neri, P., Mesuraca, M., Fulciniti, M.T., Otsuki, T., Pende, D., Groh, V., Spies, T., Pollio, G., Cosman, D., Catalano, L. HLA class I, NKG2D, and natural cytotoxicity receptors regulate multiple myeloma cell recognition by natural killer cells. Blood. 2005;105(1):251–258. doi: 10.1182/blood-2004-04-1422.
- von Lilienfeld-Toal M, Frank S, Leyendecker C, Feyler S, Jarmin S, Morgan R, Glasmacher A, Märten A, Schmidt-Wolf IGH, Brossart P. et al. Reduced immune effector cell NKG2D expression and increased levels of soluble NKG2D ligands in multiple myeloma may not be causally linked. Cancer Immunol Immunother. 2010;59(6):829–839. doi: 10.1007/s00262-009-0807-3.
- Mastaglio S, Wong E, Perera T, Ripley J, Blombery P, Smyth MJ, Koldej R, Ritchie D. Natural killer receptor ligand expression on acute myeloid leukemia impacts survival and relapse after chemotherapy. Blood Adv. 2018;2(4):335–346. doi: 10.1182/bloodadvances.2017015230.
- Vago L, Gojo I. Immune escape and immunotherapy of acute myeloid leukemia. J Clin Invest. 2020;130(4):1552–1564. doi: 10.1172/JCI129204.
- Sanchez-Correa B, Lopez-Sejas N, Duran E, Labella F, Alonso C, Solana R, Tarazona R. Modulation of NK cells with checkpoint inhibitors in the context of cancer immunotherapy. Cancer Immunol Immunother. 2019;68(5):861–870. doi: 10.1007/s00262-019-02336-6.
- Allison M, Mathews J, Gilliland T, Mathew SO. Natural Killer Cell-Mediated Immunotherapy for Leukemia. Cancers (Basel). 2022;14(3):843. doi: 10.3390/cancers14030843.
- Khaznadar Z, Boissel N, Agaugué S, Henry G, Cheok M, Vignon M, Geromin D, Cayuela J-M, Castaigne S, Pautas C. et al. Defective NK cells in acute myeloid leukemia patients at diagnosis are associated with blast transcriptional signatures of immune evasion. J Immunol. 2015;195(6):2580–2590. doi: 10.4049/jimmunol.1500262.
- Minculescu L, Fischer-Nielsen A, Haastrup E, Ryder LP, Andersen NS, Schjoedt I, Friis LS, Kornblit BT, Petersen SL, Sengelov H. et al. Improved relapse-free survival in patients with high natural killer cell doses in grafts and during early immune reconstitution after allogeneic stem cell transplantation. Front Immunol. 2020;11:1068. doi: 10.3389/fimmu.2020.01068.
- Ruggeri L, Capanni M, Urbani E, Perruccio K, Shlomchik WD, Tosti A, Posati S, Rogaia D, Frassoni F, Aversa F. et al. Effectiveness of donor natural killer cell alloreactivity in mismatched hematopoietic transplants. Science. 2002;295(5562):2097–2100. doi: 10.1126/science.1068440.
- Pende D, Marcenaro S, Falco M, Martini S, Bernardo ME, Montagna D, Romeo E, Cognet C, Martinetti M, Maccario R. et al. Anti-leukemia activity of alloreactive NK cells in KIR ligand-mismatched haploidentical HSCT for pediatric patients: evaluation of the functional role of activating KIR and redefinition of inhibitory KIR specificity. Blood. 2009;113(13):3119–3129. doi: 10.1182/blood-2008-06-164103.
- Moretta L, Locatelli F, Pende D, Marcenaro E, Mingari MC, Moretta A. Killer Ig–like receptor-mediated control of natural killer cell alloreactivity in haploidentical hematopoietic stem cell transplantation. Blood. 2011;117(3):764–771. doi: 10.1182/blood-2010-08-264085.
- Locatelli F, Pende D, Falco M, Della Chiesa M, Moretta A, Moretta L. NK cells mediate a crucial graft-versus-leukemia effect in haploidentical-HSCT to cure high-risk acute leukemia. Trends Immunol. 2018;39(7):577–590. doi: 10.1016/j.it.2018.04.009.
- Luznik L, O’Donnell PV, Symons HJ, Chen AR, Leffell MS, Zahurak M, Gooley TA, Piantadosi S, Kaup M, Ambinder RF. et al. HLA-haploidentical bone marrow transplantation for hematologic malignancies using nonmyeloablative conditioning and high-dose, posttransplantation cyclophosphamide. Biol Blood Marrow Transpl. 2008;14(6):641–650. doi: 10.1016/j.bbmt.2008.03.005.
- Bertaina A, Merli P, Rutella S, Pagliara D, Bernardo ME, Masetti R, Pende D, Falco M, Handgretinger R, Moretta F. et al. HLA-haploidentical stem cell transplantation after removal of αβ+ T and B cells in children with nonmalignant disorders. Blood. 2014;124(5):822–826. doi: 10.1182/blood-2014-03-563817.
- Oevermann L, Michaelis SU, Mezger M, Lang P, Toporski J, Bertaina A, Zecca M, Moretta L, Locatelli F, Handgretinger R. et al. KIR B haplotype donors confer a reduced risk for relapse after haploidentical transplantation in children with ALL. Blood. 2014;124(17):2744–2747. doi: 10.1182/blood-2014-03-565069.
- Merli P, Algeri M, Galaverna F, Bertaina V, Lucarelli B, Boccieri E, Becilli M, Quagliarella F, Rosignoli C, Biagini S. et al. TCRαβ/CD19 cell–depleted HLA-haploidentical transplantation to treat pediatric acute leukemia: updated final analysis. Blood. 2024;143(3):279–289. doi: 10.1182/blood.2023021336.
- Cooley S, Weisdorf DJ, Guethlein LA, Klein JP, Wang T, Le CT, Marsh SGE, Geraghty D, Spellman S, Haagenson MD. et al. Donor selection for natural killer cell receptor genes leads to superior survival after unrelated transplantation for acute myelogenous leukemia. Blood. 2010;116(14):2411–2419. doi: 10.1182/blood-2010-05-283051.
- Cooley S, Weisdorf DJ, Guethlein LA, Klein JP, Wang T, Marsh SGE, Spellman S, Haagenson MD, Saeturn K, Ladner M. et al. Donor killer cell Ig-like receptor B haplotypes, recipient HLA-C1, and HLA-C mismatch enhance the clinical benefit of unrelated transplantation for acute myelogenous leukemia. J Immunol. 2014;192(10):4592–4600. doi: 10.4049/jimmunol.1302517.
- Weisdorf D, Cooley S, Wang T, Trachtenberg E, Vierra-Green C, Spellman S, Sees JA, Spahn A, Vogel J, Fehniger TA. et al. KIR B donors improve the outcome for AML patients given reduced intensity conditioning and unrelated donor transplantation. Blood Adv. 2020;4(4):740–754. doi: 10.1182/bloodadvances.2019001053.
- Guethlein LA, Beyzaie N, Nemat-Gorgani N, Wang T, Ramesh V, Marin WM, Hollenbach JA, Schetelig J, Spellman SR, Marsh SGE. et al. Following transplantation for acute myelogenous leukemia, donor kir cen b02 better protects against relapse than KIR cen B01. J Immunol. 2021;206(12):3064–3072. doi: 10.4049/jimmunol.2100119.
- Babor F, Peters C, Manser AR, Glogova E, Sauer M, Pötschger U, Ahlmann M, Cario G, Feuchtinger T, Gruhn B. et al. Presence of centromeric but absence of telomeric group B KIR haplotypes in stem cell donors improve leukaemia control after HSCT for childhood ALL. Bone Marrow Transpl. 2019;54(11):1847–1858. doi: 10.1038/s41409-019-0543-z.
- Ciurea SO, Al Malki MM, Kongtim P, Fuchs EJ, Luznik L, Huang X-J, Ciceri F, Locatelli F, Aversa F, Castagna L. et al. The European society for blood and marrow transplantation (EBMT) consensus recommendations for donor selection in haploidentical hematopoietic cell transplantation. Bone Marrow Transpl. 2020;55(1):12–24. doi: 10.1038/s41409-019-0499-z.
- Little AM, Akbarzad‐Yousefi A, Anand A, Diaz Burlinson N, Dunn PPJ, Evseeva I, Latham K, Poulton K, Railton D, Vivers S. et al. BSHI guideline: HLA matching and donor selection for haematopoietic progenitor cell transplantation. Int J Immunogenet. 2021;48(2):75–109. doi: 10.1111/iji.12527.
- Ciurea SO, Kongtim P, Soebbing D, Trikha P, Behbehani G, Rondon G, Olson A, Bashir Q, Gulbis AM, Indreshpal K. et al. Decrease post-transplant relapse using donor-derived expanded NK-cells. Leukemia. 2022;36(1):155–164. doi: 10.1038/s41375-021-01349-4.
- Cascone T, Kar G, Spicer JD, García-Campelo R, Weder W, Daniel DB, Spigel DR, Hussein M, Mazieres J, Oliveira J. et al. Neoadjuvant durvalumab alone or combined with novel immuno-oncology agents in resectable lung cancer: the phase II NeoCOAST platform trial. Cancer Discov. 2023;13(11):2394–2411. doi: 10.1158/2159-8290.CD-23-0436.
- Sukari A, Nagasaka M, Al-Hadidi A, Lum LG. Cancer immunology and immunotherapy. Anticancer Res. 2016;36(11):5593–5606. doi: 10.21873/anticanres.11144.
- Brahmer JR, Govindan R, Anders RA, Antonia SJ, Sagorsky S, Davies MJ, Dubinett SM, Ferris A, Gandhi L, Garon EB. et al. The society for immunotherapy of cancer consensus statement on immunotherapy for the treatment of non-small cell lung cancer (NSCLC). J Immunother Cancer. 2018;6(1):75. doi: 10.1186/s40425-018-0382-2.
- Garon EB, Hellmann MD, Rizvi NA, Carcereny E, Leighl NB, Ahn M-J, Eder JP, Balmanoukian AS, Aggarwal C, Horn L. et al. Five-year overall survival for patients with advanced non‒small-cell lung cancer treated with pembrolizumab: results from the phase I KEYNOTE-001 Study. J Clin Oncol. 2019;37(28):2518–2527. doi: 10.1200/JCO.19.00934.
- Marletta S, Fusco N, Munari E, Luchini C, Cimadamore A, Brunelli M, Querzoli G, Martini M, Vigliar E, Colombari R. et al. Atlas of PD-L1 for Pathologists: Indications, Scores, Diagnostic Platforms and Reporting Systems. J Pers Med. 2022;12(7):1073. doi: 10.3390/jpm12071073.
- Doroshow DB, Bhalla S, Beasley MB, Sholl LM, Kerr KM, Gnjatic S, Wistuba II, Rimm DL, Tsao MS, Hirsch FR. et al. PD-L1 as a biomarker of response to immune-checkpoint inhibitors. Nat Rev Clin Oncol. 2021;18(6):345–362. doi: 10.1038/s41571-021-00473-5.
- Torlakovic E, Lim HJ, Adam J, Barnes P, Bigras G, Chan AWH, Cheung CC, Chung J-H, Couture C, Fiset PO. et al. “Interchangeability” of PD-L1 immunohistochemistry assays: a meta-analysis of diagnostic accuracy. Mod Pathol. 2020;33(1):4–17. doi: 10.1038/s41379-019-0327-4.
- Munari E, Rossi G, Zamboni G, Lunardi G, Marconi M, Sommaggio M, Netto GJ, Hoque MO, Brunelli M, Martignoni G. et al. PD-L1 Assays 22C3 and SP263 are not interchangeable in non–small cell lung cancer when considering clinically relevant cutoffs. Am J Surg Pathol. 2018;42(10):1384–1389. doi: 10.1097/PAS.0000000000001105.
- Munari E, Querzoli G, Brunelli M, Marconi M, Sommaggio M, Cocchi MA, Martignoni G, Netto GJ, Caliò A, Quatrini L. et al. Comparison of three validated PD-L1 immunohistochemical assays in urothelial carcinoma of the bladder: interchangeability and issues related to patient selection. Front Immunol. 2022;13:954910. doi: 10.3389/fimmu.2022.954910.
- Lu L, Zhan M, Li X-Y, Zhang H, Dauphars DJ, Jiang J, Yin H, Li S-Y, Luo S, Li Y. et al. Clinically approved combination immunotherapy: Current status, limitations, and future perspective. Curr Res Immunol. 2022;3:118–127. doi: 10.1016/j.crimmu.2022.05.003.
- Walsh RJ, Sundar R, Lim JSJ. Immune checkpoint inhibitor combinations—current and emerging strategies. Br J Cancer. 2023;128(8):1415–1417. doi: 10.1038/s41416-023-02181-6.
- Baxi V, Lee G, Duan C, Pandya D, Cohen DN, Edwards R, Chang H, Li J, Elliott H, Pokkalla H. et al. Association of artificial intelligence-powered and manual quantification of programmed death-ligand 1 (PD-L1) expression with outcomes in patients treated with nivolumab ± ipilimumab. Mod Pathol. 2022;35(11):1529–1539. doi: 10.1038/s41379-022-01119-2.
- Myers JA, Miller JS. Exploring the NK cell platform for cancer immunotherapy. Nat Rev Clin Oncol. 2021;18(2):85–100. doi: 10.1038/s41571-020-0426-7.
- Fenis A, Demaria O, Gauthier L, Vivier E, Narni-Mancinelli E. New immune cell engagers for cancer immunotherapy. Nat Rev Immunol. 2024;24(7):471–486. doi: 10.1038/s41577-023-00982-7.
- Vallera DA, Ferrone S, Kodal B, Hinderlie P, Bendzick L, Ettestad B, Hallstrom C, Zorko NA, Rao A, Fujioka N. et al. NK-Cell-Mediated Targeting of Various Solid Tumors Using a B7-H3 Tri-Specific Killer Engager in vitro and in vivo. Cancers (Basel). 2020;12(9):2659. doi: 10.3390/cancers12092659.
- Colomar-Carando N, Gauthier L, Merli P, Loiacono F, Canevali P, Falco M, Galaverna F, Rossi B, Bosco F, Caratini M. et al. Exploiting natural killer cell engagers to control pediatric b-cell precursor acute lymphoblastic leukemia. Cancer Immunol Res. 2022;10(3):291–302. doi: 10.1158/2326-6066.CIR-21-0843.
- Demaria O, Gauthier L, Vetizou M, Blanchard Alvarez A, Vagne C, Habif G, Batista L, Baron W, Belaïd N, Girard-Madoux M. et al. Antitumor immunity induced by antibody-based natural killer cell engager therapeutics armed with not-alpha IL-2 variant. Cell Rep Med. 2022;3(10):100783. doi: 10.1016/j.xcrm.2022.100783.
- Gauthier L, Virone-Oddos A, Beninga J, Rossi B, Nicolazzi C, Amara C, Blanchard-Alvarez A, Gourdin N, Courta J, Basset A. et al. Control of acute myeloid leukemia by a trifunctional NKp46-CD16a-NK cell engager targeting CD123. Nat Biotechnol. 2023;41(9):1296–1306. doi: 10.1038/s41587-022-01626-2.