ABSTRACT
Tumor-derived exosomes bind to organ resident cells, activating S100 molecules during the remodeling of the local immune microenvironment. However, little is known regarding how organ resident cell S100A10 mediates cancer metastatic progression. Here, we provided evidence that S100A10 plays an important role in regulating the lung immune microenvironment and cancer metastasis. S100A10-deficient mice reduced cancer metastasis in the lung. Furthermore, the activation of S100A10 within lung fibroblasts via tumor-derived exosomes increased the expression of CXCL1 and CXCL8 chemokines, accompanied by the myeloid-derived suppressor cells (MDSCs) recruitment. S100A10 inhibitors such as 1-Substituted-4-Aroyl-3-hydroxy-5-Phenyl-1 H-5-pyrrol-2(5 H)-ones inhibit lung metastasis in vivo. Our findings highlight the crucial role of S100A10 in driving MDSC recruitment in order to remodel the lung immune microenvironment and provide potential therapeutic targets to block cancer metastasis to the lung.
Introduction
Cancer metastasis is the primary cause of death in cancer patients. Within this local microenvironment of distant organs, immune cells and fibroblasts are known to regulate immune suppression vital for primary cancer cell metastasis.Citation1 Primary tumor-derived secreted molecular (TDSFs) and extracellular vesicles (EVs) induce bone marrow-derived cells (BMDCs) to accumulate in metastatic organs.Citation2,Citation3 These BMDCs interact with resident stromal cells and the extracellular matrix of distinct organs to promote the formation of an immunosuppressive microenvironment.Citation4 The interaction mechanisms between primary tumors and distinct organs that initiate metastasis are still unclear and require more in-depth study.
Different BMDC types have demonstrated the ability to remodel metastatic organ environment. Studies have also shown that myeloid-derived suppressor cells (MDSCs) play an important role in cancer metastasis.Citation5 MDSCs can be recruited into the local immune microenvironment by chemokines, including CCL2, CXCL1, CXCL2, CXCL5, CXCL8 and CCL26Citation6. MDSCs affect the immune microenvironment and extracellular matrix (ECM) during cancer metastasis.Citation5–7 However, how host stromal cells in distinct organs trigger MDSCs recruitment to remodel the local immune microenvironment are unclear. Fibroblasts are one of the most abundant stromal cell populations in distant tissue. In primary tumors, fibroblasts enhance tumor invasion via ECM remodeling, promoting cancer-associated inflammation and supporting immune cell activation.Citation8,Citation9 Additionally, whether fibroblasts could promote cancer metastasis by modifying the local immune microenvironment in distant organs still needs to be studied.
S100A10, a plasminogen receptor, activates the plasminogen pathway by binding to the cell surface receptor annexin 2.Citation10 In cancer cells, upregulation of S100A10 has been found to increase plasmin, promote ECM degradation, and enhance cell growth and migration.Citation11,Citation12 Furthermore, S100A10 has been reported to affect the trafficking of membrane proteins, including the serotonin 5-HT1B receptor,Citation13 channel proteins TRPV5/TRPV6Citation14 and sodium channel protein Nav1.8.Citation15 S100A10-Annexin2 heterotetramer also mediates the stemness and chemoresistance of breast cancer. Paclitaxel increases the expression of S100A10, forming a complex with the histone demethylase KDM6A and the histone chaperone SPT6. This large complex is recruited to the OCT4 binding sites, facilitating the transcription of stem cell marker genes.Citation16 Recent studies have shown that tumor-derived exosomes bind organ stromal cells and activate Src and S100 molecules in distant tissue microenvironments.Citation17 However, the effect of S100A10 in resident stromal cells on tumor progression remains unclear. Here, we focus on how resident stromal cell S100A10 contributes to cancer metastasis and the remodeling of the local immune microenvironment. In this study, we constructed conventional S100A10 knockout mice to study the effects of lung resident cell S100A10 on the remodeling of the local immune microenvironment and cancer metastasis in vivo. We found that S100A10 in lung fibroblasts activates chemokine expression and promotes MDSC recruitment within the lung immune microenvironment, thereby facilitating cancer metastasis.
Materials and methods
Mice models
Conventional C57BL/6 S100a10 knockout mice were obtained from the Saiye Model Animal Research Center. They were constructed using CRISPR/CAS9 techniques. The Genotyping Strategy is shown in Supplement . All animal experiments were carried out according to a protocol approved by the Ethics Committee and the Institutional Animal Care and Use Committee of Xi’an Jiaotong University. The animal ethical approval number is XJTU1AF2024LSK-2023-091. In our study, all mice were of the same sex and age (range between 6 and 10 weeks).
Figure 1. S100A10 deficiency prevents lung metastasis.
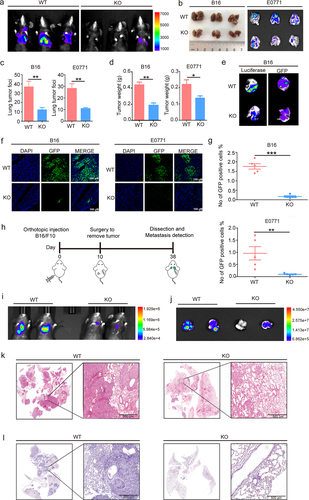
Orthotopic and tail-vein tumor models
Luciferase or GFP-labeled B16/F10 melanoma cells (5 × 105) were administered intradermally in the flank of the mice. At 10 d, the tumor-bearing mice underwent surgical excision and continuous cultivation. Twenty-eight days after surgery, lung metastasis of the orthotopical tumor was monitored via bioluminescent imaging.
The B16/F10LUC/GFP (2 × 105) and E0771GFP (5 × 105) injections were resuspended in PBS and injected into the tail-vein of C57BL/6 mice. At 12 d, the mice were sacrificed under anaesthetic, and tumor metastatic nodules in the lungs were evaluated via bioluminescent and fluorescent imaging, flow cytometry analysis and IHC/IF staining.
Adeno-associated virus 6 lung infection
Adeno-associated virus 6 encoding S100A10 (AAV6-S100A10) and AAV6-empty vector (AAV6-NC) were purchased from ViGene Biotech (Guangzhou, China). AAV6-S100A10 contained the fibroblast-specific promoter FSP1 that specifically induced the expression of S100A10 in lung fibroblast. Then, under anesthesia, every mouse was infected with 40ul of AAV6 (1011 genomic copies) by intratracheal instillation. Mice were rested for 3 weeks after infection in order to allow for rescue S100A10 expression in lung fibroblast of KO mice.
Depletion of MDSCs
Anti-Gr-1 Abs (BE0075, BioXcell) or isotype control (BE0090, BioXcell) was injected into the tail-vein of WT and KO mice at 200 µg/mouse the day before the B16/F10LUC were implanted. Subsequently, Gr-1 Abs or isotype control was injected every 3 d into tail vein of WT and KO mice until mice were euthanized.
Isolation and application of tumor exosomes
B16/F10 cells and E0771 cells were cultured using RPMI-1640 or DMEM medium for 48 h, after which the medium was collected and centrifuged at 1500 rpm for 5 min. The supernatant was collected and centrifuged at 3000 rpm for 20 min. Next, the cell debris was removed, and the supernatant was filtered using a 0.2 mm filter (Pall Corporation). The collected medium was ultracentrifuged at 100,000 g for 90 min at 4°C, and the supernatant was discarded. Pelleted exosomes were resuspended in PBS, and protein content was quantified via the BCA method. Then, we verified the concentration and particle size of exosomes via nanometer particle size meter (RuiXin, Nanocoulter I).
For in vivo induction of pre-metastatic microenvironment formation, 10 μg of exosomes in 50 μl of PBS were injected into the mice’s tail vein every 2 d. After 14 consecutive injections, the lungs were collected, and frozen sections were made. For in vivo exosome tracking experiments, purified exosomes were labeled with PKH26 red dye (Sigma). The labeled exosomes were washed, collected by ultracentrifugation, and resuspended in PBS. The labeled exosomes (10 μg) were then injected into the tail vein of the mice. One day after exosome injection, lung tissues were analyzed via immunofluorescence.
T-cell suppression assay
Isolation of Gr-1+Ly-6 G+ MDSCs, from the lung of WT and KO mice was performed. The MDSCs were isolated using a myeloid-derived suppressor cell isolation kit following the manufacturer’s standard protocol (Miltenyi Biotec, 130-094-538).
The CFSE-labeled T cells and MDSCs were co-cultured in a 24-well plate. MDSCs and T cells were cultured at ratios of 1:4. The number of T cells was defined as 100,000 cells per well. T cells were then stimulated using 1 μg/ml anti-CD3 (BD Biosciences) and 2 μg/ml anti-CD28 (BD Biosciences) and incubated at 37°C for 72 h. Cell proliferation was measured using CFSE dilution and flow cytometry.
Flow cytometry
The single-cell lung suspensions were stained using antibodies targeting CD4+ and CD8+ T lymphocytes (CD3+CD4+ and CD3+CD8+), B cells (CD3+CD19+), macrophages (CD11b+F4/80+), dendritic cells (CD11c+MHCII+), MDSCs (CD11b+Gr-1+), M-MDSCs (CD11b+Ly6C+), PMN-MDSCs (CD11b+Ly6G+) and NK cells (CD3−NK1.1+). The analyses were performed using a CanoII flow cytometer (BD) or Novocyte flow cytometer (Agilent). The antibodies were purchased from the BD Pharmingen TM or Bioleng.
In vitro differentiation of MDSCs from murine bone marrow
Murine bone marrow cells were isolated and cultured for 4 d in RPMI-1640 medium with 10% FBS, 10 mM HEPES, 1 mM sodium pyruvate, 1 mM MEM non-essential amino acids, 50 µM β-mercaptoethanol, 1% penicillin/streptomycin, 40 ng/mL GM-CSF (PeproTech 315–03), and 40ng/mL IL-6 (PeproTech 216–16).
Generation of murine bone marrow chimeras
The recipient mice were lethally irradiated to ablate their hematopoietic stem cells. Bone marrow (BM) cell suspensions were prepared from donor mice. 1 × 107 BM was transplanted into recipient mice. Recombinant mice were then treated with neomycin for 3 weeks to limit the risk of infection and were monitored regularly. After 3 weeks, flow cytometry was used to detect the bone marrow cell subtypes of the recombinant mice in order to evaluate the recombinant efficiency.
In vitro migration assay
We used a chemotaxis chamber (Corning 3422) to evaluate the effect of cell-conditioned media on the migration of MDSCs. A 100 µl MDSCs cell suspension (5 × 105 cells) aliquot was seeded into the upper wells. In the lower wells, 50 ng/ml recombinant mouse S100A10 protein (ProsPec pro-2252) or NIH3T3 cell stimulated by tumor exosome conditioned media were placed. An 8 µm pore size polycarbonate filter separated the upper and lower wells. After incubation for 3 h at 37°C with 5% CO2, the number of MDSCs that migrated to the lower wells was counted.
Immunofluorescence
The frozen tissue section was fixed using 4% paraformaldehyde and permeabilized with 0.1% Triton-X100 for 10 min. The sections were incubated with primary antibodies targeted against S100A4 (Proteintech 16,105–1-AP), Fibronectin (Santa Cruz sc-8422 1:100), Gr-1 (CST#31462 1:200), and Flag (Sigma Aldrich F1804 1:100) overnight at 4°C. The secondary antibodies, labeled with Alexa Fluor 488 (Invitrogen) and Alexa Fluor 546 (Invitrogen) were photo-imaged using a fluorescent microscope (Leica TCS SP5 II, Germany).
Statistical analysis
Each experiment was repeated at least three times. Results are expressed as mean ± SD or SEM as indicated. Statistical analysis was performed using GraphPad Prism 5 and presented in the following manners: *p < 0.05, **p < 0.01 and ***p < 0.001.
Results
S100A10 deficiency prevents lung metastasis
Recent studies have shown that tumor-derived exosomes bind to organ stromal cells and activate S100A10 in the lung microenvironment.Citation17 In this study, we tail vein inoculated S100A10−/− mice with B16/F10LUC/GFP melanoma cells and E0771GFP breast cancer cells, then observed cancer metastasis in order to study the role of organ resident cell S100A10 during tumor progression. A detailed construction of the S100A10 deficiency mice is shown in Supplemental Figure 1. Our results found that S100A10−/− (KO) mice revealed a significant reduction in lung metastasis compared to S100A10+/+ wild-type (WT) mice (). We observed that S100A10 deficiency inhibited the number of metastatic nodules and thus decreased lung-metastasis tissue weight compared to WT mice (). In vitro observation of lung tissue showed that luciferase or GFP labeled B16/F10 cells from the lungs of KO mice are less than those of WT mice (). The immunofluorescence and flow cytometry results showed that B16/F10GFP and E0771GFP cells in WT mice were higher than in KO mice ().
To further study the role of organ resident S100A10 during lung metastasis of in situ tumor, we inoculated the mice with B16/F10LUC via subcutaneous injection, allowing the cell to form a tumor. At 10 d, the tumor-bearing mice underwent surgical excision and continuous cultivation. Twenty-eight days after surgery, lung metastasis of the orthotopic tumor was monitored using bioluminescent imaging (). Compared to WT mice, KO mice had fewer metastases within the lung tissue (). The HE staining of whole lung tissue verified that S100A10 deficiency decreased the number of metastatic nodules (). Together, these observations suggest that host S100A10 contributes to lung metastasis of tumor.
S100A10 deficiency prevents the establishment of pre-metastatic microenvironment
The pre-metastatic microenvironment supports a favorable local microenvironment for the primary tumor metastasis. We used tumor-derived exosomes to induce pre-metastatic microenvironment formation within the lung of WT and KO mice (). We isolated exosomes from the B16/F10 and E0771 cells. We verified exosomes via nanometer particle size analysis and demonstrated the expression of exosome surface markers (Fig. S2). TIMP, S100A8, S100A9, and fibronectin play critical roles during pre-metastatic microenvironment formation.Citation18–21 We found that TIMP, S100A8, and S100A9 expression in the lungs of S100A10 KO mice were significantly reduced compared to the lung of WT mice (). The IF and IHC results revealed that fibronectin expression was down-regulated in the pre-metastatic microenvironment of S100A10 KO mice (). To further evaluate the effect of S100A10 deletion on pre-metastatic microenvironment formation of the lungs, transcriptome sequencing was performed on lung tissue from S100A10 KO and WT mice. We found that the biological function of the differentially expressed genes was focused on the immune response and the external stimulus (). The KEGG enrichment showed that S100A10 deficiency affected the ECM–receptor interaction, cytokine receptor interaction, and TGF-β or NF-κB signaling pathway (). Thus, S100A10 deficiency suppresses pre-metastatic microenvironment establishment within the lungs.
Figure 2. S100A10 deficiency prevents lung pre-metastatic microenvironment formation.
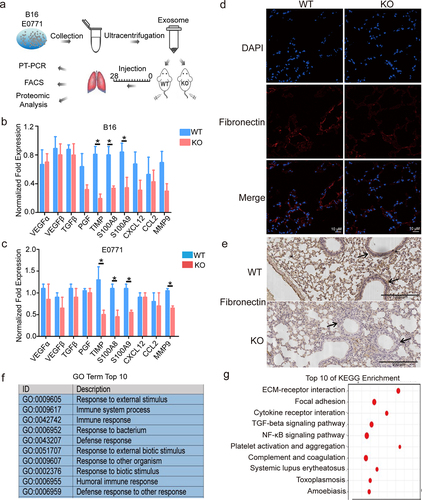
S100A10 deficiency suppresses MDSCs recruitment to the lung metastatic microenvironment
To further assess which S100A10 host cell has the effect of promoting cancer metastasis, we carried out the bone marrow (BM) adoptive transfer experiments (Fig. S3a). The first group of chimeras is the donor BM of CD45.2 subtype S100A10 KO or WT mice and recipient mice of CD45.1 subtype WT mice. The chimeras were subsequently subjected to tail vein injections with B16/F10 cells. Bright-field observation and HE staining of whole lung tissue showed that mice adoptive transfer of KO mice BM had fewer metastatic nodules than those adoptive transfer of WT mice BM (Figure S3b-d). The second group of chimers is donor BM of CD45.1 subtype WT mice and recipient mice of CD45.2 subtype S100A10 KO or WT mice. The results showed that recipient mice S100A10 KO mice had significantly reduced metastatic nodules compared to recipient mice S100A10 WT mice (Figure S3e-g). These results demonstrate that S100A10 deficiency in the host tissue or immune cells all altered lung metastatic in S100A10 KO mice. However, the absence of S100A10 in the host tissue has a more significant effect on cancer metastasis.
Next, we analyzed the number of immune cells in the pre-metastatic microenvironment of S100A10 KO and WT mice after tumor-derived inoculation. CD11b+Gr-1+ MDSCs in the pre-metastatic lungs of KO mice were significantly decreased compared to S100A10 WT (). We further found that the percentage of Ly6G+Ly6Clow PMN-MDSCs and Ly6G−Ly6Chigh M-MDSCs in the pre-metastatic lungs of KO mice is decreased compared to WT mice (). Furthermore, the number of CD3+CD4+ or CD3+CD8+ T cells in the pre-metastatic lungs of KO mice is increased (). The gating strategy for is shown in Supplemental
Figure 3. S100A10 deficiency inhibits MDSCs recruitment to the lung pre-metastatic microenvironment.
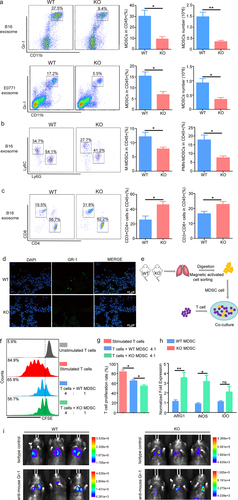
Figure 4. The S100A10 of lung fibroblasts activates CXCL1/CXCL8 expression and increases the migration of myeloid lineage via B16/F10-derived exosome induction.
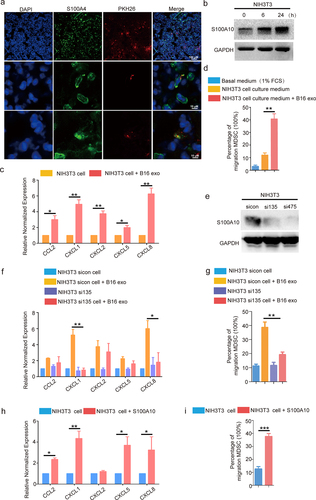
MDSCs exert immunosuppressive effects by targeting T cells. To examine the direct impact of MDSCs from S100A10 KO and WT mice lung metastatic microenvironment on effector T cells, we pre-treated T cells with anti-CD3 and anti-CD28 prior to co-culturing with lung MDSCs of WT and KO mice (
S100A10 deficiency of fibroblasts inhibits the activation of chemokines induced by exosomes and the migration of myeloid lineage
To understand the underlying mechanisms of impaired MDSCs recruitment in the pre-metastatic lungs of S100A10 KO mice after tumor-derived exosome stimulus, we first traced the location of the tumor-derived exosomes in the lung. Next, the B16/F10-derived exosomes labeled with PKH26 were injected into the tail vein of WT mice. After 24 h, we used IF to assess the B16/F10-derived exosomes in the lungs of WT mice. Our results demonstrated that the B16/F10-derived exosomes were primarily taken up by S100A4+ fibroblasts (
To test which chemokines are regulated by S100A10, we knocked down S100A10 (
Upregulation of lung fibroblasts S100A10 promotes cancer metastasis in vivo
To examine the role of lung fibroblasts S100A10 in cancer metastasis in mice models, we intratracheally delivered adeno-associated virus serotype AAV6-NC or specifically overexpressed S100A10 virus serotype AAV6-S100A10 in lung fibroblasts to WT and KO mice (
Figure 5. Upregulation of S100A10 expression in lung fibroblasts promotes lung metastasis of tumor in KO mice.
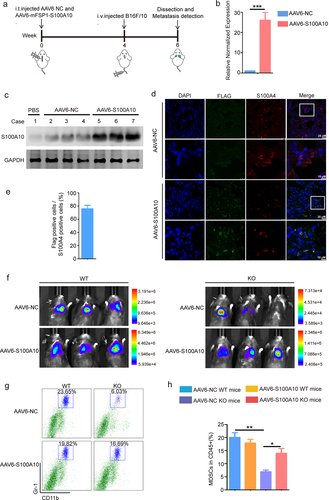
S100A10 inhibitor prevents cancer metastasis
Our results indicated that S100A10 plays an important role in cancer metastasis. To test whether the S100A10 inhibitor reduced cancer metastasis, an S100A10 inhibitor was intraperitoneally injected into mice treated with B16/F10 cells. 1-substituted 4-aroyl-3-hydroxy-5-phenyl-1 H-pyrrol-2(5 H)-ones, an effective blocker of S100A10 and AnxA2 binding inhibited the function of S100A10.Citation22 The
Figure 6. S100A10 inhibitor prevents lung metastasis.
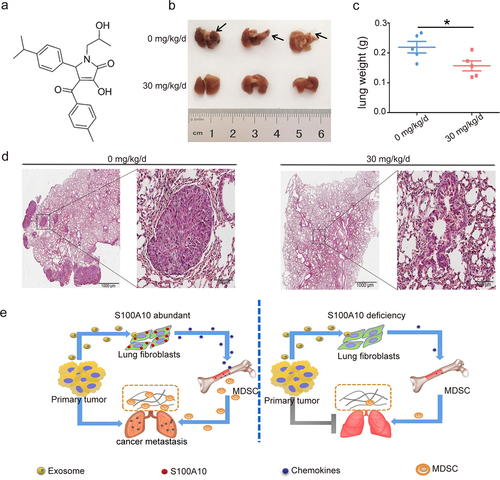
In summary, we show that the activation of S100A10 in lung fibroblasts via tumor-derived exosomes initiates MDSCs recruitment and remodels the local immune microenvironment during cancer metastasis (
Discussion
Previous studies on cancer metastasis have suggested complex interactions facilitated by cancer, BMDCs, and immune cells. Moreover, recent studies have highlighted the pleiotropic functions of MDSCs by promoting distant metastasis via inflammation and immunosuppression. MDSCs through the secretion of inflammatory cytokines and growth factors remodel the local microenvironment, resulting in the colonization and growth of cancer cells. Experimental evidence suggests that MDSCs are abundant in the pre-metastatic lung and are related to the burden of subsequent metastatic breast cancer.Citation23 MDSCs, mobilized with G-CSF in distant organs before the arrival of in situ tumor cells, produces the Bv8 protein in order to stimulate the migration of breast cancer cells.Citation24 Furthermore, MDSCs facilitate metastatic growth via the induction of vascular leakage and the remodeling of ECM, as well as systemic effects on the immune system.Citation5,Citation6,Citation24 Interestingly, in our study, we found that S100A10 deficiency reduced MDSCs accumulation during the remodeling of the local immune microenvironment of the lungs. Our results suggest that S100A10 deficiency affects MDSCs recruitment and cancer metastasis. Previous studies on the function of S100A10 on the recruitment of immune cells into the primary tumor microenvironment have revealed that S100A10 regulates the migration of macrophages to the tumor sites.Citation25,Citation26 The function of the S100A10 plasminogen receptor could partially explain this. S100A10 binding to plasminogen results in the conversion of plasminogen to plasmin via the activation of plasminogen activators-tissue-type plasminogen activator (t-PA) and urokinase-type-plasminogen activator (uPA).Citation26,Citation27 The plasminogen activation process is integral to macrophage migration under inflammatory stimulation.Citation28–30 In the S100A10 deficient mice lung immune microenvironment, the macrophage cells did not reveal any differences compared to WT mice. This may illustrate the different roles of S100A10 during the formation of the primary tumor microenvironment and cancer metastasis.
Lung fibroblasts have functional plasticity during promoting local immune microenvironment formation.Citation31 The COX-2+ lung fibroblasts improve lung-resident myeloid cells’ immune-suppressive function and drive dysfunctional dendritic cells, resulting in increased immunosuppression and lung metastasis in breast cancer models.Citation32 TDFS acts on the lung fibroblasts that are activated by the p38α kinase/type I interferon signaling/fibroblast activation protein axis, resulting in the remodeling of the ECM in the lungs.Citation33 In our WT mice model, lung fibroblast cells primarily take up exosomes derived from B16/F10 cells. Subsequently, the expression of S100A10 and the important chemokines CCL2, CXCL1, CXCL2, CXCL5, and CXCL8 were significantly activated in lung fibroblasts. However, B16/F10-derived exosomes cannot induce the expression of CXCL1 and CXCL8 in the S100A10 deficient fibroblasts. Increased expression of CXCL1 and CXCL8 is commonly seen in many tumors,Citation34–37 The up-regulated CXCL1 and CXCL8 reflect high tumor burden, poor tumor differentiation, and poor prognosis.Citation38,Citation39 The function of the CXCL1 and CXCL8 is chemotactic for MDSCs, neutrophils, and monocytes and remodels the immune microenvironment of the tumor. In co-implantation experiments, the growth of xenograft tumors was increased with MDSCs from CXCL8 transgenic mice as compared to MDSCs from WT mice.Citation40 Tumor-derived CXCL8 showed increased mobilization of MDSCs into the tumor site.Citation40,Citation41 In CXCR2−/− mice, CXCL1/2/5-CXCR2 signaling led to the recruitment of MDSCs into the liver.Citation42 These results indicate that CXCL1 and CXCL8 are important chemokines that cause immune cells infiltration into the local immune microenvironment. We found that S100A10 deficiency of lung fibroblasts inhibited the activation of CXCL1 and CXCL8. Meanwhile, tumor-derived exosomes induced migration of myeloid lineage was decreased. In particular, we found that the S100A10 recombinant protein significantly activated fibroblast CXCL1 and CXCL8 expression of and induced the migration of the myeloid lineage. We propose that S100A10 contributes to the promotion of MDSCs recruitment and pre-metastatic microenvironment formation by inducing the activation of CXCL1 and CXCL8 in lung fibroblasts. This study highlighted the vital role of S100A10 in driving MDSCs recruitment into the lungs as an early step of primary cancer metastasis.
Supplemental Material
Download MS Word (10.2 MB)Acknowledgments
The study was funded by grants from the National Natural Science Foundation Of China (No. 82373389 82173023), the Innovation Capability Support Program of Shaanxi (No. 2020TD-046), the Key Research and Development Program of Shaanxi (No. 2019SF-145) and the First Affiliated Hospital of Xian Jiaotong University College of Medicine Foundation (No. 2022 MS-01).
Disclosure statement
The authors declare that they have no known competing financial interests or personal relationships.
Data availability statement
The data that support the findings of this study are available from the corresponding author upon reasonable request.
Supplementary material
Supplemental data for this article can be accessed online at https://doi.org/10.1080/2162402X.2024.2381803.
Additional information
Funding
References
- Quail DF, Joyce JA. Microenvironmental regulation of tumor progression and metastasis. Nat Med. 2013;19:1423–13.
- Han L, Lam EW-F, Sun Y. Extracellular vesicles in the tumor microenvironment: old stories, but new tales. Mol Cancer. 2019;18(1):59. doi:10.1186/s12943-019-0980-8.
- Wortzel I, Dror S, Kenific CM, Lyden D. Exosome-mediated metastasis: communication from a distance. Dev Cell. 2019;49(3):347–360. doi:10.1016/j.devcel.2019.04.011.
- Nakamura K, Smyth MJ. Myeloid immunosuppression and immune checkpoints in the tumor microenvironment. Cell Mol Immunol. 2020;17(1):1–12. doi:10.1038/s41423-019-0306-1.
- Kumar V, Patel S, Tcyganov E, Gabrilovich DI. The nature of myeloid-derived suppressor cells in the tumor microenvironment. Trends Immunol. 2016;37(3):208–220. doi:10.1016/j.it.2016.01.004.
- Hua BL, Garstka MA, Li ZF. Chemokines and their receptors promoting the recruitment of myeloid -derived suppressor cells into the tumor. Mol Immunol. 2020;117:201–215. doi:10.1016/j.molimm.2019.11.014.
- Veglia F, Perego M, Garbrilovich D. Myeloid-derived suppressor cells coming of age. Nat Immunol. 2018;19(2):108–119. doi:10.1038/s41590-017-0022-x.
- Davidson S, Coles M, Thomas T, Kollias G, Ludewig B, Turley S, Brenner M, Buckley CD. Fibroblasts as immune regulators in infection, inflammation and cancer. Nat Rev Immunol. 2021;21(11):704–717. doi:10.1038/s41577-021-00540-z.
- Desbois M, Wang Y. Cancer-associated fibroblasts: key players in shaping the tumor immune microenvironment. Immunol Rev. 2021;302(1):241–258. doi:10.1111/imr.12982.
- Miller VA, Madureira PA, Kamaludin AA, Komar J, Sharma V, Sahni G, Thelwell C, Longstaff C, Waisman DM. Mechanism of plasmin generation by S100A10. Thromb Haemost. 2017;117(06):1058–1071. doi:10.1160/TH16-12-0936.
- Yang X, Popescu NC, Zimonjic DB. DLC1 interaction with S100A10 mediates inhibition of in vitro cell invasion and tumorigenicity of lung cancer cells through a RhoGAP-independent mechanism. Cancer Res. 2011;5(8):2916–2925. doi:10.1158/0008-5472.CAN-10-2158.
- Zhou X, Shi M, Cao J, Yuan T, Yu G, Chen Y, Fang W, Li H. S100 calcium binding protein A10, a novel oncogene, promotes the proliferation, invasion, and migration of hepatocellular carcinoma. Front Genet. 2021;12:695036. doi:10.3389/fgene.2021.695036.
- Svenningsson P, Chergui K, Rachleff I, Flajolet M, Zhang X, El Yacoubi M, Vaugeois J-M, Nomikos GG, Greengard P. Alterations in 5-HT 1B receptor function by p11 in Depression-Like States. Science. 2006;311(5757):77–80. doi:10.1126/science.1117571.
- van de Graaf SF, Hoenderop JG, Gkika D, Lamers D, Prenen J, Rescher U, Gerke V, Staub O, Nilius B, Bindels RF. Functional expression of the epithelial Ca(2+) channels (TRPV5 and TRPV6) requires association of the S100A10-annexin 2 complex. Embo J. 2003;22:1478–1487. doi:10.1093/emboj/cdg162.
- Okuse K, Malik-Hall M, Baker MD, Poon WY, Kong H, Chao MV, Wood JN. Annexin II light chain regulates sensory neuron-specific sodium channel expression. Nature. 2002;417(6889):653–656. doi:10.1038/nature00781.
- Lu H, Xie Y, Tran L, Lan J, Yang Y, Murugan NL, Wang R, Wang YJ, Semenza GL. Chemotherapy-induced S100A10 recruits KDM6A to facilitate OCT4-mediated breast cancer stemness. J Clin Invest. 2020;130(9):4607–4623. doi:10.1172/JCI138577.
- Eisenblaetter M, Flores-Borja F, Lee JJ, Wefers C, Smith H, Hueting R, Cooper MS, Blower PJ, Patel D, Rodriguez-Justo M. et al. Visualization of tumor-immune interaction-target-specific imaging of S100A8/A9 reveals pre-metastatic niche establishment. Theranostics. 2017;7(9):2392–2401. doi:10.7150/thno.17138.
- Lukanidin E, Sleeman JP. Building the niche: the role of the S100 proteins in metastatic growth. Semin Cancer Biol. 2012;22(3):216–225. doi:10.1016/j.semcancer.2012.02.006.
- Rao VS, Gu Q, Tzschentke S, Lin K, Ganig N, Thepkaysone ML, Wong FC, Polster H, Seifert L, Seifert AM. et al. Extravesicular TIMP-1 is a non-invasive independent prognostic marker and potential therapeutic target in colorectal liver metastases.Oncogene. Oncogene. 2022;41(12):1809–1820. doi:10.1038/s41388-022-02218-9.
- Hiratsuka S, Watanabe A, Sakurai Y, Akashi-Takamura S, Ishibashi S, Miyake K, Shibuya M, Akira S, Aburatani H, Maru Y. et al. The S100A8–serum amyloid A3–TLR4 paracrine cascade establishes a pre-metastatic phase. Nat Cell Biol. 2008;10(11):1349–1355. doi:10.1038/ncb1794.
- Sara F, Santos L, Sampaio-Ribeiro G, Ferreira HR, Lima N, Caetano R, Abreu M, Zuzarte M, Ribeiro AS, Paiva A. et al. Unveiling the role of osteosarcoma-derived secretome in premetastatic lung remodelling. J Exp Clin Cancer Res. 2023;42(1):328. doi:10.1186/s13046-023-02886-9.
- Reddy TR, Li C, Guo X, Myrvang HK, Fischer PM, Dekker LV. Design, synthesis, and Structure−Activity relationship exploration of 1-substituted 4-aroyl-3-hydroxy-5-phenyl-1 H -pyrrol-2(5 H)-one Analogues as inhibitors of the annexin A2−S100A10 protein interaction. J Med Chem. 2011;54(7):2080–2094. doi:10.1021/jm101212e.
- Monteran L, Ershaid N, Doron H, Zait Y, Scharff Y, Ben-Yosef S, Avivi C, Barshack I, Sonnenblick A, Erez N. et al. Chemotherapy-induced complement signaling modulates immunosuppression and metastatic relapse in breast cancer. Nat Commun. 2022;13(1):5797. doi:10.1038/s41467-022-33598-x.
- Kowanetz M, Wu X, Lee J, Tan M, Hagenbeek T, Qu X, Yu L, Ross J, Korsisaari N, Cao T. et al. Granulocyte-colony stimulating factor promotes lung metastasis through mobilization of Ly6G+Ly6C+ granulocytes. Proc Natl Acad Sci USA. 2010;107(50):21248–21255. doi:10.1073/pnas.1015855107.
- Sceneay J, Parker BS, Smyth MJ, Moller A. Hypoxia-driven immunosuppression contributes to the pre-metastatic niche. Oncoimmunology. 2013;2(1):e22355. doi:10.4161/onci.22355.
- Phipps KD, Surette AP, Pa O, Waisman DM. Plasminogen receptor S100A10 is essential for the migration of tumor-promoting macrophages into tumor sites.Cancer res. 2011;71(21):6676–6683. doi:10.1158/0008-5472.CAN-11-1748.
- O’Connell PA, Surette AP, Liwski RS, Svenningsson P, Waisman DM. Waisman DM.S100A10 regulates plasminogen-dependent macrophage invasion. Blood. 2010;116(7):1136–1146. doi:10.1182/blood-2010-01-264754.
- Surette AP, Madureira PA, Phipps KD, Miller VA, Svenningsson P, Waisman DM. Regulation of fibrinolysis by S100A10 in vivo. Blood. 2011;118(11):3172–3181. doi:10.1182/blood-2011-05-353482.
- Kubala MH, Punj V, Placencio-Hickok VR, Fang H, Fernandez GE, Sposto R, DeClerck YA. Plasminogen activator inhibitor-1 promotes the recruitment and polarization of macrophages in cancer. Cell Rep. 2018;25(8):2177–2191. doi:10.1016/j.celrep.2018.10.082.
- Sugimoto MA, Ribeiro ALC, Costa BRC, Vago JP, Lima KM, Carneiro FS, Ortiz MMO, Lima GLN, Carmo AAF, Rocha RM. et al. Plasmin and plasminogen induce macrophage reprogramming and regulate key steps of inflammation resolution via annexin A1. Blood. 2017;129(21):2896–2907. doi:10.1182/blood-2016-09-742825.
- Gong Z, Li Q, Shi J, Wei J, Li P, Chang CH, Shultz LD, Ren G. Lung fibroblasts facilitate pre-metastatic niche formation by remodeling the local immune microenvironment. Immunity. 2022;55(8):1483–1500. doi:10.1016/j.immuni.2022.07.001.
- Zhang H, Jiang H, Zhu L, Li J, Ma S. Cancer-associated fibroblasts in non-small cell lung cancer: recent advances and future perspectives. Cancer Lett. 2021;514:38–47. doi:10.1016/j.canlet.2021.05.009.
- Gui J, Zahedi F, Ortiz A, Cho C, Katlinski KV, Alicea-Torres K, Li J, Todd L, Zhang H, Beiting DP. et al. Activation of p38α stress-activated protein kinase drives the formation of the pre-metastatic niche in the lungs. Nat Cancer. 2020;1(6):603–619. doi:10.1038/s43018-020-0064-0.
- Wang D, Sun H, Wei J, Cen B, DuBois RN. CXCL1 is critical for premetastatic niche formation and metastasis in colorectal cancer. Cancer Res. 2017;77(13):3655–3665. doi:10.1158/0008-5472.CAN-16-3199.
- Su L, Li N, Tang H, Lou Z, Chong X, Zhang CG, Su J, Dong X. Kupffer cell-derived TNF-α promotes hepatocytes to produce CXCL1 and mobilize neutrophils in response to necrotic cells. Cell Death Dis. 2018;9(3):323. doi:10.1038/s41419-018-0377-4.
- Ha H, Debnath B. Neamati N.Role of the CXCL8-CXCR1/2 axis in cancer and inflammatory diseases. Theranostics. 2017;7(6):1543–1588. doi:10.7150/thno.15625.
- Liu Q, Li A, Tian Y, Wu JD, Liu Y, Li T, Chen Y, Han X, Wu K. The CXCL8-CXCR1/2 pathway in cancer. Cytokine Growth Factor Rev. 2016;3:61–71. doi:10.1016/j.cytogfr.2016.08.002.
- Cacev T, Radosevic S, Krizanac S, Kapitanovic S. Kapitanovic S.Influence of interleukin-8 and interleukin-10 on sporadic colon cancer development and progression. Carcinogenesis. 2008;29(8):1572–1580. doi:10.1093/carcin/bgn164.
- Highfill SL, Cui Y, Giles AJ, Smith JP, Zhang H, Morse E, Kaplan RN, Mackall CL. Disruption of CXCR2-mediated MDSCs tumor trafficking enhances anti-PD1 efficacy. Sci Transl Med. 2014;6(237):237–267. doi:10.1126/scitranslmed.3007974.
- Asfaha S, Dubeykovskiy AN, Tomita H, Yang X, Stokes S, Shibata W, Friedman RA, Ariyama H, Dubeykovskaya ZA, Muthupalani S. et al. Mice that express human interleukin-8 have increased mobilization of immature myeloid cells, which exacerbates inflammation and accelerates colon carcinogenesis. Gastroenterology. 2013;144(1):155–166. doi:10.1053/j.gastro.2012.09.057.
- David JM, Dominguez C, Hamilton DH, Palena C. The IL-8/IL-8R axis: a double agent in tumor immune resistance. Vaccines. 2016;4(3):22. doi:10.3390/vaccines4030022.
- Katoh H, Wang D, Daikoku T, Sun H, Dey SK, Dubois RN. CXCR2-expressing myeloid-derived suppressor cells are essential to promote colitis-associated tumorigenesis. Cancer Cell. 2013;24(5):631–644. doi:10.1016/j.ccr.2013.10.009.