Abstract
Pneumococci are capable of vaccine escape by genetic recombination at the targeted capsular locus, significantly reducing long-term vaccine effectiveness. Recently, efforts have been redirected to understanding pneumococcal biology related to potential next-generation vaccine candidates. A variety of serotype-independent protein antigens capable of inducing protective immune responses in tissue culture and animal models of infection have been identified. However, ideal vaccine candidates that are conserved across all genotypes, provide broad population coverage, and induce T-cell dependent immune responses are still under investigation. We examined whether immune responses due to the highly polymorphic CbpA antigen are due to a conserved domain capable of evoking specific immune “memory” across all genotypes of pneumococci. We defined the genotypes in a global dataset of 213 pneumococcal isolates. This isolate collection was genotypically diverse and ideal for establishing the presence of conserved CbpA epitopes as potential protein vaccine candidates. Examination of the CbpA locus sequence was highly polymorphic at both the nucleic acid and amino acid level. Despite this high polymorphism some domains are broadly conserved and consist of different amino acid residues with the same physicochemical properties, and therefore have similar tertiary structures. The two most common domains identified in the CbpA gene are modular teichoic acid phosphorylcholine esterase Pce (2bib:A), and R2 domain (1w9r:A). These conserved domains are immunogenic, therefore capable of inducing long-term host immune responses; moreover they are extracellularly located and thus accessible. We proposed their evaluation as suitable next-generation CbpA-fusion protein vaccine candidates.
Keywords:
Introduction
Streptococcus pneumoniae (pneumococcus) is an important cause of meningitis, pneumonia, sepsis, sinusitis and otitis media.Citation1-3 Each year, invasive pneumococcal disease (IPD) constitutes a significant proportion of the global morbidity and mortality, with over 800,000 deaths in children under the age of 5 years, and the majority of this disease burden is in developing countries.Citation4 Pneumococcal invasion of sterile tissue sites (lungs, blood, and cerebral spinal fluid) is preceded by its asymptomatic colonisation of the human nasopharynx.Citation5,6 The intricate multi-step invasion process first entails binding of pneumococcal surface ligands to host mucosal epithelium receptors, followed by translocation into the circulatory system.Citation7
One such important ligand is choline-binding protein A (CbpA), which during colonisation binds to polymeric immunoglobulin receptor (pIgR) facilitating mucosal cell transcytosis, while dysregulating host immune response.Citation7,8 Once in circulation CbpA subsequently binds to either laminin receptor or polymeric immunoglobulin receptor (PIgR) at the blood-brain barrier again enabling invasion of the cerebral spinal fluid (CSF), leading to meningitis.Citation3,7,9 The virulence properties of this pneumococcal adhesin have been extensively described using both cell-culture and animal models of infection.Citation9-16 However, recent data suggests that CbpA is inaccessible to circulating host antibodies compared to other immunogenic pneumococcal surface proteins,Citation17 thereby eliciting less optimal titres of protective antibodies. Development of CbpA-fusion proteins, such as those bound to cytokines elicits significantly better host immune responses,Citation18 and has been proposed as a strategy for the next-generation CbpA based pneumococcal vaccines.Citation17 Recently, the use of broadly protective protein-based pneumococcal vaccines composed of pneumolysin toxoid-CbpA peptide recombinant fusion protein has been demonstrated to elicit immune responses in vitro against Streptococcus pneumoniae, Neisseria meningitidis, and Haemophilus influenzae.Citation19 CbpA virulence has also been suggested to vary between genotypes,Citation8 and it is unclear whether a vaccine based on this antigen would elicit specific protective immune responses against all invasive pneumococcal isolates. Previous studies on CbpA sequence diversity were based on Southern blot hybridization and DNA sequencing of specific short PCR fragments.Citation20,21 However the pneumococcus is highly recombigenic and has multiply spliced genes, complicating comparisons of entire gene sequences from multiple strains.Citation22,23 In order to conclusively explore the potential of CbpA as a vaccine target, we revisited analysis of the genetic diversity of this gene locus, by exploiting next-generation sequence data from a global collection of 213 complete pneumococcal genomes. We hypothesized that although the nucleic and amino acid CbpA sequences display variation, there are conserved amino acid motifs that define conserved tertiary antigen structures, which can be exploited as potential vaccine targets against all pneumococcal genotypes. We define the genotype of the isolates in our global data set, the prevalence of CbpA and its genetic diversity at nucleic and amino acid level, and describe the effect of this sequence diversity on the tertiary CbpA protein structure. We anticipate that this study will expand knowledge on pneumococcal biology, identify conserved CbpA epitopes that can be exploited as next-generation potential vaccine targets, and also facilitate future detection of genetic changes at the CbpA locus.
Results
Determination of CbpA prevalence and MLST genotype
We identified the CbpA locus in 211 (99%) of the 213 genomes. We defined 113 distinct pneumococcal genotypes ( and ), which represent the genotypic diversity in our global pneumococcal dataset. Sequence Type (ST) 180 was the most abundant (n = 9 ; 4%), and 6 previously unidentified STs were detected. In our data set, 20 STs had a frequency of more than 2 (), 16 had a frequency of 2, and 77 STs were unique to a single isolate. This shows that the global pneumococcal isolate collection under investigation consists of pneumococcal isolates with diverse genotypes.
Diversity of the CbpA locus at nucleic and amino acid level
We examined the diversity of the CbpA locus at both the nucleic and amino acid level (). The sequences were highly polymorphic at both levels, confirming previous observations of genetically diverse alleles of the surface exposed CbpA antigen.Citation21,24 Interestingly, we did not observe uniformity in residues across isolates at their relative aligned positions for the amino acid sequences of the entire CbpA dataset (). However, amino acid residues with similar physicochemical properties aligned allowing the identification of broadly conserved CbpA domains. A statistical test of selection neutralityCitation25 across the CbpA loci considered in our study shows a significant signature for balancing selection (Tajima's D = 0.59). We speculate that this polymorphism can be attributed to diversifying selection pressure to enable evasion of host immune responses and survival in different compartments of niche on the host during colonisation and invasion.
Figure 2. Sequence diversity of the CbpA locus at the nucleic and amino acid level. The sequences were highly polymorphic at both (A) nucleic and (B) amino acid level. A maximum likelihood tree (100 bootstraps) was used to reconstruct the nucleic acid sequence relationship, while a parsimony tree (100 bootstraps) was used for the amino acid sequences. The color key is used to identify the residues corresponding on the heatmap. All similar residues across the data set are represented in yellow, and INDELs in white.
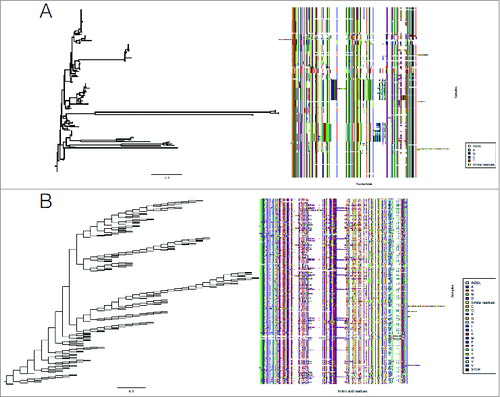
Identification of conserved tertiary structures and antigen epitopes
We used the RaptorX server to determine if the tertiary structure of CbpA is conserved. Tertiary protein structures that are conserved across the entire data set provide useful vaccine targets that are guaranteed to provide broad protection for a wider population against a broad range of invasive pneumococcal strains. We observed that the most common domains matched previously characterized pneumococcal templates in the protein data bank (PDB) for the modular teichoic acid phosphorylcholine esterase Pce (2bib:A), and the CbpA R2 domain (1w9r:A) (Tables S2 and S3). VaxiJen predicted the 2bib:A and 1w9r:A matching domains as probable antigens with an overall antigen prediction score of 0.49 and 1.28 respectively, which is above the 0.4 bacterial model threshold used in the predictions. This indicates that the 2 domains are antigenic epitopes capable of eliciting host immune responses, and are therefore ideal vaccine targets. Moreover, the sequences of these 2 domains are also extracellularly located (). Thus the protein sequences encoded by these domains qualify as potential vaccine candidates, which are broadly conserved, accessible and immunogenic against a broad range of invasive pneumococcal strains, regardless of their genotype.
Figure 3. THMM prediction of surface protein cellular location. The results show posterior probabilities of inside (thin blue line), outside (thin pink line), and the transmembrane helix (thin red line). The best prediction (over-all most probable structure) is shown at the top of the plot (between 1 and 1.2), and is obtained by calculating the total probability that a residue sits in helix (thick red line), inside (thick blue line), or outside (thick pink line) summed over all possible paths through the model. The x-axis shows the sequence length, and the y-axis the posterior probability. None of the residues was intracellularly located or formed part of a transmembrane helix, hence no blue or red lines associated with these respective posterior probabilities was observed. Both (A) modular teichoic acid phosphorylcholine esterase Pce (2bib:A), and (B) the R2 domain (1w9r:A) are located extracellularly, and are therefore accessible.
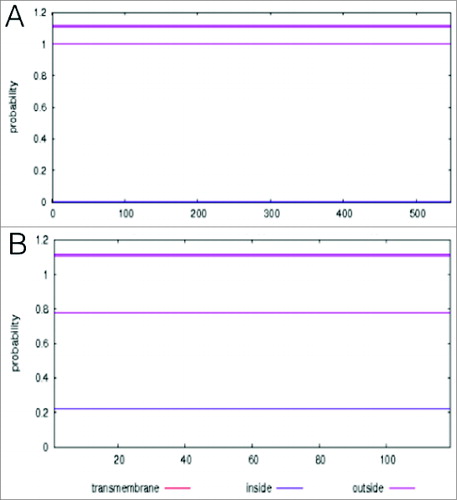
Discussion
Pneumococcal vaccines remain the principal effective means of preventing morbidity, and significantly averting IPD associated mortality. The World Health Organization recommended the introduction of pneumococcal conjugate vaccines (PCV) in developing countries with high child mortality, and the Global Alliance for Vaccines and Immunisations (GAVI) has provided support for PCV introduction into routine childhood immunization programs.Citation26,27 Universal vaccination with PCV7 in the United States led to a 77% decrease of IPD among children under the age of 5 years, and a 39% decrease in hospital pneumonia-related admissions among children under the age of 2 y.Citation27 However, pneumococci are capable of vaccine escape by genetic recombination at the targeted capsular locus, thereby reducing the long-term effectiveness of PCV vaccines.Citation28,29 This has considerably renewed interests in understanding pneumococcal biology related to potential next-generation vaccine candidates.
A variety of serotype-independent protein antigens capable of inducing protective immune responses in tissue culture have been identified.Citation7,16,17,30-35 These potential pneumococcal vaccine candidates should ideally be conserved across all genotypes, have broad population coverage, and induce T-cell dependent immune responses. One of the most well studied antigens is choline-binding protein A (CbpA), which is capable of inducing protective immune responses.Citation10,24,36,37 However, the CbpA gene locus is highly recombinant and consists of highly divergent sequences at nucleic acid level, yet still capable of functional immunogenic protein assembly. It was unclear whether immune responses due to CbpA are due to a conserved domain capable of evoking specific immune “memory” across all genotypes of pneumococci.
We hypothesized that although the nucleic sequences are divergent, and may give rise to divergent protein sequences, there are conserved amino acid motifs leading to a defined conserved tertiary antigen structure that can be exploited as potential vaccine targets against all pneumococcal genotypes. This conservation is essential for successful induction of long-term immune responses. We defined the genotypes in a global dataset of 213 pneumococcal isolates. We were limited to convenient sampling from an available global data set. However, the isolate collection was diverse and ideal for establishing whether there are conserved CbpA epitopes that are potential protein vaccine candidates. Examination of the CbpA locus sequence diversity showed high polymorphism at both the nucleic acid and amino acid level. This supports previous observations of pronounced allelic variation at this locus, using individual isolates.Citation21,24 Despite the lack of residue uniformity in the relative aligned position of the amino acid sequences. Some domains are broadly conserved and consist of different amino acid residues with the same physicochemical properties, and therefore similar tertiary structures. We postulate that this polymorphism is due to diversifying selection pressure to enable evasion of host immune responses and survival in different host compartments during colonisation and invasion. The two most common domains identified in the CbpA gene are modular teichoic acid phosphorylcholine esterase Pce (2bib:A), and the CbpA R2 domain (1w9r:A). These domains were predicted to be extracellularly located, antigenic epitopes capable of eliciting host immune responses, and thus qualify as ideal vaccine targets that are broadly conserved across pneumococcal genotypes.
Modular teichoic acid phosphorylcholine esterase Pce has been implicated in adherence and hydrolysis of the host's platelet activating factor receptors (PAFr), thereby regulating virulence by facilitating pneumococcal intracellular translocation.Citation7 R2 domain also binds to the host's polymeric immunoglobulin receptor (PIgR) facilitating pneumococcal intracellular translocation at the mucosal epithelium and the brain-blood barrier, allowing bloodstream and cerebral spinal fluid invasion respectively. Moreover, these conserved domains are immunogenic, therefore capable of inducing long-term host immune responses, and extracellularly located and thus accessible. We propose specific IPD tissue culture and animal model evaluation of modular teichoic acid phosphorylcholine esterase Pce, and the CbpA R2 domains as suitable next-generation CbpA-fusion protein vaccine candidates.
Materials and Methods
Genome sequence retrieval and CbpA prevalence
The complete genome sequences of 213 pneumococci were retrieved from the National Center for Biotechnology's (NCBI) GenBank database (Table S1). The CbpA locus nucleotide and amino acid sequences, which constitute the primary input data, were identified and retrieved using a Perl script.
Determination of MLST genotype
MLST genotyping using entire genome sequences was performed at the CGE server.Citation38
Sequence alignments and phylogeny reconstruction
Multiple sequence alignments were performed using MUSCLE.Citation39 The maximum-likelihood (ML) phylogenetic analysis of multiple aligned nucleic acid sequences with 100 bootstrap replicates was performed using PhyML (version 3.5).Citation40 A parsimony tree of the aligned amino acid sequences was reconstructed using PHYLIP (version 3.5).Citation41 Sequence diversity heatmaps were generated using an R script.
Identification of conserved tertiary structures and antigen epitopes
Conserved CbpA tertiary structures were identified using the RaptorX server.Citation42 RaptorX consists of algorithms optimised to predict 3D protein structures in the absence of homologs in the Protein Data Bank (PDB).Citation43 The VaxiJen (version 2.0) server was used to predict protective CbpA epitopes.Citation44-46 VaxiJen performs alignment-independent prediction and classification of protective antigens using their physicochemical properties. The conserved domains containing these CbpA epitopes were further evaluated using the Transmembrane Hidden Markov Models (THMM) (version 2.0) server to determine whether they are extracellularly located.Citation47
Disclosure of Potential Conflicts of Interest
No potential conflicts of interest were disclosed.
Authors' Contributions
B.K. conceived the study. M.A., K.K., F.O., W.O.,S.S., B.K. performed the analysis. BK wrote the first draft of the manuscript. M.A., K.M., F.O., W.O., S.S., B.K. contributed to writing the final version of the manuscript.
Supplemental_Material.zip
Download Zip (232.8 KB)References
- Hausdorff WP, Feikin DR, Klugman KP. Epidemiological differences among pneumococcal serotypes. Lancet Infect Dis 2005; 5: 83-93; PMID:15680778; http://dx.doi.org/10.1016/S1473-3099(05)01280-6
- McCullers JA, Tuomanen EI. Molecular pathogenesis of pneumococcal pneumonia. Front Biosci 2001; 6: D877-89; PMID:11502489; http://dx.doi.org/10.2741/McCullers
- Ring A, Weiser JN, Tuomanen EI. Pneumococcal trafficking across the blood-brain barrier. Molecular analysis of a novel bidirectional pathway. J Clin Invest 1998; 102: 347-60; PMID:9664076; http://dx.doi.org/10.1172/JCI2406
- O'Brien KL, Wolfson LJ, Watt JP, Henkle E, Deloria-Knoll M, McCall N, Lee E, Mulholland K, Levine OS, Cherian T. Burden of disease caused by Streptococcus pneumoniae in children younger than 5 years: global estimates. Lancet 2009; 374: 893-902; PMID:19748398; http://dx.doi.org/10.1016/S0140-6736(09)61204-6
- Bogaert D, Groot R, De Hermans PW. Streptococcus pneumoniae colonisation: the key to pneumococcal disease. Lancet Infect Dis 2004; 4: 144-54; PMID:14998500; http://dx.doi.org/10.1016/S1473-3099(04)00938-7
- Abdullahi O, Nyiro J, Lewa P, Slack M, Scott JA. The descriptive epidemiology of streptococcus pneumoniae and haemophilus influenzae nasopharyngeal carriage in children and adults in kilifi district, kenya. Pediatr Infect Dis J 2008; 27: 59-64; PMID:18162940; http://dx.doi.org/10.1097/INF.0b013e31814da70c
- Mook-Kanamori BB, Geldhoff M, van der Poll T, van de Beek D. Pathogenesis and pathophysiology of pneumococcal meningitis. Clinl Microbiol Rev 2011; 24: 557-91; PMID:21734248; http://dx.doi.org/10.1128/CMR.00008-11
- Kerr AR, Paterson GK, McCluskey J, Iannelli F, Oggioni MR, Pozzi G, Mitchell TJ. The contribution of PspC to pneumococcal virulence varies between strains and is accomplished by both complement evasion and complement-independent mechanisms. Infect Immun 2006; 74: 5319-24; PMID:16926426; http://dx.doi.org/10.1128/IAI.00543-06
- Orihuela CJ, Mahdavi J, Thornton J, Mann B, Wooldridge KG, Abouseada N, Oldfield NJ, Self T, Ala'Aldeen DA, Tuomanen EI. Laminin receptor initiates bacterial contact with the blood brain barrier in experimental meningitis models. J Clin Invest 2009; 119: 1638-46; PMID:19436113; http://dx.doi.org/10.1172/JCI36759
- Orihuela CJ, Gao G, Francis KP, Yu J, Tuomanen EI. Tissue-specific contributions of pneumococcal virulence factors to pathogenesis. J Infect Dis 2004; 190: 1661-9; PMID:15478073; http://dx.doi.org/10.1086/424596
- Luo R, Mann B, Lewis WS, Rowe A, Heath R, Stewart ML, Hamburger AE, Sivakolundu S, Lacy ER, Bjorkman PJ, et al. Solution structure of choline binding protein A, the major adhesin of Streptococcus pneumoniae. EMBO J 2005; 24: 34-43; PMID:15616594; http://dx.doi.org/10.1038/sj.emboj.7600490
- Gosink KK, Mann ER, Guglielmo C, Tuomanen EI, Masure HR. Role of novel choline binding proteins in virulence of Streptococcus pneumoniae. Infect Immun 2000; 68: 5690-5; PMID:10992472; http://dx.doi.org/10.1128/IAI.68.10.5690-5695.2000
- Quin LR, Moore QC, 3rd, Thornton JA, McDaniel LS. Peritoneal challenge modulates expression of pneumococcal surface protein C during bacteremia in mice. Infect Immun 2008; 76: 1122-7; PMID:18160474; http://dx.doi.org/10.1128/IAI.01066-07
- Bergmann S, Hammerschmidt S. Versatility of pneumococcal surface proteins. Microbiology 2006; 152: 295-303; PMID:16436417; http://dx.doi.org/10.1099/mic.0.28610-0
- Cao J, Chen D, Xu W, Chen T, Xu S, Luo J, Zhao Q, Liu B, Wang D, Zhang X, et al. Enhanced protection against pneumococcal infection elicited by immunization with the combination of PspA, PspC, and ClpP. Vaccine 2007; 25: 4996-5005; PMID:17524530; http://dx.doi.org/10.1016/j.vaccine.2007.04.069
- Glover DT, Hollingshead SK, Briles DE. Streptococcus pneumoniae surface protein PcpA elicits protection against lung infection and fatal sepsis. Infect Immun 2008; 76: 2767-76; PMID:18391008; http://dx.doi.org/10.1128/IAI.01126-07
- Gor DO, Ding X, Briles DE, Jacobs MR, Greenspan NS. Relationship between surface accessibility for PpmA, PsaA, and PspA and antibody-mediated immunity to systemic infection by Streptococcus pneumoniae. Infect Immun 2005; 73: 1304-12; PMID:15731027; http://dx.doi.org/10.1128/IAI.73.3.1304-1312.2005
- Wortham C, Grinberg L, Kaslow DC, Briles DE, McDaniel LS, Lees A, Flora M, Snapper CM, Mond JJ. Enhanced protective antibody responses to PspA after intranasal or subcutaneous injections of PspA genetically fused to granulocyte-macrophage colony-stimulating factor or interleukin-2. Infect Immun 1998; 66: 1513-20; PMID:9529075
- Mann B, Thornton J, Heath R, Wade KR, Tweten RK, Gao G, El Kasmi K, Jordan JB, Mitrea DM, Kriwacki R, et al. Broadly protective protein-based pneumococcal vaccine composed of pneumolysin toxoid-CbpA peptide recombinant fusion protein. J Infect Dis 2014; 209: 1116-25; PMID:24041791; http://dx.doi.org/10.1093/infdis/jit502
- Sanchez-Beato AR, Lopez R, Garcia JL. Molecular characterization of PcpA: a novel choline-binding protein of Streptococcus pneumoniae. FEMS Microbiol Lett 1998; 164: 207-14; PMID:9675866; http://dx.doi.org/10.1111/j.1574-6968.1998.tb13087.x
- Iannelli F, Oggioni MR, Pozzi G. Allelic variation in the highly polymorphic locus pspC of Streptococcus pneumoniae. Gene 2002; 284: 63-71; PMID:11891047; http://dx.doi.org/10.1016/S0378-1119(01)00896-4
- Fraser C, Hanage WP, Spratt BG. Recombination and the nature of bacterial speciation. Science 2007; 315: 476-80; http://dx.doi.org/10.1126/science.1127573
- Hanage WP, Fraser C, Tang J, Connor TR, Corander J. Hyper-recombination, diversity, and antibiotic resistance in pneumococcus. Science 2009; 324: 1454-7; http://dx.doi.org/10.1126/science.1171908
- Brooks-Walter A, Briles DE, Hollingshead SK. The pspC gene of Streptococcus pneumoniae encodes a polymorphic protein, PspC, which elicits cross-reactive antibodies to PspA and provides immunity to pneumococcal bacteremia. Infect Immun 1999; 67: 6533-42; PMID:10569772
- Tajima F. Statistical method for testing the neutral mutation hypothesis by DNA polymorphism. Genetics 1989; 123: 585-95; PMID: 2513255
- GAVI-Alliance. GAVI alliance commits further support for Vaccines against pneumonia and meningitis. GAVI Alliance progress report, 2008. http://www.gavi.org/Library/Publications/GAVI-Progress-reports/GAVI-Alliance-Progress-Report-2008/
- Progress in introduction of pneumococcal conjugate vaccine-worldwide, 2000-2008. MMWR Morbidity and Mortality Weekly Report 2008; 57: 1148-51; PMID:18946462
- Brueggemann AB, Pai R, Crook DW, Beall B. Vaccine escape recombinants emerge after pneumococcal vaccination in the United States. PLoS Pathog 2007; 3: e168; PMID:18020702; http://dx.doi.org/10.1371/journal.ppat.0030168
- Golubchik T, Brueggemann AB, Street T, Gertz RE, Jr., Spencer CC, Ho T, Giannoulatou E, Link-Gelles R, Harding RM, Beall B, et al. Pneumococcal genome sequencing tracks a vaccine escape variant formed through a multi-fragment recombination event. Nat Genet 2012; 44: 352-5; PMID:22286217; http://dx.doi.or/10.1038/ng.1072
- Barocchi MA, Ries J, Zogaj X, Hemsley C, Albiger B, Kanth A, Dahlberg S, Fernebro J, Moschioni M, Masignani V, et al. A pneumococcal pilus influences virulence and host inflammatory responses. Proc Natl Acad Sci U S A 2006; 103: 2857-62; PMID:16481624; http://dx.doi.org/10.1073/pnas.0511017103
- Regev-Yochay G, Hanage WP, Trzcinski K, Rifas-Shiman SL, Lee G, Bessolo A, Huang SS, Pelton SI, McAdam AJ, Finkelstein JA, et al. Re-emergence of the type 1 pilus among Streptococcus pneumoniae isolates in Massachusetts, USA. Vaccine 2010; 28: 4842-6; PMID:20434550; http://dx.doi.org/10.1016/j.vaccine.2010.04.042
- Basset A, Thompson CM, Hollingshead SK, Briles DE, Ades EW, Lipsitch M, Malley R. Antibody-independent, CD4+ T-cell-dependent protection against pneumococcal colonization elicited by intranasal immunization with purified pneumococcal proteins. Infect Immun 2007; 75: 5460-4; PMID:17698570; http://dx.doi.org/10.1128/IAI.00773-07
- Blue CE, Paterson GK, Kerr AR, Berge M, Claverys JP, Mitchell TJ. ZmpB, a novel virulence factor of Streptococcus pneumoniae that induces tumor necrosis factor a production in the respiratory tract. Infect Immun 2003; 71: 4925-35; PMID:12933834; http://dx.doi.org/10.1128/IAI.71.9.4925-4935.2003
- Kulohoma BW, Gray K, Kamng'ona A, Cornick J, Bentley SD, Heyderman RS, Everett DB. Piliation of Invasive Streptococcus pneumoniae Isolates in the Era before Pneumococcal Conjugate Vaccine Introduction in Malawi. Clin Vaccine Immunol 2013; 20: 1729-35; PMID:24027261; http://dx.doi.org/10.1128/CVI.00403-13
- Mann B, Orihuela C, Antikainen J, Gao G, Sublett J, Korhonen TK, Tuomanen E. Multifunctional role of choline binding protein G in pneumococcal pathogenesis. Infect Immun 2006; 74: 821-9; PMID:16428724; http://dx.doi.org/10.1128/IAI.74.2.821-829.2006
- Rosenow C, Ryan P, Weiser JN, Johnson S, Fontan P, Ortqvist A, Masure HR. Contribution of novel choline-binding proteins to adherence, colonization and immunogenicity of Streptococcus pneumoniae. Mol Microbiol 1997; 25: 819-29; PMID:9364908; http://dx.doi.org/10.1111/j.1365-2958.1997.mmi494.x
- McCool TL, Cate TR, Moy G, Weiser JN. The immune response to pneumococcal proteins during experimental human carriage. J Exp Med 2002; 195: 359-65; PMID:11828011; http://dx.doi.org/10.1084/jem.20011576
- Larsen MV, Cosentino S, Rasmussen S, Friis C, Hasman H, Marvig RL, Jelsbak L, Sicheritz-Pontén T, Ussery DW, Aarestrup FM, et al. Multilocus sequence typing of total-genome-sequenced bacteria. J Clin Microbiol 2012; 50: 1355-61; PMID:22238442; http://dx.doi.org/10.1128/JCM.06094-11
- Edgar RC. MUSCLE: multiple sequence alignment with high accuracy and high throughput. Nucleic Acids Res 2004; 32: 1792-97; PMID:15034147; http://dx.doi.org/10.1093/nar/gkh340
- Guindon S, Gascuel O. A simple, fast, and accurate algorithm to estimate large phylogenies by maximum likelihood. Syst Biol 2003; 52: 696-704; PMID:14530136; http://dx.doi.org/10.1080/10635150390235520
- Felsenstein J. An alternating least squares approach to inferring phylogenies from pairwise distances. Syst Biol 1997; 46: 101-11; PMID:11975348; http://dx.doi.org/10.1093/sysbio/46.1.101
- Kallberg M, Wang H, Wang S, Peng J, Wang Z, Lu H, et al. Template-based protein structure modeling using the RaptorX web server. Nat Protoc 2012; 7: 1511-22; PMID:22814390; http://dx.doi.org/10.1038/nprot.2012.085
- Rose PW, Bi C, Bluhm WF, Christie CH, Dimitropoulos D, Dutta S, Green RK, Goodsell DS, Prlic A, Quesada M, et al. The RCSB Protein Data Bank: new resources for research and education. Nucleic Acids Res 2013; 41: D475-82; PMID:23193259; http://dx.doi.org/10.1093/nar/gks1200
- Davies MN, Guan P, Blythe MJ, Salomon J, Toseland CP, Hattotuwagama C, Walshe V, Doytchinova IA, Flower DR. Using databases and data mining in vaccinology. Expert Opin Drug Discov 2007; 2: 19-35; PMID:23496035; http://dx.doi.org/10.1517/17460441.2.1.19
- Doytchinova IA, Flower DR. VaxiJen: a server for prediction of protective antigens, tumour antigens and subunit vaccines. BMC Bioinformatics 2007; 8: 4; PMID:17207271; http://dx.doi.org/10.1186/1471-2105-8-4
- Doytchinova IA, Flower DR. Identifying candidate subunit vaccines using an alignment-independent method based on principal amino acid properties. Vaccine 2007; 25: 856-66; PMID:17045707; http://dx.doi.org/10.1016/j.vaccine.2006.09.032
- Martelli PL, Fariselli P, Krogh A, Casadio R. A sequence-profile-based HMM for predicting and discriminating b barrel membrane proteins. Bioinformatics 2002; 18 Suppl 1: S46-53; PMID:12169530; http://dx.doi.org/10.1093/bioinformatics/18.suppl_1.S46