ABSTRACT
Peptide antigens are combined with an adjuvant in order to increase immunogenicity in vivo. The immunogenicity and safety of a RSV vaccine formulated in a novel oil-based platform, DepoVax™ (DPX), was compared to an alum formulation. A peptide B cell epitope derived from RSV small hydrophobic ectodomain (SHe) served as the antigen. Both vaccines induced SHe-specific antibodies after immunization of mice. A single dose of the DPX-based formulation resulted in anti-SHe titres for up to 20 weeks. Boosting with Alum-SHe, but not with DPX-SHe, led to unexpected clinical signs such as decreased activity, cyanosis and drop in body temperature in mice but not in rabbits. The severity of adverse reactions correlated with magnitude of SHe-specific IgG immune responses and decreased complement component 3 plasma levels, indicating a type III hypersensitivity reaction. By RP-HPLC analysis, we found that only 8–20% of the antigen was found to be adsorbed to alum in vitro, indicating that this antigen is likely released systemically upon injection in vivo. Clinical signs were not observed in rabbits, indicating the response correlates with peptide dose relative to size of animal. These results suggest that peptide antigens targeted to produce B cell mediated response may result in increased incidence of type III hypersensitivity reactions when delivered in non-depot forming vaccines. The DPX formulation induced strong antibody titres to the antigen without causing adverse events, likely due to the strength of the depot in vivo, and demonstrates the potential safety and immunogenicity of this platform for B cell peptide antigens.
Introduction
Many new vaccines are utilizing defined synthetic peptides or proteins as antigens rather than classical whole organism approaches, such as live attenuated or killed preparations.Citation1,Citation2 Advantages include inducing immune responses towards validated antigen targets and safety benefits of a fully synthetic vaccine.Citation3 Synthetic peptide vaccines have been researched for a variety of infectious disease indications, such as Respiratory Syncytial Virus (RSV), and for which the traditional whole organism vaccine approaches have not been successful due to poor immunogenicity or safety concerns.Citation4,Citation5
Peptides are not typically immunogenic on their own and must be formulated with an adjuvant to generate robust immune responses.Citation6 Aluminum compounds (alum) are currently the most widely used adjuvants to enhance antibody responses in both human and veterinary vaccines.Citation7 Alum-based vaccines are prepared by adsorbing antigens onto aluminum hydroxide (Alhydrogel®) or aluminum phosphate (Adju-Phos®) to form a gel suspension. The adjuvanting effect of alum was originally attributed to the formation of a depot at the site of immunization, allowing slow antigen release and exposure to the immune system. However, in the last decade studies have cast doubt on the alum depot as a primary mechanism of action.Citation8 Alum has actually been shown to directly stimulate the immune system by activating the inflammasome pathway, which may be a more important factor contributing to its adjuvant activity.Citation9 The depot effect attributed to alum's adjuvanting properties may not be as strong as once thought; the strength of the association between alum and antigen has been reported to vary, and is dependent on the physiochemical properties of the antigen used.Citation10 Therefore, the rate of clearance of different antigens can vary, and complete clearance can occur within 24 hours for some antigen types while still inducing strong antibody titres.Citation11
DepoVax™ (DPX) is a novel lipid and oil-based vaccine formulation which generates a true depot at the site of immunization. The encapsulation of all vaccine components into oil in the absence of an aqueous component means that antigens are not released from vaccine at the injection site.Citation12 DPX can also be formulated with small molecule adjuvants, such as TLR agonists, which can be used to enhance and shape the immune response. The DPX formulation is versatile and it has been formulated with a variety of different antigens and adjuvants.Citation12-Citation15 In preclinical studies, antigens formulated in DPX result in stronger and longer-lasting immune responses compared to emulsion-based vaccines.Citation12 A DPX-based formulation has been tested as a therapeutic cancer vaccine in early phase clinical studies that have demonstrated safety and immunogenicity.Citation13, Citation14
While synthetic vaccines are generally considered safer than whole organism preparations, vaccines that induce strong immune responses can also induce unintended adverse events. Hypersensitivity reactions are immune mediated responses that occur in pre-sensitized hosts. These reactions can be classified into four categories: type I, anaphylactic, mediated by IgE; type II, cytotoxic, mediated by antibodies recognizing self-antigens; type III, immune complex, caused by deposition of antigen-antibody complexes in tissues, leading to the tissue-damaging effects of complement and leukocytes (e.g. Arthus type reaction); and type IV, delayed-type hypersensitivity, which is not mediated by antibodies but by T cells.Citation16 Type III reactions may occur when re-immunization is performed while antibody titres from a prior immunization are still high.Citation17 This may be a particular concern for vaccines containing synthetic peptide antigens since they present pure and concentrated antigen. Presenting such antigens within a depot may be particular important to prevent unintended immune-based reactions.
In this study, we present preclinical evaluation of a DPX vaccine containing a synthetic peptide antigen for RSV strain A. The peptide antigen is a linear B cell epitope derived from the ectodomain of small hydrophobic protein (SHe).Citation18 The SHe peptide antigen was formulated in an alum based vaccine (Alum-SHe) and in the DPX formulation (DPX-SHe) to assess immune responses and safety in mice and rabbits. We report that the DPX-SHe vaccine induced stronger antibody titres than the Alum-SHe vaccine, with fewer doses, and the Alum-SHe vaccine was associated with increased incidences of type III hypersensitivity reaction. This data supported the clinical trial design for DPX-RSV, and identified a potential safety concern in using an alum based formulation for a synthetic peptide vaccine.
Results
SHe B cell epitope induces antigen-specific antibody responses when formulated in both Alum-based and DPX-based vaccines, but alum formulations are associated with adverse clinical signs
To compare the immunogenicity of alum based or DPX based vaccines, the SHe peptide antigen (25 µg) was formulated in either platform, Alum-SHe or DPX- SHe. Outbred CD-1 mice were vaccinated with a single dose of each vaccine or with two doses, three weeks apart. Immune responses were monitored by assessing serum antigen-specific antibodies by ELISA. After a single immunization, the DPX formulation resulted in a high antibody titre that persisted for at least 20 weeks, in comparison the alum formulation peaked at 4 weeks post immunization and gradually declined (). Mice that received two immunizations (four weeks apart) of either vaccine had persistent antibody titres up to 20 weeks post immunization, but DPX-SHe remained significantly higher than Alum-SHe (). No differences in antibody titres were observed when we compared SHe peptide formulated in aluminum phosphate (Adju-Phos) to aluminum hydroxide (Allhydrogel) (Fig. S1). Mice vaccinated with SHe peptide antigen in aqueous buffer (no DepoVax or alum) generally had the lowest antibody titres throughout the study period (Fig. S1).
Figure 1. Vaccine-induced IgG anti-SHe antibody response generated by mice. Groups of 10 female CD-1 mice were vaccinated with a single dose (A) or two doses (week 0 and 4) (B) of the DPX-SHe and Alum-SHe formulations. Immunizations are indicated with arrows. Endpoint titres, presented here as log10 values, were determined by ELISA using plates coated with SHe peptide conjugated to BSA. Results are shown as mean ± SEM. Statistics by two-way ANOVA with Bonferroni multiple comparisons post-test: *p < 0.05, **p < 0.01, ***p < 0.001.
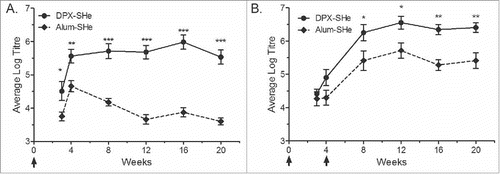
We noted that repeated vaccination with formulations containing SHe peptide antigen with alum adjuvant led to unexpected clinical signs (including decreased activity, dehydration, cyanosis, drop in body temperature, hunched body position, animal lies prone, and loss of righting reflex which leads to a terminal endpoint) in a significant number of mice. These adverse reactions occurred within an hour after vaccination (data not shown). In contrast, no adverse reactions were observed following multiple vaccinations with DPX formulated vaccines. This led us to investigate the safety profile of the alum based formulations in greater detail.
We used the outbred CD-1 strain for these studies as it can more closely recapitulate the variability expected in human subjects than inbred strains. To rule out that that the adverse events were specific to the mouse strain, we tested the effect of repeated vaccination in two other outbred mouse strains: CF-1 and J:DO (). CF-1 mice are one of a few commercially available outbred mice not descendant from the Swiss Webster mouse model (CD-1, by comparison, is a Swiss descendant). The Diversity Outbred (J:DO) stock was developed by The Jackson Laboratory. The parental lines were developed by cross breeding inbred lines and then random crossing subsequent generations. This stock is designed to be as genetically diverse as possible. Each strain of mouse received three vaccinations with the Alum-SHe vaccine, 4 weeks apart. Each of the strains generated antigen-specific antibodies, with CD-1 mice consistently generating the highest anti-SHe antibody titres, and J:DO with the lowest (). Mice were observed for clinical signs for 60 minutes following each vaccination and scored with 0 for no clinical signs, 1 for clinical signs that developed and then resolved on their own, and 2 for clinical signs requiring termination due to loss of righting reflex (). The safety profile observed in each strain differed, and generally mice with high IgG titres experienced the most severe clinical signs (Fig. S2).
Figure 2. Association between titre and toxicity between strains of outbred mice. Groups of 16 female CD-1 (squares) and 15 female CF-1 (circles) or J:DO (triangles) mice were vaccinated with three doses (day 0, 28 and 56) of Alum-SHe vaccine. (A) Endpoint titres, presented here as log10 values, were determined by ELISA. Results shown as mean ± SD. (B) Comparison of endpoint titre and severity of observed clinical signs for individual mice. Clinical signs were scored as: 0, no clinical signs; 1, non-terminal clinical signs; 2, terminal clinical signs.
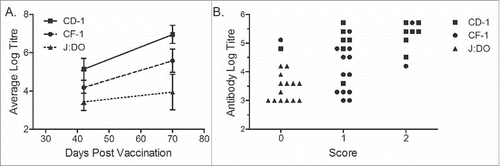
Adverse clinical signs in mice repeatedly vaccinated with alum adjuvanted vaccines are immune mediated
Development of clinical signs appeared to correlate with high antibody titres. Therefore, we investigated if an immune mediated event was responsible for the adverse reactions to boosting. The clinical signs observed upon booster immunization with Alum-SHe were consistent with either an anaphylactic IgE mediated immediate allergic response to a component of the vaccine, or a Type III hypersensitivity reaction whereby non-complexed free antigen bound circulating antibodies directed against the free antigen forming immune complexes.Citation19
CD-1 mice were immunized with Alum-SHe and boosted 11 weeks later with Alum-SHe. Serum was collected 5 days prior to boosting. After boosting, mice were observed for 60 minutes for clinical signs and euthanized if they reached endpoint. Blood samples and tissues (liver, lung, spleen and kidney) were collected on necropsy. Histological assessment of blood and tissues did not reveal any indications of cellular involvement, such as eosinophilia or mast cell infiltration (data not shown). The serum was screened for antigen-specific IgE antibodies and no significant increase in SHe-specific serum IgE was detected after boost immunization (). This indicated that an allergic reaction was not likely the cause of the adverse reactions. Previous reports have indicated that anaphylaxis-type reactions in mice correlated with increased mast cell protease (mMCPT-1) levels in blood.Citation20 In this study, we did not observe an increase in mMCPT-1 levels in mice that exhibited clinical signs post vaccination (Table S1).
Figure 3. Evaluating the mechanism of adverse clinical signs in mice. (A) Determination of IgE titres. Female CD-1 mice with an established SHe-specific IgG response were boosted with Alum-SHe vaccine. Serum was collected 5 days pre- boost and at termination due to clinical signs, where possible (within 4 hours post-boost). IgE titres determined by ELISA, ND: not determined. (B) Quantification of C3 Complement. Groups female CD-1 mice were vaccinated with two doses of DPX-SHe (n = 6) or Alum-SHe (n = 9) vaccine on study day 0 and on study day 56, indicated with (*). Plasma was collected on study day 0, 53 and 56 (two hours post boost). C3 complement activity was quantified in plasma using Mouse C3 ELISA Kit. Statistics by students t-test: *p < 0.05.
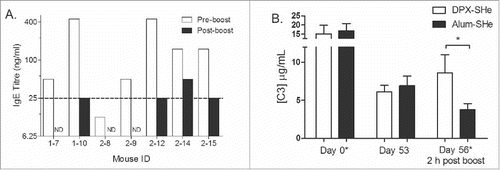
The association strength between alum and antigen is known to be variable, therefore we evaluated the association between Alum and the SHe peptide antigen in vitro. The amount of adsorption was measured by centrifuging the alum-SHe vaccines and assaying the supernatant for free peptide by RP-HPLC. Using our standard dose for the CD-1 mice experiments (25 µg SHe with 50 µg of alum), only a small fraction (8–20%) of antigen was actually complexed to the adjuvant (). This is consistent with findings by others that small proteins and peptides do not complex well to alumCitation21. This indicates that upon injection of peptide with alum, free antigen could leech from the vaccination site into systemic circulation post vaccination. In contrast, DPX-SHe vaccines were administered in an oil carrier that retains the antigen at the site of injection, which can then be accessed by antigen-presenting cells for slow trafficking of the antigen into the lymph nodes.Citation12
Table 1. Amount of alum complexation to SHe in vaccines typically giving abnormal clinical signs.
Hypersensitivity reactions have been characterized in animal models and humans by a drop in complement proteins, such as C3 and C4Citation19. Therefore, we examined the C3 levels in CD-1 mice that were vaccinated twice (SD0 and 56) with Alum-SHe. A separate group of mice was treated in parallel with DPX-SHe. Serum was collected 2 days prior to boosting and 4 hours after boosting. There was a noticeable drop in the plasma C3 levels in CD-1 mice in the hours post booster vaccination with Alum-SHe (). In contrast, C3 levels in the serum of mice that had received a boost with DPX-SHe were not reduced (). This suggests that a hypersensitivity reaction is a potential cause for the adverse clinical signs observed in these mice.
The emergence of hypersensitivity reaction in CD-1 mice is attributable to a relatively large antigen payload
We hypothesized that if the mice were experiencing a hypersensitivity reaction, it would be replicated by inducing a strong antibody titre using DPX-SHe and then boosting with free peptide. CD-1 were primed with DPX-SHe containing 25 µg peptide antigen dose and antigen-specific antibody titres were confirmed by ELISA on SD 21 and 28. Mice then received various doses of SHe peptide antigen in an aqueous buffer (25, 2.5, 0.25 and 0.025 µg) or Alum-SHe (containing 0.25 µg SHe peptide antigen) at 4, 8 and 12 weeks after the prime. Mice were monitored for clinical signs for 60 minutes following each dose ().
Table 2. Effect of antigen dilution on clinical signs upon booster vaccination in mice with an established antigen specific immune response.
All of the mice that received the 25 µg peptide dose were terminated at week 4 due to severe clinical signs. Reducing the antigen content by 10-fold (to 2.5 µg) was sufficient to reduce clinical signs and prevent endpoint in the vaccinated animals. Adverse clinical signs were not observed in the groups that received 0.25 or 0.025 µg of peptide administration. The mice that received Alum-SHe containing a lower peptide dose (0.25 µg) also did not experience severe clinical signs. We concluded that the reaction was due to providing free peptide in a boost and dependent on the amount of peptide delivered.
To evaluate if these adverse events would occur in a larger species, New Zealand White rabbits were given three intramuscular doses of 25 ug SHe peptide antigen formulated in DPX (N = 11) or in alum (N = 12). Rabbits received approximately 15 times the human dose equivalent on mg/kg basis (assuming average weighs of 4 kg and 60 kg, respectively).Citation22 For mice, the same dose is the equivalent of a 1,500 times higher dose (assuming average weights of 40 g and 60 kg). Of the total 23 rabbits immunized, 21 had strong SHe-specific IgG antibody responses at the time of booster vaccination, but no significant reactions occurred after the booster dose (). Rabbits were next administered 10-fold more SHe antigen (250 ug) in a non-adjuvanted control formulation (i.e. 100% free peptide) on study day 70. This antigen content represents 150 times the human dose on a mg/kg basis. Although a strong immune response was detect in all 23 rabbits at this time, only two rabbits exhibited a possible mild adverse reaction, characterized by a slightly hunched posture, decreased activity, and increased heart rate. Within 60 minutes all rabbits were normal without any medical intervention required and no lasting effects were noted.
Figure 4. Vaccine-induced anti-SHe antibody response generated by rabbits following vaccination. Groups of 11–12 female NZW rabbits were vaccinated with three intramuscular doses of either DPX-SHe or Alum-SHe; vaccinations occurred on days 0, 28, and 56, indicated by arrows. Endpoint titres, presented here as log10 values, were determined by ELISA. Results are shown as mean ± SEM. Results from DPX-SHe are significantly different from those of Alum-SHe at each study time point. Statistics by two-way ANOVA with Bonferroni multiple comparison post-test: *p < 0.001.
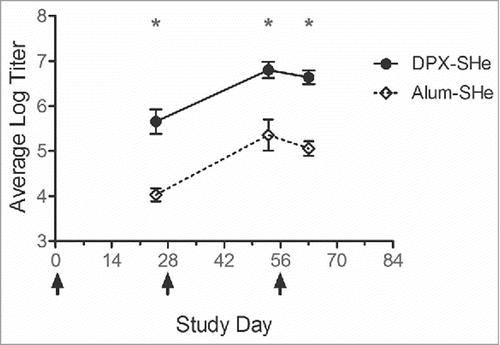
Discussion
In the development of novel vaccine adjuvants and formulations there is both a scientific and regulatory-based need for appropriate control formulations.Citation23 To advance a novel antigen and adjuvant to clinical phase testing, the formulation should be as safe as the currently accepted adjuvants and the antigen in question, and more immunogenic when formulated with the novel adjuvant. Since aluminum compounds, such as aluminum phosphate and aluminum hydroxide (commonly referred to as alum), are adjuvants used in approved vaccine formulations, they are historically added as reference adjuvants to studies of novel vaccine formulations.Citation24, Citation25 In approved vaccines formulated with alum, a significant component of its adjuvanting effect is attributed to the complexation of the antigen to the adjuvant itself. This process is highly controlled in the production of licensed alum-based vaccines, but rarely reported in preclinical studies examining adjuvants using alum as a control formulation. It has been reported that small antigens, such as synthetic peptides are difficult to fully complex to alum preparations.Citation26
In this study we evaluated a synthetic peptide antigen formulated in a novel adjuvanting platform, DepoVax, and used a standard alum based formulation for a comparator. Both formulations induced strong antibody titres, although the DPX-SHe vaccine required only a single immunization to do this. In mice, we noticed an acute hypersensitivity reaction when boosted with the alum formulation but not with the DPX formulation. We were able to experimentally eliminate the possibility of IgE-mediated hypersensitivity reactions by demonstrating the absence of correlation of the reactions with antigen-specific IgE levels or mast cell activation. The difference detected in complement C3 after alum vaccination suggested a complement mediated process. Analytical chemical analysis confirmed that the antigen was only partially (8–20%) complexed to alum. This indicated that, in mice, free antigen in the context of pre-existing antibody responses was the underlying pathogenesis, leading to a Type III hypersensitivity reaction. Furthermore, the reaction could be recapitulated by inducing IgG antibody titres with the DPX formulation and then providing free peptide, further confirming the mechanism. In mice, the severity of the reaction was dependent on the dose of free peptide provided. In rabbits, the severity of the reaction was substantially abrogated although they also generated strong anti-SHe titres upon vaccination, indicating that the dose per animal weight is also a factor contributing to the severity of the reaction. In humans, a type III reaction in response to vaccination presents as temporary pain and swelling at the site of injection, peaking at 12–36 hours after immunization and resolving completely over a few days.Citation16
These data demonstrate how a typically rare phenomenon could be possible when alum is used to adjuvant novel peptide antigen vaccines, and presents challenges in testing novel vaccine technologies in animal models. In contrast to the B cell epitope evaluated in this study, to date, the majority of novel peptide based antigens have targeted T cell epitopes. The negative outcomes of overdosing antigen in murine models thus is potentially less apparent in the absence of strong circulating antibody responses. In the analysis of novel vaccines, it is not uncommon to adjust the delivered doses to mice to compensate for the size difference between mice and humans. However, it is also common to deliver the full human dose in smaller animal models to demonstrate safety of a vaccine in the presence of excess antigen.Citation27, Citation28 In our mouse studies of SHe antigen, adverse signs occurred following administration of a large dose of free peptide or peptide in alum, after a significant immune response had already been generated. We hypothesize that this hypersensitivity reaction is unlikely to occur in larger animals or in humans who would receive a much lower dose of peptide on a mg/kg basis. Indeed, for this reason, rabbits are generally considered a better model for vaccine safety testing.Citation27
This work was focused on the RSV SHe peptide in order to support a clinical study and did not evaluate other B cell epitopes, which is a study limitation. We are continuing to explore this effect using other peptides and have found that this phenomenon is not universally demonstrated. Similar findings have been reported by Quakkelaar et al who found that a synthetic long peptide formulated in an aqueous buffer induced hypersensitivity reactions, but other similar length peptides did not.Citation29 This indicates that the peptide sequence may influence the development, and perhaps even severity, of type III hypersensitivity responses after vaccination. Due to the small size of the peptide in this study, and hence with limited available antibody-binding sites, we hypothesize that this type of reaction would not occur to the same extent with larger peptides or proteins with multiple epitopes available. For this reason, it would also be unlikely that a reaction would occur in patients with pre-existing antibody titres to RSV due to previous infection if they were vaccinated with the SHe peptide antigen, since antibodies specific to SHe peptide have been reported to be at low levels after natural infection.Citation18
The lack of adverse reactions in the animals vaccinated and boosted with DPX provides preclinical evidence of the safety of this delivery system for B cell epitope antigens. The depot formed by DPX prevented leaching of the peptide so that none was available to complex with the induced SHe specific antibodies. The extreme reactions exhibited by the mice may be attributed to using a full human dose in a murine model, however these observations highlight the importance of using an appropriate delivery system for novel antigens. Careful analysis of the underlying cause of such unexpected adverse outcomes in mice can be a sensitive indicator of potential reactions that could occur in humans. Currently, a DepoVax based RSV vaccine, called DPX-RSV(A), has completed a Phase 1 clinical trial, evaluating its safety in a healthy adult population (manuscript in preparation). This data supported the clinical trial design for DPX-RSV(A) by identifying a potential safety concern in the Alum-based control group.
Materials and methods
Animals
All mice used in studies were outbred strains. Pathogen-free, 3–8 week old female CD-1 (strain code: 022) and CF-1 (strain code: 023) mice were obtained from Charles River Laboratories (St Constant, QC, Canada); 3 week old J:DO mice were obtained from The Jackson Laboratory (stock: 009376). Mice were housed in ventilated micro-isolator caging and provided food and water ad libitum. Virus antibody free, 9–12 week old female New Zealand White rabbits were obtained from Charles River Laboratories. Rabbits were group housed in dedicated runs and provided food and water ad libitum. Species appropriate environmental enrichment was supplied. Dalhousie University animal care and use guidelines were followed for all experiments. Experiments were approved by the University Committee on Laboratory Animals and strictly followed the guidelines set by The Canadian Council on Animal Care (CCAC).
Peptides
The peptide antigen was derived from a small hydrophobic ectodomain protein (SHe) of RSV group A (NKLCEYNVFHNKTFELPRARVNT) and custom synthesized by GenScript and PolyPeptide Group. The native sequence peptides contain a single cysteine residue and so, to ensure uniformity across all formulations, were dimerized by reconstituting powdered stock in 10% DMSO, 0.5% acetic acid solution and incubating at 37°C overnight. Dimer formation was confirmed by HPLC (≥ 95% area%) and LC-MS analysis.
For ELISA assays the SHe peptide antigen was modified to include a C terminal linker sequence and was then conjugated to bovine serum albumin (BSA) protein. Briefly, BSA (A0281, Sigma) was activated with buffered Sulfo-SMCC (Thermo Scientific) then mixed with peptide. The pH was adjusted to 6.0 and the mixture incubated at 4°C for 3 hours, with gentle shaking for 30 seconds at 15 minute intervals. The conjugated protein was then dialyzed overnight at 4°C against sterile water before use in coating ELISA assay plates.
Vaccine preparation and immunization
Alum-based vaccines were formulated by mixing dimerized peptide with appropriate buffer and then combining with Adju-Phos (3471, Brenntag) or Alhydrogel (843161, Brenntag) wet gel suspensions. Each 0.05 ml dose contained 0.025 mg dimerized peptide and 0.05 mg of alum.
DPX vaccines were formulated with the adjuvant Pam3CSK4 (L2000, Polypeptide Group).Citation30 Briefly, peptide dimer and adjuvant were solubilized in sterile water and mixed with a previously prepared dioleoylphosphatidyl choline (DOPC) and cholesterol mixture (Lipoid GmBH) in appropriate buffer. The lipid mixture containing peptide dimer and adjuvant was then lyophilized to a dry cake and reconstituted with Montanide ISA 51 VG (36362Z, SEPPIC) just prior to injection. Each 0.05 ml dose contained 0.025 mg dimerized peptide and 0.002 mg Pam3CSK4 adjuvant.
In some experiments, animals were challenged with dimerized peptide in 10 mM sodium acetate buffer (pH 7.0) at indicated doses.
Vaccines were administered via intramuscular injection of 0.025 ml on each the right and left caudal thigh muscle in mice, 0.05 ml dose in total. Rabbits received a full 0.05 ml dose in the caudal thigh muscle.
Determination of clinical signs
Immediately and for 60 minutes after vaccination, animals were monitored for adverse reactions and observations recorded by a dedicated technician at 10–15 minute intervals. Any animal that became prone (lying on the cage floor) was immediately laid on its back for evaluation of the righting reflex. This was repeated about every 5 minutes until the righting reflex was lost (animal was euthanized) or the animal's condition began to improve. Any animals not completely recovered more than 60 minutes post vaccination, were monitored until they had recovered. All animals were checked again for clinical signs 2 hours post vaccination and then once daily for 3 days. Endpoint animals were terminated by exsanguination under isoflurane anesthesia. Clinical signs were scored as: 0, no clinical signs; 1, non-terminal clinical signs; 2, terminal clinical signs.
Antigen-specific ELISA
Blood samples were collected by submandibular venipuncture in mice and via the marginal ear vein in rabbits and serum separated by centrifugation after blood was allowed to clot. Antigen-specific antibody endpoint titres were determined by ELISA. Briefly, plates were coated overnight with 1 μg/ml of SHe antigen conjugated to bovine serum albumin. After blocking with 3% gelatin, serial dilutions of the serum were and plates were incubated overnight at 4°C. Next day, bound antibody was detected using an alkaline phosphatase-linked Protein G (for mice; 539305, Calbiochem) or Protein A (for rabbits; P7488, Sigma) used as the secondary detection reagent. Endpoint titres were defined as the reciprocal of the highest dilution above the cutoff value; cutoff values were calculated using a 95% confidence interval.Citation31 Titres were converted to a log10 value and group averages determined. Comparisons between groups were made using a one way ANOVA with Tukey's multiple comparison post-test.
Antigen-specific IgE titres were determined as described above using rat anti-mouse IgE conjugated to alkaline phosphatase (1130–04, Southern Biotech) as a secondary antibody.
Plasma assays
Whole blood was collected by submandibular venipuncture into K2EDTA tubes (CABD365974L, BD Biosciences) and plasma separated by centrifugation. Circulating complement component 3 (C3) and mast cell protease levels were detected and quantified by ELISA with commercially available kits following the manufacturer's instructions. Complement was detected using Mouse C3 (Complement Factor 3) ELISA Kit (GWB-7555C7, Genway Biotech) and mast cell protease was detected using MCPT-1 (mMCP-1) ELISA Ready-SET-Go!® kit (88-7503-86, eBioscience).
Determination of antigen complexation to alum
SHe peptide and alum samples were gently agitated, and 0.2 ml was transferred to a microfuge tube. The tubes were centrifuged at 5000 x g for 2 minutes to produce a clear supernatant solution. The supernatants were analyzed by RP-HPLC on an Agilent 1100 HPLC system, with a gradient of water/acetonitrile/trifluoroacetic acid, a Phenomenex Luna C8(2), 5 µm, 4.6 × 150 mm column, with UV detection at 215 nm. The solutions were quantified against an external SHe antigen standard of known concentration. The degree of adsorption was calculated as: (Amount Expected-Amount Found) / Amount Expected x 100%.
Abbreviations
DPX | = | DepoVax™ |
RSV | = | Respiratory Syncytial Virus |
SHe | = | ectodomain of small hydrophobic protein |
Disclosure of potential conflicts of interest
The authors LM, AM, VK, AP, RR, GW, MM and MS are employees of Immunovaccine, Inc.
Contributions
LM, AM, VK, RR, AP, MM, MS contributed to the conception and/ or design of the work. LM, AM, VK RR acquired data. LM, AP, RR, GW, MM, JL, SH, MS analyzed and interpreted data. LM, GW, MS wrote the manuscript. All authors contributed to critically revising the work and agree to be accountable for all aspects.
Supplemental_Material.zip
Download Zip (76.8 KB)Acknowledgments
The authors acknowledge the expert technical assistance of Andrea West, Nicolas Bartlett, Kendall Sharp, Arthvan Sharma, and Leeladhar Sammatur. We thank Bert Schepens and Xavier Saelens for valuable discussions.
Funding
This research did not receive any specific grant from funding agencies in the public, commercial, or not-for-profit sectors.
References
- Koff WC, Burton DR, Johnson PR, Walker BD, King CR, Nabel GJ, Ahmed R, Bhan MK, Plotkin SA. Accelerating next-generation vaccine development for global disease prevention. Science. 2013;340:1232910. doi:10.1126/science.1232910. PMID:23723240
- Lewis DJ, Lythgoe MP. Application of “systems vaccinology” to evaluate inflammation and reactogenicity of adjuvanted preventative vaccines. J Immunol Res. 2015;2015:909406. doi:10.1155/2015/909406. PMID:26380327
- Moyle PM, Toth I. Modern subunit vaccines: development, components, and research opportunities. ChemMedChem. 2013;8:360-76. doi:10.1002/cmdc.201200487. PMID:23316023
- Di Pasquale A, Preiss S, Tavares Da Silva F, Garcon N. Vaccine Adjuvants: from 1920 to 2015 and Beyond. Vaccines (Basel). 2015;3:320-43. doi:10.3390/vaccines3020320. PMID:26343190
- Graham BS. Biological challenges and technological opportunities for respiratory syncytial virus vaccine development. Immunol Rev. 2011;239:149-66. doi:10.1111/j.1600-065X.2010.00972.x. PMID:21198670
- Reed SG, Orr MT, Fox CB. Key roles of adjuvants in modern vaccines. Nat Med. 2013;19:1597-608. doi:10.1038/nm.3409. PMID:24309663
- O'Hagan DT, Fox CB. New generation adjuvants–from empiricism to rational design. Vaccine. 2015;33(Suppl 2):B14-20. doi:10.1016/j.vaccine.2015.01.088. PMID:26022561
- Wen Y, Shi Y. Alum: an old dog with new tricks. Emerg Microbes Infect. 2016;5:e25. doi:10.1038/emi.2016.40. PMID:27004761
- Li H, Willingham SB, Ting JP, Re F. Cutting edge: inflammasome activation by alum and alum's adjuvant effect are mediated by NLRP3. J Immunol. 2008;181:17-21. doi:10.4049/jimmunol.181.1.17. PMID:18566365
- al-Shakhshir RH, Regnier FE, White JL, Hem SL. Contribution of electrostatic and hydrophobic interactions to the adsorption of proteins by aluminium-containing adjuvants. Vaccine. 1995;13:41-4. doi:10.1016/0264-410X(95)80009-3. PMID:7762276
- Hutchison S, Benson RA, Gibson VB, Pollock AH, Garside P, Brewer JM. Antigen depot is not required for alum adjuvanticity. FASEB J. 2012;26:1272-9. doi:10.1096/fj.11-184556. PMID:22106367
- Brewer KD, Lake K, Pelot N, Stanford MM, DeBay DR, Penwell A, Weir GM, Karkada M, Mansour M, Bowen CV. Clearance of depot vaccine SPIO-labeled antigen and substrate visualized using MRI. Vaccine. 2014;32:6956-62. doi:10.1016/j.vaccine.2014.10.058. PMID:25444822
- Berinstein NL, Karkada M, Morse MA, Nemunaitis JJ, Chatta G, Kaufman H, Odunsi K, Nigam R, Sammatur L, MacDonald LD, et al. First-in-man application of a novel therapeutic cancer vaccine formulation with the capacity to induce multi-functional T cell responses in ovarian, breast and prostate cancer patients. J Transl Med. 2012;10:156. doi:10.1186/1479-5876-10-156. PMID:22862954
- Berinstein NL, Karkada M, Oza AM, Odunsi K, Villella JA, Nemunaitis JJ, Morse MA, Pejovic T, Bentley J, Buyse M, et al. Survivin-targeted immunotherapy drives robust polyfunctional T cell generation and differentiation in advanced ovarian cancer patients. Oncoimmunology. 2015;4:e1026529. doi:10.1080/2162402X.2015.1026529. PMID:26405584
- MacDonald LD, Fuentes-Ortega A, Sammatur L, Mansour M. Efficacy of a single dose hepatitis B depot vaccine. Vaccine. 2010;28:7143-5. doi:10.1016/j.vaccine.2010.08.084. PMID:20832491
- Committee IoMUVS. Adverse Events Associated with Childhood Vaccines: Evidence Bearing on Causality. Washington,(DC: National Academies Press (US), 1994.
- Siegrist CA. Mechanisms underlying adverse reactions to vaccines. J Comp Pathol. 2007;137(Suppl 1):S46-50. doi:10.1016/j.jcpa.2007.04.012. PMID:17559869
- Schepens B, Sedeyn K, Vande Ginste L, De Baets S, Schotsaert M, Roose K, Houspie L, Van Ranst M, Gilbert B, van Rooijen N, et al. Protection and mechanism of action of a novel human respiratory syncytial virus vaccine candidate based on the extracellular domain of small hydrophobic protein. EMBO Mol Med. 2014;6:1436-54. doi:10.15252/emmm.201404005. PMID:25298406
- Leach MW, Rottman JB, Hock MB, Finco D, Rojko JL, Beyer JC. Immunogenicity/hypersensitivity of biologics. Toxicol Pathol. 2014;42:293-300. doi:10.1177/0192623313510987. PMID:24240973
- Khodoun MV, Strait R, Armstrong L, Yanase N, Finkelman FD. Identification of markers that distinguish IgE- from IgG-mediated anaphylaxis. Proc Natl Acad Sci U S A. 2011;108:12413-8. doi:10.1073/pnas.1105695108. PMID:21746933
- Kurella S, Manocha M, Sabhnani L, Thomas B, Rao DN. New age adjuvants and delivery systems for subunit vaccines. Indian J Clin Biochem. 2000;15:83-100. doi:10.1007/BF02867548. PMID:23105272
- Reagan-Shaw S, Nihal M, Ahmad N. Dose translation from animal to human studies revisited. FASEB J. 2008;22:659-61. doi:10.1096/fj.07-9574LSF. PMID:17942826
- Krishnan L, Twine S, Gerdts V, Barreto L, Richards JC. Canadian Adjuvant Initiative Workshop, March 26–27, 2013–Ottawa, Canada. Hum Vaccin Immunother. 2014;10:519-26. doi:10.4161/hv.26972. PMID:24192752
- Brito LA, O'Hagan DT. Designing and building the next generation of improved vaccine adjuvants. J Control Release. 2014;190:563-79. doi:10.1016/j.jconrel.2014.06.027. PMID:24998942
- Schijns VE, Lavelle EC. Trends in vaccine adjuvants. Expert Rev Vaccines. 2011;10:539-50. doi:10.1586/erv.11.21. PMID:21506650
- Li W, Joshi MD, Singhania S, Ramsey KH, Murthy AK. Peptide Vaccine: Progress and Challenges. Vaccines (Basel). 2014;2:515-36. doi:10.3390/vaccines2030515. PMID:26344743
- Brennan FR, Dougan G. Non-clinical safety evaluation of novel vaccines and adjuvants: new products, new strategies. Vaccine. 2005;23:3210-22. doi:10.1016/j.vaccine.2004.11.072. PMID:15837222
- Forster R. Study designs for the nonclinical safety testing of new vaccine products. J Pharmacol Toxicol Methods. 2012;66:1-7. doi:10.1016/j.vascn.2012.04.003. PMID:22561062
- Quakkelaar ED, Fransen MF, van Maren WW, Vaneman J, Loof NM, van Heiningen SH, Verbeek JS, Ossendorp F, Melief CJ. IgG-mediated anaphylaxis to a synthetic long peptide vaccine containing a B cell epitope can be avoided by slow-release formulation. J Immunol. 2014;192:5813-20. doi:10.4049/jimmunol.1302337. PMID:24813207
- Karkada M, Weir GM, Quinton T, Sammatur L, MacDonald LD, Grant A, et al. A novel breast/ovarian cancer peptide vaccine platform that promotes specific type-1 but not Treg/Tr1-type responses. J Immunother. 2010;33:250-61. doi:10.1097/CJI.0b013e3181c1f1e9. PMID:20445345
- Frey A, Di Canzio J, Zurakowski D. A statistically defined endpoint titer determination method for immunoassays. J Immunol Methods. 1998;221:35-41. doi:10.1016/S0022-1759(98)00170-7. PMID:9894896