ABSTRACT
Diabetic nephropathy (DN) is a complication of diabetes that induces the development of end-stage renal disease (ESRD). The pathogenesis of DN is reported to be closely related to the activation of the NOD-like receptor 3 (NLRP3) inflammasome in renal glomerular endothelial cells. Omarigliptin is a novel dipeptidyl peptidase-4 (DPP-4) inhibitor developed for the management of type II diabetes, it has been recently reported to possess a significant anti-inflammatory property. This study aims to explore the potential therapeutic effects of Omarigliptin on DN. We established an in vitro injury model in human renal glomerular endothelial cells (HrGECs) using high glucose (HG). The severe cytotoxicity and increased oxidative stress in HrGECs induced by HG were pronouncedly reversed by the introduction of Omarigliptin. Furthermore, the activated NLRP3 inflammasome and the excessive production of interleukin 18 (IL-18) and interleukin 1β (IL-1β) in HrGECs induced by incubation with HG were pronouncedly reversed by the introduction of Omarigliptin, accompanied by the activation of the AMPK/mTOR signaling pathway. After the co-administration of the adenosine monophosphate-activated protein kinase α (AMPKα) inhibitor, compound C, the protective effects of Omarigliptin against HG-induced NLRP3 inflammasome activation and production of pro-inflammatory factors were dramatically abolished. Taken together, our data revealed that Omarigliptin ameliorated HG-induced inflammation in renal glomerular endothelial cells through suppressing NLRP3 inflammasome activation mediated by AMPKα.
Introduction
Diabetes mellitus (DM) has become a global health problem. According to reports, by 2019, the number of DM patients was 463 million, and it is expected to reach 578 million by 2030 [Citation1]. Previous studies show that about 30–40% of patients with DM get diabetic nephropathy (DN) [Citation2]. DN is a common and severe complication of diabetes with mild upregulation of microalbuminuria reported to be the main characteristic, in its early stages, gradually developing to the production of mass albuminuria and impaired renal function [Citation3]. As a consequence, end-stage renal disease (ESRD) develops [Citation4]. In the past decades, DN has become the main inducer of renal failure and an increase in DN epidemicity has been reported [Citation5]. DN is now the world’s second most common cause of ESRD. Therefore, it is critical to investigate the pathogenesis of DN and explore its potential therapeutic methods. The glomerular filtration barrier plays an important role in controlling the release of urine proteins and is composed of endothelial cells, basal membrane, and podocytes, which allow the filtration of small molecules and limits the passing of proteins [Citation6]. Renal glomerular endothelial cells are a group of specific capillary endothelial cells that are involved in the synthesis and repairment of basal membrane components and regulate the glomerular coagulation, immune response, and inflammatory processes. In recent years, it has been widely reported that glomerular endothelial cell dysfunction caused by inflammatory factors is related to the pathogenesis of DN. Sabena M. Conley reported that in the progression of DN, the pathological progression of glomerular sclerosis could be facilitated by severe inflammation, which eventually contributes to the development of ESRD. The NLRP3 inflammasome, a multimeric protein complex, holds a pivotal role in the inflammatory responses mediated by the innate immune. When activated, NLRP3 inflammasome is responsible for the production of IL-1β and IL-18 by cleaving prointerleukin-1β (pro-IL-1β) and prointerleukin-18 (pro-IL-18), which directly promote the inflammatory cascade reaction [Citation7]. Recently, studies have shown that NLRP3 inflammasome also plays an important part in the development of DM and diabetes complications including DN [Citation8,Citation9]. Furthermore, glomerular inflammation and damages to renal glomerular endothelial cells can be induced by NLRP3 inflammasome activation to trigger the glomerular injury and ESRD. NLRP3 inflammasome not only functions on immune cells but also on the renal glomerular endothelial cells and podocytes. It is reported that the infiltration of monocytes and CD8+ T cells can be induced by the transplanted glomerular inflammation and the high level of secreted inflammatory factors, such as IL-1β, and IL-18, will be induced by the activation of monocytes, which contributes to the renal glomerular endothelial injury by cytotoxicity and the local activation of the coagulation cascade. AMPK/mTOR signaling is reported to be an important pathway that negatively regulates the activation of NLRP3 inflammasome [Citation10]. Therefore, targeting NLRP3 inflammasome might be a promising approach for the treatment of DN.
Dipeptidyl peptidase-4 (DPP-4) is identified as a complex enzyme, expressed in a wide range of cell types, including endothelial, epithelial cells, and activated lymphocytes [Citation11]. DPP-4 possesses various biological functions by regulating its abundant substrates, including glucagon-like peptide-1 (GLP-1). A class of DPP-4 inhibitors has been licensed for the management of diabetes mellitus by blocking DPP4 mediated cleavage of the incretin hormone, GLP-1, and GIP, prolonging its insulinotropic activity [Citation12]. Omarigliptin is an effective, highly selective, and reversible inhibitor of DPP-4 developed by Merck to treat type II diabetes [Citation13]. With the advantages of a longer in vivo half-life, Omarigliptin is widely reported to exert promising hypoglycemic properties. Recently, significant anti-inflammatory effects have been observed during the treatment of type II diabetes patients using Omarigliptin. However, whether Omarigliptin exerts protective benefits in a complication of diabetes such as DN is still unknown. In this study, we aim to examine the potential effects of Omarigliptin against high glucose (HG) brought injury on human renal glomerular endothelial cells (HrGECs) and further investigate the possible therapy for the treatment of DN in the clinic.
Materials and methods
Cell culture and treatment
HrGECs were obtained from ScienCell Research (ScienCell, California, USA) and cultured in the endothelial cell medium (ECM) (ScienCell, California, USA) supplemented with 5% FBS at 37℃ and 5% CO2. Cells with passages three to five were used in this study. For the inducement of inflammatory injury on HrGECs, cells were treated with 25 mM high glucose (HG) [Citation14]. To examine the cytotoxicity of Omarigliptin in HrGECs, cells were stimulated with 0, 1, 2, 10, 20, 100, and 200 μM Omarigliptin for 24 hours.
Cell counting kit-8 (CCK-8) assay
Cell viability of treated HrGECs was determined with a CCK-8 assay. To determine the cell viability, HrGECs were mixed with 10 µL CCK-8 solution and incubated at 37°C for 2 hours, followed by measuring the absorbance at 450 nm using a microplate reader (J&H technology Co., Ltd, Jiangsu, China) [Citation15].
Mito SOX Red staining
The mitochondrial level of reactive oxygen species (ROS) was measured using the Mito SOX Red staining assay. Briefly, HrGECs were cultured using the endothelial culture medium (ECM) containing 5 μM MitoSox Red and incubated for 10 minutes at 37°C. After washing, the images of HrGECs were taken using the fluorescence microscope (Leica, Weztlar, Germany).
Lactate dehydrogenase (LDH) release
The cytotoxicity of treated HrGECs was measured with the LDH release assay. After necessary treatment, cell cultural supernatant was collected and cells were incubated with 1% Triton X-100 for 45 minutes to release total LDH. The reaction was initiated by adding a reaction buffer. After incubating in the dark for 30 minutes, the absorbance at 490 nm was detected using the microplate reader (J&H technology Co., Ltd, Jiangsu, China). The ratio of LDH in the cell cultural supernatant to total LDH was calculated to index the percentage of LDH release.
Real-time PCR
Following extracting the total RNAs from the treated HrGECs using the trizol reagents (Invitrogen, California, USA), quantification on RNAs was conducted using the NanodropTM 2000 c UV-Vis Spectrophotometer (Thermo, Massachusetts, USA). Then, the Taq PCR Master Mix Kit (QIAGEN, North Rhine-Westphalia, Germany) was applied for the reverse transcription from RNA to cDNA, followed by conducting the RT-PCR with the SYBR Master Mix kit (Bio-Rad, California, USA) in the Bio-Rad CFX96-new system (Bio-Rad, California, USA). Lastly, the 2−ΔΔCt method was used for the determination of the relative gene expression level of target genes following normalization using the expression of GAPDH. The following primers were used in this study:
NLRP3(Forward)5´-GGACTGAAGCACCTGTTGTGCA −3´,
(Reverse)5´- TCCTGAGTCTCCCAAGGCATTC −3´;
GAPDH(Forward)5´- AGCCTCAAGATCATCAGCAA −3´,
(Reverse)5´- GTCATGAGTCCTTCCACGAT −3´;
NOX-2(Forward)5´- CCTTTTACCTATGTGCCGGAC −3´,
(Reverse)5´;- CATGTGATGTGTAGAGTCTTGCT −3´;
mTOR(Forward)5´- TCCAGGGCTTCTTCCGTT −3´,
(Reverse)5´- GGGCTGTCGTGGTAGACTTAG −3´;
ASC(Forward)5´- CTACCTGGAGACCTACGGCG −3´,
(Reverse)5´- TTTCCGGTAGAGCAGCTTTGT −3´.
Western blot
After isolating the total proteins from the treated HrGECs, a bicinchoninic acid (BCA) protein assay kit (Merck, New Jersey, USA) was utilized to determine the concentration of proteins and about 40 μg proteins were loaded, followed by being separated with the 12% SDS-PAGE. Then, the proteins in the SDS-PAGE were transferred to the PVDF membrane (Merck, New Jersey, USA) and the membrane was subsequently incubated with 5% BSA for 2 hours, followed by being incubated with the primary antibody against NADPH oxidase-2 (NOX-2) (1:1200, Zymed, California, USA), NLRP3 (1:1500, Zymed, California, USA), apoptosis-associated speck-like protein containing a CARD (ASC) (1:800, Zymed, California, USA), p-AMPKα (1:500, Zymed, California, USA), AMPKα (1:1600, Zymed, California, USA), mTOR (1:1500, Zymed, California, USA), and β-actin (1:8000, Zymed, California, USA) overnight. The membrane was exposed to enhanced chemiluminescence (ECL) solution (Invitrogen, California, USA) after 1.5 hours of incubation in secondary antibody solution (1:2000, Zymed, California, USA) and the relative expression level of target proteins was confirmed by visualization with Image J software [Citation16].
Enzyme-linked immunosorbent assay (ELISA) assay
ELISA assay was used for the determination of the production of interleukin-18 (IL-18) and interleukin-1β (IL-1β) in treated HrGECs. Briefly, after centrifugation, the supernatant was collected and transferred into a 96-well plate, followed by incubation in 1% BSA for the removal of nonspecific binding proteins. Then, the primary antibody of IL-18 or IL-1β was added, followed by 3 washes and incubation with the streptavidin-HRP conjugated secondary antibody for half an hour. After adding the TMB solution for 20 minutes, the reaction was terminated using the stop buffer. Lastly, the fluorescence microscope (Leica, Weztlar, Germany) was utilized to measure the absorbance at 450 nm.
Statistical analysis
Data are expressed as mean ± standard deviation (S.D.) and analyzed using the GraphPad Prism 8.0 software. All data were tested for normal distribution using the Shapiro-Wilk test. Data with normal distributions were compared using a two-way analysis of variance (ANOVA) followed by Tukey’s post-hoc test. Nonparametric data were analyzed with the Kruskal–Wallis H test. P < 0.05 was regarded as a statistically significant difference.
Results
In this study, we proposed Omarigliptin as a promising therapy for DN and explored the underlying mechanism. Using a HG-challenged HrGECs model, we report that Omarigliptin reduced the expression of IL-1β and IL-18 by inhibiting the NLRP3 inflammasome.
Cytotoxicity of omarigliptin in HrGECs
To explore the optimized concentration of Omarigliptin in HrGECs, cells were stimulated with 0, 1, 2, 10, 20, 100, and 200 μM Omarigliptin for 24 hours. As shown in , compared to the control, no significant difference was found as the dosage of Omarigliptin increased from 1 to 20 μM. Nevertheless, cell viability significantly declined when the concentration of Omarigliptin reached 100, and 200 μM. Hence, 10 μM, and 20 μM Omarigliptin were used to explore its pharmacological function in HrGECs.
Omarigliptin ameliorated high glucose-induced cytotoxicity in HrGECs
Cells were challenged with HG and 10, and 20 μM Omarigliptin for 24 hours. The morphology of HrGECs is shown in . Compared to the control, cell viability () was decreased by incubation with HG but was significantly promoted by the introduction of Omarigliptin. In addition, compared to the control, LDH release () was elevated from 5.6% to 25.7% in the HG group but greatly suppressed to 17.2% and 12.8% by treatment with 10, and 20 μM Omarigliptin, respectively. These data indicate that Omarigliptin exerted promising protective effects on HG-induced cytotoxicity in HrGECs.
Figure 2. Omarigliptin ameliorated high glucose (HG)-induced cytotoxicity in HrGECs. Cells were challenged with HG and 10, and 20 μM Omarigliptin for 24 hours. (a). Morphology of HrGECs; (b). Cell viability; (c). LDH release (**, ***, P < 0.01, 0.005 vs. vehicle; #, ##, ###, P < 0.05, 0.01, 0.005 vs. HG)
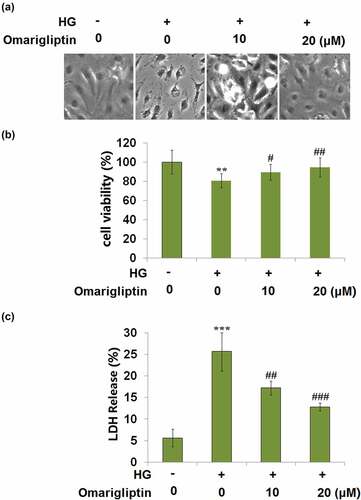
Omarigliptin mitigated high glucose-induced mitochondrial oxidative stress in HrGECs
To investigate the effects of Omarigliptin on oxidative stress in high glucose-treated HrGECs, the gene and protein expression levels of NOX-2 were evaluated. As shown in , compared to the control, NOX-2 was significantly upregulated by incubation with HG but significantly downregulated by 10, and 20 μM Omarigliptin. In addition, the elevated mitochondrial ROS production in HG-stimulated HrGECs was dramatically decreased by treatment with Omarigliptin. These data indicate that mitochondrial oxidative stress in HG-stimulated HrGECs was pronouncedly alleviated by Omarigliptin.
Omarigliptin alleviated HG-induced NLRP3 inflammasome activation
Furthermore, we investigated the activity of the NLRP3 inflammasome. As shown in , compared to the control, the expression levels of NLRP3 and ASC were dramatically upregulated by treatment with HG, which was pronouncedly inhibited by 10, and 20 μM Omarigliptin. These data indicate that the activated NLRP3 inflammasome in HrGECs induced by HG was pronouncedly ameliorated by Omarigliptin.
Figure 4. Omarigliptin alleviated high glucose-induced NLRP3 inflammasome activation. (a). NLRP3 and ASC mRNA; (b). NLRP3 and ASC protein (***, P < 0.005 vs. vehicle; ##, ###, P < 0.01, 0.005 vs. HG)
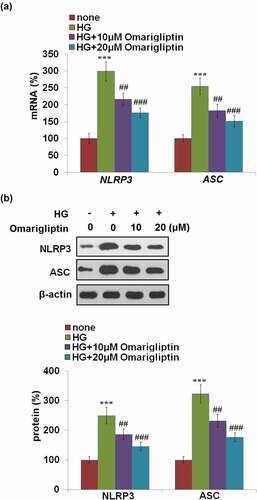
Excessive activation of NLRP3 inflammasome results in the production of IL-18 and IL-1β. Subsequently, the production of these two inflammatory factors was detected. As illustrated in , the concentration of IL-18 was significantly increased from 67.5 pg/mL to 188.7 pg/mL by stimulation with HG but greatly suppressed to 137.8 pg/mL and 102.9 pg/mL by treatment with 10, and 20 μM Omarigliptin, respectively. In addition, the secretions of IL-1β in the control, HG, 10, and 20 μM Omarigliptin groups were 92.5, 297.1, 216.5, and 173.6 pg/mL, respectively. These data reveal that the activated NLRP3 inflammasome in HrGECs induced by HG was significantly mitigated by Omarigliptin (). Consistently, the inhibitory effects of Omarigliptin in the protein expression of IL-18 and IL-1β against HG were confirmed by western blot analysis ().
The protective effects of Omarigliptin against high glucose-induced NLRP3 inflammasome activation were mediated by AMPK
To explore the potential underlying mechanism, the activity of the AMPK/mTOR signaling pathway in HrGECs was further evaluated. As shown in , compared to the control, the expression level of p-AMPKα was significantly declined and the expression level of mTOR was greatly elevated by stimulation with HG, but dramatically reversed by treatment with 20 μM Omarigliptin, indicating that the AMPK/mTOR signaling pathway in HG-treated HrGECs was dramatically restored by Omarigliptin.
Figure 6. Omarigliptin restored high glucose-induced impairment of the AMPK/mTOR signaling pathway. Cells were challenged with high glucose (HG) and 20 μM Omarigliptin for 2 hours. The expression of p-AMPKα, AMPKα, and mTOR was measured (***, P < 0.005 vs. vehicle; ##, P < 0.01 vs. HG)
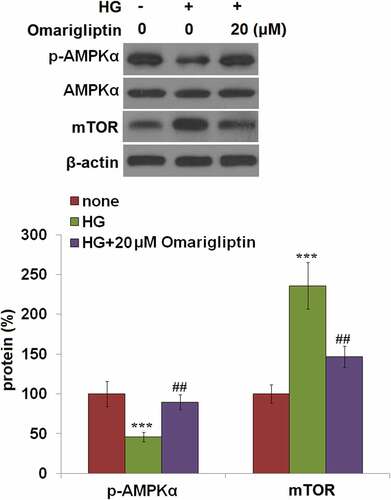
We further confirmed the involvement of AMPK/mTOR pathway in the protective effects of Omarigliptin by introducing an inhibitor of AMPKα, compound C. Cells were stimulated with HG, 20 μM Omarigliptin, and compound C. As shown in , the elevated expression levels of mTOR and NLRP3 in the HG group were obviously inhibited by treatment with Omarigliptin and dramatically reversed by the co-incubation of compound C. In addition, the secretion of IL-18 () in HG-challenged HrGECs was decreased from 193.5 pg/mL to 112.9 pg/mL by the introduction of Omarigliptin but greatly promoted to 178.6 pg/mL by the co-administration of compound C. The concentration of IL-1β in the control, HG, Omarigliptin, and Omarigliptin + compound C groups were 103.7, 322.8, 168.6, and 281.3 pg/mL, respectively. These data reveal that the protective effects of Omarigliptin against high glucose-induced NLRP3 inflammasome activation were mediated by the activation of the AMPK signaling pathway.
Figure 7. The protective effects of Omarigliptin against high glucose-induced NLRP3 inflammasome activation are mediated by AMPK. Cells were challenged with high glucose (HG) and 20 μM Omarigliptin or the AMPK inhibitor compound C for 24 hours. (a). The expression of mTOR and NLRP3; (b). Secretions of IL-18 and IL-1β as measured with ELISA (***, P < 0.005 vs. vehicle; ###, P < 0.005 vs. HG; $$, P < 0.01 vs. HG+ Omarigliptin)
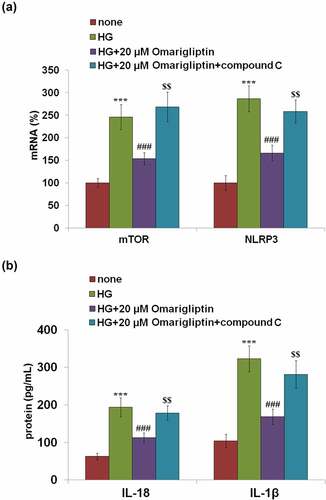
Discussion
High glucose-induced HrGECs insults are an early event of DN. Amelioration of these damages has become a promising strategy for the treatment of DN. In this study, we demonstrated the beneficial effects of Omarigliptin against high glucose-induced inflammatory insults by suppressing the activation of the NLRP3 inflammasome.
Under the condition of diabetes, increased glucose load is observed in the kidney, accompanied by the excessive release of ROS, activated polyol pathway, and upregulated injury mediators [Citation17]. The excessive accumulation of ROS can activate a series of signal molecules that cause kidney injury [Citation18]. During the progression of diabetes, pathological changes in the kidney, such as glomerular hypertrophy, increased extracellular matrix synthesis, glomerular fibrosis, destruction of the tubular basement membrane, and interstitial infiltration mediated tubular interstitial fibrosis, are gradually observed eventually contributing to the development of DN [Citation19,Citation20]. In the present study, HG was used to establish an in vitro damage model in renal glomerular endothelial cells and was verified by the declined cell viability and activated oxidative stress. After treatment with Omarigliptin, the decreased cell viability and oxidative stress in HG-treated HrGECs were remarkably alleviated, suggesting a potential beneficial effect of Omarigliptin against hyperglycemia-induced damage on endothelial cells. The production of pro-inflammatory factors, IL-1β and IL-18, is reported to be upregulated after the activation of the NLRP3 inflammasome, and they are the two critical pro-inflammatory factors that impact the adaptive immunity, regulate the inflammatory reaction, and enhance the expression of VCAM-1 and E-cadherin [Citation21]. The permeability of endothelial cells can be enlarged by the stimulation of IL-1β, which further changes the hemodynamics of the glomerulus, impacts the synthesis of prostaglandin, induces the proliferation of fibroblasts, and facilitates the generation of TGF-β [Citation22]. The secretions of TNF-α, IL-6, and VCAM-1 by monocytes and macrophages can be triggered by IL-18 further inducing tubulointerstitial fibrosis, reducing the glomerular filtration rate, and eventually contributing to the development of kidney failure [Citation23]. In this study, the activation of NLRP3 inflammasome and increased production of IL-1β and IL-18 were observed in HG-treated HrGECs but were dramatically reversed by treatment with Omarigliptin, indicating that the protective effect of Omarigliptin might be closely related to the inhibition of NLRP3 inflammasome.
As a serine/threonine protein kinase, AMPK mainly functions as the ‘energetic regulator’ in eucaryotic organisms [Citation24]. The synthesis of ATP is blocked and the metabolic pathway of ATP is triggered by the activation of AMPK, which maintains the cellular energetic balance [Citation25]. It is reported that the intracellular energetic signals can be accumulated to mTOR by AMPK. When the production of ATP declines, the activity of mTOR is suppressed by the activated AMPK to enhance the progression of autophagy [Citation26]. Recently, it has been reported that the AMPK/mTOR signaling pathway is closely involved in regulating the activation of NLRP3 inflammasome [Citation10]. In the present study, we found that the AMPK/mTOR signaling pathway was significantly activated by Omarigliptin. After the co-administration of the AMPK inhibitor, the protective effects of Omarigliptin against high glucose-induced NLRP3 inflammasome activation and excessively released inflammatory factors were dramatically abolished, indicating that Omarigliptin might protect the HG-induced injury on renal glomerular endothelial cells by activating the AMPK/mTOR pathway. In our future work, the participation of the AMPK/mTOR pathway will be further confirmed by knocking down the expression of AMPK in HrGECs. The therapeutic effects of Omarigliptin on DN will be further verified by administering the DN animal model with an appropriate dosage of Omarigliptin.
Choosing a single type of cell model (HrGECs), using only two doses of Omarigliptin (10 and 20 μM), and selecting the 24 hours are the limitations of this study. DN is a long-term, chronic, and multifactorial disease. The pathological mechanism of which is complex. Several regulators have been involved in the initiation and progression of DN. In addition to high glucose, free fatty acids (FFAs) levels are reported to be increased in the blood of humans with T2D and mediate inflammatory cascades transduction through activating the NF-κB-dependent signaling pathway. Importantly, FFAs are implicated as putative triggers of the NLRP3 inflammasome [Citation27]. Interestingly, another study reported that insulin serves as a negative regulator of NLRP3 inflammasome activation by preventing the assembly of the ASC [Citation28]. However, the precise molecular mechanisms regulating NLRP3 inflammasome are not completely understood and are intensively debated. Therefore, conducting in vivo studies with more doses of Omarigliptin and longer duration to make a more comprehensive interpretation about the clinical success of this drug, determining the function of the glomerular filtration barrier, and evaluating the clinical follow-up study results together will provide more reliable results.
Conclusion
Our data, taken together, reveals that Omarigliptin ameliorated HG-induced inflammation in renal glomerular endothelial cells through suppressing NLRP3 inflammasome activation mediated by the AMPKα/mTOR signaling pathway.
Author contribution statement
Ling Li and Xinyu Hong contributed to the conception; Ling Li, Kelei Qian, Yuli Sun, Yong Zhao contributed to the investigation; Ling Li, Yun Zhou1, Ying Xue contributed to the preparation of materials. Xinyu Hong drafted the manuscript. All the authors have read and approved the final version of the submission.
Acknowledgements
This study is funded by the “The National Natural Science Foundation of China (Grant No.81500589, 81974105)”.
Disclosure statement
No potential conflict of interest was reported by the author(s).
Data availability statement
Data are available upon reasonable request to the corresponding author.
Additional information
Funding
References
- Jung SW, Moon JY. The role of inflammation in diabetic kidney disease. Korean J Intern Med. 2021;36(4):753–766.
- Lim AK. Diabetic nephropathy–complications and treatment. Int J Nephrol Renovascular Dis. 2014;7:361. .
- Bhattacharjee N, Barma S, Konwar N, et al. Mechanistic insight of diabetic nephropathy and its pharmacotherapeutic targets: an update. Eur J Pharmacol. 2016;791:8–24.
- Mauer M, Doria A. Uric acid and diabetic nephropathy risk. Contrib Nephrol. 2018;192:103–109.
- Zeng O, Li F, Li Y, et al. Effect of Novel Gasotransmitter hydrogen sulfide on renal fibrosis and connexins expression in diabetic rats. Bioengineered. 2016;7(5):314–320. .
- Haraldsson B, Nystrom J, Deen WM. Properties of the glomerular barrier and mechanisms of proteinuria. Physiol Rev. 2008;88(2):451–487.
- Kelley N, Jeltema D, Duan Y, et al. The NLRP3 inflammasome: an overview of mechanisms of activation and regulation. Int J Mol Sci. 2019;20(13):3328. .
- Yu ZW, Zhang J, Li X, et al. A new research hot spot: the role of NLRP3 inflammasome activation, a key step in pyroptosis, in diabetes and diabetic complications. Life Sci. 2019;240:117138.
- Gao C, Chen J, Fan F, et al. RIPK2-mediated autophagy and negatively regulated ROS-NLRP3 inflammasome signaling in GMCs stimulated with high glucose. Mediators Inflamm. 2019;2019:6207563. doi: 10.1155/2019/6207563.
- Wang Q, Wei S, Zhou S, et al. Hyperglycemia aggravates acute liver injury by promoting liver-resident macrophage NLRP3 inflammasome activation via the inhibition of AMPK/mTOR-mediated autophagy induction. Immunol Cell Biol. 2020;98(1):54–66. .
- Yang F, Qin Y, Wang Y, et al. Metformin Inhibits the NLRP3 Inflammasome via AMPK/mTOR-dependent Effects in Diabetic Cardiomyopathy. Int J Biol Sci. 2019;15(5):1010–1019. .
- Yazbeck R, Jaenisch SE, Abbott CA. Dipeptidyl peptidase 4 inhibitors: applications in innate immunity. Biochem Pharmacol. 2021;188:114517.
- Krishna R, Addy C, Tatosian D, et al. Pharmacokinetics and pharmacodynamics of omarigliptin, a once-weekly dipeptidyl peptidase-4 (DPP-4) inhibitor, after single and multiple doses in healthy subjects. J Clin Pharmacol. 2016;56(12):1528–1537. .
- Ndibalema AR, Kabuye D, Wen S, et al. Empagliflozin protects against proximal renal tubular cell injury induced by high glucose via regulation of hypoxia-inducible factor 1-alpha. Diabetes Metab Syndr Obes. 2020;13:1953–1967.
- Zhu DZ, Shi CF, Jiang YN, et al. Cisatracurium inhibits the growth and induces apoptosis of ovarian cancer cells by promoting lincRNA-p21. Bioengineered. 2021;12(1):1505–1516. .
- Wang JP, Lv P. Chrysophanol inhibits the osteoglycin/mTOR and activats NF2 signaling pathways to reduce viability and proliferation of malignant meningioma cells. Bioengineered. 2021;12(1):755–762.
- Lu Q, Wang WW, Zhang MZ, et al. ROS induces epithelial-mesenchymal transition via the TGF-beta1/PI3K/Akt/mTOR pathway in diabetic nephropathy. Exp Ther Med. 2019;17(1):835–846.
- Alicic RZ, Rooney MT, Tuttle KR. Diabetic Kidney Disease: challenges, Progress, and Possibilities. Clin J Am Soc Nephrol. 2017;12(12):2032–2045.
- Dai H, Liu Q, Liu B. Research progress on mechanism of podocyte depletion in diabetic nephropathy. J Diabetes Res. 2017;2017:2615286. doi: 10.1155/2017/2615286.
- Adamiak M, Lenkiewicz AM, Cymer M, et al. Novel evidence that an alternative complement cascade pathway is involved in optimal mobilization of hematopoietic stem/progenitor cells in Nlrp3 inflammasome-dependent manner. Leukemia. 2019;33(12):2967–2970. .
- Du L, Dong F, Guo L, et al. Interleukin-1beta increases permeability and upregulates the expression of vascular endothelial-cadherin in human renal glomerular endothelial cells. Mol Med Rep. 2015;11(5):3708–3714. .
- Wu M, Han W, Song S, et al. NLRP3 deficiency ameliorates renal inflammation and fibrosis in diabetic mice. Mol Cell Endocrinol. 2018;478:115–125.
- Carling D. AMPK signaling in health and disease. Curr Opin Cell Biol. 2017;45:31–37.
- Wang Q, Liu S, Zhai A, et al. AMPK-mediated regulation of lipid metabolism by phosphorylation. Biol Pharm Bull. 2018;41(7):985–993. .
- Alers S, Loffler AS, Wesselborg S, et al. Role of AMPK-mTOR-Ulk1/2 in the regulation of autophagy: cross talk, shortcuts, and feedbacks. Mol Cell Biol. 2012;32(1):2–11. .
- Wang H, Zhong P, Sun L. Exogenous hydrogen sulfide mitigates NLRP3 inflammasome-mediated inflammation through promoting autophagy via the AMPK-mTOR pathway. Biol Open. 2019;8(7):bio043653. doi: 10.1242/bio.043653.
- Legrand-Poels S, Esser N, L’homme L, et al. Free fatty acids as modulators of the NLRP3 inflammasome in obesity/type 2 diabetes. Biochem Pharmacol. 2014;92(1):131–141. .
- Chang YW, Hung LC, Chen YC, et al. Insulin reduces inflammation by regulating the activation of the NLRP3 inflammasome. Front Immunol. 2021;11:587229.