ABSTRACT
Circular RNAs (circRNAs) have been extensively studied in various diseases, including sepsis-induced acute kidney injury (AKI). This research intended to elucidate the mechanism of circular RNA HIPK3 (circHIPK3) in sepsis-engendered AKI. Human tubule epithelial cells (HK2) were stimulated with lipopolysaccharide (LPS) to establish a septic AKI cell model. The gene expression levels were evaluated by RT-qPCR. Cell viability, apoptosis, and cell cycle distribution were assessed through CCK-8 and flow cytometry assays. The potential interactions between genes were verified by luciferase reporter and RIP assays. The results displayed that circHIPK3 expression was enhanced in septic AKI patients and LPS-triggered HK2 cells. Moreover, circHIPK3 interference expedited HK2 cell viability and attenuated apoptosis, inflammatory and oxidative damages following LPS stimulation. Furthermore, circHIPK3 functioned as a molecular sponge for miR-338, and forkhead box A1 (FOXA1) was negatively regulated by miR-338. CircHIPK3 aggravated cell injury in LPS-treated HK2 via targeting miR-338, and FOXA1 addition overturned the suppressing impacts of miR-338-3p augmentation on LPS-activated HK2 cell damage. Finally, we demonstrated that circHIPK3 modulated LPS-induced cell damage via the miR-338/FOXA1 axis. In sum, our results elaborated that circHIPK3 knockdown attenuated LPS-triggered HK2 cell injury by regulating FOXA1 expression via interacting with miR-338, suggesting that circHIPK3 might be a potential biomarker and therapeutic target for sepsis-induced AKI patients.
GRAPHICAL ABSTRACT
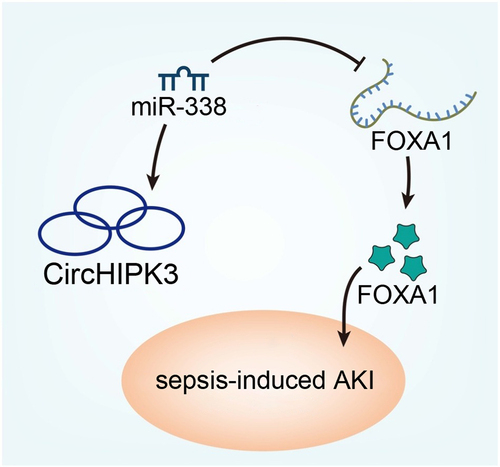
Introduction
Sepsis is a life-threatening disease characterized by systemic inflammatory response syndrome [Citation1,Citation2]. It is estimated that 18 million new sepsis cases occur each year worldwide with a high fatality rate ranging from 30% to 50% [Citation3,Citation4]. A growing number of researches displayed that sepsis could accelerate the release of inflammatory factors in renal tissues, resulting in renal cell apoptosis, which led to acute kidney injury (AKI) [Citation5,Citation6]. AKI caused by sepsis is closely associated with high mortality of up to 60–80% [Citation7]. Although extensive studies have explored the pathogenesis of sepsis-induced AKI, its pathophysiological mechanisms are still not fully understood [Citation8]. Therefore, providing new insight into the pathogenesis of sepsis-engendered AKI is vital for developing effective therapies for this disease.
Increasing studies have shown that the aberrant expression of circular RNAs (circRNAs) may exert vital biological functions in sepsis. For example, Xiong et al. reported that circ_0003420 mediated lipopolysaccharide (LPS)-induced cell viability and inflammation through decreasing NPAS4 expression in sepsis-associated liver injury [Citation9]. Hong et al. reported that the upregulation of circFADS2 restrained LPS-triggered lung cell apoptosis via repressing miR-15a-5p maturation in sepsis [Citation10]. In addition, the vital function of circRNAs has been identified in AKI. For instance, Shi et al. observed that highly expressed circPRKCI relieved LPS-induced HK2 cell injury through regulating the miR-545/ZEB2 axis [Citation11]. Circ_0114427 facilitated LPS-induced septic AKI by regulating miR-495-3p/TRAF6 via the NF-kappaB pathway [Citation12]. Recently, circular RNA HIPK3 (circHIPK3) has been reported to aggravate cardiac dysfunction in LPS-induced mice models and apoptosis in cardiomyocytes [Citation13]. However, the role and regulatory mechanism of circHIPK3 in sepsis-induced AKI needs further investigation.
In this study, we aimed to investigate the regulatory mechanism of circHIPK3 in sepsis-induced AKI. We assumed that circHIPK3 might regulate forkhead box A1 (FOXA1) expression by sponging miR-338 in sepsis-induced AKI, thus providing a theoretical basis for the treatment of sepsis-induced AKI.
Materials and methods
Serum specimens
Human serum samples were recruited from 23 septic AKI patients and 23 healthy subjects at Huzhou first people’s Hospital. This research was approved by the ethics committee of Huzhou first people’s Hospital, and written informed consent was acquired from all participants. The inclusion criteria for patients are as follows: Body temperature (<36°C or >38.5°C), heart rate (> 90 beats/min), tachypnea (>20 breaths/min or PaCO2 < 32 mmHg), leukopenia (< 4000/mm3), and leukocytosis (> 12,000/mm3). The exclusion criteria were the presence of malignancy, pregnancy in female patients, chronic renal insufficiency, use of nephrotoxic drugs, or receiving any kind of renal replacement therapy.
Cell culture and treatment
Human tubule epithelial cells (HK2) were bought from Cell Bank of the Chinese Academy and kept in DMEM medium (Invitrogen) plus 10% FBS (Procell) and 1% penicillin/streptomycin (Procell) at 37°C with 5% CO2.
HK2 cells were treated with different concentrations of LPS (0, 2.5, 5, and 10 µg/mL) for 24 h, and HK2 cells were treated with 5 µg/mL LPS for different times (0, 6, 12, and 24 h). To generate a septic model in vitro, HK2 cells were exposed to 5 µg/mL LPS (Sigma-Aldrich) for 12 h [Citation14].
Cell transfection
pcDNA3.1 vector expressing circHIPK3 (pcDNA3.1/circHIPK3), pcDNA3.1/FOXA1 and empty pcDNA3.1 vector, small interfering RNA (siRNA) against circHIPK3 (si-circHIPK3) with its control (si-NC), and miR-338 mimics/inhibitor with their controls (NC mimics/inhibitor) were obtained from GenePharma (Shanghai). Lipofectamine 2000 (Invitrogen) was utilized for the transfection.
CCK-8 assay
HK2 cells (1 × 104 cells/well) were incubated in 96-well plates. Subsequently, 10 μL CCK-8 reagent (Dojindo, Japan) was added and incubated for another 2 h. By using a microplate reader, the absorbance at 450 nm was detected [Citation15].
RT-qPCR
Total RNA from serums and cells was extracted using the TRIzol reagent (Invitrogen) and then cDNA was synthesized by a PrimeScript RT reagent Kit (Takara, Japan). RT-qPCR was performed with an SYBR Premix Ex Taq II (Takara). Relative levels of genes were evaluated with the 2−ΔΔCt method [Citation16], with U6 or GAPDH as internal references.
Luciferase reporter assay
The mutant (Mut) or wild-type (WT) sequence of circHIPK3 or FOXA1 was subcloned into pmirGLO vector (Promega) to construct circHIPK3-mut/wt or FOXA1-mut/wt reporters. The vectors were co-transfected with miR-338 mimics or NC mimics into HK2 cells, and the luciferase activity was monitored by Dual-Luciferase Reporter Assay System (Promega) [Citation17].
Flow cytometry assay
The apoptosis and cell cycle distribution were assessed via flow cytometry. For cell apoptosis, the treated cells were collected, rinsed with PBS, resuspended in 200 μL Annexin V-binding buffer, and then stained with Annexin V-FITC and PI for 15 minutes in darkness. The apoptotic cells were measured by an FCM flow cytometer with FlowJo software (BD Bioscience, USA) [Citation18].
For the examination of cell cycle distribution, transfected HK2 cells were harvested and fixed in 70% ethanol at 4°C. Then, the fixed were treated with RNase in PBS and stained with PI.
ELISA
The concentrations of IL-1β and TNF-α were evaluated using the corresponding ELISA kits (Beyotime) [Citation19].
Oxidative stress assay
The contents of malondialdehyde (MDA) and superoxide dismutase (SOD) were determined to assess cell oxidative stress. Briefly, following LPS treatment, the levels of MDA and SOD were examined using the SOD and MDA assay kits (Abcam, Cambridge, UK) [Citation14].
RIP assay
RIP experiment was tested using the Magna RIP RNA-Binding Protein Immunoprecipitation Kit (Millipore). HK2 cells were lysed in RIP lysis buffer, which was conjugated with anti-Ago2 (Abcam) or anti-IgG conjugated with magnetic beads (Sigma-Aldrich). Immunoprecipitated RNA was purified and tested using RT-qPCR [Citation20].
Western blot
Proteins were lyzed by RIPA lysis buffer (Beyotime). Then, total protein was separated by 10% SDS-PAGE and transferred onto PVDF membranes (Millipore). Then, the membranes were incubated with primary antibodies against FOXA1 and GAPDH overnight at 4°C, and further incubated with secondary antibodies for 2 h. The bands were evaluated using ECL detection kit (Sigma-Aldrich).
Statistical analysis
All experiments were performed at least three times, and data were presented as mean ± SD. SPSS 21.0 (IBM Corp.) was adopted for statistical analysis. Student’s t-test or one-way ANOVA followed by Tukey’s test was used to estimate the difference between groups. Correlation between genes was analyzed by Pearson’s correlation analysis. When p < 0.05, there was a statistical difference.
Results
This work was designed to investigate the function of circHIPK3 in sepsis-induced AKI. Our results demonstrated that circHIPK3 deletion could alleviate LPS-mediated apoptosis, inflammatory, and oxidation stress injuries by regulating FOXA1 expression via targeting miR-338. This study indicated that circHIPK3 might be employed as a potential target for the treatment of sepsis-induced AKI.
CircHIPK3 expression is elevated in septic AKI
Firstly, circHIPK3 expression was assessed in the septic AKI patients, and RT-qPCR experimental data implied that circHIPK3 level was remarkably lifted in serum of septic AKI patients (, p < 0.001). Next, HK2 cells were stimulated with different LPS concentrations, and RT-qPCR analysis showed that circHIPK3 level was enhanced by LPS treatment in unequal doses (). In addition, the expression of circHIPK3 was notably increased in a time-dependent manner (). The stability and localization of circHIPK3 were then investigated in HK2 cells. A comparative analysis of circHIPK3 and HIPK3 mRNA expression was performed in HK2 cells that had been treated with actinomycin D. The stability analysis of circHIPK3 and the corresponding linear HIPK3 in HK2 cells showed that the half-life of circHIPK3 was more than 24 h, whereas that of linear HIPK3 was less than 8 h, indicating circHIPK3 was highly stable (). In addition, the results of nuclear-cytoplasmic fractionation illustrated that circHIPK3 was predominantly localized in the cytoplasm (). These results demonstrated that circHIPK3 was a stable circular RNA and highly expressed in septic AKI.
Figure 1. CircHIPK3 expression is elevated in septic AKI patients and LPS-triggered HK2 cells.
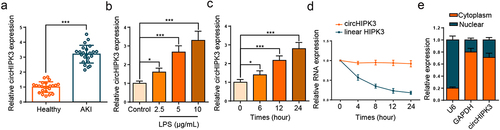
Interference of circHIPK3 alleviated sepsis-induced AKI in vitro
To determine the function of circHIPK3 in HK2 cells stimulated by LPS, we knocked down circHIPK3. The transfection efficiency of circHIPK3 is presented in . Then, HK2 cell viability was gradually declined by LPS treatment with the increase of LPS concentration (). Moreover, the inhibition of cell proliferation stimulated by LPS was neutralized by circHIPK3 knockdown in HK2 cells (). Further, flow cytometry exhibited that HK2 cell apoptosis was accelerated by LPS treatment and cell cycle was arrested at G0/G1 stage; however, circHIPK3 deletion neutralized these impacts (). In addition, ELISA results unveiled that IL-1β and TNF-α levels were lifted following LPS treatment, which was reversed by circHIPK3 silence (). Subsequently, we analyzed oxidative indicators to monitor oxidative stress, and the results uncovered that LPS could decline SOD and raise MDA level, suggesting that LPS treatment could promote oxidative stress, while circHIPK3 deficiency offset these effects (). In sum, these findings suggested that circHIPK3 silence relieved LPS-triggered HK2 cell damage.
Figure 2. Interference of circHIPK3 expedites viability and suppresses apoptosis and inflammation in LPS-induced HK2 cells.
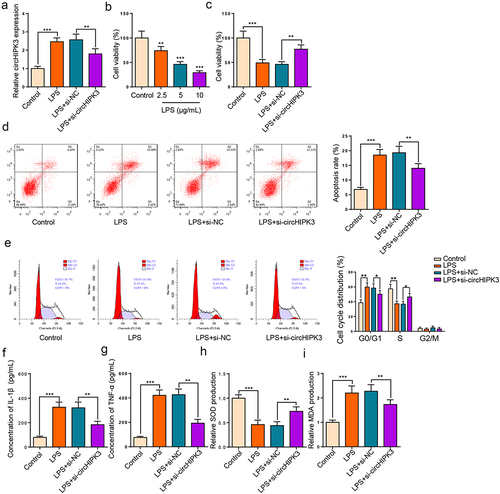
CircHIPK3 targeted miR-338 in HK2 cells
To find potential targets of circHIPK3, we used circinteractome website (https://circinteractome.nia.nih.gov) to screen the downstream miRNAs with the potential binding ability for circHIPK3. As depicted in , miR-338 was predicted as a downstream gene of circHIPK3. Moreover, the activity was inhibited in HK2 cells transfected with circHIPK3-wt and miR-338 mimics, while there was no evident impact in the cells with circHIPK3-mut (). RIP experiment elaborated that circHIPK3 and miR-338 were enriched in anti-Ago2 group of HK2 cells (). Thereafter, RT-qPCR assay elaborated that miR-338 level was downregulated in serum of septic AKI patients (, p < 0.001). Meanwhile, we found that circHIPK3 level was negatively related to miR-338 (). Moreover, LPS treatment repressed miR-338 level in a dose-dependent manner (). Furthermore, circHIPK3 addition aggravated the elevated circHIPK3 level induced by LPS (). In addition, circHIPK3 silence elevated miR-338 level, while circHIPK3 augmentation decreased miR-338 level in LPS-activated HK2 cells (). Collectively, circHIPK3 bound to and negatively modulated miR-338.
Figure 3. CircHIPK3 targeted miR-338 in HK2 cells.
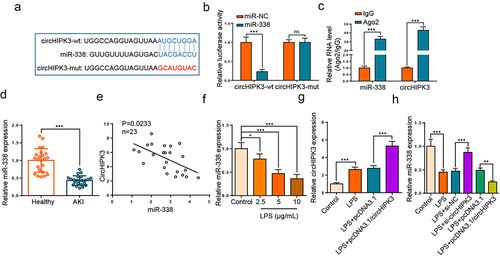
miR-338 suppression rescues the impacts of circHIPK3 interference on LPS-engendered HK2 cell damage
To further investigate whether circHIPK3 regulated LPS-caused HK-2 cell injury via targeting miR-338, si-circHIPK3 or si-circHIPK3+ miR-338 inhibitor was introduced into LPS-triggered HK-2 cells. As depicted in , miR-338 level was drastically curbed after its repression. Meanwhile, circHIPK3 repression enhanced miR-338 level, which was declined by miR-338 blocking in LPS-treated HK2 cells (). Functionally, circHIPK3 deletion restored LPS-caused the changes of cell viability and apoptosis, while these changes were reversed by miR-338 silence (). Meanwhile, circHIPK3 deficiency could restrain cell cycle progress in HK2 cells stimulated by LPS, which was mitigated by miR-338 reduction (). Further, ELISA assay elaborated that the diminution of IL-1β and TNF-α levels induced by circHIPK3 deletion in LPS-triggered HK2 cells was assuaged by miR-338 deletion (). Additionally, miR-338 repression neutralized the impacts of circHIPK3 interference on SOD and MDA levels (). As a result, circHIPK3 interference relieved LPS-activated HK2 cell injury via miR-338.
Figure 4. miR-338 suppression rescues the impacts of circHIPK3 interference on LPS-engendered HK2 cell damage.
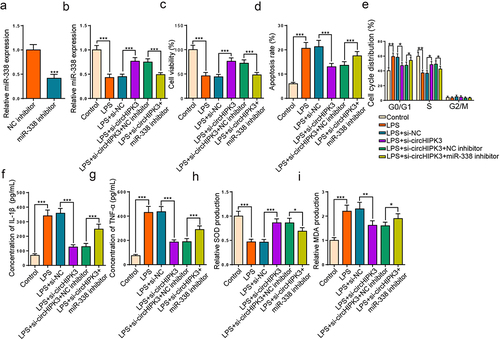
FOXA1 directly target miR-338
Through searching starBase website, FOXA1 was considered to bind with miR-338 (). Then, we manifested that the activity of FOXA1-wt was reduced by miR-338 addition, but had no effects on that of FOXA1-mut (). Moreover, RIP experiment confirmed that FOXA1 and miR-338 were enriched in Ago2 complexes (). Furthermore, the upregulation of FOXA1 was noticed in the serums of septic AKI patients (, p < 0.001). As expected, FOXA1 level was inversely associated with miR-338 in sepsis patients (). In addition, Western blotting demonstrated that LPS stimulation enhanced FOXA1 level in HK2 cells (). In addition, the level of FOXA1 was declined following circHIPK3 deletion in LPS-triggered HK2 cells, however, the impact was restored by miR-338 repression (). The aforementioned findings implied that miR-338 inversely modulated FOXA1 level by direct interaction.
Figure 5. FOXA1 directly target miR-338.
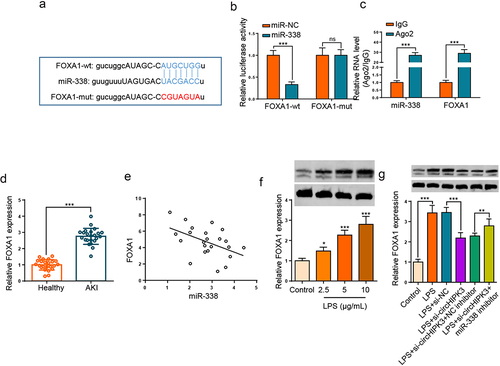
miR-338 augmentation alleviates LPS-resulted HK2 cell injury via FOXA1
Then, we further investigated whether the regulatory role of miR-338 in LPS-triggered HK2 cell damage could be mediated by regulating FOXA1. RT-qPCR manifested that augmentation of miR-338 declined FOXA1 level in LPS-triggered HK2 cells, while FOXA1 augmentation over-turned this effect (). Functional assays unveiled that the miR-338 addition boosted cell proliferation, cell cycle progression, and impeded apoptosis and inflammatory factor levels were counteracted by enhancing FOXA1 level in LPS-triggered HK2 cells (). Additionally, FOXA1 addition offset the changes of miR-338 supplementation on SOD and MDA levels (). Collectively, results verified that miR-338 elevation attenuated LPS-injured HK2 cell damage through modulating FOXA1.
Figure 6. miR-338 augmentation alleviates LPS-resulted HK2 cell injury via FOXA1.
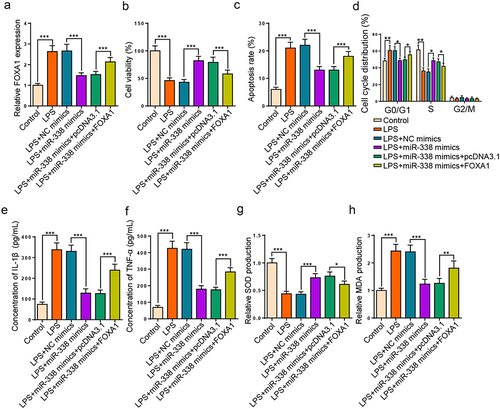
Discussion
Sepsis-caused AKI has become a global health concern and can cause shock and death [Citation21]. Multiple evidence indicated that inflammatory response is involved in the development of septic AKI [Citation22,Citation23]. The levels of pro-inflammatory factors such as IL-6, TNF-α, and IL-1β have been proven to be related to the severity of septic AKI [Citation24]. Oxidative stress refers to the imbalance between the production of oxygen-free radicals and the counteracting of its harmful effects by endogenous antioxidants, resulting in irreversible tissue damage [Citation25,Citation26]. Inflammatory factors and oxidative stress can directly facilitate apoptosis, further aggravating sepsis-induced AKI [Citation27]. Herein, we explored the function of circHIPK3 in septic AKI by establishing septic AKI cell model in vitro.
Many literatures displayed that circRNAs were involved in the development of sepsis-triggered AKI. Xu et al. unveiled that circTLK1 regulated inflammation and oxidative stress via targeting miR-106a-5p and modulating HMGB1 level to contribute to sepsis-triggered AKI [Citation28]. Wei et al. reported that circ_0068,888 facilitated the viability and inhibited inflammatory response to improve sepsis-associated AKI via targeting miR-21-5p [Citation29]. Tan et al. revealed that the addition of circ_0091702 boosted cell viability, restrained the inflammation of LPS-caused HK2 cells by modulating the miR-545-3p/THBS2 axis in septic AKI [Citation30]. CircHIPK3 (has_circ 0000284) is a circular RNA derived from the second exon of the HIPK3 gene, it was reported that circHIPK3 was enhanced in several organs, such as renal and lung [Citation31,Citation32], and acted as an essential modulator in oxidative damage and inflammation [Citation33,Citation34]. Consistent with the previous studies, we uncovered the upregulation of circHIPK3 in serums of septic AKI patients and LPS-treated HK2 cells. Moreover, circHIPK3 blocking neutralized the LPS-induced repression of HK2 cell viability and acceleration of apoptosis, inflammatory reaction, and oxidative stress. Therefore, the above data revealed that circHIPK3 deficiency could alleviate LPS-engendered HK2 cell damage.
It has been acknowledged that circRNAs can competitively bind to miRNAs to modulate mRNAs and thus take part in the pathogenesis of various diseases [Citation35]. miR-338 was uncovered to be decreased in LPS-treated bronchial epithelial cells, and regulated by circ_0038467 to participate in LPS-induced inflammatory injury [Citation36]. In addition, miR-338 was also demonstrated to be downregulated in AKI [Citation37]. Herein, we found that circHIPK3 could bind to miR-338, and miR-338 expression was decreased in septic AKI patients. In addition, circHIPK3 was negatively correlated with miR-338 level. To study the regulatory mechanism of circHIPK3/miR-338 axis, functional experiments were conducted and the results showed that miR-338 silencing restored si-circHIPK3-induced effects on HK2 cell injury. Hence, these results demonstrated that circHIPK3 participated in LPS-triggered AKI via targeting miR-338.
FOXA1, a member of FOXA family, has been reported to play a vital role in various pathological processes, including inflammation, cell viability, and apoptosis [Citation38–40]. Moreover, Lu et al. elaborated that FOXA1 expression was lifted in sepsis-induced AKI patients and promoted HK2 cell apoptosis [Citation41]. In this study, we proved that FOXA1 was negatively regulated by miR-338, and FOXA1 level was increased in septic AKI. Functional assays elaborated that FOXA1 augmentation eliminated the effects of miR-338 addition on LPS-disposed HK2 damage. Moreover, circHIPK3 served as a miR-338 sponge to regulate FOXA1 expression. These data revealed the vital function of the circHIPK3/miR-338/FOXA1 axis in sepsis-induced AKI.
Conclusion
Our study demonstrated that circHIPK3 interference ameliorated apoptosis, inflammatory factors, and oxidative stress via the miR-338/FOXA1 axis in sepsis-induced AKI, suggesting that circHIPK3 might be a potential biomarker and therapeutic target for sepsis-induced AKI patients. However, the present study had several limitations. First, other downstream regulatory mechanisms of circHIPK3 should be further explored. Second, in vivo experiments should be performed to further understand the pathogenesis of sepsis-induced AKI in the future study.
Research highlights
CircHIPK3 expression is elevated in septic AKI
Knockdown of circHIPK3 alleviated sepsis-induced AKI in vitro
CircHIPK3 interference relieved LPS-activated HK2 cell injury via miR-338
MiR-338 augmentation alleviates LPS-resulted HK2 cell injury by targeting FOXA1
Disclosure statement
No potential conflict of interest was reported by the author(s).
Additional information
Funding
References
- Kotecha A, Vallabhajosyula S, Coville HH, et al. Cardiorenal syndrome in sepsis: a narrative review. J Crit Care. 2018;43:122–127.
- Uchino S, Kellum JA, Bellomo R, et al. Ending supportive therapy for the kidney, acute renal failure in critically Ill patients: a multinational, multicenter study. JAMA. 2005;294(7):813–818.
- Liu V, Escobar GJ, Greene JD, et al. Hospital deaths in patients with sepsis from 2 independent cohorts. JAMA. 2014;312(1):90–92.
- Slade E, Tamber PS, Vincent JL. The surviving sepsis campaign: raising awareness to reduce mortality. Crit Care. 2003;7(1):1–2.
- Shum HP, Kong HH, Chan KC, et al. Septic acute kidney injury in critically ill patients - a single-center study on its incidence, clinical characteristics, and outcome predictors. Ren Fail. 2016;38(5):706–716.
- Kang K, Gao Y, Wang SC, et al. Dexmedetomidine protects against lipopolysaccharide-induced sepsis-associated acute kidney injury via an α7 nAChR-dependent pathway. Biomed Pharmacother. 2018;106:210–216.
- Xu C, Chang A, Hack BK, et al. TNF-mediated damage to glomerular endothelium is an important determinant of acute kidney injury in sepsis. Kidney Int. 2014;85(1):72–81.
- Umbro I, Gentile G, Tinti F, et al. Recent advances in pathophysiology and biomarkers of sepsis-induced acute kidney injury. J Infect. 2016;72(2):131–142.
- Xiong H, Wang H, Yu Q. Circular RNA circ_0003420 mediates inflammation in sepsis-induced liver damage by downregulating neuronal PAS domain protein 4. Immunopharmacol Immunotoxicol. 2021;43(3):271–282.
- Hong X, Li S, Wang J, et al. Circular RNA circFADS2 is overexpressed in sepsis and suppresses LPS-induced lung cell apoptosis by inhibiting the maturation of miR-15a-5p. BMC Immunol. 2021;22(1):29.
- Shi X, Ma W, Li Y, et al. CircPRKCI relieves lipopolysaccharide-induced HK2 cell injury by upregulating the expression of miR-545 target gene ZEB2. Biofactors. 2020;46(3):475–486.
- Xu L, Cao H, Xu P, et al. Circ_0114427 promotes LPS-induced septic acute kidney injury by modulating miR-495-3p/TRAF6 through the NF-κB pathway. Autoimmunity. 2021;1–13. DOI:10.1080/08916934.2021.1995861.
- Fan S, Hu K, Zhang D, et al. Interference of circRNA HIPK3 alleviates cardiac dysfunction in lipopolysaccharide-induced mice models and apoptosis in H9C2 cardiomyocytes. Ann Transl Med. 2020;8(18):1147.
- Li H, Zhang X, Wang P, et al. Knockdown of circ-FANCA alleviates LPS-induced HK2 cell injury via targeting miR-93-5p/OXSR1 axis in septic acute kidney injury. Diabetol Metab Syndr. 2021;13(1):7.
- Yao Y, Li X, Cheng L, et al. Circular RNA FAT atypical cadherin 1 (circFAT1)/microRNA-525-5p/spindle and kinetochore-associated complex subunit 1 (SKA1) axis regulates oxaliplatin resistance in breast cancer by activating the notch and Wnt signaling pathway. Bioengineered. 2021;12(1):4032–4043.
- Livak KJ, Schmittgen TD. Analysis of relative gene expression data using real-time quantitative PCR and the 2(-Delta Delta C(T)) Method. Methods. 2001;25(4):402–408.
- Zhang Y, Zhang Y, Wang S, et al. SP1-induced lncRNA ZFPM2 antisense RNA 1 (ZFPM2-AS1) aggravates glioma progression via the miR-515-5p/Superoxide dismutase 2 (SOD2) axis. Bioengineered. 2021;12(1):2299–2310.
- Zan L, Chen Q, Zhang L, et al. Epigallocatechin gallate (EGCG) suppresses growth and tumorigenicity in breast cancer cells by downregulation of miR-25. Bioengineered. 2019;10(1):374–382.
- Fouad AA, Abdel-Aziz AM, Hamouda AAH. Diacerein downregulates NLRP3/Caspase-1/IL-1β and IL-6/STAT3 pathways of inflammation and apoptosis in a rat model of cadmium testicular toxicity. Biol Trace Elem Res. 2020;195(2):499–505.
- Ye T, Zhang N, and Wu W, et al. SNHG14 promotes the tumorigenesis and metastasis of colorectal cancer through miR-32-5p/SKIL axis. In Vitro Cell Dev Biol Anim . 2019;55:812–820.
- Dellinger RP, Levy MM, Rhodes A, et al. Surviving sepsis campaign guidelines committee including the pediatric, surviving sepsis campaign: international guidelines for management of severe sepsis and septic shock: 2012. Crit Care Med. 2013;41(2):580–637. R. Moreno, S.
- Andrade-Oliveira V, Foresto-Neto O, Watanabe IKM, et al. Inflammation in renal diseases: new and old players. Front Pharmacol. 2019;10:1192.
- Sugama K, Suzuki K, Yoshitani K, et al. Changes of thioredoxin, oxidative stress markers, inflammation and muscle/renal damage following intensive endurance exercise. Exerc Immunol Rev. 2015;21:130–142.
- Han D, Fang R, Shi R, et al. LncRNA NKILA knockdown promotes cell viability and represses cell apoptosis, autophagy and inflammation in lipopolysaccharide-induced sepsis model by regulating miR-140-5p/CLDN2 axis. Biochem Biophys Res Commun. 2021;559:8–14.
- Sureshbabu A, Ryter SW, Choi ME. Oxidative stress and autophagy: crucial modulators of kidney injury. Redox Biol. 2015;4:208–214.
- Poggi C, Dani C. Sepsis and oxidative stress in the newborn: from pathogenesis to novel therapeutic targets. Oxid Med Cell Longev. 2018;2018:9390140.
- Lee KH, Tseng WC, and Yang CY, et al. The anti-inflammatory, anti-oxidative, and anti-apoptotic benefits of stem cells in acute ischemic kidney injury. Int J Mol Sci. 2019;2019;20(14):3529.
- Xu HP, Ma XY, Yang C. Circular RNA TLK1 promotes sepsis-associated acute kidney injury by regulating inflammation and oxidative stress through miR-106a-5p/HMGB1 axis. Front Mol Biosci. 2021;8:660269.
- Wei W, Yao Y, Bi H, et al. Circular RNA circ_0068,888 protects against lipopolysaccharide-induced HK-2 cell injury via sponging microRNA-21-5p. Biochem Biophys Res Commun. 2021;540:1–7.
- Tan M, Bei R. Circ_0091702 serves as a sponge of miR-545-3p to attenuate sepsis-related acute kidney injury by upregulating THBS2. J Mol Histol. 2021;52(4):717–728.
- Lai J, Xin J, Fu C, et al. CircHIPK3 promotes proliferation and metastasis and inhibits apoptosis of renal cancer cells by inhibiting MiR-485-3p. Cancer Cell Int. 2020;20(1):248.
- Yu H, Chen Y, Jiang P. Circular RNA HIPK3 exerts oncogenic properties through suppression of miR-124 in lung cancer. Biochem Biophys Res Commun. 2018;506(3):455–462.
- Wang Y, Zhao R, Liu W, et al. Exosomal circHIPK3 released from hypoxia-pretreated cardiomyocytes regulates oxidative damage in cardiac microvascular endothelial cells via the miR-29a/IGF-1 pathway. Oxid Med Cell Longev. 2019;2019:7954657.
- Shan K, Liu C, Liu BH, et al. Circular noncoding RNA HIPK3 mediates retinal vascular dysfunction in diabetes mellitus. Circulation. 2017;136(17):1629–1642.
- Yu L, Liu Y. circRNA_0016624 could sponge miR-98 to regulate BMP2 expression in postmenopausal osteoporosis. Biochem Biophys Res Commun. 2019;516(2):546–550.
- Liu G, Wan Q, Li J, et al. Circ_0038467 regulates lipopolysaccharide-induced inflammatory injury in human bronchial epithelial cells through sponging miR-338-3p. Thorac Cancer. 2020;11(5):1297–1308.
- Jiang L, Liu XQ, Ma Q, et al. hsa-miR-500a-3P alleviates kidney injury by targeting MLKL-mediated necroptosis in renal epithelial cells. Faseb J. 2019;33(3):3523–3535.
- Mu X, Wang H, Li H. Silencing of long noncoding RNA H19 alleviates pulmonary injury, inflammation, and fibrosis of acute respiratory distress syndrome through regulating the microRNA-423-5p/FOXA1 axis. Exp Lung Res. 2021;47(4):183–197.
- Zhang J, Ma D, Kang H, et al. Long noncoding RNA LINC01287 promotes proliferation and inhibits apoptosis of lung adenocarcinoma cells via the miR-3529-5p/RNASEH2A axis under the competitive endogenous RNA pattern. Environ Toxicol. 2021;36(10):2093–2104.
- Gou L, Zou H, Li B. Long noncoding RNA MALAT1 knockdown inhibits progression of anaplastic thyroid carcinoma by regulating miR-200a-3p/FOXA1. Cancer Biol Ther. 2019;20(11):1355–1365.
- Lu S, Wu H, Xu J, et al. SIKIAT1/miR-96/FOXA1 axis regulates sepsis-induced kidney injury through induction of apoptosis. Inflamm Res. 2020;69(7):645–656.