ABSTRACT
Antimicrobial resistance (AMR) has been a leading issue for human health globally threatening the achievement of several of the Sustainable Development Goals (SDGs). Originated from Bacillus cereus, carbapenemases phenotype has been considered to be a major concern in AMR. In this study, the AMR identification rate of P. aeruginosa isolates and infections in FAHJU showed an obvious upward trend from 2012 to 2016. All 88 carbapenem-resistant P. aeruginosa strains were screened for carbapenemase phenotype by modified Carbapenem Inactivation Method (mCIM), and these results of mCIM were compared with traditional PCR results. The isolates of P. aeruginosa and infected patients showed obvious upward trend from 2012 to 2016. The drug resistance to common clinical antibiotics was serious that the clinical rational use of antibiotics should be strengthened, which is in accordance with the Global Antimicrobial Resistance and Use Surveillance System (GLASS) report. In comparison, the results of mCIM showed that 18 out of 88 CRPA strains were carbapenemase positive, which were completely consistent with the results yielded by PCR method. Therefore, it is convinced that this mCIM methodology is a simple and quick method for detected carbapenemases producing P. aeruginosa and has a potential capability in carbapenemases phenotype of pathogen like B. cereus, which will undoubtedly aid in the AMR therapy.
Graphical abstract:Interpretation standards of modified carbapenem inactivation method
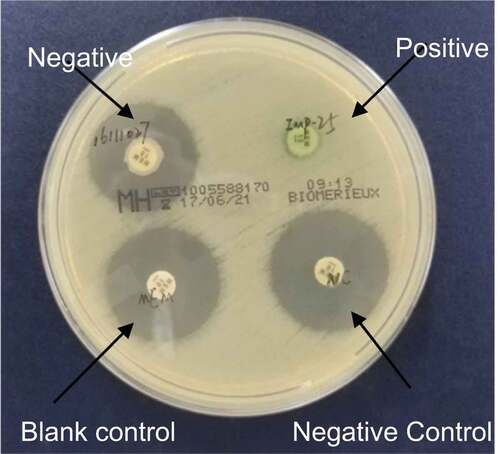
Highlights
Among 88 strains of CRPA, carbapenemase was detected in 18 strains with 16 IMP, 1 VIM, and 1 NDM.
mCIM can be a simple and rapid method applied to test P. aeruginosa carbapenem phenotype screening.
In 88 strains of carbapenem-resistant P. aeruginosa, a total of 26 strains were amplified using intI1 primers.
Among the 26 of class 1 integron-positive strains, CS-F and CS-R primers were used and 12 were negative.
1. Introduction
Antimicrobial resistance (AMR) refers to the presence of resistance to antimicrobial medicines in infectious agents by various microorganisms, including bacteria, viruses, fungi and parasites, according to Global Antimicrobial Resistance and Use Surveillance System (GLASS) Report from World Health Organization (WHO) [Citation1]. Caused by inappropriate use of antimicrobial agents, AMR has been a leading issue for human health globally. In 2016, AMR was found to threaten the achievement of several of the Sustainable Development Goals (SDGs) since 2016, including SDG2 and SDG3 [Citation2,Citation3]. However, despite the obstacle posed by emergence of AMR in microorganisms, rapid and accurate AMR detection will aid in effective therapy.
Pseudomonas aeruginosa, common pathogens in hospitals, is widely distributed in water, air, soil and normal human skin, respiratory tract and intestinal mucosa and can produce a variety of pigment [Citation4–6]. According to the 2016 China CHINET bacterial resistance monitoring results, clinical P. aeruginosa accounted for 8.66% of the total isolation rate, ranking 4th among gram-negative bacteria [Citation7]. 2009–2012 SENTEY antimicrobial drug monitoring data showed that the clinical isolation rate of P. aeruginosa ranks 1st (Europe and the Mediterranean region) and 2nd (the United States). The prevalence of P. aeruginosa infection has become an urgent public health problem in the world [Citation8].
Patients with low immunity are susceptible to P. aeruginosa as it is omnipresent in the environment and infectious via various transmission routes. Young [Citation9] founded that polymorphonuclear neutrophils are the most important defense against P. aeruginosa infection. The risk of P. aeruginosa infection was higher if neutrophils decreased for more than 10 days [Citation10]. Therefore, P. aeruginosa is the most important respiratory pathogen in patients with cystic fibrosis [Citation11]. In addition, P. aeruginosa can cause ventilator associated pneumonia, burn infection, diabetic foot infection, infectious keratitis, infective endocarditis, urinary tract infection [Citation12–23].
P. aeruginosa shows natural or acquired resistance to a variety of antimicrobial agents because aminoglycoside modifying enzymes (AMEs) can modify and inactivate the active chemical groups of gentamicin, tobramycin and amikacin [Citation24]. According to the type of reaction and the site of action, AMEs can be divided into three categories: aminoglycoside phosphotransferase, aminoglycoside nucleotide transferase and aminoglycoside acetyltransferase [Citation24]. Carbapenems are the most widely used antibiotics with the strongest antibacterial activity. Due to their high stability and low toxicity to extended spectrum β – lactamases and AmpC enzymes, carbapenems have become one of the important antibiotics to treat various bacterial infections especially severe infections caused by P. aeruginosa. Carbapenems can inhibit the synthesis of cell wall resulting in cell wall defect, and then bacteria die because of their own high osmotic pressure. However, with the widespread use of carbapenem antibiotics in clinic, the reports of carbapenem resistant P. aeruginosa are also increasing, the clinical anti infection treatment is now facing a severe test. Amongst, the production of carbapenemase is one of the important mechanisms of P. aeruginosa resistance to carbapenems, which were originally described in Gram-positive bacteria such as Bacillus cereus [Citation25]. According to Ambler classification, carbapenemases can be divided into A, B and D3. Except GES-2, all carbapenemases in P. aeruginosa belong to Ambler B class, also known as metallO-β -lactamases (MBLs) [Citation26].
The rapid, simple and accurate detection of carbapenem-producing strains is of great importance in obtaining the best therapeutic effect in clinical anti-infection therapy. Therefore, Clinical and Laboratory Standards Institute (CLSI) has recommended modified Hodge experiments (MHT) and CarbaNP experiments as phenotypic screening tests for carbapenem in Enterobacteriaceae bacteria, but both have some limitations. MHT experiments take a long time and is prone to false positive [Citation27] due to drug resistance mechanisms such as β- lactamase and or AmpC enzyme, the deletion of bacterial epimembranous protein, and the false negativein the detection of NDM and OXA carbapenemase [Citation22,Citation28]. CarbaNP experiments requires special reagents complicated procedures, short storage time of reagents. Moreover, the detection rate of some specific carbapenemases is not high as the false negative result because it is easy to overlook Slight changes in color when judging results [Citation29]. CIM were first proposed by Vander as a new type of carbapenem phenotypic screening test in 2015 [Citation30]. It hydrolyzes and deactivates carbapenem antibiotics by bacterial enzyme production to inhibit the growth of carbapenem-sensitive strains. Finally, the results are judged by measuring the size of the bacteriostasis circle. Modified Carbapenem Inactivation Method (mCIM) optimized the CIM steps and improved the detection rate of NDM genotypes [Citation29]. CLSI 2017 applied mCIM as a recommended method to the screening test of carbapenem phenotype of Enterobacteriaceae bacteria. However, few studies used mCIM in P. aeruginosa carbapenemase phenotype screening detection and CIM is mainly used for P. aeruginosa carbapenemase phenotype screening [Citation31,Citation32].
In this study, we analyzed the data of drug resistance of P. aeruginosa retrospectively. The paper disk method in CLSI 2016 was used to carry out drug sensitivity test on all carbapenemase resistant P. aeruginosa stored in our laboratory, summarized the drug resistance status and drug resistance spectrum characteristics of P. aeruginosa to common antibiotics, so as to guide clinical rational use of antibiotics. Moreover, we used mCIM as screening test of carbapenemase phenotype, and detected the coding gene of carbapenemase at gene level and compared its results with mCIM to evaluate the feasibility of mCIM in clinical application.
2. Material and methods
2.1 Hospital setting, bacterial isolates, and clinical data
A total of 1963 P. aeruginosa were isolated during 2012–2016 from First Affiliated Hospital of Jinan University (FAHJU), an AAA hospital with two medical centers and 1894 beds in the central city of Southern China. FAHJU serves patients based out of Guangzhou, extending to Guangdong-Hong Kong-Macao Greater Bay Area, other regions of China and overseas, with nearly 1.8 million annual emergency and outpatient visits, about 40,000 inpatients annually and approximately 70,000 patients discharged annually. Clinical information of the strains included isolation date, age, and gender of patients, department of isolation, infection site. Microbiological specimens were collected from the sputum, urine, blood, wound, secretion, bronchial, pus and other clinical culture.
2.2 Bacterial isolation and identification
Bacterial identification to the species level on all tested strains had been performed by VITEK2 Compact Microbial Identifier (Pioneering Diagnostics). The clinical samples were incubated in Columbia Blood Agar Base (Merieux Biological Products Co., Ltd., China) for 24 h at 37°C. Those colonies on the agar were adjusted to 0.5 McFarland turbidity with sterile saline and then performed on the VITEK2 Compact Microbial Identifier to identify the species.
2.3 Antimicrobial susceptibility testing
Among the 1936 P. aeruginosa isolates collected during 2012–2016, 874 isolates were isolated not repetitively. Antimicrobial susceptibility testing was performed on the 874 not-repetitively isolates by disk diffusion according to the Clinical and Laboratory Standard Institute 2016 (CLSI 2016). The minimum inhibitory concentrations (MICs) were determined through Vitek 2 automated system (Vitek AMS; bioMerieux Vitek Systems Inc., Hazelwood, MO). For the aim of this study, the antimicrobial drugs tested included β-lactam (TZP: Piperaxilin/tazobactm), cephems (CAZ: Ceftazidime, FEP: Cefepime), carbapenems (IMP: Imipenem, MEM: Meropenem), aminoglycosides (AMK: Amikacin, GEN: Gentamicin), fluoroquinolones (CIP: Ciprofloxacin, LEV: Levofloxacin). Quality control was performed using P. aeruginosa ATCC 27853 and E. coli ATCC 25922. All drug sensitive paper sheets were purchased from OXOID, UK.
2.4 Modified carbapenem inactivation method (mCIM)
Modified Carbapenem Inactivation Method (mCIM) was used to detect carbapenemase production P. aeruginosa, as described in CLSI M100-S27 [Citation3]. Meropenem disk was used to detect suspected carbapenemase-producing isolates. Bacteria to be tested were suspended in TSB broth and meropenem disk was added prior to incubation at 35°C for 4 h. The disks were then transferred onto MH agar, inoculated with a 0.5McFarland suspension of E. coli ATCC 25922, and incubated in ambient air at 35°C for 18 to 24 h. The positive control strain was P. aeruginosa producing IMP-25 MBL; the negative control strain was identified as clinical isolate 1,109,256, and the blank control was a sterile meropenem disk.
Carbapenemase positive: the diameter of the inhibition zone of meropenem paper is 6–15 mm or the diameter of the inhibition zone is 16–18 mm but there are scattered colonies in the inhibition zone. Carbapenemase negative: the diameter of the inhibition zone is ≥ 19 mm. Neutral: The diameter of the inhibition zone is 16–18 mm, and there is no scattered colony growth in the zone, so it is impossible to judge the presence of carbapenemase.
2.5 Detection of resistance genes in carbapenem-resistant P. aeruginosa
A total of 88 carbapenem-resistant P. aeruginosa isolated during 2012–2016 (12, 15, 21, 18 and 22 isolates in 2012, 2013, 2014, 2015 and 2016, respectively) were further studied on the carriage of different resistance genes by PCR using MBL-encoding drug resistance genes primers encoding designed by Primer5.0. In details, primer sequences for detecting the MBL coding genes include blaIMP (232bp), blaVIM (390bp), blaNDM (621bp), blaSPM (271bp), blaGIM(477bp), blaSIM(570bp) ().
Table 1. Primer sequences for detecting the MBL coding genes
The total PCR reaction volume is 50 ul, including 25 ul PremixTaq premix enzyme, 1 ul upstream and downstream primers, 3 ul DNA template, and 50 ul sterile distilled water. IMP amplification conditions are as followed: 94°C pre-denaturation for 3 min, 94°C denaturation for 45s, 55°C annealing for 30s, 72°C extension for 30s, a total of 35 cycles, and finally 72°C extension for 5 min. NDM amplification conditions are as follows: 94°C pre-denaturation Denaturation for 5 min, 94°C for 30s, 52°C annealing for 40s, 72°C extension for 50s, total 30 cycles, and finally 72°C extension for 5 min. VIM, SPM, GIM, SIM denaturation time is 30s, annealing temperature is 58, other conditions are consistent with IMP. The quality control strain is a sequence-confirmed P. aeruginosa that produces IMP-25 MBL.
2.6 Statistical analysis
In this study, the susceptibility of P. aeruginosa was defined as either resistant, intermediate resistant or susceptible. The overall antimicrobial susceptibility results and organization were managed in WHONET (version 5.6).
Microsoft Excel 2010 and statistical software SPSS20.0 were useded to statistically analyze the experimental data. The test results of mCIM and PCR test results were compared using paired χ2 test. P < 0.05 was defined as statistically significant.
3. Results
3.1 Clinical characteristics of P. aeruginosa
A total of 1936 P. aeruginosa were isolated during 2012–2016 from FAHJU (293, 293, 359, 428, and 563 for 2012, 2013, 2014, 2015 and 2016, respectively, as shown in ). Among them, 874 isolates were non-repetitively isolates (the annual number of infections were 133, 120, 159, 204, and 258, respectively, as shown in ).
Figure 1. The number of P. aeruginosa and infected patients. The 1936 P. aeruginosa strains were distributed to each year (blue bar). Amongst, 874 isolates were non-repetitively isolates from different infection cases (Orange bar).
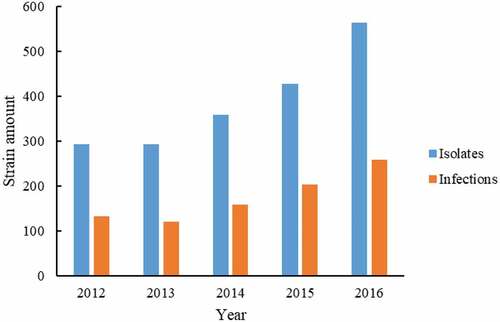
Microbiological specimens were collected from the sputum, urine, blood, wound secretion, pus, drainage and other clinical culture. Most P. aeruginosa were isolated from sputum (1437/1936, 74.2%), followed by urine (142/1936, 7.3%) and wound secretion (114/1936, 5.9%) (). Internal medicine, respiratory department, ICU, neurology, and surgery were the main department of P. aeruginosa, 22.5% (435/1936), 21.9% (356/1936), 18.4% (221/1936), 6.4% (123/1936), respectively ().
3.2 Antimicrobial resistance profiles of P. aeruginosa
Among the 1936 strains of P. aeruginosa, a total of 874 strains were non-repetitively isolated. The results of the drug sensitivity test showed that the average drug resistance rate of P. aeruginosa to imipenem was 22.1% (16.0%, 18.5%, 22.5%, 25.2%, 24.2% for each year respectively, , ) and the average resistance rate to meropenem was 21.7% (16.8%, 18.9%, 21.1%, 24.3%, 23.9% for each year respectively, ).
Figure 4. The variation of antibiotic resistance of 874 P. aeruginosa. The resistance strain numbers to imipenem (light blue bar), meropenem (Orange bar), ceftazidime (gray bar), cefepime (yellow bar), ciprofloxacin (dark blue bar), and levofloxacin (green bar) were distributed to each year according to the isolation date.
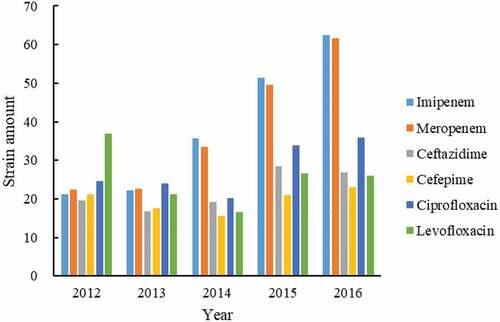
Table 2. Antimicrobial resistance profiles of P. aeruginosa during 2012–2016
The average drug resistance rate of P. aeruginosa to ceftazidime was 12.7% (14.7%, 14.0%, 12.1%, 13.9%, 10.4% for each year respectively, ) and the average resistance rate to cefepime was 11.3% (16.0%, 14.6%, 9.8%, 10.3%, 8.9% for each year respectively, ).
The susceptibility results of 874 strains to quinolone antibacterial drugs showed that the average resistance rate of P. aeruginosa to ciprofloxacin was 15.8% (18.5%, 20.0%, 12.7%, 16.6%, 13.9% for each year, ). The average drug resistance rate of levofloxacin was 14.6% (27.7%, 17.7%, 10.4%, 13%, 10.1% for each year, ).
3.4 Antimicrobial resistance profiles of carbapenem-resistant P. aeruginosa
Eighty-eight CRPAs were highly resistant to clinically common antimicrobial drugs. The resistance rates of imipenem were 100%. More than 50% CRPAs were resistant to cephalosporins. The resistance rates of amikacin, gentamicin, piperacillin/tazobactam, and meropenem were between 39.8% and 81.8% ().
Table 3. Antimicrobial resistance profiles of 88 carbapenem-resistant P. aeruginosa.
3.5 Detection of modified carbapenem inactivation method (mCIM) of carbapenem-resistant P. aeruginosa
The phenotypic screening test for carbapenemase was performed as mCIM. Among 88 carbapenem-resistant P. aeruginosa, 18 strains showed positive results, 20 carbapenem-sensitive P. aeruginosa strains showed negative results. The positive results and interpretation criteria are shown in .
Figure 5. Positive result of modified carbapenem inactivation method. Meropenem disk was used to detect suspected carbapenemase-producing isolates. Carbapenemase positive: the diameter of the inhibition zone of meropenem paper is 6–15 mm or the diameter of the inhibition zone is 16–18 mm but there are scattered colonies in the inhibition zone.
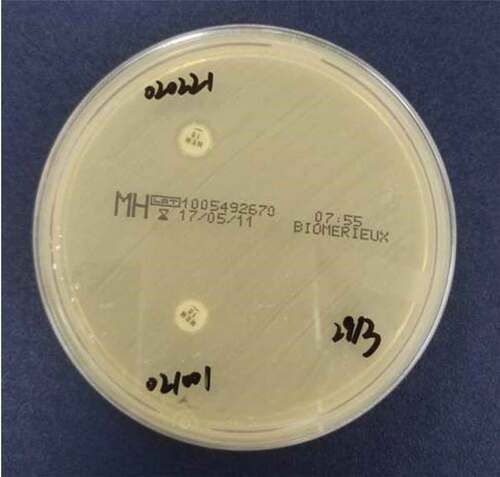
Figure 6. Interpretation standards of modified carbapenem inactivation method. Negative/blank control: the diameter of the inhibition zone is ≥ 19 mm; Positive: the diameter of the inhibition zone of meropenem paper is 6–15 mm or the diameter of the inhibition zone is 16–18 mm but there are scattered colonies in the inhibition zone.
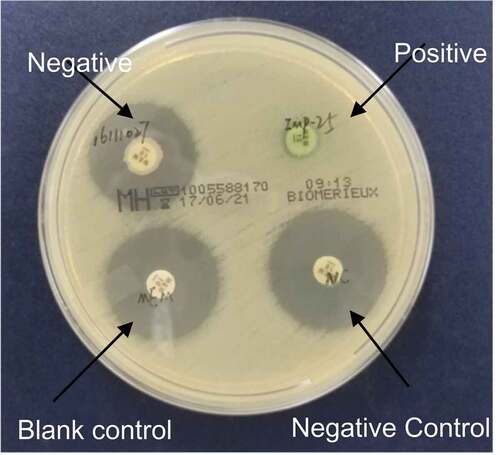
3.6 Detection of drug resistance genes in carbapenem-resistant P. aeruginosa
Among 88 carbapenem-resistant P. aeruginosa strains, 18 isolates were amplified with positive results for common resistance genes. The sequencing results of 16 strains were uploaded to the National Center for Biotechnology Information (NCBI) for blast comparison, the result of which confirmed that they all carried IMP resistance genes, with the matching rate reached 100% (). The results of the other two strains confirmed that one strain contained the VIM resistance gene and the other contained the NDM resistance gene; no SPM, SIM, GIM resistance genes were detected. The PCR amplification and electrophoresis results of some IMP primers are shown in .
3.7 Comparing between mCIM and carbapenemase resistance gene detection
The results of mCIM and PCR were further analyzed by paired χ2 test. As shown, the results yielded by mCIM and PCR were in 100% accordance, with no significant difference in the positive detection rate between them (P > 0.05, ).
Table 4. The results comparing between mCIM and carbapenemase resistance gene detection
4. Discussion
P. aeruginosa is an important pathogen of nosocomial infections as its characteristics of natural or acquired resistance to various antibacterial drugs. In recent years, with the widespread application of carbapenem antibacterial drugs, the detection rate of carbapenem-resistant P. aeruginosa is increasing, which has become a problem in clinical anti-infective treatment.
In this study, we retrospectively calculated that the number of P. aeruginosa isolates in FAHJU from 2012 to 2016, which can objectively reflect the detection rate and change trend of P. aeruginosa. According to the results, the detection rate of P. aeruginosa has increased year by year in the past 5 years. Among them, the detection rate of P. aeruginosa in 2016 increased by 156% compared with 2014, and the number of infections increased from 159 to 258. According to data in China Antibiotic Resistance Surveillance Network (CHINET), the isolation rate of P. aeruginosa ranged from 10.04% to 8.66% between 2012 and 2016, which is consistent with the uptrend of the detection rate of P. aeruginosa in our hospital [Citation7,Citation33,Citation34]. In terms of the distribution in wards, P. aeruginosa in our hospital was mainly found to be concentrated in the internal medicine (22.5%) and respiratory department (21.9%), which may be related to the lower immunity of patients being seen in these areas and their frequent use of mechanical ventilation causing damage to the respiratory mucosal barrier of those patients.
During the study period, the resistance rates of 874 non-repetitively P. aeruginosa strains to imipenem in FAHJU were 16%, 18.5%, 22.5%, 25.2%, and 24.2% respectively. The drug resistance rate is significantly lower than the national average drug resistance level except for 2012 and 2013 and is equivalent to the national average drug resistance rate during 2014–2016. However, the resistance of CRPAs to carbapenems is serious in FAHJU in recent years that the resistance rate of 88 CRPAs to cephalosporins and quinolones is greater than 50%. Therefore, the clinic should further strengthen the rational use of carbapenems in the management of antimicrobial drugs.
As CRPA in FAHJU continuous increased in recent year and the production of carbapenemase is one of the important resistance mechanisms of P. aeruginosa to carbapenem, we further studied the resistance mechanism of carbapenemase on 88 CRPA strains. First, we conducted a phenotypic screening test on carbapenemase. Although CLSI 2017 did not recommend using mCIM in carbapenemase phenotypic screening tests on bacteria other than Enterobacteriaceae, we still tried to mCIM in phenotypic screening test on the CRPA. The results showed 18 of the 88 CRPA strains were positive, and 20 were negative. In order to further clarify the genotype of P. aeruginosa carbapenemase and discuss the effectiveness and feasibility of mCIM, traditional PCR methods using six pairs of primers (IMP, VIM, NDM, SPM, GIM, and SIM) were performed for comparative research. The PCR results showed that 18 of 88 CRPA strains were carbapenemase-positive, 16, 1, and 1 strain produced IMP type, VIM type, and NDM type carbapenemase, respectively. All 88 strains showed negative results of SPM, GIM, and SIM carbapenemase. Therefore, PCR method is completely consistent with the results of mCIM. The specificity and sensitivity of mCIM are 100%, which is consistent with previous study (specificity and sensitivity were 98.8% and 97.7%, respectively) [Citation7]. The results of this study strongly suggested the applicability of the mCIM in the high screening of the carbapenemase phenotype of P. aeruginosa.
Currently, mCIM is mainly used for Enterobacteriaceae carbapenemase phenotype screening [Citation30,Citation35]. Few studies used mCIM in P. aeruginosa carbapenemase phenotype screening detection and CIM is mainly used for P. aeruginosa carbapenemase phenotype screening [Citation31,Citation32]. The results of this study showed the possibility of utilizing mCIM in P. aeruginosa carbapenemase phenotype screening, and we will expand the number of experimental strains and continue to study mCIM and its use as a screening test for the carbapenemase phenotype of P. aeruginosa. In addition, the screening of carbapenemase phenotype should not be limited within specific species as P. aeruginosa. We have also successfully applied a similarmethodology following a cell wall processing on carbapenemase phenotype on B. cereus strains to confirm its capability and applicability (data not shown). Since B. cereus has been reporting to be a more and more prevalent pathogens, the rapid screening of its carbapenemase phenotype may contain sufficient importance. The method helps avoid false high specificity and sensitivity caused by a small number of experimental strains.
Chen Cha et al. [Citation36] found that the production of carbapenemase is the main drug resistance mechanism in P. aeruginosa in a 3A hospital (First-class Hospital at Grade III) in Guangzhou, which is consistent with the results of Jiang et al. [Citation37]. In this study, combining the results of mCIM and traditional PCR methods, it showed that carbapenemase production is not the main mechanisms for the resistance of P. aeruginosa to carbapenem in FAHJU. Some studies showed that the main mechanisms of P. aeruginosa resistance to carbapenems are carbapenemase production, loss of outer membrane porin, and overexpression of efflux pumps [Citation38]. We will further study the outer membrane porin and the active efflux pump system.
5. Conclusion
In this study, the AMR identification rate of P. aeruginosa isolates and infections in FAHJU showed an obvious upward trend from 2012 to 2016. This study has successfully applied a modified carbapenemase inactivation test (mCIM) to show that 18 of the 88 CRPA strains were positive. It is a simple and rapid assay that can be used for screening P. aeruginosa for carbapenemase activity. The results from mCIM and PCR correlate completely. The production of Ambler B class carbapenemase is one of the mechanisms of resistance of P. aeruginosa to carbapenems in hospitals. Not limited within gram negative pathogens, the mCIM method also has a potential capability in carbapenemases phenotype screening in gram-positive bacteria like B. cereus, which will undoubtedly aid in the AMR therapy.
Ethical statement
An ethics approval was uploaded. The informed consent was obtained for experimentation with human subjects.
Data availability
The authors confirm that the data supporting the findings of this study are available within the article
Supplemental Material
Download PDF (226.1 KB)Disclosure statement
No potential conflict of interest was reported by the authors.
Supplementary material
Supplemental data for this article can be accessed online at https://doi.org/10.1080/21655979.2022.2072601
Additional information
Funding
References
- Global antimicrobial resistance and use surveillance system (GLASS) report: 2021. 2021.
- World Health Organization. Executive board 140th session: EB140/11 antimicrobial resistance. 2017.
- Avner BS, Fialho AM, Chakrabarty AM. Overcoming drug resistance in multi-drug resistant cancers and microorganisms: a conceptual framework. Bioengineered. 2012;3(5):262–270.
- Kumari S, Mangwani N, Das S. Synergistic effect of quorum sensing genes in biofilm development and PAHs degradation by a marine bacterium. Bioengineered. 2016;7(3):205–211.
- Ren J, Li N, Du M, et al. Study on the effect of synergy effect between the mixed cultures on the power generation of microbial fuel cells. Bioengineered. 2021;12(1):844–854.
- Zhou TY. Diagnosis and Illustration of Clinical Microbiology. Shanghai Sci Technol Press. 2017.
- Hu FP, Guo Y, Zhu DM, et al. Bacterial resistance surveillance of CHINET in China in 2016. Chin J Infec Chemotherapy. 2017;17:481–491.
- Sader HS, Farrell DJ, Flamm RK, et al. Antimicrobial susceptibility of gram-negative organisms isolated from patients hospitalised with pneumonia in US and European hospitals: results from the SENTRY antimicrobial surveillance program, 2009-2012. Int J Antimicrob Agents. 2014;43(4):328–334.
- Young LS, Armstrong D. Human immunity to Pseudomonas aeruginosa. I. In-vitro interaction of bacteria, polymorphonuclear leukocytes, and serum factors. J Infect Dis. 1972;126(3):257–276.
- Safdar A, Armstrong D. Infections in patients with hematologic neoplasms and hematopoietic stem cell transplantation: neutropenia, humoral, and splenic defects. Clin Infect Dis. 2011;53(8):798–806.
- Boucher RC. New concepts of the pathogenesis of cystic fibrosis lung disease. Eur Respir J. 2004;23(1):146–158.
- Robinson CM, Kobe BN, Schmitt DM, et al. Genetic engineering of Francisella tularensis LVS for use as a novel live vaccine platform against Pseudomonas aeruginosa infections. Bioengineered. 2015;6(2):82–88.
- Klompas M, Kleinman K, Khan Y, et al. Rapid and reproducible surveillance for ventilator-associated pneumonia. Clin Infect Dis. 2012;54(3):370–377.
- Mayhall CG, Mayhall CG. The epidemiology of burn wound infections: then and now. Clin Infect Dis. 2003;37(4):543–550.
- Oates A, Bowling FL, Boulton AJ, et al. Molecular and culture-based assessment of the microbial diversity of diabetic chronic foot wounds and contralateral skin sites. J Clin Microbiol. 2012;50(7):2263–2271.
- Sankaridurg PR, Sharma S, Willcox M, et al. Bacterial colonization of disposable soft contact lenses is greater during corneal infiltrative events than during asymptomatic extended lens wear. J Clin Microbiol. 2000;38(12):4420–4424.
- Sapico FL, Montgomerie JZ. Vertebral osteomyelitis in intravenous drug abusers: report of three cases and review of the literature. Rev Infect Dis. 1980;2(2):196–206.
- Nakamoto H, Hashikita Y, Itabashi A, et al. Changes in the organisms of resistant peritonitis in patients on continuous ambulatory peritoneal dialysis. Adv Perit Dial. 2004;20:52–57.
- Weng L, Zhang Y, Yang Y, et al. Isolation of the autoinducer-quenching strain that inhibits LasR in Pseudomonas aeruginosa. Int J Mol Sci. 2014;15(4):6328–6342.
- Wang SQ, He XJ, Li XB, et al. Advances in quorum sensing system of Pseudomonas aeruginosa. Chin J Hosp Pharm. 2016;36:149–152.
- Kim HS, Lee SH, Byun Y, et al. 6-Gingerol reduces Pseudomonas aeruginosa biofilm formation and virulence via quorum sensing inhibition. Sci Rep. 2015;5:8656.
- Mulcahy LR, Isabella VM, Lewis K. Pseudomonas aeruginosa biofilms in disease. Microb Ecol. 2014;68(1):1–12.
- Schiavano GF, Carloni E, Andreoni F, et al. Prevalence and antibiotic resistance of Pseudomonas aeruginosa in water samples in central Italy and molecular characterization of oprD in imipenem resistant isolates. PLoS One. 2017;12(12):e0189172.
- Subedi D, Vijay AK, Willcox M. Overview of mechanisms of antibiotic resistance in Pseudomonas aeruginosa: an ocular perspective. Clin Exp Optom. 2018;101(2):162–171.
- Carfi A, Pares S, Duée E, et al. The 3-D structure of a zinc metallo-beta-lactamase from Bacillus cereus reveals a new type of protein fold. EMBO J. 1995;14(20):4914–4921.
- Shibata N, Doi Y, Yamane K, et al. PCR typing of genetic determinants for metallo-beta-lactamases and integrases carried by gram-negative bacteria isolated in Japan, with focus on the class 3 integron. J Clin Microbiol. 2003;41(12):5407–5413.
- Lifshitz Z, Adler A, Carmeli Y. Comparative study of a novel biochemical assay, the Rapidec Carba NP test, for detecting carbapenemase-producing Enterobacteriaceae. J Clin Microbiol. 2016;54(2):453–456.
- Genc O, Aksu E, Gulcan A. The identification of carbapenemase types in Enterobacteriaceae by using molecular assay and phenotyping confirmation tests. J Microbiol Methods. 2016;125:8–11.
- van der Zwaluw K, de Haan A, Pluister GN, et al. The carbapenem inactivation method (CIM), a simple and low-cost alternative for the Carba NP test to assess phenotypic carbapenemase activity in gram-negative rods. PLoS One. 2015;10(3):e0123690.
- Yamada K, Kashiwa M, Arai K, et al. Evaluation of the modified carbapenem inactivation method and sodium mercaptoacetate-combination method for the detection of metallo-β-lactamase production by carbapenemase-producing Enterobacteriaceae. J Microbiol Methods. 2017;132:112–115.
- Aktaş E, Malkoçoğlu G, Otlu B, et al. Evaluation of the carbapenem inactivation method for detection of carbapenemase-producing gram-negative bacteria in comparison with the RAPIDEC CARBA NP. Microb Drug Resist. 2017;23(4):457–461.
- Yang J, Shi DY, Zhao JH. Application evaluation of different substrates in CIM test for detection of carbapenemase in gram negative bacilli. J clin lab. 2016;34:575–578.
- Hu FP. Changes of drug resistance of five important clinical isolates in China bacterial resistance monitoring network from 2005 to 2014. Chin Infect Chemotherapy. 2017;17:94–99.
- Hu FP, Zhu DM, Wang F, et al. Surveillance of bacterial resistance in CHINET in 2015. Chin J Infect Chemotherapy. 2016;16:685–694.
- Tamma PD, Opene Belita NA, Gluck A, et al. Comparison of 11 Phenotypic Assays For Accurate Detection Of Carbapenemase-Producing Enterobacteriaceae. J Clin Microbiol. 2017;55(4):1046–1055.
- Chen C, Lin DL, Zhang N, et al. Study on resistance mechanism and genetic relationship of imipenem resistant Pseudomonas aeruginosa. Chin J Nosocomial Infect. 2012;22:3201–3205.
- Jiang YT, Mai YL, Chen DQ, et al. Detection and analysis of resistance genes of carbapenem resistant Pseudomonas aeruginosa. Chin J Antibiot. 2016;41:552–556.
- Tang Y, Jiang XF. Research Progress on the resistance mechanism of Pseudomonas aeruginosa to carbapenems. Foreign Med Antibiot. 2015;36:49–54.