ABSTRACT
Neutrophils are the most abundant circulating leukocytes and the first line of defense against invading pathogens. They are key components of the innate immune system. Neutrophils also cause tissue damage in various autoimmune and inflammatory diseases and play an important role in cancer progression. Due to the complex relationship between various diseases and neutrophils, these cells have become potentially important targets for therapeutic interventions. Monitoring neutrophils in the tumor microenvironment is critical for tumor treatment and prognostic analysis but remains challenging. Molecular imaging technology has made great progress as a valuable tool for noninvasively visualizing biological events and establishing effective cancer diagnoses and treatment methods. Molecular probes designed based on the characteristics of neutrophils, such as their flexible morphology, the abundance of surface receptors, and the absence of immunogenicity, have shown great potential. This has created an opportunity for novel ideas and research methods for the diagnosis and targeted therapy of inflammatory diseases and tumors, with the goal of integrated diagnosis and treatment. This review discusses the diverse tumor detection and diagnostic imaging strategies based on neutrophils. It is anticipated that neutrophil-based imaging will soon be gradually integrated into clinical applications.
Research Highlights
Neutrophils play a role in promoting/inhibiting tumor growth in the tumor microenvironment.
To be more conducive to discovering neutrophils in tumors and guiding treatment, medical imaging technology has developed stepwise.
In the context of the intersection of imaging technology, great breakthroughs have been made in the individualized research of tumor diagnosis.
This article contributes to the promotion of new multimodal molecular imaging technology and the application of rapid diagnosis individualization.
Graphical Abstract
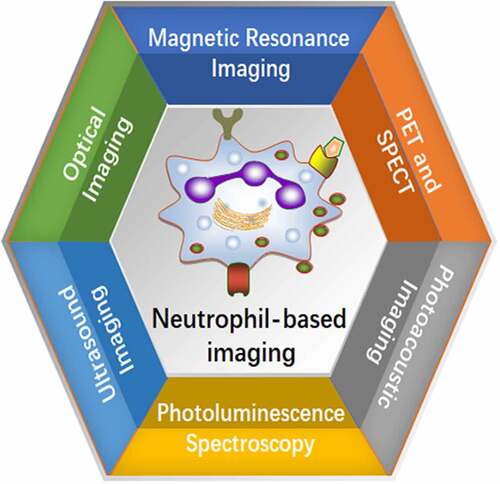
Introduction
Clinical and experimental evidence indicates that immune cell plasticity is a key factor in tumorigenesis, tumor progression, and metastasis [Citation1]. Neutrophils are unusual nonspecific immune cells that are most abundant in white blood cells. When a pathogen invades the body and causes a local infection, neutrophils quickly reach the infection site, where they phagocytose and help clear the invading pathogen. Thus, neutrophils are the first line of defense against innate immunity [Citation2]. Neutrophils also control the development of adaptive immune responses by initiating the ablation phase of inflammation and controlling a series of important biological functions of tissue homeostasis. Neutrophil dysfunction triggers pathological reactions that lead to thrombosis, autoimmune diseases, and tumor-related diseases [Citation3]. Cancer cells recruit neutrophils through the secretion of various molecules. Neutrophil infiltration promotes or inhibits tumor growth and metastasis (). Neutrophil populations in the tumor microenvironment (TME) have heterogeneous phenotypes and functional diversity and play dual roles of tumor-promoting (N2) or tumor-suppressing (N1) in the tumor microenvironment [Citation3]. A number of studies have shown that neutrophils promote tumor cell metastasis, invasion, and tumor-related thrombosis by releasing neutrophil extracellular traps (NETs), which is an emerging field of tumor research [Citation4]. NETs were increased in hepatocellular carcinoma tumor tissues compared to paired non-tumor liver tissues. NETs subsequently induce cancer cell migration and downregulate tight junction molecules in adjacent endothelial cells, thereby promoting tumor infiltration and metastasis [Citation5].
Figure 1. Neutrophil recruitment. Neutrophils are derived from hematopoietic stem cells of the bone marrow and developed in the bone marrow. The sequential steps of classical neutrophil recruitment from the vascular system to tissues in response to infection are shown. Neutrophils undergo integrin-mediated crawling to migrate. The migration of endothelial cell junctions is mediated by several other adhesion molecules. The release of proteolytic enzymes allows neutrophils to enter the extravascular spaces. Extravascular neutrophils follow a chemotactic gradient to reach the tumor site. Neutrophils are involved in various stages of tumorigenesis and development, including tumorigenesis, proliferation, and metastasis.
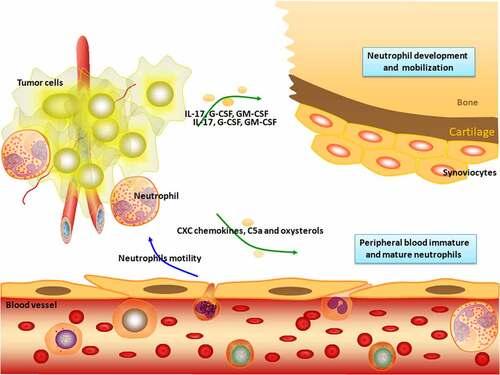
The design of effective neutrophil-based live-cell probes or neutrophil biomimetic nanoprobes for imaging diagnosis is a research goal [Citation6]. Probes are designed using the characteristics of neutrophils, including flexible morphology, abundant surface receptors, and lack of immunogenicity [Citation7]. This design strategy has great promise for overcoming the limitations of nanomaterials, including poor biocompatibility, short circulation time, and immunogenicity [Citation8]. Neutrophil-based imaging methods have been introduced for tumor diagnosis and analysis of the inflammatory state in the tumor microenvironment, achieving the goal of integrating diagnosis and treatment. This review discusses the application of molecular imaging technology in the detection and monitoring of neutrophils.
Potential tracers for imaging neutrophils
Increased neutrophil infiltration and the neutrophil-to-lymphocyte ratio (NLR) in various solid tumor tissues can be used as independent risk factors for the prognosis of patients with tumors. Several studies have reported that combining NLR metrics with positron emission tomography (PET)/computed tomography (CT) metabolic parameters can more clearly predict tumor survival time and biology [Citation9,Citation10]. PET is a pivotal molecular imaging tool for cancer diagnosis and imaging due to its relatively low spatial resolution and high sensitivity [Citation11]. 18F-fluorodeoxyglucose PET/CT (18F-FDG PET/CT) can provide morphological and functional information for cancer management and is important for cancer diagnosis, staging, treatment planning, treatment response, and recurrence [Citation12]. 18F-FDG PET/CT has been used to accurately display the metabolic status of the TME, which has helped clarify the interaction between metabolism and immune pathways [Citation13]. Wang et al. used a PET/CT scanner to acquire whole-body image data of patients with lung cancer from the base of the skull to the mid-thigh. The pH of 18F-FDG was 5–7, and the radiochemical purity of the isotonic solution was ≥95%. These parameters include the maximum standardized uptake value (SUVmax), metabolic tumor volume, tumor lesion glycolysis, and tumor infiltration immunity [Citation14]. The association between NLR and SUVmax suggests that SUVmax could be a potential predictor for selecting immunotherapy candidates. Similar findings were demonstrated in colorectal cancer (CRC), where there was a significant correlation between the pretreatment hematological parameters and metabolic parameters of 18F-FDG PET/CT [Citation15], with 4.1 mm transaxial intrinsic spatial resolution and 16.2 cm axial field width. For patients with a high NLR and low lymphocyte-to-monocyte ratio (LMR), 18F-FDG PET/CT may help detect distant metastases and predict prognosis. The hypoxic state of tumors can be assessed noninvasively using molecular imaging technology, which has the potential to characterize the tumor aggressiveness [Citation16]. Based on this theory, Kinoshita et al. evaluated the clinical utility of a newly developed tumor hypoxia PET tracer routinely performed by PET/CT imaging, and only SUVmax was reported in focal non-small cell lung cancer (NSCLC) [Citation17]. Their results demonstrated that a high NLR was associated with the accumulation of hypoxic 18F-fluororubicin (18F-FAZA) and 62Cu-diacetyl-bis(N4)-methylsemithiocarbazone (62Cu-ATSM) but not 18F-FDG.
Although 18F-FDG is the most widely used PET radiotracer, it has several limitations. These include distinguishing tumors from non-neoplastic inflammatory diseases [Citation18]. Visualization by PET imaging and autoradiography using 89Zr-oxalate in inflammatory lesions and tumors, Park et al. found that compared with [18F]FDG and sodium [18F]fluoride, 89Zr-oxalate demonstrated a high selectivity index for the tumor soon after injection and inflammation at a delayed time point [Citation19]. Histological examination revealed a large number of neutrophils in the tumor lesions, with the highest 89Zr-oxalate uptake. In a mouse model of rheumatoid arthritis (RA), 89Zr-oxalate showed a high accumulation of inflammatory lesions. These findings implicate 89Zr-oxalate as a new strategic tool for tumor imaging and inflammatory processes.
Inflammation is the basis for the fight against foreign invasion. In turn, inflammatory factors may become the accomplices of tumors under long-term stimulation. The inflammatory state of the body is directly related to tumor proliferation and metastasis, chemosensitivity, and immune function. In a complex TME, immune cells are destroyed and promote tumor growth and metastasis by secreting pro-survival and pro-migratory factors [Citation3]. As phagocytic cells, neutrophils play an important role in the immune regulation of inflammation. Therefore, neutrophil observations can be used to assess the degree of inflammation in the TME (), which is beneficial for selecting appropriate immunotherapy strategies and predicting disease severity [Citation20]. More recently, [64Cu]Cu-(PEG)-cinnamoyl-phenylalanine-(D) leucine-phenylalanine-(D) leucine-phenylalanine-lysine (cFLFLFK) and [68Ga] Ga-nanotracer-cFLFLFK have been shown to bind to formyl peptide receptor 1 (FPR1), which is mainly expressed by neutrophils [Citation21–23]. Co-registration of PET and CT images was performed using ASIPRO (Siemens) and a transformation matrix previously obtained with an imaging phantom. These imaging data confirmed that the cellular infiltrates at the sites of inflammation were exclusively neutrophils. As 99mTc can be easily obtained from its parent isotope, molybdenum 99, 99mTc-labeled-cinnamoyl-F-(D)L-F-(D)L-F (cFLFLF), and Thr-Lys-Pro-Arg (TKPR) higher affinity antagonists, TKPPR, were utilized in neutrophil-based imaging research. Single-photon emission CT (SPECT) and CT images were co-registered using the ASIPRO software (Siemens Medical Solutions USA, Inc., Knoxville, TN, USA) and a transformation matrix with a dual-modality phantom. The results proved that cFLFLF-PEG12-TKPPR-99mTc has a high affinity for neutrophils and is stable in the serum [Citation24]. Chen et al. utilized PET/CT (Discovery LS PET/CT system, GE) in their research. CT was performed at 140 kV and 80 mA immediately followed by a PET whole-body scan obtained with an acquisition time of 3 min for each bed position. These results proved that [99mTc]Tc-cFLFLF was superior to [99mTc]Tc-MDP and 2-[18F]FDG in the detection of acute osteomyelitis, with an avid affinity for neutrophil binding to FPR1 [Citation25]. Another group tracked neutrophil infiltration by SPECT imaging using a Siemens Orbiter camera outfit with a customized 1.5-mm tungsten pinhole collimator. Their data demonstrated that neutrophil-targeted imaging using [99mTc] Tc-hydrazinonicotinic acid (HYNIC)-PEG-cFLFLF could noninvasively track inflammation [Citation26].
Figure 2. Schematic illustration of the procedure of PET tracer based molecular imaging. After producing a suitable PET tracer and injecting the radioactive tracer, positrons were emitted into the body of the subject, and PET was detected and imaged. 18F-FDG is similar to glucose metabolism in cells and is currently the largest imaging agent available for clinical use. Phosphorylated glucose (glucose-6 P) can be further metabolized by cells, while phosphorylated 18F-FDG cannot be further metabolized, so it is considered ‘trapped.’ 18F-FDG PET/CT metabolic parameters have a good correlation with the clinical prognostic indicator NLR, suggesting that it may be more advantageous than traditional indicators for evaluating tumor prognosis.
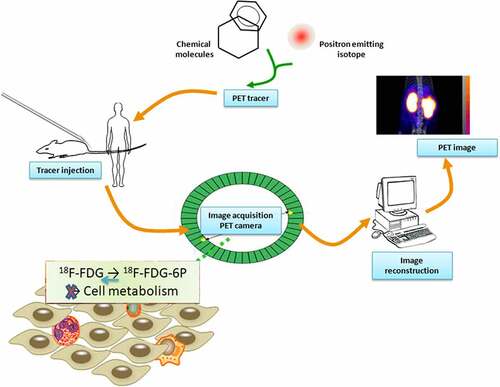
Prospect for nanoparticle-remodeled neutrophils
Advances in molecular imaging based on nanoparticles (NPs) have greatly improved the sensitivity and specificity of imaging detection methods [Citation27]. Further research using NPs loaded with gadolinium (Gd) contrast agents functionalized with ligands specifically targeting overexpressed cancer cell receptors has also achieved encouraging results in animals [Citation28,Citation29]. However, due to their rapid clearance in the blood circulation, limited tumor-targeting capabilities, and off-target effects in the body, NP-based tumor delivery platforms are rarely applied in clinical settings [Citation30]. To diagnose tumors more accurately, there is an urgent need to develop an NP molecular imaging detection platform that can circulate in the body. NP-based molecular imaging should have the characteristics of ‘exempted from inspection’ and be able to penetrate TME [Citation31]. Among the many cell carriers in use, neutrophils are particularly promising candidates [Citation32]. There are three main reasons for this finding. First, neutrophils are easily obtained and are the most abundant white blood cells in human circulation. Second, cancer cells secrete various cytokines and chemokines, which cause neutrophils to migrate rapidly to the TME. Third, neutrophils can escape immune system attacks and overcome many physiological obstacles so that they can specifically target the tumor area [Citation33,Citation34]. Taking advantage of inflammation sensing and neutrophil recruitment, Qiu et al. constructed a highly sensitive live probe for potentially accurate tumor diagnosis [Citation35]. Through 5-thio-2-nitrobenzoate (TNB)-mediated rapid and effective disulfide bond-thiol exchange, bovine serum albumin NPs loaded with imaging agents were covalently immobilized on the surface of neutrophils. Magnetic resonance imaging (MRI) revealed that these neutrophil probes were rapidly concentrated at tumor sites in lung cancer xenograft models. These findings indicate that these live probes have great potential for accurate tumor diagnosis ().
Table 1. Nanoparticle-remodeled neutrophils.
Kirschbaum et al. employed high-field MRI and fluorescently labeled cross-linked iron oxide NPs to locate immune cell infiltration [Citation43]. These ultrasmall paramagnetic iron oxide NPs (USPIO) can be engulfed by neutrophils and circulate to sites of inflammation. USPIO imaging provides a tool for noninvasive longitudinal MRI tracking of neutrophils. A 9.4-Tesla (T) horizontal-bore small animal MRI scanner (BioSpec 94/20 USR; Bruker BioSpin GmbH) with a four-channel phased-array surface receiver coil was used according to the MRI protocol. For clinical applications, particularly for gliomas, there is an urgent need to track their motion and biological effects. By combining the advantages of living cells and nanoparticle carriers, Wu et al. established internalized doxorubicin-loaded magnetic mesoporous silica NPs (ND-MMSNs) for neutrophils in an inflammatory glioma microenvironment [Citation44]. This experiment was performed on a 3.0 T clinical MRI instrument, and the results confirmed that NPs could enhance MRI contrast to achieve an accurate diagnosis.
Qiu et al. prepared polylactic acid-containing quantum dots (QD), superparamagnetic iron oxide NPs (SPIONs), and gold (Au) NPs using the with-or-without double emulsion method for labeling neutrophil-poly D, L-lactic-co-glycolic acid (PLGA) NPs [Citation45]. They first used flow cytometry and confocal microscopy to study the uptake of the hybrid PLGA NPs by human neutrophils. In addition, the ability of neutrophils to produce reactive oxygen species (ROS) after incubation with PLGA NPs was evaluated. QDs and SPIONs were encapsulated in PLGA NPs as fluorescent and MRI agents, respectively. QD encapsulation rendered the hybrid PLGA NPs highly fluorescent. NP uptake by neutrophils can be monitored by fluorescence. The uptake rate of PLGA_QD_- SPION NPs by neutrophils was significantly higher than that of the PLGA_QD_SPION_Au NPs. The samples were then scanned with a 7 T MR scanner (Bruker Biospec), and T2-weighted spin-echo MRI images were acquired at an echo time of 260 ms. The results demonstrated that the magnetic response and fluorescent labeling of neutrophil markers could be combined for tumor visualization and local photothermal treatment. In addition, a simple, fast, and biocompatible strategy was conceived by Liu et al., who constructed AIEgen-lipid-engineered neutrophils within 30s [Citation46]. This fast-labeling method has been successfully used to monitor neutrophil behavior and offers the opportunity to monitor the fate of neutrophils using fluorescence imaging both in vitro and in vivo.
Biomimetic NPs as a novel imaging platform
Biomimetic NPs are biodegradable, biocompatible, nontoxic, and have unique shapes (liposomes, polymer nanoparticles, polymer bodies, hybrid nanoparticles, inorganic nanoparticles, and solid lipid nanoparticles) and various sizes [Citation47]. A natural nanocarrier exists in grapefruit (grapefruit-derived nanovectors or GNVs). The plasma membranes of stimulated leukocytes can be coated with GNVs for molecular imaging [Citation48]. A novel construction mode was proposed by Kang et al., who designed neutrophil-mimicking NPs by combining neutrophil-derived membranes with biodegradable PLGA NPs. These ‘super neutrophils’ target circulating tumor cells and the pre-metastatic niche for tumor therapy and imaging [Citation49]. Exploiting the knowledge that formyl peptide receptors on the surface of neutrophils are upregulated during activation, Phipps et al. constructed a novel formyl peptide-targeting nanoprobe (FPR-nanoprobe) [Citation50]. This probe can be used to immediately evaluate the accumulation of neutrophils in necrotic tissues and to study the activation of innate immune cells. Sun et al. took advantage of a nanoengineering approach to combine liposomes with neutrophils to generate oxygen-carrying sonosensitizer cells termed Acouscyte/O2 [Citation51]. After intravenous injection of temoporfin-labeled neutrophils (Tp-NEs), in vivo fluorescence images of the mice were acquired at different time points and Acouscyte/O2 twice under ultrasound stimulation. These novel cells with acoustic functions can selectively accumulate in tumors and exhibit periodically enhanced inflammatory targeting and tumor suppression upon ultrasound stimulation.
Quantification of neutrophils by imaging technology
Immune protection depends on the ability of neutrophils to infiltrate the attacked tissues. In contrast, an increase in the number of neutrophils at the tumor site can inhibit the functional activation of other immune cells, such as natural killer cells, providing a favorable environment for tumor cell survival and metastasis [Citation3]. Garcia-Mendoza et al. used fluorescein to label lymphocyte antigen 6 complex locus G6D(Ly6G), a surface marker of neutrophils [Citation52]. Monocytes typically express Ly6G transiently during development, while mature granulocytes and peripheral neutrophils maintain its expression, making Ly6G a well-established cell surface marker for these populations [Citation53]. The reduction in Ly6G+ neutrophils inhibited tumor formation and lung metastasis, especially in collagen-intensive breast tumors. Other research has been performed using a new isotropic 1 μm resolution optical imaging technology termed micro-optical coherence tomography (μOCT), which performs 4D (x, y, z, t) visualization. Chu et al. applied μOCT to a transepithelial migration model and robustly monitored the spatial distribution and microscopic dynamics of neutrophils destroying the mucosal barrier [Citation54]. Turk et al. also labeled neutrophils with Ly6G to observe how the cells interact with the tumor vasculature and cells in the TME. Researchers have used in vivo microscopy (IVM) to monitor the behavior of neutrophils. A Leica SP8 inverted microscope outfitted with four excitation lasers (405, 488, 552, and 638 nm) was used in this study. These findings provide a theoretical basis for the development of molecular imaging [Citation55]. Using IVM to study the behavior of neutrophils in the TME provides critical insights not available by other methods, which will help shape better and more effective anti-cancer drugs and treatments.
New insights into the correlation of neutrophil-specific markers and imaging
Myeloperoxidase (MPO) is a heme protein abundant in neutrophils, synthesized by granulocytes in the bone marrow before entering the circulation, and stored in Aspergillus granules [Citation56]. MPO not only kills microorganisms engulfed in cells but can also be released extracellularly to destroy a variety of target substances, such as tumor cells and toxins. However, under certain conditions, MPO promotes reactions that generate excess oxidants, which may result in oxidative stress and oxidative tissue damage [Citation57]. Therefore, detection of MPO expression can reflect the function and active status of neutrophils in response to stimuli from external environments, such as tumors [Citation58]. Molecular imaging probes integrated with MPO can be retained at the site of inflammation through MPO-induced dimerization and protein attachment [Citation59]. Based on this feature, assembled 5-hydroxytryptamine (5-HT)-equipped NPs were loaded with a photosensitizer and the leukotriene inhibitor zileuton to construct a targeted MPO. The constructed MPO-Gd is an activatable molecular MRI agent specific for the noninvasive detection of MPO activity in neutrophils [Citation60]. Similarly, activity was monitored using the imaging agent indium-111-bis-5-hydroxytryptamide-diethylenetriaminepentaacetate, which is oligomerized and binds to MPO. SPECT/CT has been used in animal studies. This approach allows for the detection of subtle underlying lesions for which conventional imaging has failed to demonstrate a surgical target [Citation61]. PET imaging was performed. The mouse was intravenously (i.v.) injected with 300–800 μCi of 18F-MAPP (myeloperoxidase-activatable PET probe) under anesthesia (~2% isoflurane in oxygen). An 18F-myeloperoxidase-activatable PET probe can be used as a radioactive probe to quantify changes in MPO activity. All images were obtained using a Siemens Inveon PET-CT scanner [Citation62]. An activatable construct conjugated to a biotin moiety (MPO-activatable biotinylated sensor, MABS) containing MPO-specific biotin moieties was validated in terms of specificity and sensitivity in combination with streptavidin-labeled fluorescent agents and Au NP imaging [Citation63]. Fluorescence imaging and CT imaging were performed with different secondary probes to prove that MABS can be applied to create systems suitable for imaging MPO activities on various scales and environments. Bioluminescence imaging (BLI) enables the tracking of tumor progression and immune response in real-time [Citation64,Citation65]. Using the In Vivo Imaging System (IVIS) 100 bioluminescence imaging system (Caliper Life Sciences) (f/stop, 1; no optical filter) for in vitro, ex vivo, and in vivo experiments, Gross et al. proved that luminol-BLI provides a specific and highly sensitive noninvasive diagnostic method for quantitatively monitoring MPO activity.
Neutrophil elastase (NE) belongs to the class of serine proteases and is physiologically located in anilinophil blue granules of neutrophils. It is mainly involved in neutrophil migration and promotes the expression of inflammatory factors such as IL-2, IL-6, and IL-8 to regulate the inflammatory response [Citation66]. However, activated neutrophils release large amounts of elastase outside the cell, causing degradation of elastin, proteoglycans, and collagen, resulting in tissue, cellular, and vascular basement membrane damage [Citation67]. Recently, Zhang et al. developed an activatable semiconducting polymer nanoprobe (SPCy) for near-infrared fluorescence and photoacoustic (PA) imaging of NE, a biomarker for tumor-associated neutrophils (TANs) [Citation68]. The images were obtained using near-infrared fluorescence and PA imaging. Thus, these data have provided an optical molecular imaging approach to facilitate the noninvasive monitoring and screening of cancer immunotherapeutics. Mitra et al. used an enzyme-activatable fluorogenic probe, neutrophil elastase 680 FAST (NE680), for the in vivo imaging of NE activity [Citation69]. Mice were anesthetized throughout the imaging process and imaged using an IVIS Spectrum System (PerkinElmer). The accumulation of activated neutrophils can be qualitatively tracked using a fluorescent reporter for the extracellular release of NE.
In addition to the studies described above (), the myeloid cell marker CD11b can be explored as a radioactive target for different types of myeloid cells, including monocytes, macrophages, or neutrophils [Citation70]. Myeloid cells are extensively involved in the formation and progression of tumors and their metastasis. The infiltration of a large number of CD11b+ myeloid cells significantly inhibited tumor growth, and some metastases shrank or even disappeared [Citation71]. The degree of CD11b+ tumor-infiltrating antigen-presenting cell infiltration was significantly correlated with the tumor volume, degree of venous infiltration, lymph node metastasis, and tumor stage. Patients with a high degree of CD11b+ tumor-infiltrating antigen-presenting cell infiltration were less effective than those with a low degree of infiltration, suggesting that CD11b+ cell infiltration is an independent prognostic factors for gastric cancer [Citation72,Citation73]. Hoffmann et al. modified the previously reported intracellular labeling method and labeled in vitro-generated polymorphonuclear (PMN-) and monocyte (M-) MDSCs for PET using [Citation71]Cu-labeled 1,4,7-triazacyclononane-triacetic acid (NOTA)-modified CD11b-specific monoclonal antibody (mAb) imaging. Radiolabeled PMN- and M-MDSCs ([64Cu]PMN-MDSCs and [64Cu]M-MDSCs, respectively) were adoptively transferred to experimental animals with primary/metastatic breast cancer and melanoma. Static PET and anatomical MR images were obtained 3, 24, and 48 h after cell injection. Their data implied that NOTA could be used to modify the CD11b-specific monoclonal antibody, which is feasible for labeling neutrophils and observed by PET [Citation74].
Discussion
Neutrophils are the dominant cells that directly reflect the degree of the inflammatory response by secreting multiple cytokines, chemokines, and ROS. These molecules help evaluate tumor treatment [Citation75]. With the development of imaging technology, noninvasive visualization of neutrophil clusters and analysis of the biological functions of neutrophils have been widely utilized in clinical/preclinical research. As the first cells to migrate to damaged sites, it is necessary to noninvasively determine how neutrophil migration dynamics and inflammatory responses are self-resolved. Georgantzoglou et al. combined promoter sequences from lysozyme C and MPO genes with a single fluorescent protein, such as green fluorescent protein (GFP), a tandem fluorescent timer, such as fast-folding GFP and a slower maturing tag red fluorescent protein (tagRFP), or bicistronic expression of reporter GFP and a control membrane marker to construct multiple fluorescent tags to visualize neutrophil migration patterns during wound responses [Citation76].
The neutrophil-based imaging assay has yielded interesting results in inflammation and tumors. However, studies with a larger sample size need to be conducted, and the system needs to be further validated as to whether it is safe and effective in the clinical setting. Some labeling systems or detection platforms do not necessarily capture images of whole tissues or organs and may need to be combined with other technologies. Although studies have demonstrated that nanoparticles such as USPIO do not affect disease severity or morbidity, suggesting that particle labeling does not greatly interfere with immune cell function, related research still warrants further development. Regardless of the challenges involved, neutrophil-based live-cell probes and bionanoprobes have a bright future in the treatment of inflammatory diseases and cancer.
Noninvasive observation of neutrophil recruitment and behavior in the inflammatory or tumor microenvironment using imaging techniques will provide pivotal insights into the effectiveness of disease treatment and directly reveal potential cellular culprits of disease. In addition, we could directly identify the locations within the microvascular network where neutrophil adhesion occurs and where neutrophils leave the vascular system and enter the tissue. These imaging approaches will play an integral role in the development of personalized therapies and the design and optimization of treatments, ultimately improving tumor clearance rates.
Statements of ethical approval
This research type is a review article and does not include human or animal experiments, so there is no ethical approval.
Acknowledgements
This research work supported by Natural Science Foundation (20180550488 and 2020-ZLLH-38 to Yiming Meng) of Liaoning Province, Young and middle-aged technological innovation talents in Shenyang of Yiming Meng (RC200491), Excellent Talent Fund of Liaoning Province Cancer Hospital and Medical-Engineering Joint Fund for Liaoning Province Cancer Hospital and Dalian University of Technology (LD202117) of Yiming Meng.
Disclosure statement
No potential conflict of interest was reported by the author(s).
Additional information
Funding
References
- Kanwore K, Kanwore K, Adzika GK, et al. Cancer metabolism: the role of immune cells epigenetic alteration in tumorigenesis, progression, and metastasis of glioma. Front Immunol. 2022;13:831636.
- Ma Q, Immler R, Pruenster M, et al. Soluble uric acid inhibits beta2 integrin-mediated neutrophil recruitment in innate immunity. Blood. 2022;139:3402–3417.
- Mahmud Z, Rahman A, Mishu ID, et al. Mechanistic insights into the interplays between neutrophils and other immune cells in cancer development and progression. Cancer Metastasis Rev. 2022;41:405–432.
- Ye D, Yao J, Du W, et al. Neutrophil extracellular traps mediate acute liver failure in regulation of miR-223/neutrophil elastase signaling in mice. Cell Mol Gastroenterol Hepatol. 2022;14:587–607.
- Jiang ZZ, Peng ZP, Liu XC, et al. Neutrophil extracellular traps induce tumor metastasis through dual effects on cancer and endothelial cells. Oncoimmunology. 2022;11:2052418.
- Tsukasaki Y, Toth PT, Davoodi-Bojd E, et al. Quantitative pulmonary neutrophil dynamics using computer-vision stabilized intravital imaging. Am J Respir Cell Mol Biol. 2022;66:12–22.
- Zhang X, Jiang C, He T, et al. Engineering molecular probes for in vivo near-infrared fluorescence/photoacoustic duplex imaging of human neutrophil elastase. Anal Chem. 2022;94:3227–3234.
- Tang C, Wang Q, Li K, et al. A neutrophil-mimetic magnetic nanoprobe for molecular magnetic resonance imaging of stroke-induced neuroinflammation. Biomater Sci. 2021;9:5247–5258.
- Mirili C, Guney IB, Paydas S, et al. Prognostic significance of neutrophil/lymphocyte ratio (NLR) and correlation with PET-CT metabolic parameters in small cell lung cancer (SCLC). Int J Clin Oncol. 2019;24:168–178.
- Alagappan M, Pollom EL, von Eyben R, et al. Albumin and Neutrophil-Lymphocyte Ratio (NLR) predict survival in patients with pancreatic adenocarcinoma treated with SBRT. Am J Clin Oncol. 2018;41:242–247.
- Pemberton HG, Collij LE, Heeman F, et al. Quantification of amyloid PET for future clinical use: a state-of-the-art review. Eur J Nucl Med Mol Imaging. 2022;49:3508–3528.
- Filippi L, Schillaci O. Total-body [(18)F]FDG PET/CT scan has stepped into the arena: the faster, the better. Is it always true? Eur J Nucl Med Mol Imaging. 2022;49:3322–3327.
- Schwenck J, Schorg B, Fiz F, et al. Cancer immunotherapy is accompanied by distinct metabolic patterns in primary and secondary lymphoid organs observed by non-invasive in vivo (18)F-FDG-PET. Theranostics. 2020;10:925–937.
- Wang Y, Zhao N, Wu Z, et al. New insight on the correlation of metabolic status on (18)F-FDG PET/CT with immune marker expression in patients with non-small cell lung cancer. Eur J Nucl Med Mol Imaging. 2020;47:1127–1136.
- Xu J, Li Y, Hu S, et al. The significant value of predicting prognosis in patients with colorectal cancer using (18)F-FDG PET metabolic parameters of primary tumors and hematological parameters. Ann Nucl Med. 2019;33:32–38.
- Sanduleanu S, Jochems A, Upadhaya T, et al. Non-invasive imaging prediction of tumor hypoxia: a novel developed and externally validated CT and FDG-PET-based radiomic signatures. Radiother Oncol. 2020;153:97–105.
- Kinoshita T, Fujii H, Hayashi Y, et al. Prognostic significance of hypoxic PET using (18)F-FAZA and (62)Cu-ATSM in non-small-cell lung cancer. Lung Cancer. 2016;91:56–66.
- Alongi P, Laudicella R, Lanzafame H, et al. PSMA and choline PET for the assessment of response to therapy and survival outcomes in prostate cancer patients: a systematic review from the literature. Cancers (Basel). 2022;14:1770.
- Park JA, Lee YJ, Lee JW, et al. Evaluation of [(89)Zr]-Oxalate as a PET tracer in inflammation, tumor, and rheumatoid arthritis models. Mol Pharm. 2016;13:2571–2577.
- Cristinziano L, Modestino L, Antonelli A, et al. Neutrophil extracellular traps in cancer. Semin Cancer Biol. 2022;79:91–104.
- Locke LW, Chordia MD, Zhang Y, et al. A novel neutrophil-specific PET imaging agent: cFLFLFK-PEG-64Cu. J Nucl Med. 2009;50:790–797.
- Pellico J, Lechuga-Vieco AV, Almarza E, et al. In vivo imaging of lung inflammation with neutrophil-specific (68)Ga nano-radiotracer. Sci Rep. 2017;7:13242.
- Kong Y, Liu K, Hua T, et al. PET imaging of neutrophils infiltration in Alzheimer’s DISEASE TRANSGENIC MICE. Front Neurol. 2020;11:523798.
- Zhang Y, Xiao L, Chordia MD, et al. Neutrophil targeting heterobivalent SPECT imaging probe: cFLFLF-PEG-TKPPR-99mTc. Bioconjug Chem. 2010;21:1788–1793.
- Chen J, Cheng H, Dong Q, et al. [99mTc]cFLFLF for early diagnosis and therapeutic evaluation in a rat model of acute osteomyelitis. Mol Imaging Biol. 2015;17:337–344.
- Xiao L, Ding M, Zhang Y, et al. A novel modality for functional imaging in acute intervertebral disk herniation via tracking leukocyte infiltration. Mol Imaging Biol. 2017;19:703–713.
- Dudchenko N, Pawar S, Perelshtein I, et al. Magnetite nanoparticles: synthesis and applications in optics and nanophotonics. Materials (Basel). 2022;15:2601 .
- Savla R, Minko T. Nanoparticle design considerations for molecular imaging of apoptosis: diagnostic, prognostic, and therapeutic value. Adv Drug Deliv Rev. 2017;113:122–140.
- Thiruppathi R, Mishra S, Ganapathy M, et al. Nanoparticle functionalization and its potentials for molecular imaging. Adv Sci (Weinh). 2017;4:1600279.
- Cerqueira M, Belmonte-Reche E, Gallo J, et al. Magnetic solid nanoparticles and their counterparts: recent advances towards cancer theranostics. Pharmaceutics. 2022;14:506.
- Lin PC, Lin S, Wang PC, et al. Techniques for physicochemical characterization of nanomaterials. Biotechnol Adv. 2014;32:711–726.
- Vishnevskiy DA, Garanina AS, Chernysheva AA, et al. Neutrophil and nanoparticles delivery to tumor: is it going to carry that weight? Adv Healthc Mater. 2021;10:e2002071.
- Lyu J, Wang L, Bai X, et al. Treatment of rheumatoid arthritis by serum albumin nanoparticles coated with mannose to target neutrophils. ACS Appl Mater Interfaces. 2021;13:266–276.
- Singh S, Zhang XHF, Rosen JM. TIME is a great healer-targeting myeloid cells in the tumor immune microenvironment to improve triple-negative breast cancer outcomes. Cells. 2020;10.
- Qiu Q, Wen Y, Dong H, et al. A highly sensitive living probe derived from nanoparticle-remodeled neutrophils for precision tumor imaging diagnosis. Biomater Sci. 2019;7:5211–5220.
- Sun R, Wang X, Nie Y, et al. Targeted trapping of endogenous endothelial progenitor cells for myocardial ischemic injury repair through neutrophil-mediated SPIO nanoparticle-conjugated CD34 antibody delivery and imaging. Acta Biomater. 2022;146:421–433. doi:10.1016/j.actbio.2022.05.003.
- Zheng H, Yuan C, Cai J, et al. Early diagnosis of breast cancer lung metastasis by nanoprobe-based luminescence imaging of the pre-metastatic niche. J Nanobiotechnology. 2022;20:134. doi:10.1186/s12951-022-01346-4.
- Yan X, Lin W, Liu H, et al. Wavelength-Tunable, Long Lifetime, and Biocompatible Luminescent Nanoparticles Based on a Vitamin E-Derived Material for Inflammation and Tumor Imaging. Small. 2021;17:e2100045. doi:10.1002/smll.202100045.
- Chandrasekharan P, Fung KLB, Zhou XY, et al. Non-radioactive and sensitive tracking of neutrophils towards inflammation using antibody functionalized magnetic particle imaging tracers. Nanotheranostics. 2021;5:240–55. doi:10.7150/ntno.50721.
- Penate-Medina T, Damoah C, Benezra M, et al. Alpha-MSH Targeted Liposomal Nanoparticle for Imaging in Inflammatory Bowel Disease (IBD). Curr Pharm Des. 2020;26:3840–6. doi:10.2174/1381612826666200727002716.
- Tang L, Wang Z, Mu Q, et al. Targeting Neutrophils for Enhanced Cancer Theranostics. Adv Mater. 2020;32:e2002739. doi:10.1002/adma.202002739.
- Tang C, Wang C, Zhang Y, et al. Recognition, Intervention, and Monitoring of Neutrophils in Acute Ischemic Stroke. Nano Lett. 2019;19:4470–7. doi:10.1021/acs.nanolett.9b01282.
- Kirschbaum K, Sonner JK, Zeller MW, et al. In vivo nanoparticle imaging of innate immune cells can serve as a marker of disease severity in a model of multiple sclerosis. Proc Natl Acad Sci U S A. 2016;113:13227–13232.
- Wu M, Zhang H, Tie C, et al. MR imaging tracking of inflammation-activatable engineered neutrophils for targeted therapy of surgically treated glioma. Nat Commun. 2018;9:4777.
- Qiu Y, Palankar R, Echeverria M, et al. Design of hybrid multimodal poly(lactic-co-glycolic acid) polymer nanoparticles for neutrophil labeling, imaging and tracking. Nanoscale. 2013;5:12624–12632.
- Liu X, Wu M, Wang M, et al. AIEgen-Lipid conjugate for rapid labeling of neutrophils and monitoring of their behavior. Angew Chem Int Ed Engl. 2021;60:3175–3181.
- Pardo A, Gomez-Florit M, Barbosa S, et al. Magnetic nanocomposite hydrogels for tissue engineering: design concepts and remote actuation strategies to control cell fate. ACS Nano. 2021;15:175–209.
- Wang Q, Ren Y, Mu J, et al. Grapefruit-Derived nanovectors use an activated leukocyte trafficking pathway to deliver therapeutic agents to inflammatory tumor sites. Cancer Res. 2015;75:2520–2529.
- Kang T, Zhu Q, Wei D, et al. Nanoparticles coated with neutrophil membranes can effectively treat cancer metastasis. ACS Nano. 2017;11:1397–1411.
- Phipps MC, Huang Y, Yamaguchi R, et al. In vivo monitoring of activated macrophages and neutrophils in response to ischemic osteonecrosis in a mouse model. J Orthop Res. 2016;34:307–313.
- Sun L, Zhou JE, Luo T, et al. Nanoengineered neutrophils as a cellular sonosensitizer for visual sonodynamic therapy of malignant tumors. Adv Mater. 2022;34:e2109969.
- Garcia-Mendoza MG, Inman DR, Ponik SM, et al. Neutrophils drive accelerated tumor progression in the collagen-dense mammary tumor microenvironment. Breast Cancer Res. 2016;18:49.
- Nguyen CT, Furuya H, Das D, et al. Peripheral gammadelta T cells regulate neutrophil expansion and recruitment in experimental psoriatic arthritis. Arthritis Rheumatol. 2022;74:1524–1534.
- Chu KK, Kusek ME, Liu L, et al. Illuminating dynamic neutrophil trans-epithelial migration with micro-optical coherence tomography. Sci Rep. 2017;8:45789.
- Turk M, Naumenko V, Mahoney DJ, et al. Tracking cell recruitment and behavior within the tumor microenvironment using advanced intravital imaging approaches. Cells. 2018;7:69.
- Wang G, Nauseef WM. Neutrophil dysfunction in the pathogenesis of cystic fibrosis. Blood. 2022;139:2622–2631.
- Kargapolova Y, Geissen S, Zheng R, et al. The enzymatic and non-enzymatic function of Myeloperoxidase (MPO) in inflammatory communication. Antioxidants (Basel). 2021;10. DOI:10.3390/antiox10040562
- Zhang N, Aiyasiding X, Li WJ, et al. Neutrophil degranulation and myocardial infarction. Cell Commun Signal. 2022;20:50.
- Campuzano S, Pedrero M, Yanez-Sedeno P, et al. Nanozymes in electrochemical affinity biosensing. Mikrochim Acta. 2020;187:423.
- Pulli B, Wojtkiewicz G, Iwamoto Y, et al. Molecular MR imaging of myeloperoxidase distinguishes steatosis from steatohepatitis in nonalcoholic fatty liver disease. Radiology. 2017;284:390–400.
- Zhang Y, Seeburg DP, Pulli B, et al. Myeloperoxidase nuclear imaging for epileptogenesis. Radiology. 2016;278:822–830.
- Wang C, Keliher E, Zeller MWG, et al. An activatable PET imaging radioprobe is a dynamic reporter of myeloperoxidase activity in vivo. Proc Natl Acad Sci U S A. 2019;116:11966–11971.
- Wang C, Pulli B, Jalali Motlagh N, et al. A versatile imaging platform with fluorescence and CT imaging capabilities that detects myeloperoxidase activity and inflammation at different scales. Theranostics. 2019;9:7525–7536.
- Huang M, Huang X, Huang N. Exosomal circGSE1 promotes immune escape of hepatocellular carcinoma by inducing the expansion of regulatory T cells. Cancer Sci. 2022;113:1968–1983.
- Gross S, Gammon ST, Moss BL, et al. Bioluminescence imaging of myeloperoxidase activity in vivo. Nat Med. 2009;15:455–461.
- Blum C, Taskin MB, Shan J, et al. Appreciating the first line of the human innate immune defense: a strategy to model and alleviate the neutrophil elastase-mediated attack toward bioactivated biomaterials. Small. 2021;17:e2007551.
- Margaroli C, Madison MC, Viera L, et al. An in vivo model for extracellular vesicle-induced emphysema. JCI Insight. 2022;7. DOI:10.1172/jci.insight.153560.
- Zhang Y, He S, Xu C, et al. An activatable polymeric nanoprobe for fluorescence and photoacoustic imaging of tumor-associated neutrophils in cancer immunotherapy. Angew Chem Int Ed Engl. 2022;61:e202203184.
- Mitra S, Modi KD, Foster TH. Enzyme-activatable imaging probe reveals enhanced neutrophil elastase activity in tumors following photodynamic therapy. J Biomed Opt. 2013;18:101314.
- Zhang X, Baht GS, Huang R, et al. Rejuvenation of neutrophils and their extracellular vesicles is associated with enhanced aged fracture healing. Aging Cell. 2022;21:e13651.
- Reeves BN, Beckman JD. Novel pathophysiological mechanisms of thrombosis in myeloproliferative neoplasms. Curr Hematol Malig Rep. 2021;16:304–313.
- Urakawa S, Yamasaki M, Goto K, et al. Peri-operative monocyte count is a marker of poor prognosis in gastric cancer: increased monocytes are a characteristic of myeloid-derived suppressor cells. Cancer Immunol Immunother. 2019;68:1341–1350.
- Okita Y, Tanaka H, Ohira M, et al. Role of tumor-infiltrating CD11b+ antigen-presenting cells in the progression of gastric cancer. J Surg Res. 2014;186:192–200.
- Hoffmann SHL, Reck DI, Maurer A, et al. Visualization and quantification of in vivo homing kinetics of myeloid-derived suppressor cells in primary and metastatic cancer. Theranostics. 2019;9:5869–5885.
- Tricarico D, Convertino AS, Mehmeti I, et al. Inflammatory related reactions in humans and in canine breast cancers, A spontaneous animal model of disease. Front Pharmacol. 2022;13:752098.
- Georgantzoglou A, Coombs C, Poplimont H, et al. Live imaging of chemokine receptors in zebrafish neutrophils during wound responses. J Vis Exp. 2020. DOI:10.3791/61679