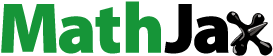
ABSTRACT
Plastic deformation and fracture behavior of Cu/V nanoscale metallic multilayers (NMMs) were systematically investigated through in situ transmission electron microscopy testing. Two different types of interfaces: sharp interfaces and interfacial transition zones (ITZs), formed in the as-fabricated Cu/V NMMs. Upon deformation, sharp interfaces and ITZs exert distinct influences on the fracture behavior of Cu/V NMMs. Sharp interfaces can impede the crack propagation by deflecting the crack propagation and blunting the crack tip, while the ITZs can induce microcracks and facilitate the fracture process. These findings shed light on the important roles of interface structures in the deformation of a broad class of NMMs.
GRAPHICAL ABSTRACT
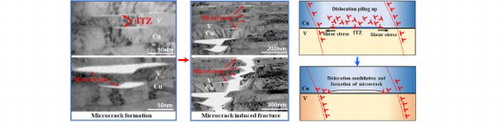
IMPACT STATEMENT
In situ nanomechanical testing reveals critical roles of interface structures on the fracture of Cu/V NMMs, where sharp interfaces imped and deflect cracks but interfacial transition zones facilitate the fracture greatly.
1. Introduction
Nanoscale metallic multilayers (NMMs) possess enhanced structural stability, mechanical properties and radiation tolerance, which have important applications under extreme conditions [Citation1–3]. It is well-established that these outstanding properties of NMMs originate from high density of interfaces, which act as practical sites for nucleation and annihilation of crystal defects, as well as the barriers for dislocation motion [Citation1,Citation3]. For NMMs composed of two immiscible metals (e.g. Cu/Nb, Cu/Ta and Cu/V), they usually possess atomically ordered and chemically sharp interfaces disregarding fabrication methods (e.g. physical vapor deposition (PVD), electron beam evaporation and accumulative roll bonding (ARB)) [Citation2,Citation4,Citation5]. Nevertheless, the fabrications based on severe plastic deformation like ARB may cause intermixing of two immiscible metals at interfaces due to mechanical alloying [Citation6,Citation7]. For instance, deformation-induced interfacial transition zones (ITZs) were observed in Cu/V multilayers fabricated by cross ARB method (CARB) [Citation8], which were identified to be deformation-induced supersaturated solid solutions. Due to the difference in the composition and phase structure of ITZs from adjacent layers, they may significantly influence the mechanical responses of NMMs. Past decades have witnessed remarkable progresses in improving the crack resistance of materials via tailoring lamellar structures. Lots of works have focused on understanding the effects of interface structure and length scale on the mechanical properties of NMMs [Citation9,Citation10]. For example, hierarchical and laminated structures of human bones were reported to be effective in hampering the crack propagation [Citation11,Citation12]; NMMs with tunable layer thickness (h) and modulation ratio (η = hx/hCu) are of increasing significance in academic researches and industrial applications [Citation13,Citation14]. Yet, few attentions were paid to the influence of uncommon interfacial transition zones that widely existed in the NMMs.
In this study, we investigated the effects of interface structures on the deformation and fracture of CARBed Cu/V NMMs using the state-of-the-art in situ transmission electron microscopy (TEM) mechanical testing. It shows that the sharp interfaces and ITZs exert different influences on the fracture behavior. Specifically, crack transmits across the layers where the interfaces possess atomistic sharp structure, while microcracks occur between ITZs and Cu lamella, facilitating the fracture. The formation of the microcracks can be attributed to three factors: (1) slip discontinuity induced deformation incompatibility; (2) weak bonding strength between Cu and ITZ; (3) dislocation-interface interaction.
2. Experimental methods
Cu/V multilayers were fabricated via the CARB method [Citation4]. Before CARB, commercial pure Cu (99.95 wt%) and V (99.95 wt%) strips were cut into dimensions of 40 mm × 60 mm × 1 mm in width, length and thickness, and then annealed at 600° for 1 h and 900° for 2 h, respectively. After surface cleaning, these strips were stacked into a sandwich structure of Cu-V-Cu for subsequent mechanical rolling at room temperature. After each rolling, the obtained sample was cut into half along the transverse direction (TD), rotated for 90°, surface-cleaned, annealed at 700°C for 180 min and then stacked together for further rolling. No thermal treatment was applied after the final rolling. Figure (a) schematically shows our experimental setup. The cross-section specimens of Cu/V NMMs were prepared by the focused ion beam (FIB) method. The dimensions of the tested thin film samples are ∼2 µm wide, ∼5 µm high and ∼60 nm thick. A notch with average width of 200 nm was pre-prepared at the bottom of the sample. All in situ mechanical testing was conducted inside a Cs-corrected TEM (FEI Titan G2 60-300) operating at 300 kV. A PicoFemto TEM-STM platform from Zeptools technology Co. was used in the in situ nanomechanical testing. In a typical experiment, one end of the sample was fixed on the static side of the holder (the right in Figure a), while an L-shaped W probe was applied to hold the beam on the sample end (the left in Figure a); a uniaxial tension loading was applied to the sample through the backward motion of the W probe driven by a pizeo-controller. In situ mechanical testing was performed at room temperature, and the W probe moved at a constant velocity of ∼10 nm/s, giving an estimated strain rate of ∼2×10−3 s−1. Five individual samples were tested using the above method, and twenty samples were fabricated by FIB for structure characterizations. Noted that the applied uniaxial loading would evolve with the deformation, which became a non-uniaxial one as the crack opening increased.
3. Results
Figure (a) shows representative TEM images of Cu/V NMMs fabricated by CARB. Both Cu and V layers are severely thinned along the rolling direction (RD) during the CARB process, resulting in uniform layer thickness of ∼80 nm. Two distinct interface structures are observed in the as-fabricated samples (Figure b), i.e. the atomically sharp interfaces (Figure c) and the uncommon ITZs (Figure d). The ITZs have an average thickness of ∼5.2 nm and contribute to ∼30% of the total interfaces in Cu/V NMMs. Atomistic characterizations show ITZs possess a cubic structure with a lattice constant of ∼3.08 Å, different from that of either Cu or V [Citation8]. It shows that the Cu and V layers possess an orientation relationship of [011]Cu||[111]V at the sharp interfaces (Figure c and e), while the ITZs show an orientation relationship of [130]Cu||[001]ITZ||[110]V with well-defined crystalline lattices, dense dislocations and stepwise boundaries (Figure d and f), different from the amorphous ribbons observed in Cu/Ta multilayers [Citation15] and Cu–Nb–Ag composite wires [Citation16]. The mismatch between {112}Cu and {110}V planes across the sharp interfaces is calculated to be 30.8%, while the mismatch is calculated to be 48.4% between {113}Cu and {110}ITZ planes across the ITZs, and 3.6% between {110}V and {110}ITZ planes across the ITZs. It can be seen that the interfaces between V and ITZs show better coherency than those between Cu and ITZs (Figure d and f), suggesting ITZs are formed based on the lattice of V, where Cu is dissolved in the lattice of V solvent. The significant differences between lattice mismatch, as well as the slip continuity across these interfaces, can strongly influence the mechanical responses of sharp interfaces and ITZs upon mechanical loading.
Figure 1. Interface structures in the as-fabricated Cu/V NMMs. (a–b) Typical TEM images of sharp interfaces and ITZs. (c–d) HRTEM images of sharp interfaces and ITZs. (e–f) Orientation relationships near the sharp interfaces and ITZs.
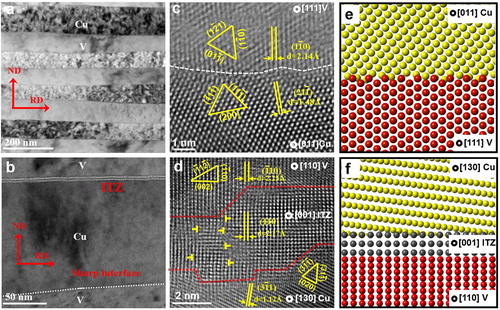
Sharp interfaces and ITZs possess distinct interface coherency and orientation relationship, which can exert strong influence on the dislocation glide across the interface and thereby control the deformation and fracture of Cu/V NMMs. In situ TEM mechanical testing further reveals the effects of interface structures on the plastic deformation and fracture of the CARBed Cu/V NMMs (see Figure a, b for the experimental setup). Before testing, the as-fabricated Cu/V NMM shows uniform layered structure without pre-existing crack or necking (Figure c). Upon deformation, some microcracks occurred firstly in front of the crack tip initiated from the pre-existing notch (Figure d–e). These microcracks gradually developed with the loading and caused the interface delamination, which finally linked together and resulted in the fast propagation of the crack (Figure e–f). It is noticed that deflections of crack occurred frequently at the interface during its propagation (Figure f), which might be correlated to the unparallel slip planes in Cu and V layers.
Figure 2. Fracture testing design and sequential TEM images of fracture behavior of Cu/V NMMs. (a) Schematic of in situ testing design. (b) Typical morphology of FIB-fabricated sample. (c–f) Microcrack-mediated fracture process in Cu/V NMMs.
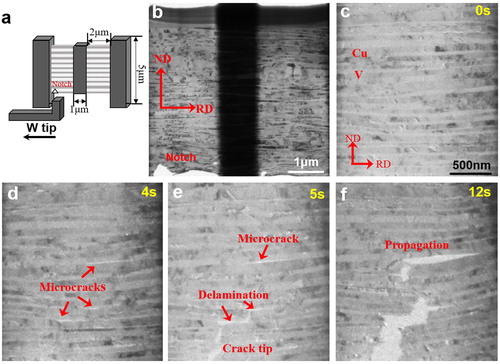
Zoomed-in observations further reveal that the ITZs significantly influence the fracture of Cu/V NMMs by inducing interface delamination (Figure ). Upon loading, the regions near ITZs deformed first due to the pre-existing dislocations, which induced the initiation of interfacial microcracks with increasing stress concentration at ITZs (Figure a, b). These microcracks mainly propagated along the interfaces, inducing interface cleavage and thereby isolating a V layer in between. Subsequently, the isolated V layer fractured via the slip offset induced shear localization (Figure c, d). Meanwhile, a surface crack was emitted at the tip of the prefabricated notch (Figure c), which blunted quickly due to the blockage of sharp interface (Figure d). With continuing loading, numerous microcracks initiated at other ITZ regions in front of the as-formed cracks (see the yellow arrows in Figure e). As microcracks developed, both Cu and V layers between these microcracks deformed in the same manner shown in Figure (c) (i.e. shear localization), which resulted in the rapid linkage of these microcracks and thereby a catastrophic fracture of the sample. Post-fracture observation confirms that the crack pathway was developed preferentially along the microcracks, see the interface delamination on the fracture surface (Figure f).
Figure 3. Sequential TEM images of ITZ-mediated fracture in Cu/V NMMs. (a) Sample morphology before loading. (b–f) Zoomed-in TEM images of ITZs-mediated fracture process. (g–i) Fracture of Cu/V NMMs dominated by the sharp interfaces. (j) Zoomed-in TEM images of the fracture surfaces.
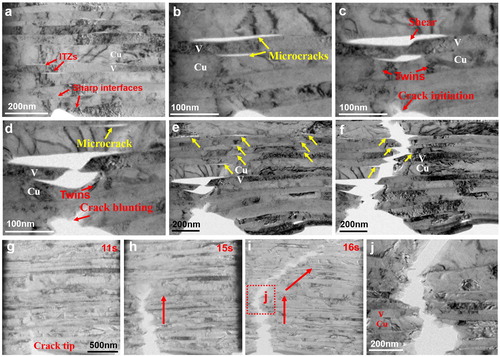
To elucidate the behavior of sharp interfaces under the same loading condition, here we present a set of in situ fracture testing dominated by sharp interfaces (The sample was obtained by chance in a sharp interface dominated region), as shown in Figure (g–j). Before testing, the as-fabricated Cu/V NMM showed uniform layered structure without pre-existing crack or shear induced necking. Upon loading, a crack initiated from the prefabricated notch (Figure g) and propagated into the film along the overall ND direction (Figure h), resulting in the fracture (Figure i). The whole fracture process can be divided into two stages: mode I (open) fracture and mode II (shear) fracture. It is noticed that deflections of crack occurred frequently at sharp interfaces (Figure j), which might be correlated to the unparallel slip planes in Cu and V layers. These deflections were considered effective in toughening layered structures [Citation17]. As the crack further propagated, the overall crack pathway was tuned gradually toward the RD direction, resulting in the change of fracture model from mode I fracture to mode II fracture. The change of overall crack pathway can be ascribed to the deviation of applied loading from the initial condition as the crack propagating, which can induce a localized shear at the crack tip. It needs to note that no interface decohesion occurred at sharp interfaces, in contrast to the ITZ-dominated deformation. These comparisons suggest the significant influence of interface structure on the deformation and fracture of Cu/V NMMs.
4. Discussions
Mechanical alloying induced intermixing between component metals may occur in NMMs fabricated by severe plastic deformation, where dense dislocation activities in the confined layers facilitate the mass transport toward the bimetal interfaces [Citation6,Citation8]. The slip transmissibility across an interface of NMMs are determined by the geometric factor:
where ω is the minimum angle between two Burgers vectors and θ is the minimum angle between the traces that the slip planes make with the interface, and ωc (∼60°) and θc (∼15°) are the critical angles for ω and θ for the direct transmission of incoming dislocation, respectively [Citation18,Citation19]. In our Cu/V samples, the slip continuity is strongly influenced by interface structures (Figure a). The continuity of slip systems at sharp interfaces is better than that of ITZs; hence, dislocation transmission is more likely to occur at sharp interfaces, rather than ITZs. During the severe plastic deformation of CARB, dislocations can transmit the [110] Cu || [111] V interfaces easily, but are more likely to be blocked and annihilated at the [130] Cu || [110] V interfaces given the poor slip continuity [Citation18,Citation19]. With continuous dislocations annihilation, intermixing between Cu and V atoms may occur at the interface via the mechanical-driven mass transport [Citation8,Citation20]. It is known that the {001} facet of V is the preferential annihilation site for foreign impurity atoms (e.g. C, O and N) [Citation21]. At ITZs with the orientation relationship of [130] Cu || [110] V, {001} facets of V are readily parallel to the interface, making it preferential sites for Cu atoms to dissolve in (Figure d and f). With increasing Cu atoms dissolving into the lattice of V, it will be distorted by elongating along the < 001 > direction (Figure b), resulting in a layer of V-Cu solid solution with [001] orientation (i.e. ITZs).
Figure 4. Schematic of the effects of slip discontinuity. (a) Slip continuity at sharp interfaces and ITZs. (b) Schematic of the formation of ITZs. (c) Schematic of the fracture process dominated by different interfaces.
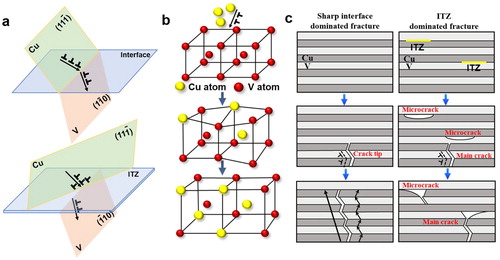
Recent studies revealed that interfaces play a significant role in mediating the plastic deformation and fracture of NMMs [Citation14,Citation22]. Typically, bi-metal interfaces of NMMs (e.g. Cu/Nb NMM) can effectively hamper the propagation of crack via multiple mechanisms [Citation23]. Moreover, the structure and coherency properties of bi-metal interfaces are known to determine the shear strength of interfaces, which significantly affect the mechanical responses of NMMs [Citation22,Citation24]. Nevertheless, previous studies mainly focused on the effects of regular interfaces on deformation of NMMs, largely neglecting the influences of widely-existed unconventional interfaces (e.g. amorphous interfaces and ITZs) [Citation8,Citation15]. Our experiments demonstrate that the regular sharp interfaces and uncommon ITZs play important yet complete different roles on the deformation and fracture of Cu/V NMMs. Sharp interfaces are effective in impeding the crack propagation. When the fracture process is exclusively controlled by sharp interfaces, the crack initiated at the artificial notch and propagated inwards the sample layer-by-layer (Figure c). When propagating across the interfaces, the crack was constantly deflected due to the different slip systems in Cu and V layers, making the sharp interfaces strong barriers for dislocation piling-up. This process can contribute to the interface sliding and thereby crack blunting (Figure d). As the stress concentration increases at crack tip, crack propagation occurs by dislocation slipping across the interface. The change of dislocation slip directions in Cu and V layers induces a zig-zag propagation of the crack, see Figure (c).
In stark contrast, ITZs accelerate the fracture process by inducing microcracks (Figures and ). The formation of microcracks at ITZs can be attributed to the following reasons: (1) slip discontinuity induced deformation incompatibility; (2) weak bonds between Cu layers and ITZs; (3) dislocation-interface interaction induced vacancies. Generally, the soft layers (e.g. Cu) of bi-metal NMMs can execute a constraining effect on the hard layers (e.g. Nb, Ta and V) through transmission of dislocation across the interface and then compatible deformation, which could suppress the deformation incompatibility and fracture [Citation13]. The level of the constraining effect/compatible deformation is controlled by the slip continuity across the interface. For interfaces with good slip continuity (i.e. sharp interfaces), dislocations can easily transmit across and stimulate the deformation of adjacent hard layer [Citation25]; whereas, interfaces with poor slip continuity (i.e. ITZs) can strongly impede the dislocation motion, resulting in the confined slip in separate layers and thereby deformation incompatibility at interfaces. In Cu/V NMMs, given the lower lattice resistance, the soft Cu layers often yield first upon loading and deform much easier than the V layers [Citation26], while the dislocation mobility in V is relatively low due to the high lattice resistance [Citation27], resulting in dislocation piling-up in V layers (Figure a). Further straining causes the slip transmission or emission of new dislocations at sharp interfaces, inducing stacking fault/deformation twinning in Cu layers (Figure a). For sharp interfaces, comparatively better slip continuity makes it preferable for twins to form by dislocation-twin transmission across the interface [Citation28]. Slip transmission or emission of partials left steps on the interface, and then these steps can be quickly compensated by the slip of dislocation from V layers to achieve a low-energy state, resulting in the change of interface morphology (similar to the interface reconstruction in Cu/Nb NMMs [Citation29]). Whereas, ITZs are comparatively opaque for dislocation transmitting due to the poor continuity of adjacent slip systems. In the regions with ITZs, the Cu layers yield first under mechanical loading and elongate gradually with the dislocation activities, while the V layers are less deformed, causing deformation incompatibility at the interfaces. This process is confirmed by the TEM observation at an ITZ away from the fracture surface, where dislocations in Cu layers were blocked by the ITZs and less dislocations were observed in adjacent V layers (Figure b). The deformation incompatibility between neighboring layers can generate a shear stress on the ITZs, facilitating the formation of microcracks.
Figure 5. Effects of sharp interfaces and ITZs on deformation. (a) Interface reconstruction at the sharp interface near the crack. (b) Dislocation piling up near the ITZs. (c) HRTEM images of fractured Cu and V layers bonded by sharp interfaces. (d) Fracture propagated along the ITZ. (e) Schematic of the formation mechanism of microcracks.
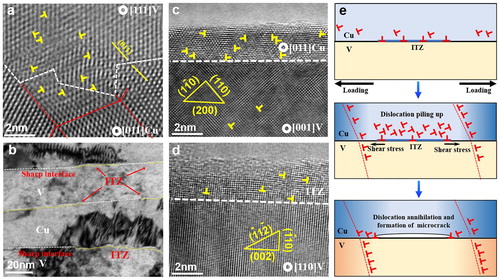
The difference in bonding strength between sharp interfaces and ITZs is another factor influencing the deformation. Post-fracture characterization indicates that interface delamination of Cu/V NMMs mainly occurred at ITZs, though crack propagation along sharp interface happened occasionally in some localized region near fracture surface. Figure (c) shows that the fracture at sharp interfaces often occurred in the Cu layer, resulting in a residual layer of Cu on V surface. In contrast, the fracture at ITZs usually happened along the interface between Cu and ITZs (Figure d), due to the microcrack-induced interface delamination. These observations suggest the bonding strength of sharp interfaces and V/ITZ should be much stronger than that of Cu/ITZ. The good bonding condition at V/ITZ may originate from that the ITZ is a V-based supersaturated solution. Another factor contributing to the formation of microcrack is the dislocation reaction induced vacancies. With the high density of preexisted dislocations in ITZs and deformation-induced dense dislocation activates near boundaries, dislocations may annihilate with each other quickly, resulting in vacancies. These deformation-induced vacancies accumulated at the interfaces [Citation6], contributing to the formation of microcracks (Figure e).
Above analysis suggests that the three mechanisms may synergize microcracking, while deformation incompatibility and dislocation-interface interaction (dislocations accumulation at ITZ interfaces) may dominate microcracking. In Cu/V NMMs, the soft Cu layers generally yield first upon loading, and dislocations in Cu layers will deposit at interfaces. For sharp Cu/V interfaces, these deposited dislocations can transmit into V layers, achieving co-deformation between Cu and V layers. However, ITZs are comparatively opaque for dislocation transmitting due to the poor continuity of adjacent slip systems, resulting in deformation incompatibility between ITZs and neighboring layers. These accumulated dislocations at Cu-ITZ interfaces may climb toward Cu-V interfaces associated with vacancy aggregation at Cu-ITZ interfaces [Citation30,Citation31], weakening Cu-ITZ interfaces, promoting interface decohesion, and resulting in microcracks.
5. Conclusion
This work systematically investigated the deformation and fracture behaviors of Cu/V NMMs fabricated by CARB. The as-fabricated NMMs contain two typical interfaces, conventional sharp interfaces and unconventional ITZs. The formation of ITZs can be attributed to the extreme discontinuity of the slip systems in Cu and V layers alongside the interface and severe stress statues. ITZs significantly affect the fracture of Cu/V NMMs by causing microcracks. The microcracks are induced by deformation incompatibility, weak bonding between Cu and ITZs, and dislocation-interface interaction. These founding are of great significance in understanding the mechanical properties of NMMs and shed light on the study of the nonconventional interfaces in all kinds of NMMs.
Acknowledgements
J. W. acknowledges the support of the National Key R&D Program of China (No.2018YFB2000704), the National Natural Science Foundation of China (Nos. 51771172 and 51701179) and the Innovation Fund of the Zhejiang Kechuang New Materials Research Institute (No. ZKN-18-Z02). X. P. acknowledges the support of the National Natural Science Foundation of China (No. 5171181).
Disclosure statement
No potential conflict of interest was reported by the author(s).
ORCID
Jian Wang http://orcid.org/0000-0001-5130-300X
Jiangwei Wang http://orcid.org/0000-0003-1191-0782
Additional information
Funding
References
- Lu K. Stabilizing nanostructures in metals using grain and twin boundary architectures. Nat Rev Mater. 2016;1:16019. doi: 10.1038/natrevmats.2016.19
- Han W, Demkowicz MJ, Mara NA, et al. Design of radiation tolerant materials via interface engineering. Adv Mater. 2013;25(48):6975–6979. doi: 10.1002/adma.201303400
- Zeng LF, Gao R, Fang QF, et al. High strength and thermal stability of bulk Cu/Ta nanolamellar multilayers fabricated by cross accumulative roll bonding. Acta Mater. 2016;110:341–351. doi: 10.1016/j.actamat.2016.03.034
- Zeng LF, Gao R, Xie ZM, et al. Development of interface-dominant bulk Cu/V nanolamellar composites by cross accumulative roll bonding. Sci Rep. 2017;7:40742. doi: 10.1038/srep40742
- Guo Q, Thompson GB. Evolution of in situ growth stresses and interfacial structure in phase changing Cu/V multilayered thin films. Acta Mater. 2018;148:63–71 doi: 10.1016/j.actamat.2018.01.023
- Ohsaki S, Kato S, Tsuji N, et al. Bulk mechanical alloying of Cu–Ag and Cu/Zr two-phase microstructures by accumulative roll-bonding process. Acta Mater. 2007;55(8):2885–2895. doi: 10.1016/j.actamat.2006.12.027
- Sauvage X, Dinda GP, Wilde G. Non-equilibrium intermixing and phase transformation in severely deformed Al/Ni multilayers. Scripta Mater. 2007;56(3):181–184. doi: 10.1016/j.scriptamat.2006.10.021
- Wei S, Zhang L, Zheng S, et al. Deformation-induced interfacial transition zone in Cu/V nanolamellar multilayers. Scripta Mater. 2019;159:104–108. doi: 10.1016/j.scriptamat.2018.09.031
- Li YP, Zhang GP. On plasticity and fracture of nanostructured Cu/X (X = Au, Cr) multilayers: the effects of length scale and interface/boundary. Acta Mater. 2010;58(11):3877–3887. doi: 10.1016/j.actamat.2010.03.042
- Wang Y, Li J, Hamza AV, et al. Ductile crystalline–amorphous nanolaminates. Proc Natl Acad Sci. 2007;104(27):11155–11160. doi: 10.1073/pnas.0702344104
- Koyama M, Zhang Z, Wang M, et al. Bone-like crack resistance in hierarchical metastable nanolaminate steels. Science. 2017;355(6329):1055–1057. doi: 10.1126/science.aal2766
- Gao H, Ji B, Jäger IL, et al. Materials become insensitive to flaws at nanoscale: lessons from nature. Proc Natl Acad Sci. 2003;100(10):5597–5600. doi: 10.1073/pnas.0631609100
- Zhang J, Zhang X, Wang R, et al. Length-scale-dependent deformation and fracture behavior of Cu/X (X = Nb, Zr) multilayers: the constraining effects of the ductile phase on the brittle phase. Acta Mater. 2011;59(19):7368–7379. doi: 10.1016/j.actamat.2011.08.016
- Zhu X, Zhang B, Gao J, et al. Evaluation of the crack-initiation strain of a Cu–Ni multilayer on a flexible substrate. Scripta Mater. 2009;60(3):178–181. doi: 10.1016/j.scriptamat.2008.10.004
- Wei MZ, Cao ZH, Shi J, et al. Evolution of interfacial structures and creep behavior of Cu/Ta multilayers at room temperature. Mater Sci Engin A. 2015;646:163–168. doi: 10.1016/j.msea.2015.08.068
- Raabe D, Ohsaki S, Hono K. Mechanical alloying and amorphization in Cu–Nb–Ag in situ composite wires studied by transmission electron microscopy and atom probe tomography. Acta Mater. 2009;57(17):5254–5263. doi: 10.1016/j.actamat.2009.07.028
- Koyama M, Zhang Z, Wang M, et al. Bone-like crack resistance in hierarchical metastable nanolaminate steels. Science. 2017;355(6329):1055–1057. doi: 10.1126/science.aal2766
- Beyerlein IJ, Mayeur JR, Zheng S, et al. Emergence of stable interfaces under extreme plastic deformation. Proc Natl Acad Sci. 2014;111(12):4386–4390. doi: 10.1073/pnas.1319436111
- Wang J, Zhou Q, Shao S, et al. Strength and plasticity of nanolaminated materials. Mater Res Lett. 2017;5(1):1–19. doi: 10.1080/21663831.2016.1225321
- Kormout KS, Pippan R, Bachmaier A. Deformation-induced supersaturation in immiscible material systems during high-pressure torsion. Adv Eng Mater. 2017;19(4):1600675. doi: 10.1002/adem.201600675
- Gong L, Su Q, Deng H, et al. The stability and diffusion properties of foreign impurity atoms on the surface and in the bulk of vanadium: a first-principles study. Comput Mater Sci. 2014;81:191–198. doi: 10.1016/j.commatsci.2013.08.011
- Radchenko I, Anwarali H, Tippabhotla S, et al. Effects of interface shear strength during failure of semicoherent metal–metal nanolaminates: an example of accumulative roll-bonded Cu/Nb. Acta Mater. 2018;156(1):125–135. doi: 10.1016/j.actamat.2018.06.023
- Hattar K, Misra A, Dosanjh M, et al. Direct observation of crack propagation in copper–niobium multilayers. J Eng Mater Technol. 2012;134(2):021014. doi: 10.1115/1.4005953
- Zheng SJ, Wang J, Carpenter JS, et al. Plastic instability mechanisms in bimetallic nanolayered composites. Acta Mater. 2014;79(41):282–291. doi: 10.1016/j.actamat.2014.07.017
- Zhao YF, Zhang JY, Wang YQ, et al. Unusual plastic deformation behavior of nanotwinned Cu/high entropy alloy FeCoCrNi nanolaminates. Nanoscale. 2019;11(23):11340–11350. doi: 10.1039/C9NR00836E
- GRÖGER. Stress dependence of the Peierls barrier of 1/2⟨111⟩ screw dislocations in bcc metals. Acta Mater. 2013;61(17):6362–6371. doi: 10.1016/j.actamat.2013.06.047
- Xu S, Su Y. Dislocation nucleation from symmetric tilt grain boundaries in body-centered cubic vanadium. Phys Lett A. 2018;382(17):1185–1189. doi: 10.1016/j.physleta.2018.03.002
- Zheng S, Beyerlein IJ, Carpenter JS, et al. High-strength and thermally stable bulk nanolayered composites due to twin-induced interfaces. Nat Commun. 2013;4:1696. doi: 10.1038/ncomms2651
- Han WZ, Carpenter JS, Wang J, et al. Atomic-level study of twin nucleation from face-centered-cubic/body-centered-cubic interfaces in nanolamellar composites. Appl Phys Lett. 2012;100(1):011911. doi: 10.1063/1.3675447
- Jian W, Hoagland GR, et al. Room-temperature dislocation climb in metallic interfaces. Appl Phys Lett. 2009;94(13):131910. doi: 10.1063/1.3111137
- Li N, Wang J, Huang JY, et al. In situ TEM observations of room temperature dislocation climb at interfaces in nanolayered Al/Nb composites. Scripta Mater. 2010;63(4):363–366. doi: 10.1016/j.scriptamat.2010.04.005