ABSTRACT
Climate change is adversely affecting coffee production, impacting both yields and quality. Coffee production is dominated by the cultivation of Arabica and Robusta coffee, species that represent 99% of production, but both will be affected by climate change. Sustainable management practices that can enhance the resilience of production and livelihoods to climate change are urgently needed as production supports the livelihoods of over 25 million people globally, the majority of whom are smallholder farmers located in the coffee belt spanning the tropics. These communities are already experiencing the impacts of climate change. We conducted a systematic review, identifying 80 studies that describe the direct and indirect impacts of climate change on coffee agroecosystems, or that identify agroecological practices with the potential to enhance climate resilience. Adverse environmental impacts include a reduction in area suitable for production, lower yields, increased intensity and frequency of extreme climate events, and greater incidence of pests and diseases. Potential environmental solutions include altitudinal shifts, new, resilient cultivars, altering agrochemical inputs, and agroforestry. However, financial, environmental and technical constraints limit the availability of many of these approaches to farmers, particularly smallholder producers. There is therefore an urgent need to address these barriers through policy and market mechanisms, and stakeholder engagement to continue meeting the growing demand for coffee.
Introduction
Coffee is of global economic, cultural and social importance, grown in more than 60 countries by about 25 million farmers, often smallholders, and supporting the livelihoods of 120 million people (Chengappa, Devika, and Rudragouda Citation2017; Läderach et al. Citation2017; Rahn et al. Citation2014). Despite there being over 100 species of coffee, Robusta (Coffea canephora Pierre ex Froehner) and Arabica (Coffea arabica L.) species represent 99% of commercial the coffee trade (Davis et al. Citation2019). Coffee is produced in the “coffee belt” across Central and South America, Africa and Asia () (Bunn et al. Citation2015; Ovalle-Rivera et al. Citation2015; Reay Citation2019). However, production across these regions are highly vulnerable to climate change which is likely to impact yields, crop quality, and ultimately the livelihoods of farmers and their families. There is therefore a critical need to build on-farm climate resilience by increasing the capacity of coffee agroecosystems to cope with climate impacts (Shapiro-Garza et al. Citation2020). Achieving this in an equitable way will contribute to increasing livelihood resilience, namely the capacity of people to sustain and improve their livelihood opportunities and well-being despite disturbances (Tanner et al. Citation2015).
Figure 1. Global green coffee bean production (t yr−1), 2010 to 2019 by country of origin (FAOSTAT Citation2019).
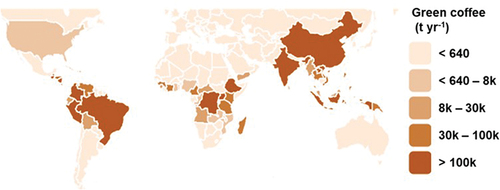
Optimal conditions for coffee growth are species-dependent: Arabica, representing 70% of coffee production and producing the highest-grades of coffee, is more climate-sensitive than Robusta, and grows best at altitudes of 1300–1500 masl (meters above sea level) (Bunn et al. Citation2015; Läderach et al. Citation2017). The optimal mean annual temperature range for Arabica is 18 to 22°C, compared with the warmer range of 22 to 26°C for Robusta, allowing easier cultivation at lower altitudes (Davis et al. Citation2012; Zullo et al. Citation2011). Temperatures beyond these may prevent blossoming and accelerated ripening, affecting flavor profiles, whilst heatwaves and frosts can cause significant yield and quality declines (Camargo Citation2010; dos Santos et al. Citation2015). Quality is typically measured by electronic sensors or coffee tasters who score samples on tasting notes (Vietoris et al. Citation2021).
Most coffee is produced in rainfed systems, and hence the intensity and seasonal variation in precipitation can affect coffee plant development. Periods of drought can limit flowering, but can be advantageous for the drying and harvesting of the fruits (Gidey et al. Citation2020). The distinction between dry and wet seasons is especially important in Arabica cultivation, as it is more sensitive to drought than Robusta (Reay Citation2019). Patterns of rainfall and temperature in existing coffee areas will shift under climate change, threatening the viability of coffee production (Camargo Citation2010; Chengappa, Devika, and Rudragouda Citation2017; IPCC Citation2018). Bunn et al. (Citation2015) predict that increasing mean annual temperatures, more variable patterns of rainfall and increased likelihood of extreme weather events will decrease the global area suitable for Arabica coffee cultivation by at least half by 2050, with East Africa and Asian island states being the only major areas where the suitable area may increase. Simultaneously, more indirect effects of climate change on coffee production, such as increased disease outbreaks and climate extremes, are already having observable impacts on coffee and ultimately those whose livelihoods are supported by the sector (Chengappa, Devika, and Rudragouda Citation2017). Nevertheless, selecting coffee cultivars resistant to such pests could enable the successful production of coffee under otherwise unfavorable environmental conditions (Davis et al. Citation2019).
Climate change is placing several stresses on coffee production systems that are driving innovative adaptation measures to mitigate these negative impacts on yield and quality (Eakin et al. Citation2014). Coffee plants take at least three years until they begin to bear fruit and, as a perennial crop, plantations typically last 30–50 years (Davis et al. Citation2012; Läderach et al. Citation2017). Field-level management decisions, therefore, require foresight and potentially more future planning than found with the cultivation of annual crops (Läderach et al. Citation2017). An increasing focus is on the potential of regenerative farming practices to provide benefits for soil health and biodiversity at field and landscape scales, ultimately benefiting climate resilience and thus the resilience of local community livelihoods. Examples of such practices include agroforestry, intercropping, improved irrigation strategies, relocating areas of production, and the development and selection of new coffee cultivars (Chengappa, Devika, and Rudragouda Citation2017; Nesper et al. Citation2019; Pappo et al. Citation2021). To date, there has been no systematic review of the effectiveness of these techniques in enhancing coffee crop climate resilience, although a previous review (Pham et al. Citation2019) has synthesized the impacts of climate change on crop production. The effectiveness of coffee production’s climate resilience depends on understanding local context, barriers to implementation and crucially, necessitates the inclusion of coffee producers in the process (Castellanos et al. Citation2013; Quiroga et al. Citation2020).
The aim of this study is therefore to identify soil, water and crop management practices that can create more climate resilient coffee production in the coming decades. Our central hypothesis is that the adoption of specific management practices can enhance the climate resilience of coffee production. Optimal resilience-building strategies will need to be multifaceted, draw on understandings of local and global changes, and utilize holistic perspectives of coffee production ecosystems.
Methodology
Using the Scopus database (www.scopus.com), we conducted a systematic review to identify and collate papers relating to field-management practices that have the potential to build resilience, and mitigate the impact of climate change on coffee production (Pickering et al. Citation2015). Systematic reviews are multi-stage processes () involving specified criteria for the inclusion and exclusion of papers (Hammersley Citation2001). Keywords were identified from the aim and objectives to create the following search strings with Boolean operators, searching in the title, abstract and keyword section of documents: “climate change” AND “adaptation” OR “mitigation” OR “management” AND “coffee.” The initial search yielded 240 papers. The documents identified from this search were collated into a database to record the processes of inclusion and exclusion. Of the total papers found in the initial search, 175 were excluded through screening. This included the removal of duplicate papers (n = 3), papers that were inaccessible (n = 10), non-English language (n = 3), publications which may not have been peer-reviewed (e.g. conference papers, n = 15), and studies identified as not being relevant based on the contents of the abstract (n = 144). An additional 15 papers identified from the reference lists of the remaining papers were also selected based on their relevance, leading to a total of 80 studies.
Figure 2. Systematic review process. *search strings: “climate change” and “adaptation” or “mitigation” or “management” and “coffee”.
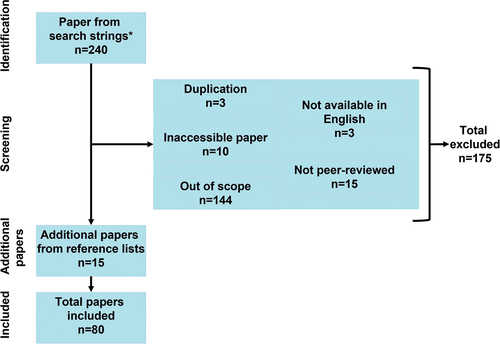
Each paper was subsequently categorized into themes, including the impacts of climate change, long-term environmental solutions (e.g. the introduction of new cultivars), soil water and crop management interventions that may be introduced in the shorter term, agroforestry, and the wider policy context, barriers to implementation, and links to livelihood resilience. These themes have been drawn from the studies themselves (Hammersley Citation2001). The approach taken to understanding coffee’s climate resilience opportunities has its limitations. Using Scopus is likely to omit the experiences of coffee producers themselves, as those authoring papers tend to be in academia or industry. The exclusion of gray literature and non-English language papers in the review represents a further omission of knowledge that has the potential to inform this study and discussion. Our approach, in particular the selection of keywords, also potentially excludes some of the wider body of research from anthropological, sociological, and human geographical research that specifically seeks to interview coffee farmers and incorporates their perspectives and may have captured additional practices. While this is not the primary focus of the review, such knowledge, particularly in the context of the accessibility of approaches and opportunities for shared learning, has been incorporated in the review when identified (see Barriers to implementation and Access to Knowledge Networks).However, there is a potential gap in understanding of those facing the effects of climate change and their direct experience of adapting coffee production. In addition, there our selected keywords and approach may also have excluded some of the literature regarding opportunities for the diversification of incomes and livelihoods, or the interplay between changes in coffee production and the food security of farmers.
Coffee research trends
Recent research trends have predominantly focussed on the adoption of agroforestry (24 studies) and the accessibility of different farming practices, predominantly for smallholder farmers (25 studies; ). The majority of studies have been undertaken in the Americas (40 studies), and to a lesser extend in Africa (12 studies; ). This analysis indicates significant knowledge gaps around specific crop, soil and water management practices in many regions, and few studies in general have been conducted in Asia, despite Indonesia and India being amongst the ten most significant coffee producers (FAOSTAT Citation2019). Moreover, most research to date has focussed on Arabica production, with only one identified study that explicitly applied to Robusta, four which covered both varieties, and 17 studies which did not specify a variety ().
Climate change impacts on coffee production
Temperature and drought responses of coffee
Climate change, particularly changes in precipitation and temperature will substantially affect coffee production by directly impacting coffee crops, and through indirect effects (for example pollinator abundance and pest and disease outbreaks; ). The effects of drought on coffee plants are wide-ranging and include reduced production of new leaves, chlorosis, reduced stem elongation, a reduction in the number of fruiting nodes per tree, fewer fruits per note, and reduced seed size, and reduced bean quality (Carr Citation2000). Although periods of water stress are necessary for the development of flower buds, consistent temperatures above the optimal ranges of 18 to 22°C for Arabica, and 22 to 26°C for Robusta can reduce crop growth and cause damage to the crop physiology, particularly during flower bud development (Carr Citation2001; Zullo et al. Citation2011). However, vulnerability to extreme temperatures and droughts differs between cultivars bred for specific characteristics, such as deeper-rooting systems that increase access to scarce water resources and earlier stomatal closure (DaMatta and Ramalho Citation2006). There are also more indirect effects of extreme temperatures and drought, such as reduced pollinator populations that can contribute to yield declines (Carr Citation2001). Exposure to lower temperatures, in a process known as chilling, is especially damaging to coffee crops, affecting Robusta more than Arabica varieties (Reay Citation2019). Such chilling can damage the chloroplasts in coffee plant cells and in turn reduce rates of photosynthesis which are reflected in lower yields and quality of the coffee crop (DaMatta and Ramalho Citation2006). Consequently, coffee production is reliant on a stable climate envelope to maintain yields and thus farmer livelihoods (Carr Citation2000).
Regional climate change impacts
Global climate patterns are changing rapidly due to anthropogenic greenhouse gas emissions. Within the next two decades, global mean surface temperatures are anticipated to reach at least 1.5°C above pre-industrial levels (IPCC Citation2021). The consequences of this are severe and diverse: from more frequent extreme weather events, increases in drought, to changes in precipitation patterns and El Niño events, all of which affect agriculture (IPCC Citation2021). The effect of local landscapes and climatic zones mean that global patterns of climate change manifest differently between and within regions (Abebe Citation2020) (), particularly as the tropics are expected to experience the most extreme warming in the short term (IPCC Citation2018).
Where temperatures are already high, increasing mean temperatures can be problematic for the development of the coffee fruit (Camargo Citation2010). Craparo et al. (Citation2015) note that increasing mean temperature has a greater influence on coffee crop yields than other climatic variables; whilst precipitation change is the main driver of coffee land suitability changes (Bunn et al. Citation2015; Läderach et al. Citation2011). High temperatures can be especially damaging during the sensitive development stage of flowering (Gidey et al. Citation2020). Unshaded production systems are especially vulnerable to higher temperatures which may cause faster plant growth and over-ripening of the fruit, lowering bean quality (Camargo Citation2010). Changes to patterns and intensity of rainfall will also affect coffee crop development which is dependent on distinctive seasons for its reproductive stages (Bunn et al. Citation2015; Camargo Citation2010). Ultimately, Gidey et al. (Citation2020) estimate that climate change could lead to a 4–25% decrease in coffee yields under agroforestry and a 20–60% decrease in full-sun systems by 2060, with the range depending on the climate scenario considered.
Areas suitable for coffee cultivation are predicted to decline significantly in the coming decades, particularly for the higher-value Arabica (Pham et al. Citation2019). This land suitability decline is expected to be diverse across regions (), although some places, especially upland regions, may become more suitable than at present. Importantly, these data are based on changes in total land suitable for coffee cultivation, rather than land currently used, so may not completely reflect future impacts on production. Increases in atmospheric carbon dioxide (CO2) concentrations can increase rates of photosynthesis and improve certain coffee species’ heat tolerance which may also influence future areas suitable for cultivation (DaMatta et al. Citation2019). Critically, Ovalle-Rivera et al. (Citation2015) identified no major countries currently producing coffee with overall increases in land suitability.
Table 1. Predicted decrease in land suitable for Arabica cultivation (%) for selection of leading coffee producers. Area harvested from FAOSTAT (Citation2019).
Indirect effects
Climate change can have indirect effects on coffee production, in particular the incidence of pests and diseases (Gidey et al. Citation2020). Jaramillo et al. (Citation2009) proposed that for each 1°C increase in local temperature, the rate of coffee berry borer (Hypothenemus hampei) population growth increases by 8.5%, particularly under drought-stress. The increasing damage and geographical spread of berry borer into higher altitudes have already been reported in East Africa, alongside temperature increases there (Jaramillo et al. Citation2011). Coffee leaf rust (CLR) (Hemileia vastatrix) is an increasingly problematic fungal disease for coffee producers, particularly as it spreads into areas previously too cold for it to survive (Avelino et al. Citation2015; Davis et al. Citation2019). However, other studies have suggested that while fungal pathogen life cycles are strongly determined by weather (particularly temperature and water availability), many CLR outbreaks (for example the 2008–2011 outbreak in Colombia) may not have been driven by climate change, given a lack of a long-term trend between 1990 and 2015 (Bebber, Castillo, and Gurr Citation2016). Beyond environmental drivers, CLR outbreaks are likely exacerbated by changes in the profitability of coffee farming, with price declines or high fertilizer costs reducing the ability of farmers to invest in the necessary management across the industry (Rhiney et al. Citation2021).
Numbers of pollinating insects essential for coffee reproduction such as bees are anticipated to decline by 8–18% under projected climate change scenarios (Imbach et al. Citation2017). Global climate change patterns are thus increasing the vulnerability of coffee production through several pathways. Building resilience into these systems is, therefore, paramount to mitigating the worst impacts and enhancing ecosystem capacity to absorb climate shocks (Gallopín Citation2006; IPCC Citation2018).
Adapting coffee production to climate change in the long-term
Cultivar selection
Selecting new, different or a wider mix of coffee cultivars may improve the resilience of coffee production to climate change in the longer term, but requires long-term consideration of market value, climatic suitability, and management practices for optimal yields. The current dependency on two main species of coffee with limited genetic diversity makes the global coffee production system vulnerable to climate change (Bunn et al. Citation2015). Robusta coffee is a lower-grade coffee than Arabica, but more tolerant of higher temperatures and less prone to diseases such as CLR which is increasingly common in Arabica production in Central and South America (Chengappa, Devika, and Rudragouda Citation2017; Davis et al. Citation2019). Robusta yields can be double those of Arabica under the same conditions, and since Robusta beans are commonly sold without pulping, less labor is required in the post-harvest stages of production (Ruiz Meza Citation2015). However, Robusta is often cultivated in unshaded systems due to its heat tolerance, making these systems more exposed to landslips, soil erosion, extreme weather events and loss of ecosystem services (DaMatta et al. Citation2007; Ruiz Meza Citation2015).
Recent data from Kath et al. (Citation2020) suggests Robusta has a lower optimal temperature range than previously thought, with every 1°C increase in the mean maximum temperature above about 24°C associated with a yield reduction of ~ 14% (350–460 kg ha−1). Hence, there will be limits to the resilience of yields to increased temperatures when transitioning from Arabica to Robusta production. Pappo et al. (Citation2021) suggest that sustainable coffee production requires the identification and selection of new cultivars. Catimor is a high-yield CLR-resistant Arabica cultivar developed in the 1950s that is popular in Central and South America (Bro et al. Citation2020). Similarly, the Castillo variety has increased CLR resistance. Collectively CLR resistant varieties now account for 6% of total coffee producing areas in Colombia (Avelino et al. Citation2015). Newer hybrid varieties are increasingly being developed with increased CLR resistance, flavor and ability to grow under more diverse environments (e.g. lower altitudes and under canopy shading). However, such hybrids can be expensive for smallholder producers (Rhiney et al. Citation2021) as they need to be produced using in vitro or vegetative propagation.
Due to the limitations imposed by genetics and breeding for Arabica and Robusta, there is growing interest in the potential for wild species of coffee, of which over 100 species have been identified. This species pool likely contains beneficial characteristics such as disease resistance and heat tolerance (Davis et al. Citation2019). It is critical to maintain the genetic diversity within these coffee wild relatives to allow adaption to changing threats to future production, like resistant pests and disease (Bro et al. Citation2020). Coffea stenophylla is an example of a wild coffee species originating from West Africa with potential to create more climate resilient coffee production as it grows in annual temperatures 6.2–6.8°C hotter on average than Arabica (Davis et al. Citation2021). Significantly, the flavor profile of stenophylla has greater similarity to Arabica than Robusta and therefore, if its historically low commercial yields can be overcome, could provide greater financial security to coffee farmers (Davis et al. Citation2021).
The extinction of wild coffee species presents a significant future challenge. Monoculture coffee production, in addition to deforestation and fragmentation of biodiverse landscapes, increasingly threaten wild coffee populations that contain the genes with potential to provide climate resilience (Aerts et al. Citation2017). Davis et al. (Citation2019) quantify this threat. Given the rate of emerging climatic threats to coffee production; identifying species with the potential to provide secure yields of quality coffee alongside practices that encourage on-farm biodiversity are paramount (Bro et al. Citation2020; Watts Citation2016). At present, changing dominant cultivars takes several years and the viability of these also depends on consumer’s acceptance and desire for such change.
(: IUCN categorization of extinction risk to known coffee species. Critically endangered (CE), endangered I, vulnerable (V), near-threatened (NT), least concern (LC), data not available (DA). Data from (Davis et al. Citation2019), using extinction risk data from the International Union for Conservation of Nature (IUCN).
Figure 5. IUCN categorization of extinction risk to known coffee species. Critically endangered (CE), endangered (E), vulnerable (V), near-threatened (NT), least concern (LC), data not available (DA). Data from (Davis et al. Citation2019).
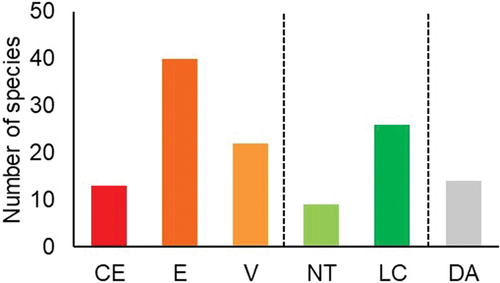
Seed banks can improve farmers’ access to different varieties, which may build climate and livelihood resilience (Shapiro-Garza et al. Citation2020). Collective seed banks that involve smallholders and operate at a scale larger than independent seed stores, can potentially encourage the identification and preservation of more resilient varieties (Li and Pritchard Citation2009). However, there are challenges in terms of the social impacts and financial capital required to operate seed banks effectively (Shapiro-Garza et al. Citation2020). There is also a need to better understand which cultivars may thrive under future environmental conditions, as well as optimizing production under current climates (Pappo et al. Citation2021). This kind of selection for varieties based on future climate change represents a different approach to that identified by Eakin et al. (Citation2014), who found that coffee grow’rs’ motivation to adopt different varieties is based on maximizing present productivity, yields and disease resistance.
Altitudinal changes
The relocation of coffee production to higher altitudes, to address the increases in mean temperatures, has been proposed as a resilience-building strategy, although will require long-term planning (Craparo et al. Citation2015; Moat et al. Citation2017; Pham et al. Citation2019). Land suitability is expected to shift upwards in the coming years (). In Tanzania, for example, the optimal area for coffee production is estimated to shift upwards by 150–200 masl to sustain viable coffee yields and quality (Craparo et al. Citation2015). Coffee bean quality is thought to benefit from cooler found at air higher elevations, as a slower rate of bean-filling during fruit development is associated with flavorful, acidic coffee (Chemura et al. Citation2016). The aforementioned effect of higher atmospheric CO2 concentrations that can increase rates of photosynthesis increase and thus, yields may also be enhanced when higher temperatures are moderated at higher elevations (DaMatta et al. Citation2019). Understanding the impact of higher altitudes and increased atmospheric CO2 on viable coffee yields under different climate change scenarios remains an important area of research.
Table 2. Predicted altitudinal changes in land suitability for growing Arabica for a selection of leading coffee producing countries.
However, moving production to higher altitudes will be constrained by the availability of land with suitable soil types, water retention, and slope gradients (Craparo et al. Citation2015; Reay Citation2019). For example, soil depths tend to be shallower at higher elevations than in lowland areas, whilst greater precipitation and steeper slopes typically can also increase the loss of nutrients through leaching and runoff (Chemura et al. Citation2016). In Tanzania, Craparo et al. (Citation2015) noted that higher altitudes often contain protected forests and are sources of rich biodiversity. Deforestation to clear land for coffee production thus has inevitable negative impacts on local biodiversity and CO2 emissions (Ovalle-Rivera et al. Citation2015). Schroth et al. (Citation2015) therefore suggest that the movement of production should be limited to already cleared locations where coffee production can provide a positive impact on the local ecology.
There are also cost implications of establishing new coffee production systems (which can take 5–8 years from planting to first harvest), which can be prohibitive for smallholder farmers (Bunn et al. Citation2015; Davis et al. Citation2012). The remoteness of higher-altitude areas can also make it difficult and costly to secure the labor needed to manage the coffee crop (Schroth et al. Citation2015). In areas where shifting altitude is not a viable option and land is predicted to be unsuitable for coffee production, alternative crop, water and soil management strategies should be considered (Läderach et al. Citation2011).
Adapting coffee production to climate change in the short-term
Managing soil and water resources
Maintaining soil health is important for sustainable coffee production. However, extreme weather events, nutrient leaching and excessive use of agrochemicals can adversely affect soil health (Reay Citation2019). Soil erosion can be reduced through terracing and by planting crops, such as Pennisetum purpureum (elephant grass) that can enhance soil structure (Abebe Citation2020). In some steep areas, collaborative soil conservation practices between coffee producers may be important to minimize landslides (Eakin et al. Citation2014).
Similarly, water availability is essential for sustaining harvests of high-quality coffee. Under climate change, irregular precipitation may mean that increased numbers of coffee smallholders will require secure means of accessing water to improve the climate resilience of production systems (Quiroga et al. Citation2020). Practices that improve water security include those that capture and store precipitation in-field, and those that enable access to alternative supplementary sources of water, like borewells, and the use of irrigation (Baca et al. Citation2014; Chengappa, Devika, and Rudragouda Citation2017). Developing farm-based water supplies and irrigation can enable producers to have greater control of harvesting patterns, whilst also reducing strain on domestic water supplies (Shapiro-Garza et al. Citation2020).
Water conservation can be enhanced by increasing the organic matter (OM) content of soils (Camargo Citation2010). Applying OM mulches to soil can also reduce soil evaporation and suppress weeds that can reduce the need for agrochemicals (Bro et al. Citation2019; Reay Citation2019). Additional benefits of scale can accrue when carried out through networks or cooperatives of smallholder farmers, for example collective compositing in Chiapas, a major coffee producing region in Mexico (Schepp Citation2009), Steeper topographies at higher altitudes can be challenging for implementing water-conserving tools (Shapiro-Garza et al. Citation2020). This is exemplified in the mountainous coffee-producing regions of Southern Mexico which are often too steep for irrigation to be a viable option (Lin Citation2009). In this case, shade trees can be used to conserve water by limiting evapotranspiration (Lin Citation2009). Financial capital is essential for implementing and maintaining water conservation practices and thus represents a major potential challenge preventing rapid adoption (see Barriers to Implementation for a more detailed discussion) (Shapiro-Garza et al. Citation2020). Additional crop water requirements under future higher temperatures may also increase the cost of these systems over time, an important consideration when evaluating their sustainability (Chemura et al. Citation2016).
Coffee crop management
Improving management of the coffee crop itself may mitigate declines in yields and quality under climate change. For example, adjusting the planting date for new coffee crops in South-West Ethiopia, in response to better local access to meteorological data, is an effective practice in mitigating the effects of a delayed wet season (Eshetu et al. Citation2021). Pruning of dead branches to reduce the number of stems per coffee plant can also encourage crop growth (Sarmiento-Soler et al. Citation2020). Dufour, Kerana, and Ribeyre (Citation2019) study in Sumatra found pruning coffee trees did not change yields significantly in the first year but produced over twice the number of ripe berries per tree than unpruned plants in the subsequent year. Effective management of the crop in the post-harvest stages through drying can also build climate resilience. Unlike the more traditional method of drying harvested beans through exposed air-drying, which can expose beans to unpredictable weather conditions and animals; solar dryers that are physical structures which protect the beans and provide more sheltered drying conditions are currently being used in Guatemala and Peru (Shapiro-Garza et al. Citation2020).
One option to mitigate increases in temperature and outbreaks of pests and disease under climate change on the income of coffee producers is to select non-coffee crops to substitute or supplement coffee production (Baca et al. Citation2014). For example, in response to climate change, some coffee farmers in Ethiopia have started to grow khat (Catha edulis (Vahl) Forssk. ex Endl.): a disease and drought resistant crop; although in other countries it production is illegal (Abebe Citation2020). Similarly, the introduction of trees with harvestable fruit, such as bananas and avocado, into coffee systems in Mexico has been found to mitigate climate-induced yield losses and provide additional economic benefits (Ruiz Meza Citation2015). However, whilst these methods support producers, it does not provide a sustainable solution for production and consumers of coffee.
Altering agrochemical inputs
One of the challenges posed by changing climatic conditions is the effect on the incidence of pests and diseases that affect coffee production. Recent outbreaks of CLR, for example, have caused large economic losses in Central and South America, particularly in the period 2008–2013, with such outbreaks anticipated to become more frequent and widespread (Avelino et al. Citation2015; Shapiro-Garza et al. Citation2020).
Effective monitoring of outbreaks is one pathway to building greater resilience so pests can be identified at the source and controlled before they cause wider damage (FAO Citation2015). Implementing strategies of identification and reporting beyond the field level, such as through cooperatives, can generate resilience to these outbreaks but they require social and human capital to be successful. In participatory research with smallholder producers in Central America, Shapiro-Garza et al. (Citation2020) found that knowledge regarding pest identification and best practices for integrated pest management was uneven and presented a barrier to building resilience.
Beyond monitoring, agrochemical applications are often used to manage pest and disease, although there are negative ecological implications from their use (FAO Citation2015). For example, Endosulfan is a commonly used insecticide in coffee production but can be toxic to those applying it in the field and also further down the food chain as it can accumulate in coffee beans (Reay Citation2019). There have been successful approaches to mitigating such pest attacks without the use of hazardous agrochemicals (FAO Citation2015). Coffee cooperatives in Chiapas, Mexico have applied Beauvaria bassiana, a fungus that attacks pests such as coffee borer, as a biological control method (Schepp Citation2009). Physical methods of control in Central American include improving the hygiene of the coffee crops themselves, such as manually removing damaged fruits, and targeting pests like coffee borers with alcohol-based traps (Reay Citation2019). Taking these approaches to limit the use of agrochemicals in coffee agroforestry production can also enhance bacterial and fungal activity in the soil and thereby increase resilience of the soil system to climate variability (Garzón, Rivas, and Avellaneda-Torres Citation2020).
In a survey of coffee farmers in Central America, in response to climatic changes, about 17% stated they were applying more fungicides and herbicides, and about 15% stated that had increased the use of fertilizers (Harvey et al. Citation2018). A similar trend was observed in Mexico following continuous outbreaks of CLR where farmers introduced CLR-resistant coffee cultivars and applied more agrochemicals than before (Valencia et al. Citation2018). Commonly used nitrogen-based fertilizers can be a significant source of nitrous oxide, a potent greenhouse gas that contributes to driving climate change (Girkin and Cooper Citation2022; Salamanca-Jimenez, Doane, and Horwath Citation2017). Reducing fertilizer inputs and moving to organic systems can provide more sustainable management for coffee production systems which may involve aligning plants’ needs for fertilizer with inputs. Organic coffee farmers therefore rely on the addition of substantial quantities of compost, the availability of which may be lower than agronomic demand (Van Der Vossen Citation2005), the relative balance of greenhouse gas emissions from these organic versus inorganic systems is highly variable (Noponen et al. Citation2012). For example, the carbon footprints for 1 kg of fresh coffee has been reported to be between 0.26 and 0.67 kg CO2e for inorganic production, compared to 0.12 and 0.52 kg CO2e for organic systems, largely depending on the choice of emissions factor used for emissions from pruning inputs. These contributed between 7 and 42% of the total footprint of organic systems. An overall lack of quantification of the potential benefits and trade-offs, and availability of the required resources limits the potential adoption of such approaches by smallholder farmers.
Climate adaptation and mitigation potential of coffee agroforestry
Benefits for production and ecosystem services
Agroforestry involves the incorporation of trees alongside coffee crops to deliver ecological and economic benefits (). Reported ecological benefits include improved soil health, nutrient cycling, ecosystem biodiversity and carbon storage (Garedew et al. Citation2017; Gomes et al. Citation2020). Growing coffee crops under tree canopies creates multi-strata systems that can be further developed through the incorporation of companion crops at different heights (Tschora and Cherubini Citation2020). The shade provided by the trees can protect the coffee crop from temperature extremes (Camargo Citation2010; Gidey et al. Citation2020; Läderach et al. Citation2017). Agroforestry’s economic benefits can include improved economic stability from selling additional crops’ produce (Chengappa, Devika, and Rudragouda Citation2017). Desirable traits for agroforestry species selection include compatibility with coffee, fruit and biomass production, and minimal labor requirements (Souza et al. Citation2010).
Figure 6. Potential integrated ecological and economic benefits from agroforestry for coffee production including increased (a) above and below ground species biodiversity; (b) temperature stabilization; (c); wind speed reduction; (d) carbon storage; (e) diversified income from non-timber products(.
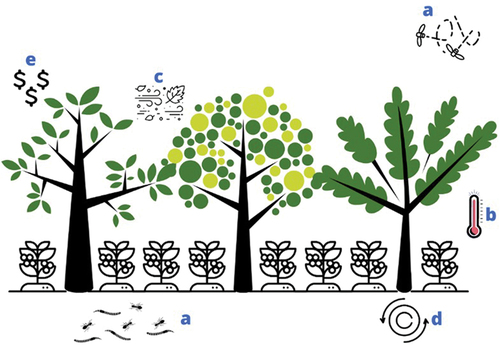
In the wild, coffee grows naturally as an understory crop, and producing coffee under shade trees is a traditional method of cultivating coffee (Bro et al. Citation2020). Nevertheless, since the 1900s, with the coffee demand growing and the global intensification of agriculture, there has been a shift toward monocultural coffee production characterized by shade trees removal, high coffee crop densities and increased agrochemical inputs (Gidey et al. Citation2020; Perfecto, Jiménez-Soto, and Vandermeer Citation2019). For example, Central American coffee farms are estimated to have lost more than half of their tree cover in the latter half of the twentieth century (Rice Citation1999). Globally, just 24% of coffee is produced under diverse shade systems (Jha et al. Citation2014). Importantly, the impact of agroforestry on ecosystem ecology is variable and often depends on the diversity of shade trees planted (Perfecto and Vandermeer Citation2015).
The shade provided by agroforestry trees creates some protection to coffee crops under anticipated increases in mean temperatures (de Souza et al. Citation2012; IPCC Citation2021; Schroth et al. Citation2009). Simultaneously, coffee’s vulnerability to low nighttime temperatures and frost is reduced through the sheltered microclimate (Gidey et al. Citation2020). For example, in a Brazilian study, shade trees increased coffee leaf minimum temperatures by 2–4°C compared to full-sun systems (Caramori, Androcioli Filho, and Leal Citation1996). Likewise, this microclimate reduces temperature fluctuations by approximately 2–3°C, creating a more stable growing environment (Camargo Citation2010). Multiple levels of vegetation and a more stable soil structure under agroforestry than sun-exposed coffee systems can also mitigate the effects of extreme weather events such as hurricanes, floods and landslides that pose high-risk threats to coffee production (Schroth et al. Citation2009). Wind speed can be reduced by 22–99% by the presence of trees: compared to full sun systems, limiting damage to coffee fruits and flowers (Coltri et al. Citation2019; DaMatta et al. Citation2007). Nonetheless, there is a threshold for the effectiveness of trees; in extreme weather events such as Hurricane Maria in Puerto Rico, Perfecto et al. (Citation2019) found that this wind protection effect was overridden by the strength of the hurricane.
Shade trees also increase humidity levels around coffee crops. Rates of evapotranspiration from the coffee crops and soil have been reduced by 38% in high-shade systems compared with lower-shade systems, in the Soconusco region of Chiapas, Mexico (Lin Citation2007). This is important in the context of coffee production as high levels of soil moisture are critical during the flowering stages of the plant’s development (Lin Citation2010). It is also pertinent to consider the additional water demand associated with planting and maintaining shade trees. Burgess et al. (Citation1998) report that shade trees can redistribute water from deeper within the soil profile into the upper horizons via hydraulic lift. Hence, Lin (Citation2007) argues that shade trees do not compromise coffee plants’ capacity to access soil water and decrease coffee’s water loss from evapotranspiration.
The incorporation of shade trees can improve soil structure (Tschora and Cherubini Citation2020). For example, Aguiar (Citation2008) found that after more than a decade of growing coffee alongside trees in South-Eastern Brazil, the soil had greater water retention and a lower bulk density, leading to an overall improvement in the soil quality and nutrient availability to plants. Litterfall can protect the soil surface from run-off during rainfall and replenish soil carbon and nitrogen stocks (Perfecto and Vandermeer Citation2015; Wagner et al. Citation2021). Such enhancements of nutrient cycling loops through agroforestry embed principles of regenerative agriculture into coffee production systems (Elevitch, Mazaroli, and Ragone Citation2018). Shade trees further influence the carbon stocks of the area of coffee production, both in terms of soil organic carbon (SOC) and above-ground biomass, and thus offer the potential of coffee farming systems as being part of a wider system of on-farm nature based solutions for climate change mitigation (Häger Citation2012; Noponen et al. Citation2012; Rahn et al. Citation2014). Zaro et al. (Citation2020) study of coffee production in Brazil found that agroforestry stores 47 Mg C ha−1 more in the biomass and soil (0–70 cm depth) than open coffee systems. Likewise, rates of carbon fixation in plant biomass were found to increase by 5.5–13.9 Mg C ha−1 yr−1 when changing from low to medium and high shade agroforestry in a study of three coffee production systems in Colombia (Andrade and Zapata Citation2019). The potential benefits are dependent on the local conditions and management practices. For example, Noponen et al. (Citation2013) reported no change to the soil organic carbon stocks between shaded and unshaded systems over nine years, whilst in a Costa Rica site, above-ground carbon stocks increased by 3.3–12.9 Mg C ha−1 yr−1 due to biomass production under agroforestry. Aggregate carbon stocks, including above and below-ground biomass can result in agroforestry coffee systems being a net carbon sink (Betemariyam, Negash, and Worku Citation2020). Alongside the ecological benefits, agroforestry has the additional ecosystem service of carbon sequestration, with the potential to reduce further climate change (Rahn et al. Citation2014; Soto-Pinto et al. Citation2010).
Above and below-ground biodiversity is encouraged in the presence of shade trees, particularly when native tree species are planted, in contrast to the common practice of planting exotic species like Grevillea Robusta (Devagiri et al. Citation2020). The resilience of ecosystems and provision of essential ecosystem services can be enhanced through greater biodiversity, for example, reduced pest outbreaks and vulnerability to climate shocks (Perfecto and Vandermeer Citation2015; Rice Citation1999). For example, the incidence of coffee berry borer was 6.8% in open-sun production, compared with 0.6% under shade in Kenya (Jaramillo et al. Citation2013). Shade trees can also provide pollination services that have great economic value to coffee producers (Beer et al. Citation1998; Chain-Guadarrama et al. Citation2019). Bees, amongst other pollinators, are both essential to the production of coffee beans and capable of increasing yields (Boreux et al. Citation2013). Since areas of coffee production overlap with some of the most biodiverse areas in the world, by conserving diversity through shade coffee systems, forest areas can be connected and used to maintain the movement of migratory birds and other essential pollinators (Imbach et al. Citation2017; Jha et al. Citation2014).
While shade trees provide varied benefits to a range of ecosystem services, the impacts on yield are debated, which therefore remains a key challenge (alongside the availability of finance) for adoption (Tschora and Cherubini Citation2020). For example, comparing shade and sun systems in Kenya, coffee grown under shade had a greater berry density per branch, 10.8% more than their sun-grown equivalents (Jaramillo et al. Citation2013). Moreira et al. (Citation2018) proposed that increases in coffee productivity under palm shade in Brazil was driven by increased soil moisture at a depth of 20–40 cm. Conversely, Lin’s (Citation2009) study in Mexico found little difference in the yield and size of the coffee berries produced under high and low shade systems. Finding the optimal spacing and shade tree species to mitigate the effects of climate change whilst maintaining viable yields will be dependent on local conditions and management (Bro et al. Citation2020). For example, Sarmiento-Soler et al. (Citation2020) found in Uganda that when shade cover was greater than 30%, the yield was reduced. In Gidey et al. (Citation2020) future coffee yield simulations, it was predicted that coffee yields will decline in both shade and monoculture systems, but critically the overall vulnerability of yields to climate change under agroforestry were reduced. DaMatta (Citation2004) also reports that the typical biennial fluctuations in yields experienced in unshaded systems can be replaced with more consistent annual yields under shade.
Shade trees can also impact coffee bean quality, with higher quality reported under shade trees (Bro et al. Citation2020), which can potentially attract a price premium for farmers. The drivers of this were investigated in the lower-altitude coffee-producing areas of Costa Rica where Muschler (Citation2001) found that shade encouraged slower berry ripening resulting in higher-quality, larger, and more uniform beans. Importantly, this study focussed on the coffee production scenarios that were under stress, and therefore, the effect on higher-altitude crops may differ. Ultimately, to have a successful system in which agroforestry benefits coffee production under increasing climatic stresses requires consideration of local conditions, tree selection, spacing, and crop management (DaMatta Citation2004).
Challenges and trade-offs
There are several challenges and trade-offs from more widespread implementation of agroforestry in coffee. Nesper et al. (Citation2019) highlight that the observable trend of creating shade canopies with one species of non-native tree termed “monospecific shade” systems, limits the ecological benefits and climate resilience of agroforestry (Armbrecht, Perfecto, and Vandermeer Citation2004). Limited diversity in the tree species, can reduce nutrient cycling in the soil and overall biodiversity compared to more diverse agroforestry systems (Bro et al. Citation2020; Canal-Daza and Andrade-Castañeda Citation2019).
Planting density and the spacing of trees in these systems is also important to maximize both yields and agroforestry benefits. It was found that coffee planted 4.2 m away from palm trees produced 0.2 kg per plant (70% increase) more than those 1.4 m away (Moreira et al. Citation2018). Likewise, the coffee species’ sensitivity to temperature ranges will also affect the spacing of the trees and the amount of shade required (Craparo et al. Citation2015). Evidently, the optimal spacing will vary, dependent on the selected tree and coffee species, field conditions and local climate.
Souza et al. (Citation2010) collated a list through a participatory methodology with coffee farmers in Brazil to establish species most suitable to coffee agroforestry. By accounting for the economic and ecological value that specific species can bring to coffee production systems, the list highlights the criteria for selection that were most important to farmers. Examples include Musa paradisiaca and Inga spp. which both have relatively high rates of food and biomass production whilst being compatible with coffee (Souza et al. Citation2010). The main criteria were compatibility with coffee production (e.g., rooting depth), labor requirements, biomass production and diversity of products from the trees (Souza et al. Citation2010). The authors emphasize the importance of selection for the success of coffee agroforestry systems and the necessity for farmers to have access to knowledge regarding different tree species and their effect on the ecosystem. Similarly, Dumont et al. (Citation2019) collected a list of 20 tree species from coffee smallholders in Rwanda based on a number of attributes, such as ease of management, rooting depth and timber strength, such as Ficus thonningii. Another consideration is the potential value of harvestable timber or fruit from the trees that can supplement farmer’s income, and potentially offset the cost of implementation (Bro et al. Citation2020). Potential sources of secondary income from the shade trees foreground the interrelation between two climate-adaptation strategies for resilience: income diversification and agroforestry (Nesper et al. Citation2019).
However, just as coffee crops are affected by the new environmental conditions under climate change, so are trees planted for agroforestry (de Sousa et al. Citation2019). Habitat suitability in Mesoamerica for popular nitrogen-fixing trees like Erythrina poeppigiana and members of the Inga species will decline significantly by 2050, particularly in the low-mid altitude areas. Some trees will benefit from the changing environmental conditions, including the fruit tree Brosimum alicastrum and timber trees such as Simarouba glauca (de Sousa et al. Citation2019). Therefore, agroforestry management needs to consider the effect of climate change on both the coffee crop and tree species in order to successfully mitigate the impacts of climate change.
There is an additional challenge posed by the potential competition for nutrients, light and water between the trees and coffee crops. Zaro et al. (Citation2020) highlight that competition for such resources is greatest between crops and trees planted close together, but overall, the impacts of competition can be outweighed by the benefits on yield from increased shade. Similarly, the management of the trees, through practices such as pruning and thoughtful species selection can limit such competition (Gomes et al. Citation2020). Importantly, although greater biodiversity can suppress the incidence of pests, more humid, sheltered conditions under shade can also provide some pests with optimal environments, such as white stem borer, especially at lower altitudes (Chengappa, Devika, and Rudragouda Citation2017).
Challenges, policies and accessibility for farmers
Barriers to implementation
Developing coffee management practices to improve climate resilience involves highly dynamic and multifaceted decision-making processes for farmers (Eakin et al. Citation2014; Hochachka Citation2021). The barriers to improving the resilience of the livelihoods of coffee farmers to climate change are evident in Harvey et al. (Citation2018) research who found that less than half of the interviewed Central American farmers were changing management practices despite witnessing climate change impacts. The adoption of certain practices (such as relocating production to higher elevations) can require significant financial capital, as well as technical and social resources which can be prohibitive for some farmers (Baca et al. Citation2014). For example, integrated pest management can be most successful through organized and accessible collectives that source data and encourage producers to document change (Shapiro-Garza et al. Citation2020).
A key barrier to implementation is often underlying inequalities, exacerbated by climate change, that limit farmers’ access to sufficient resources to change management practices (Watts Citation2016). Quiroga et al. (Citation2020) reports that perceptions of climate risk and capacity to adapt are significantly lower in populations living below poverty lines. Such socio-economic factors influence present climate change vulnerability, how that risk is perceived, and the capacity of farmers to adapt to embed resilience (Chengappa, Devika, and Rudragouda Citation2017; Nguyen and Drakou Citation2021). The effect of climate change on the conditions of labor may also present an additional barrier to implementing resilience-building strategies. As a labor-intensive crop, during its management and harvest, those working in production are vulnerable to extreme heat conditions (Kjellstrom, Holmer, and Lemke Citation2009).
Farmer decision making and adaptive capacity in responding to the direct and indirect impacts of climate change does not occur in isolation but rather in the wider context of their individual and community livelihoods, and wider society. Such factors are wide-ranging and can include incomes, holding sizes, stability of land tenure, and connectivity of communities, education, and access to knowledge (Fain et al. Citation2018; Hirons et al. Citation2018; Jezeer et al. Citation2019)
Access to knowledge networks
Many studies highlight how access to farming cooperatives and information about adaptation opportunities can be central to driving sustainable change in adapting management (Abebe Citation2020; Barrucand, Giraldo Vieira, and Canziani Citation2017; Quiroga, Suárez, and Solís Citation2015). Importantly, knowledge networks that are most beneficial for encouraging effective adaptation to climate change are those that are grounded in local knowledge and smallholders’ experiences of change. Jacobi et al. (Citation2017) suggest that promoting conversations between farmers, as well as local and regional collectives alongside those in policy-making positions provide a strong starting point. Alpízar, Carlsson, and Naranjo (Citation2011) found that in Costa Rica, collective dialogue between coffee farmers was pivotal in producing more coordinated responses to change and avoiding high-risk adaptation strategies. Such collective networks facilitate more sustained climate resilience strategies over time (Baca et al. Citation2014; Barrucand, Giraldo Vieira, and Canziani Citation2017; Shapiro-Garza et al. Citation2020). Membership of cooperatives and collective organizations has also been found to be a means of driving higher rates of implementation of sustainable farming practices (Bro et al. Citation2019). For example, Shinbrot et al. (Citation2019) found that in Mexico, membership of such groups provided social capital that increased the likelihood of smallholders adopting a range of practices to mitigate climate change. Cooperatives provide knowledge and training for adaptation opportunities and provide important social capital that supports the process of building climate resilience (Bro et al. Citation2020).
In addition to knowledge about adaptation, Abebe (Citation2020) and Eshetu et al. (Citation2021) highlight the need for accessible and usable climate and meteorological data for farmers. Access to information about climate-related threats to water sources has also been noted as a key source of data for coffee smallholders to use in adaptation decision-making (Quiroga, Suárez, and Solís Citation2015). An example of resilience-building decision support based on data is (Van Der Wolf et al. Citation2019) tool that inputs local climate data and farmers preferences to generate agroforestry tree species recommendations.
Developing integrated coffee climate resilience strategies
Building climate resilience into coffee production requires multiscale action, from the field to national and international policy; described by Ramirez-Villegas et al. (Citation2012) as “traversal measures” enabling widespread change. Practices that drive sustainability in coffee production (e.g. improved soil management combined with diversification) often need to be incentivized to overcome identified financial barriers to implementation. One option is applying financial values to outcomes of adapted practices, such as payment for ecosystem services schemes where producers are rewarded for measurable ecological benefits, like biodiversity (Fain et al. Citation2018). Addressing underlying economic inequalities through low-interest agricultural loans or subsidies can make adaptation more accessible to coffee producers (Bro et al. Citation2020). Improving access to financial resources can be critical to enable producers to invest in practices that have upfront costs such as installing irrigation systems (Baca et al. Citation2014). Furthermore, instigating change within the coffee market so more sustainable production practices are reflected in the end-product value could also drive positive change. Premium prices of shade-grown beans in sustainable coffee markets reflect such opportunities for resilience-building practices to be more financially viable (Giovannucci and Koekoek Citation2007). Fundamentally, policies that can reduce producers’ financial risk of adapting practices can encourage systems that are both profitable and ecologically resilient in the long term, thus providing environmental and livelihood benefits (Fain et al. Citation2018).
Creating and improving data systems that coffee producers can easily access, regarding outbreaks of pests and meteorological data may reduce barriers for building resilience (Chengappa, Devika, and Rudragouda Citation2017). For example, since the epidemics of CLR in Central America, early warning systems have been developed that identify and communicate risks to coffee producers based on weather, economic, ecological and phenological indicators from previous outbreaks (Avelino et al. Citation2015). Tailoring policies to local conditions, and involving coffee producers themselves are imperative to sustaining production under climate change and thus maintaining livelihoods (Hirons et al. Citation2018). Local impacts of climate change on coffee production differ dramatically depending on altitude, existing land use and biodiversity, and socioeconomic variables (Jezeer et al. Citation2019). For example, the priority of coffee farmers reported by Harvey et al. (Citation2018) in Guatemala was agrochemical accessibility, whilst in Costa Rica, technical support was in greatest demand.
Approaching resilience-building as an ongoing, dynamic process is essential given the future possibilities of how climate change (and the effects of other cumulative environmental stresses) could manifest and impact coffee production. Looking broadly at the institutions and networks relating to climate vulnerability and resilience in coffee production will help to create more connected strategies of climate resilience beyond the field scale (Capitani et al. Citation2019; Eakin, Winkels, and Sendzimir Citation2009).
A provisional research agenda for resilient coffee and coffee farming communities
Our review has highlighted a series of research questions for the sector to address, and that resilient coffee production and livelihoods needs insights from both natural and social science. The majority of studies have focussed more on Arabica rather than Robusta, although many studies will have implications for both (). In addition, the majority of studies have been conducted in the Americas, with comparatively fewer studies elsewhere in major coffee producing regions. While we have identified a range of studies investigating different climate resilient farming practices, less research has focused on the integrated effects of multiple combined practices. Integrating multiple practices may be more costly than implementing a single practice, but there are likely to be synergistic benefits from integrating multiple regenerative techniques alongside new coffee cultivars (Häger Citation2012), with diversified income streams for farmers (for example through intercropping with a second crop; Moreira et al. Citation2018). These are also few studies which have quantified the potential of the upscaling of individual or integrated farming practices for climate change mitigation. This is likely due to a shortage of suitable datasets. Smallholder farmers often undertake a highly diverse range of practices across a coffee farming landscape, which can contribute to heterogeneity and potentially resilience, but have not been validated at scale. Thus, co-development and farmer-led research is also urgently needed to better understand the benefits of current practices, combined with knowledge sharing across the sector to better accelerate adoption of best management practices.
Fundamentally, adaptation to the rapidly changing climate necessitates multiscale collaboration and knowledge-sharing (Fain et al. Citation2018). Smallholder experiences of cultivating coffee under local climatic conditions are invaluable and need to be part of policy that drives accessible changes to make coffee production more sustainable. There is an urgent need to use existing knowledge of practices that build resilience into the coffee production systems on which the livelihoods of millions of people depend, as well as driving innovative development of regenerative forms of coffee production that overcome the challenges posed by climate change and preserve ecosystem health. Finally, continuing research on the benefits and trade-offs of different interventions for enhancing resilience in terms of environmental and agronomic impacts must also be integrated with assessments of the accessibility of such practices to smallholder farmers, including an understanding of the costs, technical and social barriers to adoption (Abebe Citation2020).
Conclusions
Here, we have identified and assessed a range of approaches that can enhance the resilience of coffee production and coffee-based livelihoods to climate change through improved crop, water and soil management. Our review highlights agroforestry as an important and relatively financially accessible practice for mitigating climate effect in coffee. Other practices, including selective breeding for more heat and drought tolerant varieties of coffee, conserving water resources, and employing principles of regenerative agriculture, can also contribute to the increased climate resilience. Significantly, current climate change trajectories will make large proportions of land currently used to cultivate coffee unviable in the future, and there are technical, financial, and environment constraints to shifting production to higher altitudes. We have identified a series of knowledge gaps that require addressing in order to enhance the climate resilience of coffee production systems, and the livelihood resilience of coffee farming communities, including a shortage of adaptation strategies for Robusta, and a need for co-development of research questions with farmers.
Crucially, enhancing climate and livelihood resilience in the sector will require farmer-led initiatives supported by available technical and financial resources. Our review indicates that few studies consider the synergies or trade-offs from integrating more than one practice on climate resilience. Undoubtedly, implementing integrated solutions will be central to building climate resilience into coffee production. Due to the delay between planting new coffee crops and harvesting the first yields, it is important that adaptations to improve the resilience of coffee production to climate change are facilitated as soon as possible. In addition, the time scale on which the effects of changing these practices will influence resilience is an imperative consideration moving forward as a consequence of the time gap between implementing change and enhanced resilience.
Supplemental Material
Download Comma-Separated Values File (3.1 KB)Acknowledgment
The authors would like to thank colleagues in the Cranfield School of Water, Energy and Environment for feedback on earlier drafts, as well as the reviewers of this manuscript.
Disclosure statement
No potential conflict of interest was reported by the author(s).
Supplemental data
Supplemental data for this article can be accessed online at https://doi.org/10.1080/21683565.2023.2225438.
Additional information
Funding
References
- Abebe, G. 2020. Dealing with climate change and other stressors: Small-scale coffee farmers in the fero-two peasant association in the Wensho district, southern Ethiopia. GeoJournal, 86(6):2539–54. Available at doi:10.1007/s10708-020-10210-7.
- Aerts, R., L. Geeraert, G. Berecha, K. Hundera, B. Muys, H. De Kort, and O. Honnay. 2017. Conserving wild Arabica coffee: Emerging threats and opportunities. Agriculture, Ecosystems & Environment 237:75–79. doi:10.1016/j.agee.2016.12.023.
- Aguiar, M. I. D. (2008) Qualidade física do solo em sistemas agroflorestais, Master’s Thesis. Universidade Federal de Viçosa, Brasil.
- Alpízar, F., F. Carlsson, and M. A. Naranjo. 2011. The effect of ambiguous risk, and coordination on farmers’ adaptation to climate change – a framed field experiment. Ecological Economics 70 (12):2317–26. doi:10.1016/j.ecolecon.2011.07.004.
- Andrade, H. J., and P. C. Zapata. 2019. Mitigation of climate change of coffee production systems in cundinamarca, colombia. Floresta e Ambiente, 26 Instituto de Florestas da Universidade Federal Rural do Rio de Janeiro 26 (3): Available at. doi:10.1590/2179-8087.012618.
- Armbrecht, I., I. Perfecto, and J. Vandermeer. 2004. Enigmatic biodiversity correlations: Ant diversity responds to diverse resources. Science 304 (5668):284–86. doi:10.1126/science.1094981.
- Avelino, J., M. Cristancho, S. Georgiou, P. Imbach, L. Aguilar, G. Bornemann, P. Läderach, F. Anzueto, A. J. Hruska, and C. Morales. 2015. The coffee rust crises in Colombia and Central America (2008–2013): Impacts, plausible causes and proposed solutions. Food Security 7 (2):303–21. doi:10.1007/s12571-015-0446-9.
- Baca, M., P. Läderach, J. Haggar, G. Schroth, O. Ovalle, and B. Bond-Lamberty. 2014. An integrated framework for assessing vulnerability to climate change and developing adaptation strategies for coffee growing families in mesoamerica. PLoS ONE 9 (2):e88463. doi:10.1371/journal.pone.0088463.
- Barrucand, M. G., C. Giraldo Vieira, and P. O. Canziani. 2017. Climate change and its impacts: Perception and adaptation in rural areas of Manizales, Colombia. Climate and Development 9 (5):415–27. doi:10.1080/17565529.2016.1167661.
- Bebber, D. P., A. D. Castillo, and S. J. Gurr. 2016. ‘Modelling coffee leaf rust risk in Colombia with climate reanalysis data’ Phil, R. Soc. Trans., B371. doi:10.1098/rstb.2015.0458
- Beer, J., R. Muschler, D. Kass, and E. Somarriba. 1998. Shade management in coffee and cacao plantations. In Directions in tropical agroforestry research, P. K. R. Nair and C. R. Latt, ed. Forestry SciencesAvailable at, 139–64. Dordrecht, Netherlands: Springer Netherlands. doi:10.1007/978-94-015-9008-2_6.
- Betemariyam, M., M. Negash, and A. Worku. 2020. Comparative analysis of carbon stocks in home garden and adjacent coffee based agroforestry systems in Ethiopia. Small-Scale Forestry 19 (3):319–34. doi:10.1007/s11842-020-09439-4.
- Boreux, V., C. G. Kushalappa, P. Vaast, and J. Ghazoul. 2013. Interactive effects among ecosystem services and management practices on crop production: Pollination in coffee agroforestry systems. Proceedings of the National Academy of Sciences 110 (21):8387–92. doi:10.1073/pnas.1210590110.
- Bro, A. S., D. C. Clay, D. L. Ortega, and M. C. Lopez. 2019. Determinants of adoption of sustainable production practices among smallholder coffee producers in Nicaragua. Environment, Development and Sustainability 21 (2):895–915. doi:10.1007/s10668-017-0066-y.
- Bro, A. S., D. L. Ortega, D. C. Clay, and R. B. Richardson. 2020. Understanding individuals’ incentives for climate change adaptation in Nicaragua’s coffee sector. Climate and Development 12 (4):332–42. doi:10.1080/17565529.2019.1619506.
- Bunn, C., P. Läderach, J. G. P. Jimenez, C. Montagnon, T. Schilling, and J. A. Añel. 2015. Multiclass classification of agro-ecological zones for arabica coffee: An improved understanding of the impacts of climate change. PLos One, 10(10):e0140490. Public Library of Science doi:10.1371/journal.pone.0140490.
- Bunn, C., P. Läderach, O. Ovalle Rivera, and D. Kirschke. 2015. A bitter cup: Climate change profile of global production of Arabica and Robusta coffee. Climatic Change 129 (1–2):89–101. doi:10.1007/s10584-014-1306-x.
- Burgess, S. S. O., M. A. Adams, N. C. Turner, and C. K. Ong. 1998. The redistribution of soil water by tree root systems. Oecologia 115 (3):306–11. doi:10.1007/s004420050521.
- Camargo, M. B. P. D. 2010. The impact of climatic variability and climate change on Arabic coffee crop in Brazil. Bragantia 69 (1):239–47. doi:10.1590/S0006-87052010000100030.
- Canal-Daza, D., and H. Andrade-Castañeda. 2019. Adaptation to climate change in coffee production systems in Tolima. Floresta e Ambiente 26 (3). doi:10.1590/2179-8087.116517.
- Capitani, C., W. Garedew, A. Mitiku, G. Berecha, B. T. Hailu, J. Heiskanen, P. Hurskainen, P. J. Platts, M. Siljander, F. Pinard, et al. 2019. Views from two mountains: Exploring climate change impacts on traditional farming communities of Eastern Africa highlands through participatory scenarios. Sustainability Science 14 (1):191–203. doi:10.1007/s11625-018-0622-x.
- Caramori, P. H., A. Androcioli Filho, and A. C. Leal. 1996. Coffee shade with Mimosa scabrella benth. for frost protection in southern Brazil. Agroforestry Systems 33 (3):205–14. doi:10.1007/BF00055423.
- Carr, M. K. V. 2000. The Water relations and irrigation requirements of coffee. Experimental agriculture 37 (1):1–36. doi:10.1017/S0014479701001090.
- Carr, M. K. V. 2001. The water relations and irrigation requirements of coffee. Experimental Agriculture 37 (1):1–36.
- Castellanos, E. J., C. Tucker, H. Eakin, H. Morales, J. F. Barrera, and R. Díaz. 2013. Assessing the adaptation strategies of farmers facing multiple stressors: Lessons from the coffee and global changes project in Mesoamerica. Environmental Science & Policy 26:19–28. doi:10.1016/j.envsci.2012.07.003.
- Chain-Guadarrama, A., A. Martínez-Salinas, N. Aristizábal, and T. H. Ricketts. 2019. Ecosystem services by birds and bees to coffee in a changing climate: A review of coffee berry borer control and pollination. Agriculture, Ecosystems & Environment 280:53–67. doi:10.1016/j.agee.2019.04.011.
- Chemura, A., D. Kutywayo, P. Chidoko, and C. Mahoya. 2016. Bioclimatic modelling of current and projected climatic suitability of coffee (Coffea Arabica) production in Zimbabwe. Regional Environmental Change 16 (2):473–85. doi:10.1007/s10113-015-0762-9.
- Chengappa, P. G., C. M. Devika, and C. S. Rudragouda. 2017. Climate variability and mitigation: Perceptions and strategies adopted by traditional coffee growers in India. Climate and Development 9 (7):593–604. doi:10.1080/17565529.2017.1318740.
- Coltri, P. P., H. S. Pinto, R. R. D. V. Gonçalves, J. Zullo Junior, and V. Dubreuil. 2019. Low levels of shade and climate change adaptation of Arabica coffee in southeastern Brazil. Heliyon 5 (2):e01263. doi:10.1016/j.heliyon.2019.e01263.
- Craparo, A. C. W., P. J. A. Van Asten, P. Läderach, L. T. P. Jassogne, and S. W. Grab. 2015. Coffea Arabica yields decline in Tanzania due to climate change: Global implications. Agricultural and Forest Meteorology 207:1–10. doi:10.1016/j.agrformet.2015.03.005.
- DaMatta, F. M. 2004. Ecophysiological constraints on the production of shaded and unshaded coffee: A review. Field Crops Research 86 (2):99–114. doi:10.1016/j.fcr.2003.09.001.
- DaMatta, F. M., E. Rahn, P. Läderach, R. Ghini, and J. C. Ramalho. 2019. Why could the coffee crop endure climate change and global warming to a greater extent than previously estimated? Climatic Change 152 (1):167–78. doi:10.1007/s10584-018-2346-4.
- DaMatta, F. M., and J. D. C. Ramalho. 2006. Impacts of drought and temperature stress on coffee physiology and production: A review. Brazilian Journal of Plant Physiology 18 (1):55–81. doi:10.1590/S1677-04202006000100006.
- DaMatta, F. M., C. P. Ronchi, M. Maestri, and R. S. Barros. 2007. Ecophysiology of coffee growth and production. Brazilian Journal of Plant Physiology 19 (4):485–510. doi:10.1590/S1677-04202007000400014.
- Davis, A. P., H. Chadburn, J. Moat, R. O’Sullivan, S. Hargreaves, and E. N. Lughadha. 2019. High extinction risk for wild coffee species and implications for coffee sector sustainability. Science Advances 5 (1). doi:10.1126/sciadv.aav3473.
- Davis, A. P., T. W. Gole, S. Baena, J. Moat, and B. Fenton. 2012. The impact of climate change on indigenous arabica coffee (Coffea Arabica): predicting future trends and identifying priorities. PLoS ONE 7 (11):e47981. doi:10.1371/journal.pone.0047981.
- Davis, A. P., D. Mieulet, J. Moat, D. Sarmu, and J. Haggar. 2021. Arabica-like flavour in a heat-tolerant wild coffee species. Nature Plants 7 (4):413–18. doi:10.1038/s41477-021-00891-4.
- de Sousa, K., M. van Zonneveld, M. Holmgren, R. Kindt, and J. C. Ordoñez. 2019. The future of coffee and cocoa agroforestry in a warmer Mesoamerica. Scientific Reports 9 (1): Nature Publishing Group. doi:10.1038/s41598-019-45491-7.
- de Souza, H. N., R. G. M. de Goede, L. Brussaard, I. M. Cardoso, E. M. G. Duarte, R. B. A. Fernandes, L. C. Gomes, and M. M. Pulleman. 2012. Protective shade, tree diversity and soil properties in coffee agroforestry systems in the Atlantic Rainforest biome. Agriculture, Ecosystems & Environment 146 (1):179–96. doi:10.1016/j.agee.2011.11.007.
- Devagiri, G. M., A. K. Khaple, H. B. Anithraj, C. G. Kushalappa, A. K. Krishnappa, and S. B. Mishra. 2020. Assessment of tree diversity and above-ground biomass in coffee agroforest dominated tropical landscape of India’s Central Western Ghats. Journal of Forestry Research 31 (3):1005–15. doi:10.1007/s11676-019-00885-1.
- dos Santos, C. A. F., A. E. Leitao, I. P. Pais, F. C. Lidon, and J. C. Ramalho. 2015. Perspectives on the potential impacts of climate changes on coffee plant and bean quality. Emirates Journal of Food and Agriculture 27 (2):152–63. doi:10.9755/ejfa.v27i2.19468.
- Dufour, B. P., I. W. Kerana, and F. Ribeyre. 2019. Effect of coffee tree pruning on berry production and coffee berry borer infestation in the Toba Highlands (North Sumatra). Crop Protection 122:151–58. doi:10.1016/j.cropro.2019.05.003.
- Dumont, E. S., A. Gassner, G. Agaba, R. Nansamba, and F. Sinclair. 2019. The utility of farmer ranking of tree attributes for selecting companion trees in coffee production systems. Agroforestry Systems 93 (4):1469–83. doi:10.1007/s10457-018-0257-z.
- Eakin, H., C. M. Tucker, E. Castellanos, R. Diaz-Porras, J. F. Barrera, and H. Morales. 2014. Adaptation in a multi-stressor environment: Perceptions and responses to climatic and economic risks by coffee growers in Mesoamerica. Environment, Development and Sustainability 16 (1):123–39. doi:10.1007/s10668-013-9466-9.
- Eakin, H., A. Winkels, and J. Sendzimir. 2009. Nested vulnerability: Exploring cross-scale linkages and vulnerability teleconnections in Mexican and Vietnamese coffee systems. Environmental Science & Policy 12 (4):398–412. doi:10.1016/j.envsci.2008.09.003.
- Elevitch, C. R., D. N. Mazaroli, and D. Ragone. 2018. Agroforestry standards for regenerative agriculture. Sustainability 10 (9): Multidisciplinary Digital Publishing Institute. doi:10.3390/su10093337.
- Eshetu, G., T. Johansson, W. Garedew, and T. Yisahak. 2021. Determinants of smallholder farmers’ adaptation options to climate change in a coffee-based farming system of Southwest Ethiopia. Climate and Development, 13(4):318–25. Taylor & Francis doi:10.1080/17565529.2020.1772706.
- Fain, S. J., M. Quiñones, N. L. Álvarez-Berríos, I. K. Parés-Ramos, and W. A. Gould. 2018. Climate change and coffee: Assessing vulnerability by modeling future climate suitability in the Caribbean island of Puerto Rico. Climatic Change 146 (1–2):175–86. doi:10.1007/s10584-017-1949-5.
- FAO. (2015). ‘Phasing out Highly Hazardous Pesticides is possible! Farmer experiences in growing coffee without endosulfan’. Available at: http://www.fao.org/3/i4573e/i4573e.pdf/ [Accessed 10 July 2021].
- FAOSTAT. (2019). Crop and livestock products. Available at: http://www.fao.org/3/i4573e/i4573e.pdf [Accessed 15 June 2021].
- Gallopín, G. C. 2006. Linkages between vulnerability, resilience, and adaptive capacity. Global Environmental Change 16 (3):293–303. doi:10.1016/j.gloenvcha.2006.02.004.
- Garedew, W., B. T. Hailu, F. Lemessa, P. Pellikka, and F. Pinard. 2017. Coffee shade tree management: An adaptation option for climate change impact for small scale coffee growers in South-West Ethiopia. In Climate Change Adaptation in Africa. Climate Change Management, W. Leal Filho, S. Belay, J. Kalangu, W. Menas, P. Munishi, and K. Musiyiwa, ed., 647–59. Cham, Switzerland: Springer International Publishing. doi:10.1007/978-3-319-49520-0_40.
- Garzón, A. F., L. P. P. Rivas, and L. M. Avellaneda-Torres. 2020. Effect of management (ecological and conventional) on functional groups of soil microorganisms in coffee agroecosystems with different resilience to climate variability, Colombia. Acta Scientiarum Biological Sciences 42:e48620. doi:10.4025/actascibiolsci.v42i1.48620.
- Gidey, T., T. S. Oliveira, J. Crous-Duran, and J. H. N. Palma. 2020. Using the yield-SAFE model to assess the impacts of climate change on yield of coffee (Coffea Arabica L.) under agroforestry and monoculture systems. Agroforestry Systems 94 (1):57–70. doi:10.1007/s10457-019-00369-5.
- Giovannucci, D., and F. J. Koekoek (2007) The State of Sustainable Coffee: A Study of Twelve Major Markets. Rochester, NY: Social Science Research Network. https://papers.ssrn.com/abstract=996763
- Girkin, N. T., and H. V. Cooper. 2022. Nitrogen and ammonia in soils. In Module in earth systems and environmental sciences. Netherlands: Elsevier. https://doi.org/10.1016/B978-0-12-822974-3.00010-0
- Gomes, L. C., F. J. J. A. Bianchi, I. M. Cardoso, R. B. A. Fernandes, E. I. F. Filho, and R. P. O. Schulte. 2020. Agroforestry systems can mitigate the impacts of climate change on coffee production: A spatially explicit assessment in Brazil. Agriculture, Ecosystems & Environment 294:106858. doi:10.1016/j.agee.2020.106858.
- Häger, A. 2012. The effects of management and plant diversity on carbon storage in coffee agroforestry systems in Costa Rica. Agroforestry Systems 86 (2):159–74. doi:10.1007/s10457-012-9545-1.
- Hammersley, M. 2001. On “systematic” reviews of research literatures: A “narrative” response to evans & benefield. British Educational Research Journal 27 (5):543–54. doi:10.1080/01411920120095726.
- Harvey, C. A., M. Saborio-Rodríguez, M. R. Martinez-Rodríguez, B. Viguera, A. Chain-Guadarrama, R. Vignola, and F. Alpizar. 2018. Climate change impacts and adaptation among smallholder farmers in Central America. Agriculture & Food Security 7 (1):57. doi:10.1186/s40066-018-0209-x.
- Hirons, M., Z. Mehrabi, T. A. Gonfa, A. Morel, T. W. Gole, C. McDermott, E. Boyd, E. Robinson, D. Sheleme, Y. Malhi, et al. 2018. Pursuing climate resilient coffee in Ethiopia – a critical review. Geoforum 91:108–16. doi:10.1016/j.geoforum.2018.02.032.
- Hochachka, G. (2021) ‘Integrating the four faces of climate change adaptation: Towards transformative change in Guatemalan coffee communities’, World Development, 105361
- Imbach, P., E. Fung, L. Hannah, C. E. Navarro-Racines, D. W. Roubik, T. H. Ricketts, C. A. Harvey, C. I. Donatti, P. Läderach, B. Locatelli, et al. 2017. Coupling of pollination services and coffee suitability under climate change. Proceedings of the National Academy of Sciences 114 (39):10438–42. doi:10.1073/pnas.1617940114.
- IPCC. 2018. Summary for Policymakers. In Global Warming of 1.5°C. An IPCC Special Report on the impacts of global warming of 1.5°C above pre-industrial levels and related global greenhouse gas emission pathways, in the context of strengthening the global response to the threat of climate change, sustainable development, and efforts to eradicate poverty’, ed., Masson-Delmotte, V., P. Zhai, H.-O. Pörtner, D. Roberts, J. Skea, P. R. Shukla, A. Pirani, W. Moufouma-Okia, C. Péan, R. Pidcock, S. Connors, J. B. R. Matthews, Y. Chen, X. Zhou, M. I. Gomis, E. Lonnoy, T. Maycock, M. Tignor, and T. Waterfield, Geneva: World Meteorological Organization. Available at https://www.ipcc.ch/sr15/
- IPCC (2021) Climate change 2021: The physical science basis. Contribution of Working Group I to the Sixth Assessment Report of the Intergovernmental Panel on Climate Change. Cambridge University Press.
- Jacobi, J., S.-L. Mathez-Stiefel, H. Gambon, S. Rist, and M. Altieri. 2017. Whose knowledge, whose development? Use and role of local and external knowledge in agroforestry projects in Bolivia. Environmental Management 59 (3):464–76. doi:10.1007/s00267-016-0805-0.
- Jaramillo, J., A. Chabi-Olaye, C. Kamonjo, A. Jaramillo, F. E. Vega, H.-M. Poehling, and C. Borgemeister. 2009. Thermal tolerance of the coffee berry borer hypothenemus hampei: Predictions of climate change impact on a tropical insect pest. PLos One, 4(8):e6487. Public Library of Science doi:10.1371/journal.pone.0006487.
- Jaramillo, J., E. Muchugu, F. E. Vega, A. Davis, C. Borgemeister, A. Chabi-Olaye, and S. Thrush. 2011. Some like it hot: The influence and implications of climate change on coffee berry borer (Hypothenemus hampei) and coffee production in East Africa. PloS One 6 (9):e24528. doi:10.1371/journal.pone.0024528.
- Jaramillo, J., M. Setamou, E. Muchugu, A. Chabi-Olaye, A. Jaramillo, J. Mukabana, J. Maina, S. Gathara, C. Borgemeister, and J. P. Hart. 2013. Climate change or urbanization? Impacts on a traditional coffee production system in East Africa over the Last 80 Years. PLoS ONE 8 (1):e51815. doi:10.1371/journal.pone.0051815.
- Jezeer, R. E., P. A. Verweij, R. G. A. Boot, M. Junginger, and M. J. Santos. 2019. Influence of livelihood assets, experienced shocks and perceived risks on smallholder coffee farming practices in Peru. Journal of Environmental Management 242:496–506. doi:10.1016/j.jenvman.2019.04.101.
- Jha, S., C. M. Bacon, S. M. Philpott, V. Ernesto Méndez, P. Läderach, and R. A. Rice. 2014. Shade coffee: Update on a disappearing refuge for biodiversity. BioScience 64 (5):416–28. doi:10.1093/biosci/biu038.
- Kath, J., V. M. Byrareddy, A. Craparo, T. Nguyen-Huy, S. Mushtaq, L. Cao, and L. Bossolasco. 2020. Not so robust: Robusta coffee production is highly sensitive to temperature. Global Change Biology 26 (6):3677–88. doi:10.1111/gcb.15097.
- Kjellstrom, T., I. Holmer, and B. Lemke. 2009. Workplace heat stress, health and productivity – an increasing challenge for low and middle-income countries during climate change. Globalization and Health, 2(1):2047. Action, 2 doi:10.3402/gha.v2i0.2047.
- Läderach, P., M. Lundy, A. Jarvis, J. Ramirez, E. P. Portilla, K. Schepp, and A. Eitzinger. 2011. Predicted impact of climate change on coffee supply chains. In Leal Filho, W. (ed.) The Economic, Social and Political Elements of Climate Change, 703–23. Berlin: Springer. Climate Change Management. 10.1007/978-3-642-14776-0_42.
- Läderach, P., J. Ramirez–Villegas, C. Navarro-Racines, C. Zelaya, A. Martinez–Valle, and A. Jarvis. 2017. Climate change adaptation of coffee production in space and time. Climatic Change 141 (1):47–62. doi:10.1007/s10584-016-1788-9.
- Lin, B. B. 2007. Agroforestry management as an adaptive strategy against potential microclimate extremes in coffee agriculture. Agricultural and Forest Meteorology 144 (1–2):85–94. doi:10.1016/j.agrformet.2006.12.009.
- Lin, B. B. 2009. Coffee (Café Arabica var. Bourbon) fruit growth and development under varying shade levels in the soconusco region of chiapas, Mexico. Journal of Sustainable Agriculture 33 (1):51–65. doi:10.1080/10440040802395007.
- Lin, B. B. 2010. The role of agroforestry in reducing water loss through soil evaporation and crop transpiration in coffee agroecosystems. Agricultural and Forest Meteorology 150 (4):510–18. doi:10.1016/j.agrformet.2009.11.010.
- Li, D.-Z., and H. W. Pritchard. 2009. The science and economics of ex situ plant conservation. Trends in Plant Science 14 (11):614–21. doi:10.1016/j.tplants.2009.09.005.
- Moat, J., J. Williams, S. Baena, T. Wilkinson, T. W. Gole, Z. K. Challa, S. Demissew, and A. P. Davis. 2017. Resilience potential of the Ethiopian coffee sector under climate change. Nature Plants 3 (7):1–14. doi:10.1038/nplants.2017.81.
- Moreira, S. L. S., C. V. Pires, G. E. Marcatti, R. H. S. Santos, H. M. A. Imbuzeiro, and R. B. A. Fernandes. 2018. Intercropping of coffee with the palm tree, macauba, can mitigate climate change effects. Agricultural and Forest Meteorology 256–257:379–90. doi:10.1016/j.agrformet.2018.03.026.
- Muschler, R. G. 2001. Shade improves coffee quality in a sub-optimal coffee-zone of Costa Rica. Agroforestry Systems 85 (2):131–39. doi:10.1023/A:1010603320653.
- Nesper, M., C. Kueffer, S. Krishnan, C. G. Kushalappa, J. Ghazoul, and J. Firn. 2019. Simplification of shade tree diversity reduces nutrient cycling resilience in coffee agroforestry. Journal of Applied Ecology 56 (1):119–31. doi:10.1111/1365-2664.13176.
- Nguyen, N., and E. G. Drakou. 2021. Farmers intention to adopt sustainable agriculture hinges on climate awareness: The case of Vietnamese coffee. Journal of Cleaner Production 303:126828. doi:10.1016/j.jclepro.2021.126828.
- Noponen, M. R. A., G. Edwards-Jones, J. P. Haggar, G. Soto, N. Attarzadeh, and J. R. Healey. 2012. Greenhouse gas emissions in coffee grown with differing input levels under conventional and organic management. Agriculture, Ecosystems & Environment 151:6–15. doi:10.1016/j.agee.2012.01.019.
- Noponen, M. R. A., J. R. Healey, G. Soto, and J. P. Haggar. 2013. Sink or source—The potential of coffee agroforestry systems to sequester atmospheric CO2 into soil organic carbon. Agriculture, Ecosystems & Environment 175:60–68. doi:10.1016/j.agee.2013.04.012.
- Ovalle-Rivera, O., P. Läderach, C. Bunn, M. Obersteiner, G. Schroth, and R. D. Loyola. 2015. Projected shifts in coffea arabica suitability among major global producing regions due to climate change. PLOS ONE 10 (4):e0124155. doi:10.1371/journal.pone.0124155.
- Pappo, E., C. Wilson, S. L. Flory, and M. Schipanksi. 2021. Hybrid coffee cultivars may enhance agroecosystem resilience to climate change. AoB Plants 13 (2): Available at. doi:10.1093/aobpla/plab010.
- Perfecto, I., Z. Hajian-Forooshani, A. Iverson, A. D. Irizarry, J. Lugo-Perez, N. Medina, C. Vaidya, A. White, and J. Vandermeer. 2019. Response of coffee farms to hurricane maria: Resistance and resilience from an extreme climatic event. Scientific Reports 9 (1):15668. doi:10.1038/s41598-019-51416-1.
- Perfecto, I., M. E. Jiménez-Soto, and J. Vandermeer. 2019. Coffee landscapes shaping the anthropocene: Forced simplification on a complex agroecological landscape. Current Anthropology 60 (S20):S236–S50. doi:10.1086/703413.
- Perfecto, I., and J. H. Vandermeer. 2015. Coffee agroecology: A new approach to understanding agricultural biodiversity, ecosystem services, and sustainable development. London, New York, USA: Routledge. doi:10.4324/9780203526712.
- Pham, Y., K. Reardon-Smith, S. Mushtaq, and G. Cockfield. 2019. The impact of climate change and variability on coffee production: A systematic review. Climatic Change 156 (4):609–30. doi:10.1007/s10584-019-02538-y.
- Pickering, C., J. Grignon, R. Steven, D. Guitart, and J. Byrne. 2015. Publishing not perishing: How research students transition from novice to knowledgeable using systematic quantitative literature reviews. Studies in Higher Education 40 (10):1756–69. doi:10.1080/03075079.2014.914907.
- Quiroga, S., C. Suárez, J. Diego Solís, and P. Martinez-Juarez. 2020. Framing vulnerability and coffee farmers’ behaviour in the context of climate change adaptation in Nicaragua. World Development 126:104733. doi:10.1016/j.worlddev.2019.104733.
- Quiroga, S., C. Suárez, and J. D. Solís. 2015. Exploring coffee farmers’ awareness about climate change and water needs: Smallholders’ perceptions of adaptive capacity. Environmental Science & Policy 45:53–66. doi:10.1016/j.envsci.2014.09.007.
- Rahn, E., P. Läderach, M. Baca, C. Cressy, G. Schroth, D. Malin, H. van Rikxoort, and J. Shriver. 2014. Climate change adaptation, mitigation and livelihood benefits in coffee production: Where are the synergies? Mitigation and Adaptation Strategies for Global Change 19 (8):1119–37. doi:10.1007/s11027-013-9467-x.
- Ramirez-Villegas, J., M. Salazar, A. Jarvis, and C. E. Navarro-Racines. 2012. A way forward on adaptation to climate change in Colombian agriculture: Perspectives towards 2050. Climatic Change 115 (3–4):611–28. doi:10.1007/s10584-012-0500-y.
- Reay, D. 2019. Climate-smart coffee. In Climate-smart food, D. Reay, ed. Available at, 93–104. Cham, Switzerland: Springer International Publishing. doi:10.1007/978-3-030-18206-9_8.
- Rhiney, K., Z. Guido, C. Knudson, J. Avelino, C. M. Bacon, G. Leclerc, and M. C. Aime, D. P. Bebber. 2021. Epidemics and the future of coffee production. Proceedings of the National Academy of Sciences 118 (27). doi:10.1073/pnas.2023212118.
- Rice, R. A. 1999. A place unbecoming: The coffee farm of Northern Latin America*. Geographical Review 89 (4):554–79. doi:10.1111/j.1931-0846.1999.tb00234.x.
- Ruiz Meza, L. E. 2015. Adaptive capacity of small-scale coffee farmers to climate change impacts in the Soconusco region of Chiapas, Mexico. Climate and Development 7 (2):100–09. doi:10.1080/17565529.2014.900472.
- Salamanca-Jimenez, A., T. A. Doane, and W. R. Horwath. 2017. Nitrogen use efficiency of coffee at the vegetative stage as influenced by fertilizer application method. Frontiers in Plant Science 8: Frontiers Available at. doi:10.3389/fpls.2017.00223.
- Sarmiento-Soler, A., P. Vaast, M. P. Hoffmann, L. Jassogne, P. van Asten, S. Graefe, and R. P. Rötter. 2020. Effect of cropping system, shade cover and altitudinal gradient on coffee yield components at Mt. Elgon, Uganda. Agriculture, Ecosystems & Environment 295:106887. doi:10.1016/j.agee.2020.106887.
- Schepp, K. 2009. Smallholders adapt to climate change. Appropriate Technology 36 (3):64–67. Burnham, United Kingdom: Research Information Ltd.
- Schroth, G., P. Läderach, D. S. Blackburn Cuero, J. Neilson, and C. Bunn. 2015. Winner or loser of climate change? A modeling study of current and future climatic suitability of Arabica coffee in Indonesia. Regional Environmental Change 15 (7):1473–82. doi:10.1007/s10113-014-0713-x.
- Schroth, G., P. Laderach, J. Dempewolf, S. Philpott, J. Haggar, H. Eakin, T. Castillejos, J. Garcia Moreno, L. Soto Pinto, R. Hernandez, et al. 2009. Towards a climate change adaptation strategy for coffee communities and ecosystems in the Sierra Madre de Chiapas, Mexico. Mitigation and Adaptation Strategies for Global Change 14 (7):605–25. doi:10.1007/s11027-009-9186-5.
- Shapiro-Garza, E., D. King, A. Rivera-Aguirre, S. Wang, and J. Finley-Lezcano. 2020. A participatory framework for feasibility assessments of climate change resilience strategies for smallholders: Lessons from coffee cooperatives in Latin America. International Journal of Agricultural Sustainability 18 (1):21–34. doi:10.1080/14735903.2019.1658841.
- Shinbrot, X. A., K. W. Jones, A. Rivera-Castañeda, W. López-Báez, and D. S. Ojima. 2019. Smallholder farmer adoption of climate-related adaptation strategies: the importance of vulnerability context, livelihood assets, and climate perceptions. Environmental Management 63 (5):583–95. doi:10.1007/s00267-019-01152-z.
- Soto-Pinto, L., M. Anzueto, J. Mendoza, G. J. Ferrer, and B. de Jong. 2010. Carbon sequestration through agroforestry in indigenous communities of Chiapas, Mexico. Agroforestry Systems 78 (1):39–51. doi:10.1007/s10457-009-9247-5.
- Souza, H. N., I. M. Cardoso, J. M. Fernandes, F. C. P. Garcia, V. R. Bonfim, A. C. Santos, A. F. Carvalho, and E. S. Mendonça. 2010. Selection of native trees for intercropping with coffee in the Atlantic Rainforest biome. Agroforestry Systems 80 (1):1–16. doi:10.1007/s10457-010-9340-9.
- Tanner, T., D. Lewis, D. Wrathall, R. Bronen, N. Cradock-Henry, S. Huq, C. Lawless, R. Nawrotzki, V. Prasad, M. A. Rahman et al. 2015. Livelihood resilience in the face of climate change. Nature Climate Change. 5(1):23–26. doi:10.1038/nclimate2431.
- Tschora, H., and F. Cherubini (2020) ‘Co-benefits and trade-offs of agroforestry for climate change mitigation and other sustainability goals in West Africa’, Global Ecology and Conservation, 22.
- Valencia, V., L. García-Barrios, E. J. Sterling, P. West, A. Meza-Jiménez, and S. Naeem. 2018. Smallholder response to environmental change: Impacts of coffee leaf rust in a forest frontier in Mexico. Land Use Policy 79:463–74. doi:10.1016/j.landusepol.2018.08.020.
- Van Der Vossen, H. A. M. 2005. A critical analysis of the agronomic and economic sustainability of organic coffee production. Experimental Agriculture 41 (4):449–73. doi:10.1017/S0014479705002863.
- Van Der Wolf, J., L. Jassogne, G. Gram, and P. Vaast. 2019. Turning local knowledge on agroforestry into an online decision-support tool for tree selection in smallholders’ farms. Experimental Agriculture 55 (S1):50–66. doi:10.1017/S001447971600017X.
- Vietoris, V., P. Zajác, J. Čapla, A. Mendelová, K. Križanová, and L. Benešová. 2021. Comparison of coffee species by sensory panel and electronic nose. Journal of Microbiology, Biotechnology and Food Sciences 234–37.
- Wagner, S., L. Jassogne, E. Price, M. Jones, and R. Preziosi. 2021. Impact of climate change on the production of coffea arabica at Mt. Kilimanjaro, Tanzania. Agriculture 11 (1):53.
- Watts, C. (2016) ‘A Brewing Storm: The climate change risks to coffee’, available at: https://files.fairtrade.net/publications/2016_TCI_ABrewingStorm.pdf [Accessed 10 July 2021].
- Zaro, G. C., P. H. Caramori, G. M. Yada Junior, C. R. Sanquetta, A. A. Filho, A. L. P. Nunes, C. E. C. Prete, and P. Voroney. 2020. Carbon sequestration in an agroforestry system of coffee with rubber trees compared to open-grown coffee in southern Brazil. Agroforestry Systems 94 (3):799–809. doi:10.1007/s10457-019-00450-z.
- Zullo, J., H. S. Pinto, E. D. Assad, and A. M. H. de Ávila. 2011. Potential for growing Arabica coffee in the extreme south of Brazil in a warmer world. Climatic Change 109 (3–4):535–48. doi:10.1007/s10584-011-0058-0.