Abstract
Cadherin-catenin mediated adhesion is an important determinant of tissue architecture in multicellular organisms. Cancer progression and maintenance is frequently associated with loss of their expression or functional activity, which not only leads to decreased cell-cell adhesion, but also to enhanced tumor cell proliferation and loss of differentiated characteristics. This review is focused on the emerging implications of cadherin-catenin proteins in the regulation of polarized divisions through their connections with the centrosomes, cytoskeleton, tissue tension and signaling pathways; and illustrates how alterations in cadherin-catenin levels or functional activity may render cells susceptible to transformation through the loss of their proliferation-differentiation balance.
Introduction
Since the formulation of the Cell Theory in the 19th century, giant steps in diverse scientific areas have contributed to our current understanding of the function and organization of cells within tissues and how they lead to cancer when gone awry. One major breakthrough that advanced the understanding of the architectural organization of tissues was published in 1963, where Farquar and Palade using electron microscopy analyses defined 3 major intercellular structures as contact points between cells in epithelial tissues.Citation1 These 3 structures defined as zonula occludens (tight junctions), zonula adherens (adherens junctions) and desmosomes exhibit a paradigmatic organization as complexes formed by transmembrane proteins. Among them, the role of cadherin-catenin complexes at adherens junctions (AJs) in maintaining epithelial homeostasis is the best understood. Traditionally, cadherin-catenin complexes were thought to function as static complexes that anchor to the cytoskeleton via cytoplasmic scaffolding proteins, thereby sustaining tissue architecture. However it is now well acknowledged that cadherin-catenin complexes present a dynamic organization and the fundamental paradigms about their mechanical and signaling functions have been constantly subjected to active remodeling. Remarkably, their constitutive and associated proteins can also function outside of the realm of intercellular adhesion. These seemingly jack-of-all-trade proteins are involved in the control of cell polarity, transcription, signaling, proliferation, cell fate and migration.Citation2-4
E-cadherin is the prototypical member of the family of type I classical cadherins, which are transmembrane proteins that through their extracellular domain promote adhesion between adjacent epithelial cells in a calcium dependent manner.Citation5 The intracellular domain associates to β-catenin and p120-catenin, while α-catenin binds to the complex via β-catenin.Citation3 Cadherin-catenin complexes present a dynamic stability, which allows the modulation of adhesion strength to preserve adult tissue homeostasis and the reshaping of epithelial tissues to drive morphogenetic movements during development. The mechanism underlying this key function is the association of catenins with the actin and microtubule networks via actin- and microtubule- binding proteins.Citation6,7 Several studies have provided evidence that alterations in the expression and/or functional activity of the cadherin-catenin complex lead to tumor initiation and progression. This is a consequence not only of decreased cell-cell adhesion, but also of alterations in signaling cascades, and loss of cell contact inhibition of growth.Citation8 Moreover, loss of E-cadherin expression, together with upregulation of N-cadherin, is associated with loss of epithelial characteristics and increased cell migration: a process known as epithelial to mesenchymal transition (EMT). All these processes have been thoroughly delineated in excellent reviews elsewhere.Citation9-12 Less appreciated are the implications of cadherin-catenin complex malfunctioning for the polarized orientation of cell divisions. In this review, we focus on the emerging roles of cadherin-catenin complex proteins in the regulation of oriented cell divisions in mammalian epithelia. First, we briefly describe the polarized features of epithelia to later highlight the novel connections of AJ proteins with centrosomes and spindle positioning during cell division. This is followed by a discussion on the potential role of AJ/actin complexes as mechanosensors that promote polarized cell divisions. Finally, we discuss how when these connections go awry may render epithelial cells more susceptible to transformation, cancer progression and tumor maintenance.
Cell polarity and polarized cell divisions
The correct establishment and maintenance of cell polarity is crucial for cell physiology and tissue homeostasis, and occurs in response to cell intrinsic and extrinsic cues.Citation13,14 At the cellular level, cell polarity involves the acquisition and maintenance of a high level of cellular organization, including a differential distribution of proteins and lipids along the plasma membrane, and the spatial positioning of organelles in the cytoplasmic space, such as the centrosome, the Golgi apparatus and the nuclei.Citation15
A robust polarized distribution of proteins and organelles is also maintained during cell division. Polarized cell divisions can be symmetric, when one cell gives raise to 2 identical daughter cells, or asymmetric, when a dividing cell gives rise to 2 daughter cells that are different in molecular composition, cell size, developmental potential and/or cell fate.Citation14,16,17 Although it still debated for some mammalian tissues, asymmetric cell divisions are particularly relevant in certain adult epithelial stem cell compartments such as the intestine,Citation18,19 mammary epithelia,Citation20,21 lungCitation22,23 and skin,Citation24-28 where the orientation of cell divisions or the final positioning of the daughter cells have major implications in the self-renewal or differentiation properties of progenitor cells, thereby regulating their homeostatic regeneration (). Given the implications of neoplastic alterations in some stem cell compartments to promote tumor initiation and cancer maintenance, or the acquisition of self-renewal characteristics of differentiated cells, it has been proposed that alterations that favor an increase in symmetric cell divisions could serve as a mechanism to expand the cancer stem cell pool.Citation14,17,29 Indeed, the connection between increased symmetric cell divisions and the expansion of cancer stem cells has been observed in some tumors such as breast,Citation20,30 intestine,Citation31,32 lung,Citation33 and skin carcinomas.Citation34
Table 1. Spindle position and cellular fates in different mammalian epithelial tissues
The cellular events that occur during polarized cell divisions involve the organization of intracellular components in relation to the axis of cell division and the basal membrane. This includes an asymmetry of duplicated centrosomes between the mother and daughter cells, the orientation of the mitotic spindle, and the distribution of cell polarity and cell fate determinants. The centrosome functions as the major microtubule-organizing center (MTOC) both in interphase cells and during mitosis.Citation35,36 During mitosis, 3 types of microtubules nucleate from the centrosomes: kinetochore microtubules that attach to the chromosomes, polar microtubules, and astral microtubules that extend to the cell cortex.Citation37 Centrosomes are composed of 2 centrioles surrounded by a pericentriolar material, which contains numerous proteins necessary for microtubule nucleation (γ-tubulin) and anchoring (e.g. Ninein or the motor protein Dynein).Citation35,36 During cell division the centrosome duplicates at the G1/S phase of the cell cycle, and as cell cycle progresses one centrosome migrates to the opposite pole of the dividing cell to form the polar mitotic spindle.Citation17,38,39 The oriented separation/positioning of centrosomes is controlled by several kinases of the cell cycle including members of the NEK, Aurora, and Polo kinase families.Citation40 This is also regulated by a crosstalk with cell polarity determinants promoting the polarized inheritance of the mother or the daughter centrosome by the mother and daughter cell.Citation41 Interestingly, as discussed below, catenin proteins have also been recently associated to the centrosome and the regulation of some of the kinases involved in centrosome duplication.
The orientation of the mitotic spindle requires the interaction of the spindle with cortical sites, and a coordinated crosstalk with the polarity machinery.Citation42 Many of the polarity factors involved in mitotic spindle orientation have been described in Drosophila and are generally conserved in mammalian cells.Citation14,16,17,43 Their role in spindle positioning and asymmetric cell division has been examined in reviews to which we refer the readers for more comprehensive information.Citation14,16,17,42-44 Briefly, before an asymmetric cell division occurs, the apical Par polarity proteins Par3/Par6/atypical protein kinase C (aPKC) localize to the apical cell cortex, along with the Gαi subunit of heteromeric G proteins. aPKC requires the small G-protein Cdc42 for its apical localization and activation. During mitosis, Gαi interacts with proteins associated with astral microtubules at the spindle pole, LGN and NuMA, which are physically linked by the adaptor protein Inscuteable in a mutually exclusive manner.Citation45,46 This complex is also associated with the motor complex Dynein/Dynactin, which generates the force to pull astral microtubules and the centrosome toward the apical cell cortex, ensuring that the mitotic cleavage plane is perpendicular to the apical-basal axis. The cleavage plane then influences the identity and fate adopted by the 2 daughter cells since it is coupled with the asymmetric distribution of cell fate determinants. The Gαi complex also partakes in planar epithelial divisions of epithelial monolayers.Citation47-49 In this case, the Gαi complex recruits Dynein-dynactin to the lateral cortex, which pull spindle poles toward the lateral side of the dividing cells. In certain cell types aPKC plays an active role excluding LGN from the apical domain and restricting it to the lateral cortex.Citation47,50 Citation48
How cells choose their axis of division has been a matter of intense investigation. Recently cadherins are emerging as components of the polarizing machinery during cell division in some cells and tissues. Hence, it is tantalizing to speculate that cadherins and their connections with the cytoskeleton may regulate the position of the mitotic spindles.
Links between cadherin-catenins and positioning of mitotic spindles
The direct functional involvement of AJs in the maintenance of tissue integrity makes it difficult to distinguish the contributions of AJs to organelle positioning from a general disruption of epithelial architecture when AJ proteins are lost or dysfunctional. However, the direct contributions of cadherin-mediated contacts in promoting intracellular asymmetry have been recently substantiated in various mammalian cell types in culture.Citation51-53 In these studies, it was observed that cadherins control the positioning of the nucleus and centrosomes of cells in interphase,Citation51,52 and the spindle orientation of dividing cells.Citation53 In the context of organisms, the best examples of the contributions of cadherin-mediated adhesion to intracellular asymmetry and oriented cell divisions have been obtained from studies in Drosophila and Caenorhabditis elegans. For example, in the Drosophila ovaryCitation54 and in the male germ stem cell niche,Citation55 germ stem cells differentiate precociously when the levels of E-cadherin are reduced or absent and stem cells are no longer maintained within their niche. Interestingly, in the male germline stem cell niche, E-cadherin contributes to centrosome and spindle positioning.Citation55 In addition, the development of the Drosophila neuroepithelium and the sensory organ depends on the AJ-mediated regulation of the distribution of polarity determinants and the orientation of asymmetric cell divisions.Citation56 As a final example, it has also been observed that the ortholog of β-catenin in C.elegans controls cell division orientation in early embryos.Citation57
In mammals, a connection between AJ proteins and intracellular asymmetry during cell division and cell fate has been observed in certain tissues, but mostly characterized in stratified epithelia. For example, in embryonic neural stem cells, it has been documented that AJs are organized into different microdomains that are split unequally during asymmetric cell divisions by the cleavage plane.Citation58 The inheritance of cell fate determinants together with reduced levels of AJs may explain the posterior detachment of the cells that undergo differentiation. Moreover, robust levels of N-cadherin in progenitor cells support their maintenance in their niche by the activation of β-catenin signaling.Citation59 In simple epithelia, it has been proposed that mutations in E-cadherin correlate with an increase in symmetric cell divisions and the expansion of the cancer stem cell pool.Citation60 In stratified epithelia such as the skin, the absence of α-catenin in the basal progenitor cells of the epidermis leads to reductions of AJs, loss of the cortical distribution of polarity determinants and randomized orientation of mitotic spindles.Citation24 In the epicardium, absence of β-catenin leads to a disruption of AJs and a randomization of mitotic spindle orientation.Citation61 These results suggest that AJs may play an active role in the regulation of oriented cell divisions promoting the occurrence of asymmetric cell divisions in certain tissue types. However, as opposed to Drosophila male germ cells, neuroblasts, and sensory organ cells, in Drosophila follicle cells mitotic spindles are not aligned with AJs and reductions on cadherins do not result in spindle misorientation.Citation62 A similar scenario was described in Drosophila imaginal discs and in Xenopus embryonic epithelia.Citation63,64 In mammals, absence of E-cadherin in mouse skin and mammary progenitor epithelial cells does not lead to an expansion of the stem cell compartment,Citation65-67 suggesting the involvement of additional regulatory mechanisms. Clearly, more insights about the role of cadherin-catenin proteins are needed to understand the extent to which AJs contribute as spatial cues in the regulation of centrosome and mitotic spindle positioning in different tissues and species.
Potential insights into cadherin-catenin mediated positioning of mitotic spindles: known interactions between AJs, centrosomes and microtubules
During cell division, AJs undergo a dynamic remodeling thereby allowing the regenerative process of epithelial tissues.Citation68,69 This has been better defined during cytokinesis and abscission of the 2 nascent daughter cells. In Drosophila, it has been observed that the levels of AJs are reduced at the cleavage furrow of dividing cells.Citation70-72 However, in mammalian epithelia such as MDCK cells, intestinal crypt cells, or basal progenitor keratinocytes AJs are maintained during this process.Citation73,74 It will be interesting to explore how this process is regulated in different cell types and tissues, and the implications of adhesion disengagement and the formation of new junctional contacts for the regenerative properties of progenitor cells. While the dynamic organization of AJs is modulated during cytokinesis, recent reports have unveiled a role for cadherin-catenin proteins in the position of the mitotic spindle and the organization of centrosomes. The potential mechanisms at hand may function through their interactions with the microtubules and centrosomes. Typically, microtubules nucleate from the centrosome and their dynamic growing plus-ends explore the periphery of the cell. In 1986, Kirschner and Mitchison proposed a “search and capture” model for the attachment of microtubules to cortical sites.Citation75 This model proposes that dynamic microtubules can be captured at specific sites, such as the membrane, the kinetochores or cell adhesion sites. In interphase cells, microtubules minus- and plus-ends interact with AJs through microtubule binding proteins,Citation76-78 including ACF7,Citation79 APC,Citation80,81 CLIP170,Citation81 Dynein,Citation82,83 CLASP2,Citation84,85 and the microtubule minus-end binding protein Nezha.Citation86 Whether all these connections between AJs and microtubule binding proteins take place during cell division awaits further investigation.
An interesting candidate is the microtubule motor Dynein. During mitosis it localizes to spindle poles and astral microtubules and generates the pulling force to orient the mitotic spindle; whereas in interphase it binds to β-catenin at AJs.Citation82 This has raised the possibility that β-catenin promotes the proper positioning of centrosomes and mitotic spindles by anchoring astral microtubules to the cell cortex. It has also been recently shown that β-catenin localizes to interphase centrosomes and spindle poles, where it promotes centrosome separation and spindle formation.Citation87-91 The underlying mechanism involves the interaction of β-catenin with NIMA-related protein kinase 2 (Nek2), a protein with critical roles in centrosome separation.Citation91 The mitotic protein polo-like kinase acts upstream of Nek2, which in turn phosphorylates β-catenin promoting its stabilization and preventing its degradation.Citation89
Another candidate to link AJs to centrosome and spindle positioning is the adenomatous polyposis coli protein (APC). APC binds to β-catenin as well as to the microtubule binding protein EB1.Citation92,93 APC localizes to kinetochores, centrosomes and cell adhesion sites.Citation94-96 Interestingly, in the budding yeast, Kar9 (the proposed functional homolog of APC) is required for the attachment of astral microtubules to the cortex to orient mitotic spindles.Citation97 In Drosophila neuroepithelial cells and germline stem cells, the orientation of the mitotic spindles is regulated in an APC dependent manner.Citation98,99 In addition, APC and β-catenin function in the canonical Wnt signaling pathway.Citation100 A link between Wnt signaling and spindle orientation was recently uncovered in mouse embryonic stem cells. Habib et al. (2013) observed that a localized Wnt signal leads to asymmetric spindle orientation, partitioning of cell fate determinants, asymmetric inheritance of β-catenin and differential fates of the 2 daughter cells.Citation101 It is tempting to speculate that in this context spindle orientation is controlled through the APC-β-catenin interaction, but the precise molecular mechanism remains to be explored.
It is well accepted that inactivating mutations of APC and stabilization of β-catenin lead to cancer.Citation102,103 Their emergent roles in spindle orientation and cell fate regulation add additional ways by which these proteins contribute to cancer progression. APC has also been linked to genetic instability.Citation94,95 The heterozygous loss of APC is sufficient to cause spindle misorientation in gastric tissues leading to a pre-tumorigenic state,Citation18,104 while inactivation of both APC alleles is required for carcinogenesis.Citation105
p120-catenin has also been found at centrosomes, associated to kinesin motors, the microtubule network and mitotic spindles.Citation106,107 At centrosomes, p120-catenin colocalizes and associates with cyclin E and cyclin-dependent kinase 2, key proteins involved in centrosome duplication during mitosis.Citation108 Interestingly, the transcription factor Kaiso, originally described as a binding partner of p120-catenin,Citation109 also localizes to centrosomes, mitotic spindles and the midbody,Citation110,111 and colocalizes with p120-catenin at spindle poles in HeLa cells.Citation110 Through these novel connections, and the roles of p120-catenin in the regulation of RhoGTPases and the cytoskeleton,Citation112,113 p120-catenin may potentially regulate centrosomal and mitotic spindle functions and integrity. Indeed decreased levels of p120-catenin in epidermal cells lead to alterations in centrosomes and spindles, mitotic defects and aneuplody in a cell autonomous manner.Citation114 In this context, it has been recently found that p120-catenin interacts with the microtubule binding protein CLASP2 at AJs in epidermal basal cells.Citation84 Whether p120-catenin interacts with CLASP2 at interphase or mitotic centrosomes awaits further investigation. It is tempting to speculate that given the pivotal roles of CLASP2 in mitosis,Citation115,116 a potential CLASP2-p120 interaction at centrosomes could have an impact on centrosome function and mitotic spindle position and integrity. Interestingly, it has been documented that CLASP1, another member of the family, cooperates to align the spindle along the long axis of division in mammalian cells.Citation117,118
Overall, these recent results point to another interesting facet of AJs, in which their proper levels of expression may also regulate cell division through centrosome and spindle organization.
Cadherin-catenins, actin cytoskeleton and spindle orientation
The dynamic anchoring of the mitotic spindle to the cell cortex by astral microtubules underlies most of the mechanisms that orient cell division relative to the shape of the cell or to cortical regulators.Citation42,119 To allow the alignment and separation of the mitotic spindles, 3 major elements partake: 1) cell cortical tension, 2) microtubule dynamics and 3) a restoring force preventing the collapse of the mitotic spindle on the cortex.Citation42,120 It was originally described in the budding yeast that disruption of either astral microtubules or actin function results in improper spindle orientation. These results sparked the notion that proper spindle orientation is maintained by directly or indirectly tethering astral microtubules to cortical actin to generate a pulling force on the spindle.Citation121 The involvement of cortical actin in the orientation of spindles has also been recently observed in mammalian tissues. For example, in mouse epidermal cells, the absence of the serum response transcription factor SRF, which induces actin polymerization and regulates the expression of several actin and actin binding proteins, leads to reductions in cortical actin, alterations in the distribution of LGN, NuMA and randomized positioning of mitotic spindles.Citation25
It is well established that cadherin-catenin proteins integrate cell-cell adhesion with cytoskeletal dynamics to establish and maintain tissue architecture. Reductions or alterations in the functional activity of cadherin-catenins lead to defects in the organization of both actin and microtubule cytoskeletal networks. Studies in mammalian cell lines indicate that the cadherin-mediated regulation of centrosome and spindle positioning requires both actin and microtubule cytoskeletons.Citation51,52 This suggests that cytoskeletal dynamics and forces are involved in centrosome and spindle positioning in a manner directly associated to AJs. α-catenin plays a central role in recruiting a number of proteins that link cadherin-catenin complexes to cortical actin, including vinculin, and the Arp2/3 complex actin nucleator.Citation122-125 The consequences of reduced α-catenin for the orientation of mitotic spindles are exemplified in the mouse epidermis, in which absence of α-catenin results in the loss of LGN localization to the cell cortex, random NuMA crescents and misoriented spindles.Citation24 This is also accompanied by reductions in intercellular adhesion, loss of epithelial architecture and hyperproliferation. Another interesting candidate that may be involved in spindle orientation is the Arp2/3 complex. It acts at nascent contacts upon homophilic cadherin binding and marks sites for actin assembly at the cell surface.Citation126 In cooperation with the nucleator factor WAVE,Citation127 it is also necessary for junctional integrity and contractile tension at AJs in a process coupled to myosin driven contractility.Citation128 Recently, it has been shown that loss of Arp2/3 activity in the epidermis leads to an increased proliferation of basal progenitor cells, alterations in terminal differentiation, disorganization of epidermal architecture and impaired Tight Junction assembly and function.Citation129,130 Future studies will determine whether spindle positioning is altered in the absence of the Arp2/3 complex.
Another interesting link between AJs, the actin cytoskeleton and oriented cell divisions in the epidermis is the interaction of α-catenin with the neurofibromatosis-2 (NF2) tumor suppressor gene Merlin, a member of the FERM (Four-point-one/Ezrin/Radixin/Moesin) domain family of proteins. It has been observed that Merlin directly links α-catenin with Par3, allowing the formation of a cortical actin ring and regulating cell polarity and the orientation of cell divisions within the basal epidermis.Citation131 Whether the observed defects triggered by the loss of α-catenin, Arp2/3 or other α-catenin associated proteins are a consequence of spindle misorientation awaits further investigation. However, given their roles in cytoskeletal organization, an interesting possibility is that they control spindle orientation by regulating tissue tension.
Emerging roles of cadherin mediated adhesion in growth control and differentiation: Possible links to cortical tension and mitotic spindle orientation
Cells have the ability to sense their physical environment and translate mechanical forces into intracellular signals that regulate cell behavior and tissue homeostasis.Citation132 These mechanical signals are able to influence a plethora of cellular responses that range from changes in cell shape, proliferation, migration to the acquisition of a different cell fate.Citation133 Alterations that perturb mechanosensing (the ability of a cell to sense its physical environment) may result in evasion of growth inhibitory signals and alterations in differentiation, potentially endowing cells with neoplastic characteristics.Citation134 Mechanical inputs are sensed by cells and translated by mechanosensors in an equivalent way to the ligand-receptor binding events that initiate signal transduction cascades and influence cell behavior.Citation135 The best studied mechanosensors are integrins at focal adhesions, which transduce physical cues via the actin cytoskeleton through a number of adaptor proteins, including vinculin.Citation136 Over past years, it is becoming increasingly clear that cadherin-catenin proteins are also able to respond to the mechanical environment through their connections with the actin cytoskeleton, allowing groups of cells to behave as a coordinated tissue.Citation2,137 Recently, several elegant studies have demonstrated that cadherins are bona-fide mechanosensors that transduce physical forces to the actin cytoskeleton.Citation138-140 These studies have unveiled how mechanical stresses trigger a conformational change in α-catenin at cadherin complexes allowing its direct interaction with the actin cytoskeletonCitation141 or with vinculin, which in turn associates directly with the actin cytoskeleton,Citation138,140 leading to the remodeling of AJs.Citation142
Is AJ mechanosensing activity a contributor to spindle orientation and what are the potential molecular players? Although this field is still in its infancy, an exciting possibility relates to the finding that AJs act as upstream regulators of the transcription factor Yap1. Yap1 is a major effector of the Hippo signaling pathway, which regulates growth, organ size and tumorigenesis.Citation143,144 Briefly, the activation of the Hippo pathway leads to Yap1 phosphorylation and its cytoplasmic retention. Conversely, the inactivation of Hippo signaling allows Yap1 to enter into the nucleus to activate transcription. Yap1 can also be activated by Hippo-independent mechanisms such as mechanical cues of the physical environment, including changes in cell shape, and tissue tension.Citation145-147 It is important to mention that it is not yet well understood if Hippo/Yap1 are directly involved in the regulation of spindle orientation. However, it has been observed that cell polarity regulators,Citation148–150 and the organization of the actin cytoskeleton influence the localization of Yap1.Citation151,152 Interestingly, loss of Yap1 has been associated to centrosomal and mitotic spindle defects, by a mechanism that involves cyclin-dependent kinase CDK1 mediated phosphorylation,Citation153 which is also important to regulate mitotic spindle bi-orientation.Citation154 Moreover, members of the Hippo pathway are important to orient the plane of cell division in certain tissues such as in the developing kidney.Citation155,156 In addition, upstream regulators of the Hippo/Yap pathway such as the tumor suppressor LKB1,Citation157,158 AMPKCitation159 and TAO1 kinaseCitation160,161 are involved in spindle orientation.
The connections between AJs and Hippo/Yap1 have been observed in several contexts. In Drosophila, the AJ associated protein Echinoid regulates the activation of the Hippo pathway and inhibits cell growth.Citation162 Additionally, the LIM protein Ajuba that associates to α-catenin and the actin cytoskeletonCitation163 has been recently identified as an inhibitor of the Hippo pathway.Citation164 In the context of cultured mammalian cells, it has been recently shown that E-cadherin ligation can directly activate the Hippo pathway independently of other types of interactions,Citation165 which strongly positions AJs as important regulators of the pathway. In terms of cell fate in mammalian organisms, one of the most interesting examples is the formation of the first 2 cell lineages of the mammalian embryo, the inner cell mass and the outer epithelial trophectoderm. During this process, the TJ protein Angiomontin is found localized at AJs in nonpolar inner cells of preimplantation embryos, where it activates the Hippo pathway preventing differentiation. In contrast, in outer epithelial cells Angiomontin localizes to the apical domain, suppressing Hippo signaling and activating the trophectoderm differentiation program.Citation166-168 In the context of expansion of stem cell pools, a link between Yap1 and α-catenin in the control of the proliferation of multipotent epidermal progenitor basal cells has been recently observed. Specifically, α-catenin inactivates Yap1 in the epidermis and controls epidermal proliferation in a cadherin independent manner.Citation169,170 Remarkably, the absence of α-catenin and an increase in nuclear Yap1 levels were found to be a feature of squamous cell carcinomas.Citation169 Thus, this positions α-catenin as an upstream regulator of the Hippo pathway effector Yap1 in skin.
Overall, the connection of AJs and cortical tension to the Hippo/Yap1 signaling pathway provides a mechanistic link to the regulation of cell fate decisions in response to architectural and mechanical cues. Whether these connections converge to potentially regulate oriented cell divisions in some tissues is still ill understood. Future research will shed light into this tantalizing hypothesis, and its implications for tumor formation and growth.
Conclusions and Future Directions
The molecular mechanisms regulated by cadherin-catenin molecules in epithelial tissues are not yet completely understood. Much remains to be done to understand how, through a plethora of different connections, AJs integrate and transmit signals to sustain cell physiology and tissue homeostasis. It is well established that cadherin dysfunction is a major contributor to cancer progression and transformation to malignancy.Citation9-12 However, the molecular mechanisms by which cadherin-catenin proteins regulate the cell proliferation and differentiation balance, beyond their canonical adhesive roles, continue expanding over the years. In this review, we aimed to summarize the emerging implications of cadherin-catenins in the regulation of polarized divisions through their connections with the centrosome, microtubule and actin cytoskeletons; and their implications in cell physiology, tissue function and neoplastic transformation.
Although the precise mechanistic events are still unclear, the emerging picture suggests that AJ levels are important regulators of cell fate in certain tissues and organisms, by maintaining cellular asymmetries and regulating stem cell spindle orientation (). Through their novel connections to the centrosome, β- and p120-catenin are emerging as regulators of the centrosomal cycle, fundamental for the proper inherence of centrosomes between daughter cells. These observations could explain how their loss leads to apoptosis (β-catenin deficiency) or genetic instability and aneuploidy (p120 deficiency), and increases the susceptibility to oncogenic transformation. In the context of polarized cell divisions, AJs can potentially regulate the orientation of cell divisions by anchoring astral microtubules to the cell cortex through their documented associations with microtubule binding proteins. Moreover, through their bona-fide roles as mechanosensors, AJs via α-catenin and its interaction with the actin cytoskeleton and actin binding proteins, integrate mechanical signals to possibly control cell fate decisions. This also includes the emerging role of AJs in the regulation of cell proliferation via the Hippo/Yap1 pathway. It will be interesting to learn in the future whether these events converge to coordinate the orientation of mitotic spindles and regulate polarized cell divisions in stem cell compartments. Future research will shed light on how the complex repertoire of molecular functions in which cadherin-catenins partake may synergistically contribute to the expansion of cancer stem cells pools in different ways in specific cells and tissues.
Figure 1. Links between Adherens Junctions, centrosomes and the cytoskeleton during interphase and cell division. (A) In interphase, classical cadherins mediate homophilic cell-cell adhesion through their extracellular domains. The cadherin cytoplasmic domain binds to β- and p120-catenin, while α-catenin binds to the complex via β-catenin. Cadherin-catenin complexes are linked to the actin and microtubule networks via actin- and microtubule- binding proteins. (B) During cell division, centrosomes duplicate and one of the centrosomes migrates to the other pole of the dividing cell forming the mitotic spindle (not shown), which is anchored to the cell cortex through the astral microtubules. Both β- and p120-catenin are found in centrosomes and at the cell cortex potentially promoting the proper positioning of centrosomes and mitotic spindles by anchoring astral microtubules to the cell cortex through their interaction with microtubule binding proteins. Moreover, through their mechanosensing functions, cadherin-catenin complexes may transmit the forces exerted on astral microtubules pulling the spindles toward their final position, leading to either symmetric or asymmetric divisions through their connections with the actin cytoskeleton and/or the Hippo effector Yap1. (C) A proper balance between symmetric and asymmetric cell divisions needs to be maintained in order to preserve tissue homeostasis. Loss of the expression or functional activity of cadherin-catenin proteins may perturb centrosome organization and the oriented positioning of mitotic spindles rendering cells susceptible to transformation. Microtubules and microtubule binding proteins are shown in blue; actin filaments and actin binding proteins are shown in green, and Adherens Junction proteins are highlighted in orange. Actin BP: actin binding proteins.
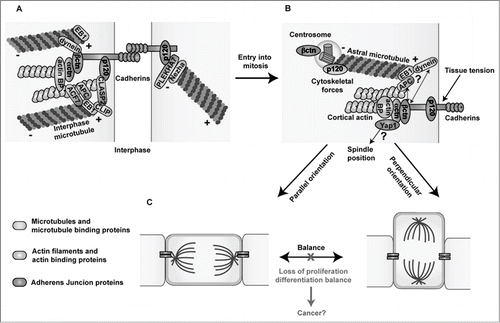
In summary, the connection of AJ proteins to centrosomes and mitotic spindles, their roles as mechanosensors and their connection with the Hippo/Yap1 signaling, are unraveling additional ways by which AJs preserve tissue homeostasis, and novel mechanisms by which their loss fosters tumor growth.
Disclosure of Potential Conflicts of Interest
No potential conflicts of interest were disclosed.
Funding
M.N.S. is a recipient of a Fundación Ramon Areces postdoctoral fellowship. M.P-M laboratory is funded by a grant from the Spanish Secretary of Science and Innovation (BFU2012–33910).
References
- Farquhar MG, Palade GE. Junctional complexes in various epithelia. J Cell Biol 1963; 17:375-412; PMID:13944428; http://dx.doi.org/10.1083/jcb.17.2.375
- Gumbiner BM. Regulation of cadherin-mediated adhesion in morphogenesis. Nat Rev Mol Cell Biol 2005; 6:622-34; PMID:16025097; http://dx.doi.org/10.1038/nrm1699
- Perez-Moreno M, Jamora C, Fuchs E. Sticky business: orchestrating cellular signals at adherens junctions. Cell 2003; 112:535-48; PMID:12600316; http://dx.doi.org/10.1016/S0092-8674(03)00108-9
- Pieters T, van Roy F. Role of cell-cell adhesion complexes in embryonic stem cell biology. J Cell Sci 2014; 127:2603-13; PMID:24931943; http://dx.doi.org/10.1242/jcs.146720
- Takeichi M. Cadherin cell adhesion receptors as a morphogenetic regulator. Science 1991; 251:1451-5; PMID:2006419; http://dx.doi.org/10.1126/science.2006419
- Brieher WM, Yap AS. Cadherin junctions and their cytoskeleton(s). Curr Opin Cell Biol 2013; 25:39-46; PMID:23127608; http://dx.doi.org/10.1016/j.ceb.2012.10.010
- Niessen CM, Leckband D, Yap AS. Tissue organization by cadherin adhesion molecules: dynamic molecular and cellular mechanisms of morphogenetic regulation. Physiol Rev 2011; 91:691-731; PMID:21527735; http://dx.doi.org/10.1152/physrev.00004.2010
- Perrais M, Chen X, Perez-Moreno M, Gumbiner BM. E-cadherin homophilic ligation inhibits cell growth and epidermal growth factor receptor signaling independently of other cell interactions. Mol Biol Cell 2007; 18:2013-25; PMID:17392517; http://dx.doi.org/10.1091/mbc.E06-04-0348
- Birchmeier W, Behrens J. Cadherin expression in carcinomas: role in the formation of cell junctions and the prevention of invasiveness. Biochim Biophys Acta 1994; 1198:11-26; PMID:8199193
- Berx G, Nollet F, van Roy F. Dysregulation of the E-cadherin/catenin complex by irreversible mutations in human carcinomas. Cell Adhes Commun 1998; 6:171-84; PMID:9823469; http://dx.doi.org/10.3109/15419069809004474
- Jeanes A, Gottardi CJ, Yap AS. Cadherins and cancer: how does cadherin dysfunction promote tumor progression? Oncogene 2008; 27:6920-9; PMID:19029934; http://dx.doi.org/10.1038/onc.2008.343
- Thiery JP, Acloque H, Huang RY, Nieto MA. Epithelial-mesenchymal transitions in development and disease. Cell 2009; 139:871-90; PMID:19945376; http://dx.doi.org/10.1016/j.cell.2009.11.007
- Muthuswamy SK, Xue B. Cell polarity as a regulator of cancer cell behavior plasticity. Annu Rev Cell Dev Biol 2012; 28:599-625; PMID:22881459; http://dx.doi.org/10.1146/annurev-cellbio-092910-154244
- Martin-Belmonte F, Perez-Moreno M. Epithelial cell polarity, stem cells and cancer. Nat Rev Cancer 2012; 12:23-38.
- Bornens M. Organelle positioning and cell polarity. Nat Rev Mol Cell Biol 2008; 9:874-86; PMID:18946476; http://dx.doi.org/10.1038/nrm2524
- Knoblich JA. Mechanisms of asymmetric stem cell division. Cell 2008; 132:583-97; PMID:18295577; http://dx.doi.org/10.1016/j.cell.2008.02.007
- Knoblich JA. Asymmetric cell division: recent developments and their implications for tumour biology. Nat Rev Mol Cell Biol 2010; 11:849-60; PMID:21102610; http://dx.doi.org/10.1038/nrm3010
- Quyn AJ, Appleton PL, Carey FA, Steele RJ, Barker N, Clevers H, Ridgway RA, Sansom OJ, Näthke IS. Spindle orientation bias in gut epithelial stem cell compartments is lost in precancerous tissue. Cell Stem Cell 2010; 6:175-81; PMID:20144789; http://dx.doi.org/10.1016/j.stem.2009.12.007
- De Mey JR, Freund JN. Understanding epithelial homeostasis in the intestine: An old battlefield of ideas, recent breakthroughs and remaining controversies. Tissue Barriers 2013; 1:e24965; PMID:24665395; http://dx.doi.org/10.4161/tisb.24965
- Cicalese A, Bonizzi G, Pasi CE, Faretta M, Ronzoni S, Giulini B, Brisken C, Minucci S, Di Fiore PP, Pelicci PG. The tumor suppressor p53 regulates polarity of self-renewing divisions in mammary stem cells. Cell 2009; 138:1083-95; PMID:19766563; http://dx.doi.org/10.1016/j.cell.2009.06.048
- Regan JL, Sourisseau T, Soady K, Kendrick H, McCarthy A, Tang C, Brennan K, Linardopoulos S, White DE, Smalley MJ. Aurora A kinase regulates mammary epithelial cell fate by determining mitotic spindle orientation in a Notch-dependent manner. Cell Rep 2013; 4:110-23; PMID:23810554; http://dx.doi.org/10.1016/j.celrep.2013.05.044
- El-Hashash AH, Turcatel G, Al Alam D, Buckley S, Tokumitsu H, Bellusci S, Warburton D. Eya1 controls cell polarity, spindle orientation, cell fate and Notch signaling in distal embryonic lung epithelium. Development 2011; 138:1395-407; PMID:21385765; http://dx.doi.org/10.1242/dev.058479
- El-Hashash AH, Warburton D. Cell polarity and spindle orientation in the distal epithelium of embryonic lung. Dev Dyn 2011; 240:441-5; PMID:21246661; http://dx.doi.org/10.1002/dvdy.22551
- Lechler T, Fuchs E. Asymmetric cell divisions promote stratification and differentiation of mammalian skin. Nature 2005; 437:275-80; PMID:16094321; http://dx.doi.org/10.1038/nature03922
- Luxenburg C, Pasolli HA, Williams SE, Fuchs E. Developmental roles for Srf, cortical cytoskeleton and cell shape in epidermal spindle orientation. Nat Cell Biol 2011; 13:203-14; PMID:21336301; http://dx.doi.org/10.1038/ncb2163
- Williams SE, Beronja S, Pasolli HA, Fuchs E. Asymmetric cell divisions promote Notch-dependent epidermal differentiation. Nature 2011; 470:353-8; PMID:21331036; http://dx.doi.org/10.1038/nature09793
- Niessen MT, Scott J, Zielinski JG, Vorhagen S, Sotiropoulou PA, Blanpain C, Leitges M, Niessen CM. aPKClambda controls epidermal homeostasis and stem cell fate through regulation of division orientation. J Cell Biol 2013; 202:887-900; PMID:24019538; http://dx.doi.org/10.1083/jcb.201307001
- Williams SE, Ratliff LA, Postiglione MP, Knoblich JA, Fuchs E. Par3-mInsc and Galphai3 cooperate to promote oriented epidermal cell divisions through LGN. Nat Cell Biol 2014; 16:758-69; PMID:25016959; http://dx.doi.org/10.1038/ncb3001
- Pease JC, Tirnauer JS. Mitotic spindle misorientation in cancer–out of alignment and into the fire. J Cell Sci 2011; 124:1007-16; PMID:21402874; http://dx.doi.org/10.1242/jcs.081406
- Pece S, Tosoni D, Confalonieri S, Mazzarol G, Vecchi M, Ronzoni S, Bernard L, Viale G, Pelicci PG, Di Fiore PP. Biological and molecular heterogeneity of breast cancers correlates with their cancer stem cell content. Cell 2010; 140:62-73; PMID:20074520; http://dx.doi.org/10.1016/j.cell.2009.12.007
- O'Brien CA, Kreso A, Ryan P, Hermans KG, Gibson L, Wang Y, Tsatsanis A, Gallinger S, Dick JE. ID1 and ID3 regulate the self-renewal capacity of human colon cancer-initiating cells through p21. Cancer Cell 2012; 21:777-92; PMID:22698403; http://dx.doi.org/10.1016/j.ccr.2012.04.036
- Schepers AG, Snippert HJ, Stange DE, van den Born M, van Es JH, van de Wetering M, Clevers H. Lineage tracing reveals Lgr5+ stem cell activity in mouse intestinal adenomas. Science 2012; 337:730-5; PMID:22855427; http://dx.doi.org/10.1126/science.1224676
- Pine SR, Ryan BM, Varticovski L, Robles AI, Harris CC. Microenvironmental modulation of asymmetric cell division in human lung cancer cells. Proc Natl Acad Sci U S A 2010; 107:2195-200; PMID:20080668; http://dx.doi.org/10.1073/pnas.0909390107
- Driessens G, Beck B, Caauwe A, Simons BD, Blanpain C. Defining the mode of tumour growth by clonal analysis. Nature 2012; 488:527-30; PMID:22854777; http://dx.doi.org/10.1038/nature11344
- Luders J, Stearns T. Microtubule-organizing centres: a re-evaluation. Nat Rev Mol Cell Biol 2007; 8:161-7; PMID:17245416; http://dx.doi.org/10.1038/nrm2100
- Bornens M. The centrosome in cells and organisms. Science 2012; 335:422-6; PMID:22282802; http://dx.doi.org/10.1126/science.1209037
- Tanaka TU. Kinetochore-microtubule interactions: steps towards bi-orientation. EMBO J 2010; 29:4070-82; PMID:21102558; http://dx.doi.org/10.1038/emboj.2010.294
- Yamashita YM. The centrosome and asymmetric cell division. Prion 2009; 3:84-8; PMID:19458491; http://dx.doi.org/10.4161/pri.3.2.8821
- Pelletier L, Yamashita YM. Centrosome asymmetry and inheritance during animal development. Curr Opin Cell Biol 2012; 24:541-6; PMID:22683192; http://dx.doi.org/10.1016/j.ceb.2012.05.005
- Malumbres M. Physiological relevance of cell cycle kinases. Physiol Rev 2011; 91:973-1007; PMID:21742793; http://dx.doi.org/10.1152/physrev.00025.2010
- Elric J, Etienne-Manneville S. Centrosome positioning in polarized cells: common themes and variations. Exp Cell Res 2014; 328:240-8; PMID:25218948; http://dx.doi.org/10.1016/j.yexcr.2014.09.004
- Morin X, Bellaiche Y. Mitotic spindle orientation in asymmetric and symmetric cell divisions during animal development. Dev Cell 2011; 21:102-19; PMID:21763612; http://dx.doi.org/10.1016/j.devcel.2011.06.012
- Siller KH, Doe CQ. Spindle orientation during asymmetric cell division. Nat Cell Biol 2009; 11:365-74; PMID:19337318; http://dx.doi.org/10.1038/ncb0409-365
- Williams SE, Fuchs E. Oriented divisions, fate decisions. Curr Opin Cell Biol 2013; 25:749-58; PMID:24021274; http://dx.doi.org/10.1016/j.ceb.2013.08.003
- Culurgioni S, Alfieri A, Pendolino V, Laddomada F, Mapelli M. Inscuteable and NuMA proteins bind competitively to Leu-Gly-Asn repeat-enriched protein (LGN) during asymmetric cell divisions. Proc Natl Acad Sci U S A 2011; 108:20998-1003; PMID:22171003; http://dx.doi.org/10.1073/pnas.1113077108
- Mapelli M, Gonzalez C. On the inscrutable role of Inscuteable: structural basis and functional implications for the competitive binding of NuMA and Inscuteable to LGN. Open Biol 2012; 2:120102; PMID:22977735; http://dx.doi.org/10.1098/rsob.120102
- Hao Y, Du Q, Chen X, Zheng Z, Balsbaugh JL, Maitra S, Shabanowitz J, Hunt DF, Macara IG. Par3 controls epithelial spindle orientation by aPKC-mediated phosphorylation of apical Pins. Curr Biol 2010; 20:1809-18; PMID:20933426; http://dx.doi.org/10.1016/j.cub.2010.09.032
- Zheng Z, Zhu H, Wan Q, Liu J, Xiao Z, Siderovski DP, Du Q. LGN regulates mitotic spindle orientation during epithelial morphogenesis. J Cell Biol 2010; 189:275-88; PMID:20385777; http://dx.doi.org/10.1083/jcb.200910021
- Peyre E, Jaouen F, Saadaoui M, Haren L, Merdes A, Durbec P, Morin X. A lateral belt of cortical LGN and NuMA guides mitotic spindle movements and planar division in neuroepithelial cells. J Cell Biol 2011; 193:141-54; PMID:21444683; http://dx.doi.org/10.1083/jcb.201101039
- Konno D, Shioi G, Shitamukai A, Mori A, Kiyonari H, Miyata T, Matsuzaki F. Neuroepithelial progenitors undergo LGN-dependent planar divisions to maintain self-renewability during mammalian neurogenesis. Nat Cell Biol 2008; 10:93-101; PMID:18084280; http://dx.doi.org/10.1038/ncb1673
- Desai RA, Gao L, Raghavan S, Liu WF, Chen CS. Cell polarity triggered by cell-cell adhesion via E-cadherin. J Cell Sci 2009; 122:905-11; PMID:19258396; http://dx.doi.org/10.1242/jcs.028183
- Dupin I, Camand E, Etienne-Manneville S. Classical cadherins control nucleus and centrosome position and cell polarity. J Cell Biol 2009; 185:779-86; PMID:19487453; http://dx.doi.org/10.1083/jcb.200812034
- den Elzen N, Buttery CV, Maddugoda MP, Ren G, Yap AS. Cadherin adhesion receptors orient the mitotic spindle during symmetric cell division in mammalian epithelia. Mol Biol Cell 2009; 20:3740-50; PMID:19553471; http://dx.doi.org/10.1091/mbc.E09-01-0023
- Song X, Zhu CH, Doan C, Xie T. Germline stem cells anchored by adherens junctions in the Drosophila ovary niches. Science 2002; 296:1855-7; PMID:12052957; http://dx.doi.org/10.1126/science.1069871
- Inaba M, Yuan H, Salzmann V, Fuller MT, Yamashita YM. E-cadherin is required for centrosome and spindle orientation in Drosophila male germline stem cells. PLoS One 2010; 5:e12473; PMID:20824213; http://dx.doi.org/10.1371/journal.pone.0012473
- Le Borgne R, Bellaiche Y, Schweisguth F. Drosophila E-cadherin regulates the orientation of asymmetric cell division in the sensory organ lineage. Curr Biol 2002; 12:95-104; PMID:11818059; http://dx.doi.org/10.1016/S0960-9822(01)00648-0
- Kim S, Ishidate T, Sharma R, Soto MC, Conte D, Jr., Mello CC, Shirayama M. Wnt and CDK-1 regulate cortical release of WRM-1/beta-catenin to control cell division orientation in early Caenorhabditis elegans embryos. Proc Natl Acad Sci U S A 2013; 110:E918-27; PMID:23431196; http://dx.doi.org/10.1073/pnas.1300769110
- Marthiens V, ffrench-Constant C. Adherens junction domains are split by asymmetric division of embryonic neural stem cells. EMBO Rep 2009; 10:515-20; PMID:19373255; http://dx.doi.org/10.1038/embor.2009.36
- Zhang J, Woodhead GJ, Swaminathan SK, Noles SR, McQuinn ER, Pisarek AJ, Stocker AM, Mutch CA, Funatsu N, Chenn A. Cortical neural precursors inhibit their own differentiation via N-cadherin maintenance of beta-catenin signaling. Dev Cell 2010; 18:472-9; PMID:20230753; http://dx.doi.org/10.1016/j.devcel.2009.12.025
- Humar B, Guilford P. Hereditary diffuse gastric cancer: a manifestation of lost cell polarity. Cancer Sci 2009; 100:1151-7; PMID:19432899; http://dx.doi.org/10.1111/j.1349-7006.2009.01163.x
- Wu M, Smith CL, Hall JA, Lee I, Luby-Phelps K, Tallquist MD. Epicardial spindle orientation controls cell entry into the myocardium. Dev Cell 2010; 19:114-25; PMID:20643355; http://dx.doi.org/10.1016/j.devcel.2010.06.011
- Bergstralh DT, Lovegrove HE, St Johnston D. Discs large links spindle orientation to apical-basal polarity in Drosophila epithelia. Curr Biol 2013; 23:1707-12; PMID:23891112; http://dx.doi.org/10.1016/j.cub.2013.07.017
- Woolner S, Papalopulu N. Spindle position in symmetric cell divisions during epiboly is controlled by opposing and dynamic apicobasal forces. Dev Cell 2012; 22:775-87; PMID:22406140; http://dx.doi.org/10.1016/j.devcel.2012.01.002
- Nakajima Y, Meyer EJ, Kroesen A, McKinney SA, Gibson MC. Epithelial junctions maintain tissue architecture by directing planar spindle orientation. Nature 2013; 500:359-62; PMID:23873041; http://dx.doi.org/10.1038/nature12335
- Tinkle CL, Lechler T, Pasolli HA, Fuchs E. Conditional targeting of E-cadherin in skin: insights into hyperproliferative and degenerative responses. Proc Natl Acad Sci U S A 2004; 101:552-7; PMID:14704278; http://dx.doi.org/10.1073/pnas.0307437100
- Tunggal JA, Helfrich I, Schmitz A, Schwarz H, Gunzel D, Fromm M, Kemler R, Krieg T, Niessen CM. E-cadherin is essential for in vivo epidermal barrier function by regulating tight junctions. EMBO J 2005; 24:1146-56; PMID:15775979; http://dx.doi.org/10.1038/sj.emboj.7600605
- Derksen PW, Liu X, Saridin F, van der Gulden H, Zevenhoven J, Evers B, van Beijnum JR, Griffioen AW, Vink J, Krimpenfort P, et al. Somatic inactivation of E-cadherin and p53 in mice leads to metastatic lobular mammary carcinoma through induction of anoikis resistance and angiogenesis. Cancer Cell 2006; 10:437-49; PMID:17097565; http://dx.doi.org/10.1016/j.ccr.2006.09.013
- Le Bras S, Le Borgne R. Epithelial cell division - multiplying without losing touch. J Cell Sci 2014; 127:5127-37; PMID:25344250; http://dx.doi.org/10.1242/jcs.151472
- Ragkousi K, Gibson MC. Cell division and the maintenance of epithelial order. J Cell Biol 2014; 207:181-8; PMID:25349258; http://dx.doi.org/10.1083/jcb.201408044
- Founounou N, Loyer N, Le Borgne R. Septins regulate the contractility of the actomyosin ring to enable adherens junction remodeling during cytokinesis of epithelial cells. Dev Cell 2013; 24:242-55; PMID:23410939; http://dx.doi.org/10.1016/j.devcel.2013.01.008
- Guillot C, Lecuit T. Adhesion disengagement uncouples intrinsic and extrinsic forces to drive cytokinesis in epithelial tissues. Dev Cell 2013; 24:227-41; PMID:23410938; http://dx.doi.org/10.1016/j.devcel.2013.01.010
- Herszterg S, Leibfried A, Bosveld F, Martin C, Bellaiche Y. Interplay between the dividing cell and its neighbors regulates adherens junction formation during cytokinesis in epithelial tissue. Dev Cell 2013; 24:256-70; PMID:23410940; http://dx.doi.org/10.1016/j.devcel.2012.11.019
- Baker J, Garrod D. Epithelial cells retain junctions during mitosis. J Cell Sci 1993; 104 (Pt 2):415-25; PMID:7685036
- Reinsch S, Karsenti E. Orientation of spindle axis and distribution of plasma membrane proteins during cell division in polarized MDCKII cells. J Cell Biol 1994; 126:1509-26; PMID:8089182; http://dx.doi.org/10.1083/jcb.126.6.1509
- Kirschner MW, Mitchison T. Microtubule dynamics. Nature 1986; 324:621; PMID:3796729; http://dx.doi.org/10.1038/324621a0
- Stehbens SJ, Akhmanova A, Yap AS. Microtubules and cadherins: a neglected partnership. Front Biosci (Landmark Ed) 2009; 14:3159-67; PMID:19273264; http://dx.doi.org/10.2741/3442
- Akhmanova A, Stehbens SJ, Yap AS. Touch, grasp, deliver and control: functional cross-talk between microtubules and cell adhesions. Traffic 2009; 10:268-74; PMID:19175539; http://dx.doi.org/10.1111/j.1600-0854.2008.00869.x
- Bellett G, Carter JM, Keynton J, Goldspink D, James C, Moss DK, Mogensen MM. Microtubule plus-end and minus-end capture at adherens junctions is involved in the assembly of apico-basal arrays in polarised epithelial cells. Cell Motil Cytoskeleton 2009; 66:893-908; PMID:19479825; http://dx.doi.org/10.1002/cm.20393
- Karakesisoglou I, Yang Y, Fuchs E. An epidermal plakin that integrates actin and microtubule networks at cellular junctions. J Cell Biol 2000; 149:195-208; PMID:10747097; http://dx.doi.org/10.1083/jcb.149.1.195
- Mogensen MM, Tucker JB, Mackie JB, Prescott AR, Nathke IS. The adenomatous polyposis coli protein unambiguously localizes to microtubule plus ends and is involved in establishing parallel arrays of microtubule bundles in highly polarized epithelial cells. J Cell Biol 2002; 157:1041-8; PMID:12058019; http://dx.doi.org/10.1083/jcb.200203001
- Bienz M. Spindles cotton on to junctions, APC and EB1. Nat Cell Biol 2001; 3:E67-8; PMID:11231588; http://dx.doi.org/10.1038/35060140
- Ligon LA, Karki S, Tokito M, Holzbaur EL. Dynein binds to beta-catenin and may tether microtubules at adherens junctions. Nat Cell Biol 2001; 3:913-7; PMID:11584273; http://dx.doi.org/10.1038/ncb1001-913
- Ligon LA, Holzbaur EL. Microtubules tethered at epithelial cell junctions by dynein facilitate efficient junction assembly. Traffic 2007; 8:808-19; PMID:17550375; http://dx.doi.org/10.1111/j.1600-0854.2007.00574.x
- Shahbazi MN, Megias D, Epifano C, Akhmanova A, Gundersen GG, Fuchs E, Perez-Moreno M. CLASP2 interacts with p120-catenin and governs microtubule dynamics at adherens junctions. J Cell Biol 2013; 203:1043-61; PMID:24368809; http://dx.doi.org/10.1083/jcb.201306019
- Shahbazi MN, Perez-Moreno M. Microtubules CLASP to Adherens Junctions in epidermal progenitor cells. Bioarchitecture 2014; 4:25-30; PMID:24522006; http://dx.doi.org/10.4161/bioa.28177
- Meng W, Mushika Y, Ichii T, Takeichi M. Anchorage of microtubule minus ends to adherens junctions regulates epithelial cell-cell contacts. Cell 2008; 135:948-59; PMID:19041755; http://dx.doi.org/10.1016/j.cell.2008.09.040
- Kaplan DD, Meigs TE, Kelly P, Casey PJ. Identification of a role for beta-catenin in the establishment of a bipolar mitotic spindle. J Biol Chem 2004; 279:10829-32; PMID:14744872; http://dx.doi.org/10.1074/jbc.C400035200
- Mbom BC, Nelson WJ, Barth A. beta-catenin at the centrosome: discrete pools of beta-catenin communicate during mitosis and may co-ordinate centrosome functions and cell cycle progression. Bioessays 2013; 35:804-9; PMID:23804296; http://dx.doi.org/10.1002/bies.201300045
- Mbom BC, Siemers KA, Ostrowski MA, Nelson WJ, Barth AI. Nek2 phosphorylates and stabilizes beta-catenin at mitotic centrosomes downstream of Plk1. Mol Biol Cell 2014; 25:977-91; PMID:24501426; http://dx.doi.org/10.1091/mbc.E13-06-0349
- Chilov D, Sinjushina N, Rita H, Taketo MM, Makela TP, Partanen J. Phosphorylated beta-catenin localizes to centrosomes of neuronal progenitors and is required for cell polarity and neurogenesis in developing midbrain. Dev Biol 2011; 357:259-68; PMID:21736876; http://dx.doi.org/10.1016/j.ydbio.2011.06.029
- Bahmanyar S, Kaplan DD, Deluca JG, Giddings TH, Jr., O'Toole ET, Winey M, Salmon ED, Casey PJ, Nelson WJ, Barth AI. beta-Catenin is a Nek2 substrate involved in centrosome separation. Genes Dev 2008; 22:91-105; PMID:18086858; http://dx.doi.org/10.1101/gad.1596308
- Berrueta L, Kraeft SK, Tirnauer JS, Schuyler SC, Chen LB, Hill DE, Pellman D, Bierer BE. The adenomatous polyposis coli-binding protein EB1 is associated with cytoplasmic and spindle microtubules. Proc Natl Acad Sci U S A 1998; 95:10596-601; PMID:9724749; http://dx.doi.org/10.1073/pnas.95.18.10596
- Askham JM, Vaughan KT, Goodson HV, Morrison EE. Evidence that an interaction between EB1 and p150(Glued) is required for the formation and maintenance of a radial microtubule array anchored at the centrosome. Mol Biol Cell 2002; 13:3627-45; PMID:12388762; http://dx.doi.org/10.1091/mbc.E02-01-0061
- Fodde R, Kuipers J, Rosenberg C, Smits R, Kielman M, Gaspar C, van Es JH, Breukel C, Wiegant J, Giles RH, et al. Mutations in the APC tumour suppressor gene cause chromosomal instability. Nat Cell Biol 2001; 3:433-8; PMID:11283620; http://dx.doi.org/10.1038/35070129
- Kaplan KB, Burds AA, Swedlow JR, Bekir SS, Sorger PK, Nathke IS. A role for the Adenomatous Polyposis Coli protein in chromosome segregation. Nat Cell Biol 2001; 3:429-32; PMID:11283619; http://dx.doi.org/10.1038/35070123
- McCartney BM, Dierick HA, Kirkpatrick C, Moline MM, Baas A, Peifer M, Bejsovec A. Drosophila APC2 is a cytoskeletally-associated protein that regulates wingless signaling in the embryonic epidermis. J Cell Biol 1999; 146:1303-18; PMID:10491393; http://dx.doi.org/10.1083/jcb.146.6.1303
- Miller RK, Rose MD. Kar9p is a novel cortical protein required for cytoplasmic microtubule orientation in yeast. J Cell Biol 1998; 140:377-90; PMID:9442113; http://dx.doi.org/10.1083/jcb.140.2.377
- Lu B, Roegiers F, Jan LY, Jan YN. Adherens junctions inhibit asymmetric division in the Drosophila epithelium. Nature 2001; 409:522-5; PMID:11206549; http://dx.doi.org/10.1038/35054077
- Yamashita YM, Jones DL, Fuller MT. Orientation of asymmetric stem cell division by the APC tumor suppressor and centrosome. Science 2003; 301:1547-50; PMID:12970569; http://dx.doi.org/10.1126/science.1087795
- Clevers H, Loh KM, Nusse R. Stem cell signaling. An integral program for tissue renewal and regeneration: Wnt signaling and stem cell control. Science 2014; 346:1248012; PMID:25278615; http://dx.doi.org/10.1126/science.1248012
- Habib SJ, Chen BC, Tsai FC, Anastassiadis K, Meyer T, Betzig E, Nusse R. A localized Wnt signal orients asymmetric stem cell division in vitro. Science 2013; 339:1445-8; PMID:23520113; http://dx.doi.org/10.1126/science.1231077
- Nelson WJ, Nusse R. Convergence of Wnt, beta-catenin, and cadherin pathways. Science 2004; 303:1483-7; PMID:15001769; http://dx.doi.org/10.1126/science.1094291
- Clevers H. Wnt/beta-catenin signaling in development and disease. Cell 2006; 127:469-80; PMID:17081971; http://dx.doi.org/10.1016/j.cell.2006.10.018
- Fleming ES, Temchin M, Wu Q, Maggio-Price L, Tirnauer JS. Spindle misorientation in tumors from APC(min/+) mice. Mol Carcinog 2009; 48:592-8; PMID:19123231; http://dx.doi.org/10.1002/mc.20506
- Reya T, Clevers H. Wnt signalling in stem cells and cancer. Nature 2005; 434:843-50; PMID:15829953; http://dx.doi.org/10.1038/nature03319
- Franz CM, Ridley AJ. p120 catenin associates with microtubules: inverse relationship between microtubule binding and Rho GTPase regulation. J Biol Chem 2004; 279:6588-94; PMID:14660598; http://dx.doi.org/10.1074/jbc.M312812200
- Yanagisawa M, Kaverina IN, Wang A, Fujita Y, Reynolds AB, Anastasiadis PZ. A novel interaction between kinesin and p120 modulates p120 localization and function. J Biol Chem 2004; 279:9512-21; PMID:14676216; http://dx.doi.org/10.1074/jbc.M310895200
- Chartier NT, Oddou CI, Laine MG, Ducarouge B, Marie CA, Block MR, Jacquier-Sarlin MR. Cyclin-dependent kinase 2/cyclin E complex is involved in p120 catenin (p120ctn)-dependent cell growth control: a new role for p120ctn in cancer. Cancer Res 2007; 67:9781-90; PMID:17942908; http://dx.doi.org/10.1158/0008-5472.CAN-07-0233
- Daniel JM, Reynolds AB. The catenin p120(ctn) interacts with Kaiso, a novel BTB/POZ domain zinc finger transcription factor. Mol Cell Biol 1999; 19:3614-23; PMID:10207085
- Kantidze OL, Kamalyukova IM, Razin SV. Association of the mammalian transcriptional regulator kaiso with centrosomes and the midbody. Cell Cycle 2009; 8:2303-4; PMID:19502788; http://dx.doi.org/10.4161/cc.8.14.8944
- Soubry A, Staes K, Parthoens E, Noppen S, Stove C, Bogaert P, van Hengel J, van Roy F. The transcriptional repressor Kaiso localizes at the mitotic spindle and is a constituent of the pericentriolar material. PLoS One 2010; 5:e9203; PMID:20169156; http://dx.doi.org/10.1371/journal.pone.0009203
- Anastasiadis PZ, Moon SY, Thoreson MA, Mariner DJ, Crawford HC, Zheng Y, Reynolds AB. Inhibition of RhoA by p120 catenin. Nat Cell Biol 2000; 2:637-44; PMID:10980705; http://dx.doi.org/10.1038/35023588
- Anastasiadis PZ, Reynolds AB. Regulation of Rho GTPases by p120-catenin. Curr Opin Cell Biol 2001; 13:604-10; PMID:11544030; http://dx.doi.org/10.1016/S0955-0674(00)00258-1
- Perez-Moreno M, Song W, Pasolli HA, Williams SE, Fuchs E. Loss of p120 catenin and links to mitotic alterations, inflammation, and skin cancer. Proc Natl Acad Sci U S A 2008; 105:15399-404; PMID:18809907; http://dx.doi.org/10.1073/pnas.0807301105
- Pereira AL, Pereira AJ, Maia AR, Drabek K, Sayas CL, Hergert PJ, Lince-Faria M, Matos I, Duque C, Stepanova T, et al. Mammalian CLASP1 and CLASP2 cooperate to ensure mitotic fidelity by regulating spindle and kinetochore function. Mol Biol Cell 2006; 17:4526-42; PMID:16914514; http://dx.doi.org/10.1091/mbc.E06-07-0579
- Logarinho E, Maffini S, Barisic M, Marques A, Toso A, Meraldi P, Maiato H. CLASPs prevent irreversible multipolarity by ensuring spindle-pole resistance to traction forces during chromosome alignment. Nat Cell Biol 2012; 14:295-303; PMID:22307330; http://dx.doi.org/10.1038/ncb2423
- Samora CP, Mogessie B, Conway L, Ross JL, Straube A, McAinsh AD. MAP4 and CLASP1 operate as a safety mechanism to maintain a stable spindle position in mitosis. Nat Cell Biol 2011; 13:1040-50; PMID:21822276; http://dx.doi.org/10.1038/ncb2297
- Bird SL, Heald R, Weis K. RanGTP and CLASP1 cooperate to position the mitotic spindle. Mol Biol Cell 2013; 24:2506-14; PMID:23783028; http://dx.doi.org/10.1091/mbc.E13-03-0150
- Thery M, Bornens M. Cell shape and cell division. Curr Opin Cell Biol 2006; 18:648-57; PMID:17046223; http://dx.doi.org/10.1016/j.ceb.2006.10.001
- Grill SW, Hyman AA. Spindle positioning by cortical pulling forces. Dev Cell 2005; 8:461-5; PMID:15809029; http://dx.doi.org/10.1016/j.devcel.2005.03.014
- Palmer RE, Sullivan DS, Huffaker T, Koshland D. Role of astral microtubules and actin in spindle orientation and migration in the budding yeast, Saccharomyces cerevisiae. J Cell Biol 1992; 119:583-93; PMID:1400594; http://dx.doi.org/10.1083/jcb.119.3.583
- Vasioukhin V, Bauer C, Yin M, Fuchs E. Directed actin polymerization is the driving force for epithelial cell-cell adhesion. Cell 2000; 100:209-19; PMID:10660044; http://dx.doi.org/10.1016/S0092-8674(00)81559-7
- Adams CL, Chen YT, Smith SJ, Nelson WJ. Mechanisms of epithelial cell-cell adhesion and cell compaction revealed by high-resolution tracking of E-cadherin-green fluorescent protein. J Cell Biol 1998; 142:1105-19; PMID:9722621; http://dx.doi.org/10.1083/jcb.142.4.1105
- Perez-Moreno M, Fuchs E. Catenins: keeping cells from getting their signals crossed. Dev Cell 2006; 11:601-12; PMID:17084354; http://dx.doi.org/10.1016/j.devcel.2006.10.010
- Scott JA, Yap AS. Cinderella no longer: alpha-catenin steps out of cadherin's shadow. J Cell Sci 2006; 119:4599-605; PMID:17093264; http://dx.doi.org/10.1242/jcs.03267
- Kovacs EM, Goodwin M, Ali RG, Paterson AD, Yap AS. Cadherin-directed actin assembly: E-cadherin physically associates with the Arp2/3 complex to direct actin assembly in nascent adhesive contacts. Curr Biol 2002; 12:379-82; PMID:11882288; http://dx.doi.org/10.1016/S0960-9822(02)00661-9
- Padrick SB, Rosen MK. Physical mechanisms of signal integration by WASP family proteins. Annu Rev Biochem 2010; 79:707-35; PMID:20533885; http://dx.doi.org/10.1146/annurev.biochem.77.060407.135452
- Verma S, Han SP, Michael M, Gomez GA, Yang Z, Teasdale RD, Ratheesh A, Kovacs EM, Ali RG, Yap AS. A WAVE2-Arp2/3 actin nucleator apparatus supports junctional tension at the epithelial zonula adherens. Mol Biol Cell 2012; 23:4601-10; PMID:23051739; http://dx.doi.org/10.1091/mbc.E12-08-0574
- Zhou K, Muroyama A, Underwood J, Leylek R, Ray S, Soderling SH, Lechler T. Actin-related protein2/3 complex regulates tight junctions and terminal differentiation to promote epidermal barrier formation. Proc Natl Acad Sci U S A 2013; 110:E3820-9; PMID:24043783; http://dx.doi.org/10.1073/pnas.1308419110
- Lechler T. Arp2/3 complex function in the epidermis. Tissue Barriers 2014; 2:e944445; PMID:25610753
- Gladden AB, Hebert AM, Schneeberger EE, McClatchey AI. The NF2 tumor suppressor, Merlin, regulates epidermal development through the establishment of a junctional polarity complex. Dev Cell 2010; 19:727-39; PMID:21074722; http://dx.doi.org/10.1016/j.devcel.2010.10.008
- Ingber DE. The riddle of morphogenesis: a question of solution chemistry or molecular cell engineering? Cell 1993; 75:1249-52; PMID:8269508; http://dx.doi.org/10.1016/0092-8674(93)90612-T
- Wang N, Tytell JD, Ingber DE. Mechanotransduction at a distance: mechanically coupling the extracellular matrix with the nucleus. Nat Rev Mol Cell Biol 2009; 10:75-82; PMID:19197334; http://dx.doi.org/10.1038/nrm2594
- Jaalouk DE, Lammerding J. Mechanotransduction gone awry. Nat Rev Mol Cell Biol 2009; 10:63-73; PMID:19197333; http://dx.doi.org/10.1038/nrm2597
- DuFort CC, Paszek MJ, Weaver VM. Balancing forces: architectural control of mechanotransduction. Nat Rev Mol Cell Biol 2011; 12:308-19; PMID:21508987; http://dx.doi.org/10.1038/nrm3112
- Humphrey JD, Dufresne ER, Schwartz MA. Mechanotransduction and extracellular matrix homeostasis. Nat Rev Mol Cell Biol 2014; 15:802-12; PMID:25355505; http://dx.doi.org/10.1038/nrm3896
- Harris TJ, Tepass U. Adherens junctions: from molecules to morphogenesis. Nat Rev Mol Cell Biol 2010; 11:502-14; PMID:20571587; http://dx.doi.org/10.1038/nrm2927
- le Duc Q, Shi Q, Blonk I, Sonnenberg A, Wang N, Leckband D, de Rooij J. Vinculin potentiates E-cadherin mechanosensing and is recruited to actin-anchored sites within adherens junctions in a myosin II-dependent manner. J Cell Biol 2010; 189:1107-15; PMID:20584916; http://dx.doi.org/10.1083/jcb.201001149
- Leckband DE, de Rooij J. Cadherin adhesion and mechanotransduction. Annu Rev Cell Dev Biol 2014; 30:291-315; PMID:25062360; http://dx.doi.org/10.1146/annurev-cellbio-100913-013212
- Yonemura S, Wada Y, Watanabe T, Nagafuchi A, Shibata M. alpha-Catenin as a tension transducer that induces adherens junction development. Nat Cell Biol 2010; 12:533-42; PMID:20453849; http://dx.doi.org/10.1038/ncb2055
- Buckley CD, Tan J, Anderson KL, Hanein D, Volkmann N, Weis WI, Nelson WJ, Dunn AR. Cell adhesion. The minimal cadherin-catenin complex binds to actin filaments under force. Science 2014; 346:1254211; PMID:25359979; http://dx.doi.org/10.1126/science.1254211
- Huveneers S, Oldenburg J, Spanjaard E, van der Krogt G, Grigoriev I, Akhmanova A, Rehmann H, de Rooij J. Vinculin associates with endothelial VE-cadherin junctions to control force-dependent remodeling. J Cell Biol 2012; 196:641-52; PMID:22391038; http://dx.doi.org/10.1083/jcb.201108120
- Gumbiner BM, Kim NG. The Hippo-YAP signaling pathway and contact inhibition of growth. J Cell Sci 2014; 127:709-17; PMID:24532814; http://dx.doi.org/10.1242/jcs.140103
- Pan D. The hippo signaling pathway in development and cancer. Dev Cell 2010; 19:491-505; PMID:20951342; http://dx.doi.org/10.1016/j.devcel.2010.09.011
- Halder G, Dupont S, Piccolo S. Transduction of mechanical and cytoskeletal cues by YAP and TAZ. Nat Rev Mol Cell Biol 2012; 13:591-600; PMID:22895435; http://dx.doi.org/10.1038/nrm3416
- Aragona M, Panciera T, Manfrin A, Giulitti S, Michielin F, Elvassore N, Dupont S, Piccolo S. A mechanical checkpoint controls multicellular growth through YAP/TAZ regulation by actin-processing factors. Cell 2013; 154:1047-59; PMID:23954413; http://dx.doi.org/10.1016/j.cell.2013.07.042
- Dupont S, Morsut L, Aragona M, Enzo E, Giulitti S, Cordenonsi M, Zanconato F, Le Digabel J, Forcato M, Bicciato S, et al. Role of YAP/TAZ in mechanotransduction. Nature 2011; 474:179-83; PMID:21654799; http://dx.doi.org/10.1038/nature10137
- Tepass U. The apical polarity protein network in Drosophila epithelial cells: regulation of polarity, junctions, morphogenesis, cell growth, and survival. Annu Rev Cell Dev Biol 2012; 28:655-85; PMID:22881460; http://dx.doi.org/10.1146/annurev-cellbio-092910-154033
- Boggiano JC, Fehon RG. Growth control by committee: intercellular junctions, cell polarity, and the cytoskeleton regulate Hippo signaling. Dev Cell 2012; 22:695-702; PMID:22516196; http://dx.doi.org/10.1016/j.devcel.2012.03.013
- Grusche FA, Richardson HE, Harvey KF. Upstream regulation of the hippo size control pathway. Curr Biol 2010; 20:R574-82; PMID:20619814; http://dx.doi.org/10.1016/j.cub.2010.05.023
- Gaspar P, Tapon N. Sensing the local environment: actin architecture and Hippo signalling. Curr Opin Cell Biol 2014; 31:74-83; PMID:25259681; http://dx.doi.org/10.1016/j.ceb.2014.09.003
- Wada K, Itoga K, Okano T, Yonemura S, Sasaki H. Hippo pathway regulation by cell morphology and stress fibers. Development 2011; 138:3907-14; PMID:21831922; http://dx.doi.org/10.1242/dev.070987
- Yang S, Zhang L, Liu M, Chong R, Ding SJ, Chen Y, Dong J. CDK1 phosphorylation of YAP promotes mitotic defects and cell motility and is essential for neoplastic transformation. Cancer Res 2013; 73:6722-33; PMID:24101154; http://dx.doi.org/10.1158/0008-5472.CAN-13-2049
- Tsukahara T, Tanno Y, Watanabe Y. Phosphorylation of the CPC by Cdk1 promotes chromosome bi-orientation. Nature 2010; 467:719-23; PMID:20739936; http://dx.doi.org/10.1038/nature09390
- Saburi S, Hester I, Fischer E, Pontoglio M, Eremina V, Gessler M, Quaggin SE, Harrison R, Mount R, McNeill H. Loss of Fat4 disrupts PCP signaling and oriented cell division and leads to cystic kidney disease. Nat Genet 2008; 40:1010-5; PMID:18604206; http://dx.doi.org/10.1038/ng.179
- Mao Y, Tournier AL, Bates PA, Gale JE, Tapon N, Thompson BJ. Planar polarization of the atypical myosin Dachs orients cell divisions in Drosophila. Genes Dev 2011; 25:131-6; PMID:21245166; http://dx.doi.org/10.1101/gad.610511
- Wei C, Bhattaram VK, Igwe JC, Fleming E, Tirnauer JS. The LKB1 tumor suppressor controls spindle orientation and localization of activated AMPK in mitotic epithelial cells. PLoS One 2012; 7:e41118; PMID:22815934; http://dx.doi.org/10.1371/journal.pone.0041118
- Mohseni M, Sun J, Lau A, Curtis S, Goldsmith J, Fox VL, Wei C, Frazier M, Samson O, Wong KK, et al. A genetic screen identifies an LKB1-MARK signalling axis controlling the Hippo-YAP pathway. Nat Cell Biol 2014; 16:108-17; PMID:24362629; http://dx.doi.org/10.1038/ncb2884
- Thaiparambil JT, Eggers CM, Marcus AI. AMPK regulates mitotic spindle orientation through phosphorylation of myosin regulatory light chain. Mol Cell Biol 2012; 32:3203-17; PMID:22688514; http://dx.doi.org/10.1128/MCB.00418-12
- Poon CL, Lin JI, Zhang X, Harvey KF. The sterile 20-like kinase Tao-1 controls tissue growth by regulating the Salvador-Warts-Hippo pathway. Dev Cell 2011; 21:896-906; PMID:22075148; http://dx.doi.org/10.1016/j.devcel.2011.09.012
- Wojtala RL, Tavares IA, Morton PE, Valderrama F, Thomas NS, Morris JD. Prostate-derived sterile 20-like kinases (PSKs/TAOKs) are activated in mitosis and contribute to mitotic cell rounding and spindle positioning. J Biol Chem 2011; 286:30161-70; PMID:21705329; http://dx.doi.org/10.1074/jbc.M111.228320
- Yue T, Tian A, Jiang J. The cell adhesion molecule echinoid functions as a tumor suppressor and upstream regulator of the Hippo signaling pathway. Dev Cell 2012; 22:255-67; PMID:22280890; http://dx.doi.org/10.1016/j.devcel.2011.12.011
- Marie H, Pratt SJ, Betson M, Epple H, Kittler JT, Meek L, Moss SJ, Troyanovsky S, Attwell D, Longmore GD, et al. The LIM protein Ajuba is recruited to cadherin-dependent cell junctions through an association with alpha-catenin. J Biol Chem 2003; 278:1220-8; PMID:12417594; http://dx.doi.org/10.1074/jbc.M205391200
- Das Thakur M, Feng Y, Jagannathan R, Seppa MJ, Skeath JB, Longmore GD. Ajuba LIM proteins are negative regulators of the Hippo signaling pathway. Curr Biol 2010; 20:657-62; PMID:20303269; http://dx.doi.org/10.1016/j.cub.2010.02.035
- Kim NG, Koh E, Chen X, Gumbiner BM. E-cadherin mediates contact inhibition of proliferation through Hippo signaling-pathway components. Proc Natl Acad Sci U S A 2011; 108:11930-5; PMID:21730131; http://dx.doi.org/10.1073/pnas.1103345108
- Leung CY, Zernicka-Goetz M. Angiomotin prevents pluripotent lineage differentiation in mouse embryos via Hippo pathway-dependent and -independent mechanisms. Nat Commun 2013; 4:2251; PMID:23903990; http://dx.doi.org/10.1038/ncomms3251
- Hirate Y, Hirahara S, Inoue K, Suzuki A, Alarcon VB, Akimoto K, Hirai T, Hara T, Adachi M, Chida K, et al. Polarity-dependent distribution of angiomotin localizes Hippo signaling in preimplantation embryos. Curr Biol 2013; 23:1181-94; PMID:23791731; http://dx.doi.org/10.1016/j.cub.2013.05.014
- Hirate Y, Sasaki H. The role of angiomotin phosphorylation in the Hippo pathway during preimplantation mouse development. Tissue Barriers 2014; 2:e28127; PMID:24843842; http://dx.doi.org/10.4161/tisb.28127
- Schlegelmilch K, Mohseni M, Kirak O, Pruszak J, Rodriguez JR, Zhou D, Kreger BT, Vasioukhin V, Avruch J, Brummelkamp TR, et al. Yap1 acts downstream of alpha-catenin to control epidermal proliferation. Cell 2011; 144:782-95; PMID:21376238; http://dx.doi.org/10.1016/j.cell.2011.02.031
- Silvis MR, Kreger BT, Lien WH, Klezovitch O, Rudakova GM, Camargo FD, Lantz DM, Seykora JT, Vasioukhin V. alpha-catenin is a tumor suppressor that controls cell accumulation by regulating the localization and activity of the transcriptional coactivator Yap1. Sci Signal 2011; 4:ra33; PMID:21610251; http://dx.doi.org/10.1126/scisignal.2001823