ABSTRACT
Curcumin has anti-inflammatory, anti-oxidant and anti-proliferative properties established largely by in vitro studies. Accordingly, oral administration of curcumin beneficially modulates many diseases including diabetes, fatty-liver disease, atherosclerosis, arthritis, cancer and neurological disorders such as depression, Alzheimer's or Parkinson's disease. However, limited bioavailability and inability to detect curcumin in circulation or target tissues has hindered the validation of a causal role. We established curcumin-mediated decrease in the release of gut bacteria-derived lipopolysaccharide (LPS) into circulation by maintaining the integrity of the intestinal barrier function as the mechanism underlying the attenuation of metabolic diseases (diabetes, atherosclerosis, kidney disease) by curcumin supplementation precluding the need for curcumin absorption. In view of the causative role of circulating LPS and resulting chronic inflammation in the development of diseases listed above, this review summarizes the mechanism by which curcumin affects the several layers of the intestinal barrier and, despite negligible absorption, can beneficially modulate these diseases.
Introduction
Curcumin is regarded as a natural anti-inflammatory, anti-oxidant and anti-neoplastic agent and has been used for a very long time in Indian and Chinese medicine. However, due to poor bioavailability of orally administered curcumin, there is skepticism regarding its causal role. Due to negligible levels of curcumin in circulation and significantly lower concentrations at target cell types than used in cell culture studies to demonstrate its direct effects, considerable efforts are currently being directed towards increasing the absorption of curcumin. In this review, we provide insights into a novel and alternate mechanism of action for curcumin. Increased levels of circulating bacterial lipopolysaccharide or LPS are causally linked to the development of diet-induced metabolic diseases (diabetes and atherosclerosis), chronic inflammatory diseases (chronic kidney disease, arthritis), several neurological diseases (Alzheimer's, Parkinson's) and cancers. Gut bacteria are the source of LPS and it is translocated to systemic circulation due to disruption of the intestinal barrier function. Studies from our laboratory and those from several others are reviewed herein to demonstrate that curcumin beneficially modulates the different layers of the intestinal barrier and significantly reduces plasma LPS levels. Given the causal link between systemic LPS levels and development of multiple diseases, curcumin-dependent restoration of intestinal barrier function represents a novel mechanism underlying the observed beneficial effects of curcumin.
What is curcumin?
Curcumin (1,7-bis(4-hydroxy-3-methoxyphenyl)-1,6-heptadiene-3,5-dione), also called diferuloylmethane, is principal curcuminoid of the Asian spice turmeric (Curcuma longa), a member of the ginger family. It was first isolated by Vogel and Pelletier in 1815 as a “yellow coloring-matter” from the rhizomes of turmeric.Citation1 While turmeric is extensively used as a spice in India, pure curcumin is commonly used as a dietary supplement, in cosmetics and as a coloring agent. Although the use of turmeric in Ayurvedic medicine has been described and the therapeutic use of Curcuma was recorded as early as 1748,Citation2 Oppenheimer published the first article describing the use of curcumin in human disease in 1937.Citation3 It was subsequently shown to exhibit antibacterial activity in 1949Citation4 and since then curcumin is demonstrated to have multiple beneficial properties including anti-inflammatory and antioxidant activities leading to hypoglycemic as well as wound-healing effects.Citation5 Consistently, several preclinical studies have demonstrated the therapeutic potential of curcumin against a wide range of human diseases (Reviewed by Aggarwal and Harikumar).Citation6 However, the health benefits of curcumin/turmericCitation7 have gained tremendous popularity in the last 5–10 years leading to sales in North America exceeding $20 million in 2014. Curcumin/curcuminoids have also been approved by the US Food and Drug Administration (FDA) as “Generally Recognized As Safe” (GRAS) and Curcumin is available as a supplement in several forms including capsules, tablets and energy drinks. Numerous clinical trials have been conducted thus far to indicate the therapeutic potential of curcumin against a wide range of human diseases and some of these are summarized in .
Table 1. Targeting various diseases by curcumin supplementation. A large number of clinical trials have been conducted to evaluate the beneficial effects of Curcumin supplementation and have been summarized by Gupta et al.Citation86 Listed below are some of the recent trials for modulation of targeted diseases.
The curcumin “paradox”
Despite demonstrated benefits of curcumin in both pre-clinical and clinical studies, poor bioavailability due to negligible absorption and almost undetectable levels in systemic circulation have led to the recent characterization of curcumin as a highly improbable lead for therapeutic development.Citation29 From a medicinal chemistry standpoint, the essentials for the development of a natural product as prototypical “lead” compound for therapeutic discovery and development are: less than 1 μM potency at its desired target(s), evidence of selectivity and tractable mechanism(s) of action, good bioavailability and chemical stability. Unfortunately, due to its poor bioavailability and consequently the inability to demonstrate direct effects on target tissues, orally administered curcumin fails to meet these traditional requirements for future therapeutic development. Yet the observed beneficial effects of curcumin in both pre-clinical studies as well as clinical trials summarized in cannot be overlooked and rather point to the need to explore “unconventional” mechanism(s) of action of curcumin. Following are the important considerations in defining these “unconventional” mechanisms:
• | Given that sufficient curcumin concentrations persist in colonic mucosa, intestine is likely an important site of curcumin action and consistently the most encouraging clinical results of curcumin administration are currently limited to people with colorectal cancer. | ||||
• | Structure-activity relationship studies in vitro as well as in vivo using a DSS-induced colitis model successfully identified the role of the methoxy groups in the anti-inflammatory activity of curcumin providing evidence for beneficial actions of curcumin in the gut.Citation30 | ||||
• | Curcumin-drug pharmacokinetic interactions are also almost exclusively in the enterocytes, owing to extensive first pass metabolism and poor curcumin bioavailability suggesting that enterocytes are the likely target cell type for curcumin action for the increased efficacy of co-administered drugs.Citation31 | ||||
• | Curcuminoids are metabolized by gut bacteria to produce active colonic metabolites that may exert local or even systemic effects.Citation32 | ||||
• | Chronic inflammation underlies majority of the disease conditions where curcumin is shown to be effective and intestinal barrier dysfunction with subsequent release of bacteria/bacterial products into the systemic circulation is one of the major pathways involved in the etiology of this inflammatory condition. By virtue of sustained high concentrations in the gut and action on enterocytes, curcumin may exert its effects by modulating intestinal barrier function and thereby affecting chronic inflammatory diseases. |
With gut as the site of action, the issue of poor bioavailability of curcumin becomes moot and opens up the novel avenue of mechanistic exploration centered on the role of intestinal barrier function in the development of multiple diseases attenuated by curcumin administration.
“Layers” of the intestinal barrier and their modulation by oral supplementation with curcumin
Intestinal barrier consists of multiple layers including: 1) luminal intestinal alkaline phosphatase (IAP) that dephosphorylates bacterial endotoxin lipopolysaccharide (LPS) to detoxify it; 2) the mucus layer that provides a physical barrier preventing interactions between gut bacteria and intestinal epithelial cells; 3) the tight junctions between the epithelial cells that limit the paracellular transport of bacteria and/or bacterial products to systemic circulation; and 4) the anti-bacterial proteins secreted by the intestinal epithelial cells ().
Figure 1. The four layers of the Intestinal barrier. (1) Intestinal alkaline phosphatase (IAP) secreted by the intestinal epithelial cells constitutes the first or the luminal layer and it detoxifies LPS; (2) Loosely attached outer mucin layer and the firmly attached inner mucin layer form the second layer of the barrier and are involved in restricting entry of pathogenic bacteria thereby preventing direct contact of bacteria with the intestinal epithelial cells; (3) Intestinal epithelial cells with tight junctions regulate the transcellular or paracellular transport from the lumen to the systemic circulation (4) Paneth cells located in the crypts produce antibacterial proteins the block any bacteria that have penetrated the overlying layers of the barrier.
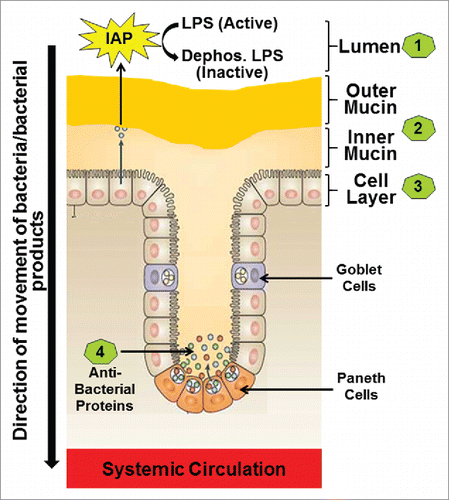
Layer 1 or the Intestinal alkaline phosphatase (IAP)
IAP is part of the luminal first line of defenseCitation33 and catalyzes the removal of one of the two phosphate groups from the toxic lipid A moiety of LPS producing monophosphoryl-LPS that still binds to TLR4 but predominantly acts as an TLR4 antagonist.Citation34 Therefore, IAP is central in maintaining the critical homeostasis that exists between the host and the luminal microbial environment underscoring the importance of exploring supplementation with exogenous IAP to alleviate pathological conditions where host/microbial homeostasis is perturbed such as colitis or chronic inflammation. Consistently, Tuin et al have reported a decrease in IAP in patients with inflammatory bowel diseaseCitation35 and we have demonstrated a significant reduction in IAP activity in response to high fat high cholesterol containing western type (WD) diet resulting in increased translocation of luminal LPS into the systemic circulation.Citation36 Furthermore, oral supplementation with curcumin led to a two-fold increase in IAP activity and attenuated the circulating LPS levels demonstrating the direct effect of curcumin in modulating this first “layer” of the intestinal barrier.Citation36 Improving intestinal barrier function by targeted enhancement of IAP activity is increasing being recognized as a viable approach for modulating chronic inflammatory diseases and in vivo “proof-of-concept” is currently being sought in our laboratory by developing intestine-specific IAP transgenic mice expressing heat stable, chimeric human alkaline phosphatase containing the catalytic domain of human placental alkaline phosphatase that imparts enhanced catalytic efficiency.Citation37
Layer 2 or the mucus layer
The intestinal mucosal layer or the second layer of the intestinal barrier serves as the major defensive wall between the luminal content and the epithelial cells and in the absence of this barrier, intestinal epithelial cells can be in direct contact with luminal bacteria leading to enhanced intestinal inflammation. It mainly consists of water (∼95%) and glycoproteins (1 to 10%), as well as electrolytes, antibodies, and nucleic acidsCitation38 and exists as a stratified firmly attached layer close to the epithelial cells and a loose outer layer which contains the commensal bacteria that prevent the entry of pathogenic bacteria into the outer as well as inner mucus layer. Increase in the number of mucus-residing commensal bacteria, by pro- or prebiotic interventions is thought to improve the barrier function of the mucosal layer.Citation39 The amount and composition of the mucus layer reflect a balance between mucus secretion, and its erosion and degradation by bacteria.Citation40 Depletion of mucus layer either by increased degradation or deficient synthesis will, therefore, have a profound effect on this layer of the intestinal barrier. Consistently, Muc2−/− mice have increased bacterial adherence to the intestinal epithelium, enhanced susceptibility to colitis and disrupted intestinal barrier function.Citation41 Mucin production is affected by both the increase in numbers of terminally differentiated goblet cells and the increase in Muc gene expression within these cells.Citation42 Dietary components, especially the dietary fiber type and content affect mucin secretion by the goblet cells.Citation43,Citation44 In contrast, WD with reduced fiber content disrupts the mucin layer exposing the intestinal epithelial cells to the luminal contents and this effect is attenuated by curcumin supplementation (unpublished observations). It is noteworthy that Junior et al reported curcumin mediated increase in the quantity of acid mucins in the intestineCitation45 and Xun et al showed that dietary addition of curcumin was more effective than quinocetone in improving intestinal mucosal barrier integrity, morphology, and immune status of weaned pigs.Citation46 Collectively, these data provide strong evidence for maintenance of the integrity of the mucus layer by curcumin.
Layer 3 or the epithelium
Intestinal epithelium is a single layer of cells that acts as a highly selective barrier preventing the passage of harmful luminal contents such as foreign antigens, microorganisms and their toxins while allowing the translocation of essential dietary nutrients, electrolytes and water from the intestinal lumen into the systemic circulation. This selectivity of transport is mediated by regulation of two major mechanisms: transepithelial/ transcellular and paracellular transport pathways. Amino acids,Citation47 electrolytes,Citation48 short chain fatty acids and sugarsCitation49 are routinely transported via the transcellular pathway through the epithelial cells and predominantly regulated by selective transporters. Transport through the space between epithelial cells is called the paracellular transport and is regulated by intercellular complexes localized at the apical-lateral membrane junction and along the lateral membrane.Citation50,Citation51 Three components, namely desmosomes, adherens junctions and tight junctions, establish the contact between the adjacent epithelial cells.Citation52 The adhesive junctional complexes consist of transmembrane proteins that link adjacent cells to the actin cytoskeleton via cytoplasmic scaffolding proteins. Along with desmosomes, these are important in the mechanical linkage of adjacent cells.Citation53 The tight junctions, on the other hand, are responsible for sealing the intercellular space and regulating selective paracellular transport.Citation54 These multi-protein complexes facilitate the passage of ions and solutes through the intercellular space but prevent the translocation of luminal antigens, microorganisms and their toxins underscoring their importance in maintaining the integrity of this layer of the intestinal barrier. Consequently, integrity of epithelial cell layer and tight junction proteins is often referred to as the intestinal barrier despite the important contributions of other three “layers”.
Multiple proteins are involved in the assembly of these tight junctions forming fibrils that cross the plasma membrane to interact with proteins from the adjoining cell (). These fibrils between the cells consists of at least two types of tetraspanning membrane proteins, occludin, members of the claudin family, immunoglobulin-like molecules such as junctional adhesion molecule A (JAM-A) and CAR. On the intracellular side of the membrane, the carboxyl terminal ends of these proteins interact with other tight junction proteins such as ZO-1, ZO-2 and ZO-3. Intracellularly, these proteins associate with a ring of actin microfilaments (For detailed review of the organization of tight junction proteins see references Citation55 and Citation56.) The appropriate expression and organization of these proteins as well as organization/contractility of the actin microfilaments regulate paracellular transport. Furthermore, these tight junctions open and close in response to a variety of stimuli including dietary components and luminal microbial factors. One such pathway that is relevant to intestinal barrier dysfunction and the disease process is the zonulin pathway. Analogous to bacterial toxin (zonula occludens), inappropriate upregulation of zonulin secretion from the intestinal epithelial cells into the lumen increases paracellular permeabilityCitation57,Citation58 and fecal levels of Zonulin are used as a clinical measure of intestinal permeability. Therefore, interventions that modulate expression and/or organization of these tight junction proteins are likely to influence paracellular transport of luminal bacteria or bacterial products into the systemic circulation.
Figure 2. Organization of the tight junctions. Membrane spanning proteins Occludin, Claudins, Junctional adhesion molecules (JAMs) and CAR form the fibrils connecting two adjacent cells. The intracellular domains of these proteins are associated with ZO-1, ZO-2 and ZO-3 and also connected to the actin microfilaments. This organization of these tight junction proteins is crucial to maintaining the paracellular transport and is disrupted by changes in expression or phosphorylation of these proteins as well as disorganization of actin microfilaments.
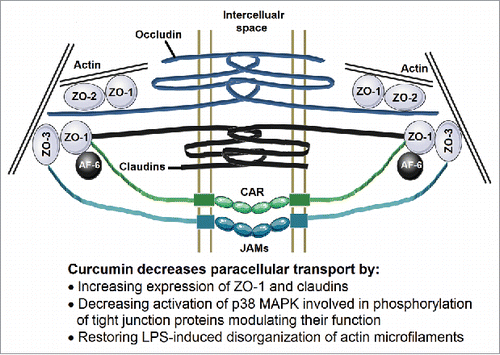
Studies from our laboratory have shown that exposure to curcumin increased the expression of ZO-1 and Claudin-1 in human intestinal epithelial Caco-2 cells, thereby improving the barrier function and reducing paracellular permeability.Citation36 Detailed examination of the signaling pathways involved demonstrated that curcumin attenuated activation of p38 MAPK involved in the phosphorylation of tight junction proteins resulting in disruption of their normal arrangement. Furthermore, curcumin also reduced bacterial endotoxin (LPS) or inflammatory cytokine (IL-1β)-induced disorganization of ZO-1, claudin-1 and claudin-7 as well as actin filaments in intestinal epithelial cells leading to attenuation of paracellular transport.Citation59 Consistent with the data from our laboratory, Martinez et al showed that curcumin preserves the tissue content of claudin-3 and occludin in the colonic mucosa.Citation60 Tian et al also proposed that curcumin protects the intestine from ischemia/reperfusion injury through restoration of the epithelial structure, promotion of the recovery of intestinal permeability, as well as enhancement of ZO-1 protein expression.Citation61 Collectively, these data provide strong evidence for curcumin-mediated beneficial modulation of the layer 3 of the intestinal barrier.
Layer 4 or the antibacterial peptides
In addition to providing a single cell layer physical barrier, the specialized secretory cells of the intestinal epithelium or the Paneth cellsCitation62 secrete antimicrobial peptidesCitation63 that play an important role in host defense against the gut microbes. Thus, secreted anti-bacterial peptides constitute the “fourth” layer of the intestinal barrier. The most abundant anti-microbial peptide in human intestine is α-defensin, a member of the defensing family of peptides. Both α- and β-defensins are bacteriocidal with activity against Gram-negative as well as Gram-positive bacteria. Thus, Paneth cell derived antimicrobial peptides regulate the composition of gut microbiota and a shift in the favor of inflammation rather than homeostasis can result in chronic inflammation. Consistently, disruption of Paneth cell function underlies the susceptibility to chronic inflammatory Crohn's disease providing a direct link between disruptions of this layer of intestinal barrier function to disease development. Guo et al have demonstrated that curcumin increases the expression of anti-microbial peptidesCitation64 providing evidence for curcumin-mediated modulation of this fourth “layer” of the intestinal barrier.
Taken together, considerable evidence exists to demonstrate the direct modulation of all four layers of the intestinal barrier by curcumin and underscore the recognition of curcumin-mediated improvement of intestinal barrier function as the likely mechanism of the beneficial effects of curcumin despite its poor systemic bioavailability.
Diseases linked to intestinal barrier dysfunction and effects of curcumin supplementation
The barrier function of the intestine is critical for normal homeostasis of the gut and breakdown or dysfunction of this barrier is a critical determinant in the etiology of intestinal inflammation and a number of gastrointestinal diseases such as inflammatory bowel disease,Citation65 Crohn's diseaseCitation66 and ulcerative colitis (extensively reviewed recentlyCitation67 and not the emphasis of the present review that is focused on establishing curcumin-mediated improvement of intestinal barrier dysfunction as the mechanism underlying its systemic effects). However, it is noteworthy that breach of the intestinal barrier in these diseases leads to activation of immune cells in the lamina propria by interaction with gut bacteria derived lipopolysaccharide (LPS) resulting in the secretion of pro-inflammatory mediators that perpetuate local inflammation.
In addition to the obvious effects of intestinal barrier function in modulating the local intestinal inflammation, dysfunctional intestinal barrier is increasingly being shown to underlie the development of a variety of diseases and pathological conditions where systemic LPS plays a causative role. Fiddian-Green first described the role of enhanced translocation of bacterial toxins or bacteria during cardiac surgery contributing to morbidity and mortality and recommended interventional measures to reduce this breach of intestinal barrier to improve outcomes.Citation68 While ischemic injury to the intestine was considered to be the underlying trigger, in recent years the role of exogenous or dietary triggers is increasingly being recognized. High fat high cholesterol containing western type diets responsible for the obesity epidemic and metabolic syndrome have been shown to increase intestinal permeability resulting in release of LPS into systemic circulation leading to metabolic endotoxemia.Citation36,Citation69,Citation70 Consistently, continuous infusion of low-dose LPS to mimic metabolic endotoxemia leads to the development of T2DM and atherosclerosis emphasizing the causal relationship between intestinal barrier dysfunction and development of metabolic diseases.Citation71 Disruption of LPS signaling by deletion of its membrane receptor TLR4 prevents diet-induced weight gain as well as development of metabolic disorders.Citation72,Citation73 Furthermore, deletion of LPS-TLR4 signaling partner MyD88 specifically in intestinal epithelial cells protected the animals from diet-induced obesity.Citation74,Citation75 Even in humans, obese phenotype is associated with endotoxemiaCitation76,Citation77 establishing the critical role of gut derived LPS in the development of metabolic diseases and emphasizing the importance of intestinal barrier function that regulates the release of LPS into circulation.
The presence of gut-derived bacteria LPS in systemic circulation, is also identified as a causal or complicating factor in diverse diseases such as autism,Citation78 Alzheimer's diseaseCitation79 as well as asthma.Citation80 Increased intestinal permeability with high levels of circulating LPS and low levels of LPS binding protein are also seen in patients with Parkinson's disease.Citation81 Abnormal intestinal permeability similarly plays a critical role in the pathogenesis of several autoimmune diseases.Citation82 By demonstrating the presence of significantly higher levels of circulating gut bacterial products in subjects with juvenile idiopathic arthritis, Fotis et al reinforced the concept that the intestine is a source of immune stimulation in arthritis.Citation83 Consistent with this hypothesis, Ciccia et al demonstrated increased zonulin expression, damaged intestinal mucosal barrier as well as gut vascular barrier in patients with ankylosing spondylitis.Citation84 Furthermore, they hypothesized that modulation of monocyte behavior by these bacterial products and zonulin may be the mechanism underlying the disease development. It is also noteworthy that metabolic endotoxemia, caused by impaired barrier function and low-grade chronic inflammation is thought to contribute to the onset and progression of osteoarthritis in obese patients.Citation85
In addition to contributing to the above-mentioned diseases, obesity associated risk for development of certain cancers is also related to the chronic pro-inflammatory state resulting from the breach of the intestinal barrier.Citation86 Endotoxin tolerance leading to a compensatory hypo-inflammatory state is thought to underlie immune-escape of cancer cells directly linking circulating LPS to likely development of multiple cancers.Citation87 Increased levels of portal vein LPS are causally linked to the development and progression of hepatocellular carcinoma.Citation88 Similarly, LPS-induced TLR4 signaling is a factor in immune evasion by glioma cancer stem cells.Citation89
Taken together, multiple diseases ranging from metabolic diseases such as diabetes and atherosclerosis to autoimmune as well as neurological disorders and cancer are causally linked to the presence of increased levels of systemic LPS and any intervention that reduces translocation of gut-bacteria derived LPS into the circulation by maintaining the integrity of the intestinal barrier is likely to attenuate the development of these diseases. Oral supplementation with curcumin represents one such strategy and studies from our laboratory have demonstrated curcumin mediated reduction in plasma LPS levels induced by consumption of western diet that led to significant reduction in the development of atherosclerosis and glucose intolerance.Citation36 As summarized in , western diet affects the different “layers” of the intestinal barrier and oral supplementation with curcumin attenuate these effects resulting in decreases release of LPS from the lumen into the systemic circulation. Increased infiltration of activated macrophages into the adipose tissue or the arterial wall underlie the development of T2DMCitation90 and atherosclerosis,Citation91 respectively. Therefore, curcumin-dependent reduction in plasma LPS and subsequent decrease in macrophage activation thus represent the mechanism underlying the anti-diabetic and anti-atherosclerotic effects of curcumin despite its negligible bioavailability.
Figure 3. High fat high cholesterol containing Western diet (WD)-mediated disruption of intestinal barrier function and its systemic consequences. WD decreases the activity of intestinal alkaline phosphatase (IAP), disrupts the mucin layers and increase inflammation of intestinal epithelial cell layer by enhancing infiltration of macrophages. Collectively, these effects lead to increased paracellular permeability and lead to an increase in circulating LPS levels. Systemic and tissue macrophages are activated in response to this metabolic endotoxemia and lead to a chronic inflammatory state that underlie the development of multiple diseases including chronic kidney disease (CKD). Infiltration of activated macrophages in adipose tissue or artery wall lead to the development of Type 2 Diabetes (T2DM) or atherosclerosis, respectively.
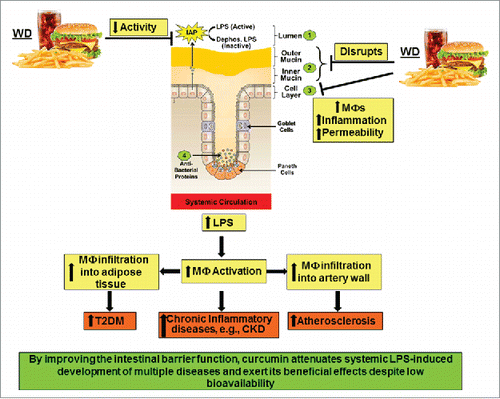
Chronic inflammation also underlies the development of chronic kidney disease and in 5/6th nephrectomy model of this disease we demonstrated significant reduction in tissue inflammation with curcumin supplementationCitation92 and identified the involvement of phospholipases as well as cyclooxygenases.Citation93 However, given the absence of significant levels of bioavailable curcumin at the tissue site, we further established curcumin-mediated modulation of intestinal barrier function as the underlying mechanism.Citation94 Furthermore, nephrectomy and western diet-induced changes in gut microbiota, intestinal barrier function as well as increase in plasma LPS levels were equally modulated by supplementation with curcumin or LPS-binding non-absorbable antibiotic polymyxin underscoring the importance of curcumin dependent reduction in plasma LPS as a key event in its observed systemic effects.Citation95
Based on the accumulating evidence of the role of gut-derived LPS in the pathogenesis of multiple diseases and the direct action of curcumin on intestinal barrier functionCitation59 critical to the translocation of bacterial LPS into systemic circulation, it can be concluded that the observed beneficial effects of curcumin as exemplified by the clinical trials shown in are largely related to curcumin mediated improvement of intestinal barrier function.
Other intestine-specific effects of curcumin
While we have defined some of the intracellular signaling mechanisms by which curcumin modulates intestinal barrier function,Citation59 other studies have shown that curcumin also protects intestinal damage. Kanter et al showed that curcumin treatment has a protective effect against intestinal damage induced by bile duct ligation (BDL) where curcumin treatment inhibits BDL-induced oxidative stress, apoptosis, and cell proliferation.Citation96 Curcumin reduced the expression of pro-apoptotic Bax and stimulated anti-apoptotic Bcl-2 to attenuate 5-fluorouracil-induced apoptosis of intestinal epithelial cells. Consequently, curcumin significantly reversed chemotherapy-induced weight-loss, increase of serum endotoxin and damage to intestinal mucosa structure and Yao et al hypothesized that the clinical administration of Curcumin may improve chemotherapy-induced intestinal dysfunction, thus increasing the clinical efficacy of chemotherapy.Citation97 The beneficial effects of curcumin on tumorigenesis in colorectal cancer have been shown to be associated with the maintenance of a more diverse colonic microbial ecology.Citation98 Curcumin facilitates the recovery of damaged colonic mucosa in inflammatory bowel disease by activation of dendritic cells to enhance the suppressive functions of regulatory T- cells.Citation99 Curcumin also exerts the effects on irritable bowel syndrome through regulating neurotransmitters, BDNF and CREB signaling both in the brain and peripheral intestinal system.Citation100 Curcumin attenuates CCL4-induced liver cirrhosis by reducing the degradation of hepatic LDLR that detoxifies intestinally derived LPS.Citation101 It is also noteworthy that increased translocation of LPS from the intestine is also related to intestinal lipid absorption as well as secretion of chylomicrons and curcumin reduces cholesterol absorption by reducing NPC1L1.Citation102 Oh et al recently elucidated the unsolved mechanism of the anti-cancer effect of curcumin by identifying IL-2 as a direct molecular target since IL-2 is a crucial growth factor for both regulatory and effector T cells.Citation103 Curcumin is taken up by the intestinal epithelial cellsCitation104 and Bartik et al showed that curcumin binds to intracellular vitamin D receptor in intestinal epithelial cells and facilitates colon cancer chemoprevention.Citation105 It needs to be emphasized that interaction between vitamin D and HDAC is associated with the regulation of epithelial barrier functionsCitation106 and it is likely that by enhancing vitamin D signaling, curcumin regulates barrier function.
Conclusions and future perspectives
Gut dysbiosis is increasing being recognized as an important determinant in the development of several diseases. While considerable efforts are being directed towards detailed analyses of the changes in the bacterial diversity, the mechanisms by which these changes affect the “host” and initiate the development of related diseases are not completely defined. Under normal conditions, an intact intestinal barrier thwarts the translocation or bacteria or bacterial products into the systemic circulation of the “host” preventing the deleterious consequences. As reviewed herein, increase in circulating levels of gut bacteria-derived LPS underlies the development of a large number of diseases underscoring the importance of targeted improvement of intestinal barrier function as a viable therapeutic strategy. Oral supplementation with curcumin may represent one such strategy.
Despite demonstrated beneficial effects of curcumin supplementation in several clinical trials, extremely poor bioavailability of curcumin has hindered the establishment of a causal relationship. We have herein described an alternate mechanism of curcumin action focused on its intestine-specific effects especially its role in maintaining the barrier function. summarizes the different aspects of this novel role of curcumin. Curcumin is effectively taken up by the intestinal epithelial cell by an as yet unidentified mechanism.Citation104 Within these cells, curcumin initiates or modulates several signaling pathways that ultimately lead to preventing the disruption of intestinal barrier function induced by external dietary factors or endogenous events such as ischemia/reperfusion injury. The resulting attenuation of luminal bacteria or bacterial products such as LPS underlies the observed beneficial effects of curcumin. By reducing the chronic inflammatory state via its action at the intestinal level, curcumin is well positioned to affect other non-metabolic diseases such as arthritis, cancer and other neurological disorders. It is, therefore, imperative to focus future studies on further characterization of the intracellular pathways modulated by curcumin to improve intestinal barrier function to not only increase our current understanding of its role in modulating such diverse disease processes but also to identify novel intracellular pathways for therapeutic targeting.
Figure 4. Mechanism(s) of action of curcumin in systemic LPS levels. Oral supplementation with curcumin increases the activity of intestinal alkaline phosphatase (IAP) enhancing luminal deactivation of LPS. De-phospho LPS acts as a TLR4 antagonist further reducing LPS-TLR4 interaction and downstream signaling resulting in reduced secretion of pro-inflammatory cytokine IL-1β. As a result LPS or IL-1β mediated activation of MAPK and subsequent phosphorylation and expression of tight junction proteins is attenuated. These modulatory effects of curcumin prevent the disruption of tight junction organization and decrease LPS-mediated increase in paracellular permeability. Additionally, curcumin is taken up by the intestinal epithelial cells and via binding to the vitamin D receptor initiates intracellular signaling events leading to an increase in the expression of tight junction proteins that reduce paracellular permeability. Luminal LPS is also translocated bound to chylomicrons and curcumin decreases cholesterol absorption by reducing the expression of apical cholesterol transporter NPC1L1 resulting in attenuated chylomicron secretion. This pathway may represent yet another mechanism by which curcumin can decrease systemic LPS levels. All events negatively or positively regulated by curcumin are indicated by “-“ or “+” sign in the green circles, respectively.
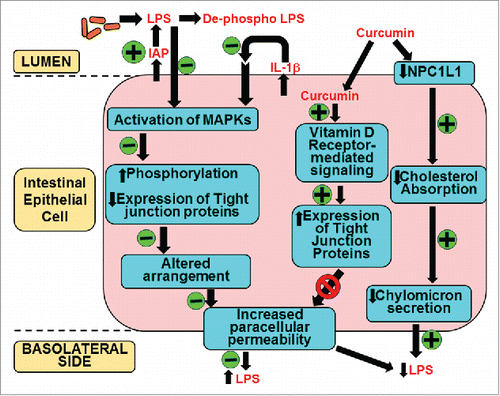
Acknowledgments
This work was partially supported by Basic Innovative Science Award (1-16-IBS-105) from the American Diabetes Association to SG.
Additional information
Funding
References
- Vogel H, Pelletier J. Curcumin –biological and medicinal properties. Journal de Pharmacie. 1815;I:289
- Loeber CC, Buechner AE. Dissertatio inauguralis medica de curcuma officinarum ejusque genuinis virtutibus. In: Praes, Büchnero AE, editors. Diss Inaug Halae. Halle: Halae Magdeburgicae; 1748. p. 28.
- Oppenheimer A. Turmeric (curcumin) in biliary diseases. Lancet. 1937;229:619–621. doi:10.1016/S0140-6736(00)98193-5
- Schraufstatter E, Bernt H. Antibacterial action of curcumin and related compounds. Nature. 1949;164(4167):456. doi:10.1038/164456a0
- Aggarwal BB, Sung B. Pharmacological basis for the role of curcumin in chronic diseases: an age-old spice with modern targets. Trends Pharmacol Sci. 2009;30(2):85–94. doi:10.1016/j.tips.2008.11.002
- Aggarwal BB, Harikumar KB. Potential therapeutic effects of curcumin, the anti-inflammatory agent, against neurodegenerative, cardiovascular, pulmonary, metabolic, autoimmune and neoplastic diseases. Int J Biochem Cell Biol. 2009 Jan;41(1):40–59. doi:10.1016/j.biocel.2008.06.010
- Hewlings SJ, Kalman DS. Curcumin: A Review of Its' Effects on Human Health. Foods. 2017;6:92–102.
- Ganjali S, Sahebkar A, Mahdipour E, Jamialahmadi K, Torabi S, Akhlaghi S, Ferns G, Parizadeh SM, Ghayour-Mobarhan M. Investigation of the effects of curcumin on serum cytokines in obese individuals: a randomized controlled trial. Scientific World Journal. 2014;2014:898361. doi:10.1155/2014/898361.
- Franco-Robles E, Campos-Cervantes A, Murillo-Ortiz BO, Segovia J, López-Briones S, Vergara P, Pérez-Vázquez V, Solís-Ortiz MS, Ramírez-Emiliano J. Effects of curcumin on brain-derived neurotrophic factor levels and oxidative damage in obesity and diabetes. Appl Physiol Nutr Metab. 2014;39(2):211–218. doi:10.1139/apnm-2013-0133
- Yang YS1, Su YF, Yang HW, Lee YH, Chou JI, Ueng KC. Lipid-lowering effects of curcumin in patients with metabolic syndrome: a randomized, double-blind, placebo-controlled trial. Phytother Res. 2014;28(12):1770–1777. doi:10.1002/ptr.5197
- Alwi I, Santoso T, Suyono S, Sutrisna B, Suyatna FD, Kresno SB, Ernie S. The effect of curcumin on lipid level in patients with acute coronary syndrome. Acta Med Indones. 2008;40(4):201–210
- Appendino G, Belcaro G, Cornelli U, Luzzi R, Togni S, Dugall M, Cesarone MR, Feragalli B, Ippolito E, Errichi BM, Pellegrini L, Ledda A, Ricci A, Bavera P, Hosoi M, Stuard S, Corsi M, Errichi S, Gizzi G. Potential role of curcumin phytosome (Meriva) in controlling the evolution of diabetic microangiopathy. A pilot study. Panminerva Med. 2011;53(3 Suppl 1):43–49
- Steigerwalt R1, Nebbioso M, Appendino G, Belcaro G, Ciammaichella G, Cornelli U, Luzzi R, Togni S, Dugall M, Cesarone MR, Ippolito E, Errichi BM, Ledda A, Hosoi M, Corsi M. Meriva(R), a lecithinized curcumin delivery system, in diabetic microangiopathy and retinopathy. Panminerva Med. 2012;54(1 Suppl 4):11–16
- Chuengsamarn S, Rattanamongkolgul S, Luechapudiporn R, Phisalaphong C, Jirawatnotai S. Curcumin extract for prevention of type 2 diabetes. Diabetes Care. 2012;35(11):2121–2127. doi:10.2337/dc12-0116
- Yang H, Xu W, Zhou Z, Liu J, Li X, Chen L, Weng J, Yu Z. Curcumin attenuates urinary excretion of albumin in type II diabetic patients with enhancing nuclear factor erythroid-derived 2-like 2 (Nrf2) system and repressing inflammatory signaling efficacies. Exp Clin Endocrinol Diabetes. 2015;123(6):360–367. doi:10.1055/s-0035-1545345
- Panahi Y, Kianpour P, Mohtashami R, Jafari R, Simental-Mendía LE, Sahebkar A. Efficacy and safety of phytosomal curcumin in non-alcoholic fatty liver disease: a randomized controlled trial. Drug Res (Stuttg). 2017;67(4):244–251. doi:10.1055/s-0043-100019
- Rahmani S, Asgary S, Askari G, Keshvari M, Hatamipour M, Feizi A, Sahebkar A. Treatment of non-alcoholic fatty liver disease with curcumin: a randomized placebo-controlled trial. Phytother Res. 2016;30(9):1540–1548. doi:10.1002/ptr.5659
- Mahammedi H1, Planchat E, Pouget M, Durando X, Curé H, Guy L, Van-Praagh I, Savareux L, Atger M, Bayet-Robert M, Gadea E, Abrial C, Thivat E, Chollet P, Eymard JC. The new combination docetaxel, prednisone and curcumin in patients with castration-resistant prostate cancer: a pilot phase ii study. Oncology. 2016;90(2):69–78 doi:10.1159/000441148
- Ryan JL, Heckler CE, Ling M, Katz A, Williams JP, Pentland AP, Morrow GR. Curcumin for radiation dermatitis: a randomized, double-blind, placebo-controlled clinical trial of thirty breast cancer patients. Radiat Res. 2013;180(1):34–43. doi:10.1667/RR3255.1
- Bayet-Robert M, Kwiatkowski F, Leheurteur M, Gachon F, Planchat E, Abrial C, Mouret-Reynier MA, Durando X, Barthomeuf C, Chollet P. Phase I dose escalation trial of docetaxel plus curcumin in patients with advanced and metastatic breast cancer. Cancer Biol Ther. 2010;9(1):8–14. doi:10.4161/cbt.9.1.10392
- Kanai M, Yoshimura K, Asada M, Imaizumi A, Suzuki C, Matsumoto S, Nishimura T, Mori Y, Masui T, Kawaguchi Y, Yanagihara K, Yazumi S, Chiba T, Guha S, Aggarwal BB. A phase I/II study of gemcitabine-based chemotherapy plus curcumin for patients with gemcitabine-resistant pancreatic cancer. Cancer Chemother Pharmacol. 2011;68(1):157–164. doi:10.1007/s00280-010-1470-2
- Yu JJ, Pei LB, Zhang Y, Wen ZY, Yang JL. Chronic supplementation of curcumin enhances the efficacy of antidepressants in major depressive disorder: a randomized, double-blind, placebo-controlled pilot study. J Clin Psychopharmacol. 2015;35:406–410
- Bergman J1, Miodownik C, Bersudsky Y, Sokolik S, Lerner PP, Kreinin A, Polakiewicz J, Lerner V. Curcumin as an add-on to antidepressive treatment: a randomized, double-blind, placebo-controlled, pilot clinical study. Clin Neuropharmacol. 2013;36(3):73–77. doi:10.1097/WNF.0b013e31828ef969
- Nakagawa Y, Mukai S, Yamada S, Matsuoka M, Tarumi E, Hashimoto T, Tamura C, Imaizumi A, Nishihira J, Nakamura T. Short-term effects of highly-bioavailable curcumin for treating knee osteoarthritis: a randomized, double-blind, placebo-controlled prospective study. J Orthop. Sci. 2014;19(6):933–939. doi:10.1007/s00776-014-0633-0
- Appelboom T, Maes N, Albert A. A new curcuma extract (flexofytol®) in osteoarthritis: results from a belgian real-life experience. The Open Rheumatology Journal. 2014;8:77–81. doi:10.2174/1874312901408010077
- Belcaro, G., et al., Product-evaluation registry of Meriva(R), a curcuminphosphatidylcholine complex, for the complementary management of osteoarthritis. Panminerva Med. 2010;52(2 Suppl 1):55–62
- Belcaro G, Cesarone MR, Dugall M, Pellegrini L, Ledda A, Grossi MG, Togni S, Appendino G. Efficacy and safety of Meriva(R), a curcumin-phosphatidylcholine complex, during extended administration in osteoarthritis patients. Altern Med Rev. 2010;15(4):337–344
- Chandran B, Goel A. A randomized, pilot study to assess the efficacy and safety of curcumin in patients with active rheumatoid arthritis. Phytother Res. 2012;26(11):1719–25. doi:10.1002/ptr.4639
- Nelson KM, Dahlin JL, Bisson J, Graham J, Pauli GF, Walters MA. The essential medicinal chemistry of curcumin – mini perspective. J. Med. Chem. 2017;60:1620–1637. doi:10.1021/acs.jmedchem.6b00975
- Yang H, Du Z, Wang W, Song M, Sanidad K, Sukamtoh E, Zheng J, Tian L, Xiao H, Liu Z, Zhang G. Structure-activity relationship of curcumin: role of the methoxy group in anti-inflammatory and anticolitis effects of curcumin. J Agric Food Chem. 2017;65(22):4509–4515. doi:10.1021/acs.jafc.7b01792
- Adiwidjaja J, McLachlan AJ, Boddy AV. Curcumin as a clinically-promising anti-cancer agent: pharmacokinetics and drug interactions. Expert Opin Drug Metab Toxicol. 2017;13(9):953–972. doi:10.1080/17425255.2017.1360279
- Burapan S, Kim M, Han J. Curcuminoid demethylation as an alternative metabolism by human intestinal microbiota. J Agric Food Chem. 2017;65(16):3305–3310. doi:10.1021/acs.jafc.7b00943
- Lalles JP. Intestinal alkaline phosphatase: novel functions and protective effects. Nutr Rev. 2014;72:82–94. doi:10.1111/nure.12082
- Bentala H, Verweij WR, Huizinga-Van der Vlag A, van Loenen-Weemaes AM, Meijer DK, et al. Removal of phosphate from lipid A as a strategy to detoxify lipopolysaccharide. Shock. 2002;18:561–566. doi:10.1097/00024382-200212000-00013
- Tuin A, Poelstra K, de Jager-Krikken A, Bok L, Raaben W, et al. Role of alkaline phosphatase in colitis in man and rats. Gut. 2009;58:379–387. doi:10.1136/gut.2007.128868
- Ghosh SS, Bie J, Wang J, Ghosh S. Oral supplementation with non-absorbable antibiotics or curcumin attenuates western diet-induced atherosclerosis and glucose intolerance in LDLR−/− mice–role of intestinal permeability and macrophage activation. PLoS One. 2014;9(9):e108577. doi: 10.1371/journal.pone.0108577
- Kiffer-Moreira T, Sheen CR, Gasque KC, Bolean M, Ciancaglini P, et al. Catalytic signature of a heat-stable, chimeric human alkaline phosphatase with therapeutic potential. PLoS One. 2014;9:e89374. doi:10.1371/journal.pone.0089374
- Macfarlane, S., Woodmansey EJ, Macfarlane GT. Colonization of mucin by human intestinal bacteria and establishment of biofilm communities in a two-stage continuous culture system. Appl. Environ. Microbiol. 2005;71:7483–7492 doi:10.1128/AEM.71.11.7483-7492.2005
- Everard A, Belzer C, Geurts L, Ouwerkerk JP, Druart C, Bindels LB, Guiot Y, Derrien M, Muccioli GG, Delzenne NM, de Vos WM, Cani PD. Cross-talk between Akkermansia muciniphila and intestinal epithelium controls diet-induced obesity. Proc Natl Acad Sci U S A. 2013;110(22):9066–9071. doi:10.1073/pnas.1219451110
- Deplancke B, Gaskins HR. Microbial modulation of innate defense: goblet cells and the intestinal mucus layer. Am J Clin Nutr. 2001;73(6):1131S–1141S
- Kissoon-Singh V, Moreau F, Trusevych E, Chadee K. Entamoeba histolytica exacerbates epithelial tight junction permeability and proinflammatory responses in Muc2(−/−) mice. Am J Pathol. 2013;182(3):852–865. doi:10.1016/j.ajpath.2012.11.035
- Theodoropoulos G, Carraway KL. Molecular signaling in the regulation of mucins. J Cell Biochem. 2007;102:1103–1116. doi:10.1002/jcb.21539
- Ito H, Satsukawa M, Arai E, Sugiyama K, Sonoyama K, Kiriyama S, Morita T. Soluble fiber viscosity affects both goblet cell number and small intestine mucin secretion in rats. J Nutr. 2009;139:1640–1647. doi:10.3945/jn.109.110171
- Morita T. Small intestinal goblet cell proliferation induced by ingestion of soluble and insoluble dietary fiber is characterized by an increase in sialylated mucin in rats. J Nutr. 2012;142:1429–1436. doi:10.3945/jn.112.159731
- Alves AJ Júnior, Pereira JA, Pansani AH, Magro DO, Coy CS, Martinez CA. Tissue sulfomucin and sialomucin content in colon mucosa without intestinal transit subjected to intervention with curcuma longa (curcumin). Acta Cir Bras. 2017;32(3):182–193. doi:10.1590/s0102-865020170030000002
- Xun W, Shi L, Zhou H, Hou G, Cao T, Zhao C. Effects of curcumin on growth performance, jejunal mucosal membrane integrity, morphology and immune status in weaned piglets challenged with enterotoxigenic Escherichia coli. Int Immunopharmacol. 2015;27(1):46–52. doi:10.1016/j.intimp.2015.04.038
- Broer S. Amino acid transport across mammalian intestinal and renal epithelia. Physiol Rev. 2008;88:249–86. doi:10.1152/physrev.00018.2006
- Kunzelmann K, Mall M. Electrolyte transport in the mammalian colon: Mechanisms and Implications for disease. Physiol Rev. 2002;82:245–289. doi:10.1152/physrev.00026.2001
- Ferraris RP, Diamond J. Regulation of intestinal sugar transport. Physiol Rev. 1997;77:257–302. doi:10.1152/physrev.1997.77.1.257
- Tsukita S, Furuse M, Itoh M. Multifunctional strands in tight junctions. Nat Rev Mol Cell Biol. 2001;2:285–293. doi:10.1038/35067088
- Van Itallie CM, Anderson JM. Claudins and epithelial paracellular transport. Annu Rev Physiol. 2006;68:403–29. doi:10.1146/annurev.physiol.68.040104.131404
- Farquher MG, Palade GE. Junctional complexes in various epithelia. J Cell Biol. 1963;17:375–412. doi:10.1083/jcb.17.2.375
- Hartsock A, Nelson WJ. Adherens and tight junctions: structure, function and connections to the actin cytoskeleton. Biochim Biophys Acta. 2008;1778:660–669. doi:10.1016/j.bbamem.2007.07.012
- Schneeberger EE, Lynch RD. Structure, function, and regulation of cellular tight junctions. Am J Physiol. 1992;262:L647–L661
- Anderson JM. Molecular structure of tight junctions and their role in epithelial transport. News Physiol Sci. 2001;16:126–130
- Tsukita S, Furuse M, Itoh M. Multifunctional strands in tight junctions. Nat Rev Mol Cell Biol. 2001;2:285–293. doi:10.1038/35067088
- El Asmar R, Panigrahi P, Bamford P, et al. Host-dependent zonulin secretion causes the impairment of the small intestine barrier function after bacterial exposure. Gastroenterology. 2002;123:1607–1615. doi:10.1053/gast.2002.36578
- Fasano A, Not T, Wang W, et al. Zonulin, a newly discovered modulator of intestinal permeability, and its expression in coeliac disease. Lancet. 2000;355:1518–1519. doi:10.1016/S0140-6736(00)02169-3
- Wang J, Ghosh SS, Ghosh S. Curcumin improves intestinal barrier function: modulation of intracellular signaling, and organization of tight junctions. Am J Physiol Cell Physiol. 2017 Apr 1;312(4):C438–C445. doi:10.1152/ajpcell.00235.2016
- Martinez CA, Kadri CJ, Kanno DT, Alves AJ Júnior, Coy CS, Pereira JA. Claudin-3 and occludin content in the glands of colonic mucosa devoid from fecal stream submitted to topical intervention with oil extract of curcuma longa. Acta Cir Bras. 2017;32(1):65–73. doi:10.1590/s0102-865020170108
- Tian S, Guo R, Wei S, Kong Y, Wei X, Wang W, Shi X, Jiang H. Curcumin protects against the intestinal ischemia-reperfusion injury: involvement of the tight junction protein ZO-1 and TNF-α related mechanism. Korean J Physiol Pharmacol. 2016;20(2):147–152. doi:10.4196/kjpp.2016.20.2.147
- Porter EM, Bevins CL, Ghosh D, Ganz T. The multifaceted Paneth cell. Cell. Mol. Life Sci. 2002;59:156–170. doi:10.1007/s00018-002-8412-z
- Zasloff, M. Antimicrobial peptides of multicellular organisms. Nature. 2002;415:389–395. doi:10.1038/415389a
- Guo C, Rosoha E, Lowry MB, Borregaard N, Gombart AF. Curcumin induces human cathelicidin antimicrobial peptide gene expression through a vitamin D receptor-independent pathway. J Nutr Biochem. 2013;24(5):754–759. doi:10.1016/j.jnutbio.2012.04.002
- Michielan A, D'Inca R. Intestinal permeability in inflammatory bowel disease: pathogenesis, clinical evaluation, and therapy of leaky gut. Mediators of Inflamm. 2015;1258:628157.
- Teshima CW, Dieleman LA, Meddings JB. Abnormal intestinal permeability in Crohn's disease pathogenesis. Ann N Y Acad Sci. 2012;1258:159–165. doi:10.1111/j.1749-6632.2012.06612.x
- Martini E, Krug SM, Siegmund B, Neurath MF, Becker C. Mend your fences: the epithelial barrier and its relationship with mucosal immunity in inflammatory bowel disease. Cell Mol Gastroenterol Hepatol. 2017;4(1):33–46. doi:10.1016/j.jcmgh.2017.03.007
- Fiddian-Green RG. Gut mucosal ischemia during cardiac surgery. Semin Thorac Cardiovasc Surg. 1990;2(4):389–399
- Moreira AP, Texeira TF, Ferreira AB, Peluzio Mdo C, Alfenas Rde C. Influence of a high-fat diet on gut microbiota, intestinal permeability and metabolic endotoxaemia. Br J Nutr. 2012;108:801–809. doi:10.1017/S0007114512001213
- Pendyala S, Walker JM, Holt PR. A high-fat diet is associated with endotoxemia that originates from the gut. Gastroenterology. 2012;142:1100–1101. doi:10.1053/j.gastro.2012.01.034
- Cani PD, Amar J, Iglesias MA, Poggi M, Knauf C, Bastelica D, Neyrinck AM, Fava F, Tuohy KM, Chabo C, Waget A, Delmee E, Cousin B, Sulpice T, Chamontin B, Ferrieres J, Tanti JF, Gibson GR, Casteilla L, Delzenne NM, Alessi MC, Burcelin R. Metabolic endotoxemia initiates obesity and insulin resistance. Diabetes. 2007;56:1761–1772. doi:10.2337/db06-1491
- Davis JE, Gabler NK, Walker-Daniels J, Spurlock ME. Tlr-4 deficiency selectively protects against obesity induced by diets high in saturated fat. Obesity. 2008;16:1248–1255. doi:10.1038/oby.2008.210
- Tsukumo DM, Carvalho-Filho MA, Carvalheira JB, Prada PO, Hirabara SM, Schenka AA, Araujo EP, Vassallo J, Curi R, Velloso LA, Saad MJ. Loss-of-function mutation in Toll-like receptor 4 prevents diet-induced obesity and insulin resistance. Diabetes. 2007;56:1986–1998. doi:10.2337/db06-1595
- Everard A, Geurts L, Caesar R, Van Hul M, Matamoros S, Duparc T, Denis RG, Cochez P, Pierard F, Castel J, Bindels LB, Plovier H, Robine S, Muccioli GG, Renauld JC, Dumoutier L, Delzenne NM, Luquet S, Backhed F, Cani PD. Intestinal epithelial MyD88 is a sensor switching host metabolism towards obesity according to nutritional status. Nat Commun. 2014;5:5648. doi:10.1038/ncomms6648
- Hosoi T, Yokoyama S, Matsuo S, Akira S, Ozawa K. Myeloid differentiation factor 88 (MyD88)-deficiency increases risk of diabetes in mice. PloS One. 2010;5:e12537. doi:10.1371/journal.pone.0012537
- Creely SJ, McTernan PG, Kusminski CM, Fisher FM, Da Silva NF, Khanolkar M, Evans M, Harte AL, Kumar S. Lipopolysaccharide activates an innate immune system response in human adipose tissue in obesity and type 2 diabetes. Am J Physiol Endocrinol Metab. 2007;292:E740–E747. doi:10.1152/ajpendo.00302.2006
- Kallio KA, Hatonen KA, Lehto M, Salomaa V, Mannisto S, Pussinen PJ. Endotoxemia, nutrition, and cardiometabolic disorders. Acta Diabetol. 2015;52:395–404. doi:10.1007/s00592-014-0662-3
- Emanuele E, Orsi P, Boso M, Broglia D, Brondino N, Barale F, di Nemi SU, Politi P. Low-grade endotoxemia in patients with severe autism. Neurosci Lett. 2010;471:162–165. doi:10.1016/j.neulet.2010.01.033
- Zhang R, Miller RG, Gascon R, Champion S, Katz J, Lancero M, Narvaez A, Honrada R, Ruvalcaba D, McGrath MS. Circulating endotoxin and systemic immune activation in sporadic amyotrophic lateral sclerosis (sALS). J Neuroimmunol. 2009;206:121–124. doi:10.1016/j.jneuroim.2008.09.017
- Dubin W, Martin TR, Swoveland P, Leturcq DJ, Moriarty AM, Tobias PS, Bleecker ER, Goldblum SE, Hasday JD. Asthma and endotoxin: lipopolysaccharide-binding protein and soluble CD14 in bronchoalveolar compartment. Am J Physiol Lung Cell Mol Physiol. 1996;270:L736–L744. doi:10.1152/ajplung.1996.270.5.L736
- Hasegawa S, Goto S, Tsuji H, Okuno T2, Asahara T, Nomoto K, Shibata A, Fujisawa Y, Minato T, Okamoto A, Ohno K, Hirayama M. Intestinal dysbiosis and lowered serum lipopolysaccharide-binding protein in parkinson's disease. PLoS One. 2015;10(11):e0142164. doi:10.1371/journal.pone.0142164
- Fasano A. Leaky gut and autoimmune disease. Clinic Rev Allerg Immunol. 2012;42:71–78. doi:10.1007/s12016-011-8291-x
- Fotis L, Shaikh N, Baszis KW, Samson CM, Lev-Tzion R, French AR, Tarr PI. Serologic evidence of gut-driven systemic inflammation in juvenile idiopathic arthritis. J Rheumatol. 2017;44(11):1624–1631. doi:10.3899/jrheum.161589
- Ciccia F, Guggino G, Rizzo A, Alessandro R, Luchetti MM, Milling S, Saieva L, Cypers H, Stampone T, Di Benedetto P, Gabrielli A, Fasano A, Elewaut D, Triolo G. Dysbiosis and zonulin upregulation alter gut epithelial and vascular barriers in patients with ankylosing spondylitis. Ann Rheum Dis. 2017;76(6):1123–1132. doi:10.1136/annrheumdis-2016-210000
- Metcalfe D, Harte AL, Aletrari MO, Al Daghri NM, Al Disi D, Tripathi G, McTernan PG. Does endotoxaemia contribute to osteoarthritis in obese patients? Clin Sci (Lond). 2012;123(11):627–634. doi:10.1042/CS20120073
- Djuric Z. Obesity-associated cancer risk: the role of intestinal microbiota in the etiology of the host proinflammatory state. Transl Res. 2017;179:155–167. doi:10.1016/j.trsl.2016.07.017
- Wirthgen E, Hoeflich A. Endotoxin-induced tryptophan degradation along the kynurenine pathway: the role of indolamine 2,3-dioxygenase and aryl hydrocarbon receptor-mediated immunosuppressive effects in endotoxin tolerance and cancer and its implications for immunoparalysis. J Amino Acids. 2015;2015:973548. doi:10.1155/2015/973548
- Roderburg C, Luedde T. The role of the gut microbiome in the development and progression of liver cirrhosis and hepatocellular carcinoma. Gut Microbes. 2014;5(4):441–445. doi:10.4161/gmic.29599
- Che F, Yin J, Quan Y, Xie X, Heng X, Du Y, Wang L. TLR4 interaction with LPS in glioma CD133+ cancer stem cells induces cell proliferation, resistance to chemotherapy and evasion from cytotoxic T lymphocyte-induced cytolysis. Oncotarget. 2017;8(32):53495–53507
- Bie J, Zhao B, Song J, Ghosh S. Improved insulin sensitivity in high fat- and high cholesterol-fed Ldlr−/− mice with macrophage-specific transgenic expression of cholesteryl ester hydrolase: role of macrophage inflammation and infiltration into adipose tissue. J Biol Chem. 2010;285(18):13630–7. doi:10.1074/jbc.M109.069781
- Bie J, Zhao B, Ghosh S. Atherosclerotic lesion progression is attenuated by reconstitution with bone marrow from macrophage-specific cholesteryl ester hydrolase transgenic mice. Am J Physiol Regul Integr Comp Physiol. 2011;301(4):R967–R974. doi:10.1152/ajpregu.00277.2011
- Ghosh SS, Massey HD, Krieg R, Fazelbhoy ZA, Ghosh S, Sica DA, Fakhry I, Gehr TW. Curcumin ameliorates renal failure in 5/6 nephrectomized rats: role of inflammation. Am J Physiol Renal Physiol. 2009;296(5):F1146–F1157. doi:10.1152/ajprenal.90732.2008
- Ghosh SS, Krieg R, Massey HD, Sica DA, Fakhry I, Ghosh S, Gehr TW. Curcumin and enalapril ameliorate renal failure by antagonizing inflammation in 5/6 nephrectomized rats: role of phospholipase and cyclooxygenase. Am J Physiol Renal Physiol. 2012;302(4):F439–F454. doi:10.1152/ajprenal.00356.2010
- Ghosh SS, Gehr TW, Ghosh S. Curcumin and chronic kidney disease (CKD): major mode of action through stimulating endogenous intestinal alkaline phosphatase. Molecules. 2014;19(12):20139–20156. doi:10.3390/molecules191220139
- Ghosh SS, Righi S, Krieg R, Kang L, Carl D, Wang J, Massey HD, Sica DA, Gehr TW, Ghosh S. High fat high cholesterol diet (western diet) aggravates atherosclerosis, hyperglycemia and renal failure in nephrectomized ldl receptor knockout mice: role of intestine derived lipopolysaccharide. PLoS One. 2015;10(11):e0141109. doi:10.1371/journal.pone.0141109
- Kanter M, Takir M, Mutlu HH, Kanter B, Kostek O, Toprak AE. Protective effects of curcumin on intestinal damage in cholestatic rats. J Invest Surg. 2016;29(3):128–136. doi:10.3109/08941939.2015.1088604
- Yao Q, Ye X, Wang L, Gu J, Fu T, Wang Y, Lai Y, Wang Y, Wang X, Jin H, Guo Y. Protective effect of curcumin on chemotherapy-induced intestinal dysfunction. Int J Clin Exp Pathol. 2013;6(11):2342–2349
- McFadden RM, Larmonier CB, Shehab KW, Midura-Kiela M, Ramalingam R, Harrison CA, Besselsen DG, Chase JH, Caporaso JG, Jobin C, Ghishan FK, Kiela PR. The role of curcumin in modulating colonic microbiota during colitis and colon cancer prevention. Inflamm Bowel Dis. 2015;21(11):2483–2494. doi:10.1097/MIB.0000000000000522
- Zhao HM, Xu R, Huang XY, Cheng SM, Huang MF, Yue HY, Wang X, Zou Y, Lu AP, Liu DY. Curcumin improves regulatory T cells in gut-associated lymphoid tissue of colitis mice. World J Gastroenterol. 2016;22(23):5374–5383. doi:10.3748/wjg.v22.i23.5374
- Yu Y, Wu S, Li J, Wang R, Xie X, Yu X, Pan J, Xu Y, Zheng L. The effect of curcumin on the brain-gut axis in rat model of irritable bowel syndrome: involvement of 5-HT-dependent signaling. Metab Brain Dis. 2015;30(1):47–55. doi:10.1007/s11011-014-9554-z
- Cai Y, Lu D, Zou Y, Zhou C, Liu H, Tu C, Li F, Liu L, Zhang S. Curcumin protects against intestinal origin endotoxemia in rat liver cirrhosis by targeting PCSK9. J Food Sci. 2017;82(3):772–780. doi:10.1111/1750-3841.13647
- Feng D, Zou J, Zhang S, Li X, Lu M. Hypocholesterolemic activity of curcumin is mediated by down-regulating the expression of niemann-pick C1-like 1 in hamsters. J Agric Food Chem. 2017;65(2):276–280. doi:10.1021/acs.jafc.6b04102
- Oh JG, Hwang DJ, Heo TH. Direct regulation of IL-2 by curcumin. Biochem Biophys Res Commun. 2017 Nov 7;pii:S0006-291X(17) 32216–7
- Dempe JS, Scheerle RK, Pfeiffer E, Metzler M. Metabolism and permeability of curcumin in cultured Caco-2 cells. Mol Nutr Food Res. 2013;57(9):1543–1549. doi:10.1002/mnfr.201200113
- Bartik L, Whitfield GK, Kaczmarska M, Lowmiller CL, Moffet EW, Furmick JK, Hernandez Z, Haussler CA, Haussler MR, Jurutka PW. Curcumin: a novel nutritionally derived ligand of the vitamin D receptor with implications for colon cancer chemoprevention. J Nutr Biochem. 2010;21(12):1153–1161. doi:10.1016/j.jnutbio.2009.09.012
- Liu FH, Li SS, Li XX, Wang S, Li MG, Guan L, Luan TG, Liu ZG, Liu ZJ, Yang PC. Vitamin D3 induces vitamin D receptor and HDAC11 binding to relieve the promoter of the tight junction proteins. Oncotarget. 2017;8(35):58781–58789