Abstract
Culex pipiens pallens are the most common mosquito’s vector in Asia. In order to protect the people from diseases, the anti-mosquito population is necessary that uses safe and new bio-pesticides such as bacteria-AgNPs. In our report, we used two kinds of bacteria to synthesize silver nanoparticles to examine the toxic effect on the larvae and pupae of Cx. pipiens pallens and also used as antimicrobial activity. The biosynthesis of AgNPs and its characterization was carried out by UV-Vis spectrophotometry, FTIR, TEM, SEM, and EDX. The larvicidal and pupicidal assays revealed that the lethal concentration LC50 values of Bacillus amyloliquefaciens-AgNPs were 0.72 ppm (I), 0.73 ppm (II), 0.69 ppm (III), 1.16 ppm (IV), and 4.18 (Pupae), while LC50 values of Bacillus subtilis-AgNPs were 0.60 ppm (I), 0.62 ppm (II), 0.21 ppm (III), 0.28 ppm (IV), and 3.46 ppm (Pupae) after 72 h of exposure. Antibacterial activity test of AgNPs reveals better results against rice pathogenic bacteria than bacteria alone. Thus, bacteria-mediated silver nanoparticles have a rapid effect on vector mosquito and microbial pathogen suggesting savings of energy and resources. Hence, bacteria-AgNPs may be used in the future as an effective weapon to control vector mosquito and harmful bacteria.
Introduction
Culex mosquito serves as vector for many diseases for humans, especially in urban and tropical areas. Lymphatic filariasis, commonly known as elephantiasis is transmitted to humans through mosquitoes, causing hidden damage to the lymphatic system, which leads to permanent disability (WHO Citation2015). Presently, more than 1.4 billion people in 73 countries are residing in areas where lymphatic filariasis is transmitted and the population is at risk of being infected (WHO Citation2014). Furthermore, the recent outbreaks of Zika virus infections, occurring in South America, Central America and the Caribbean, represent the most recent of four arrivals of arboviruses in the Western Hemisphere. There are no specific treatments for these arboviruses (Benelli and Mehlhorn Citation2016). Zika virus follows dengue which slyly arrived in the hemisphere over decades and became more aggressive in the 1990s, west nile virus emerged in 1999 and chikungunya emerged in 2013 (Benelli and Mehlhorn Citation2016). China is considered one of those countries facing health hazards of filariasis and the Chinese government has been working hard to prevent the spread of the filariasis in all its provinces (De-Jian et al. Citation2013).
In most cases, mosquito larvae are usually targeted using organophosphates, insect growth regulators, microbial agents and insecticide-treated bed nets. In fact, these chemicals have a negative impact on human health and the environment. Recently, new and safe tools were developed to strengthen the control of mosquitoes, like sterile insect technique, “SIT” and mosquitocidal nanoparticles, “MNP”. Few parts per million of different MNP are highly toxic against the malaria vector Anopheles stephensi, the dengue vector Aedes aegypti, and the filariasis mosquito Culex quiquefasciatus. However, in spite of the increasing evidences about the effectiveness of MNP, moderate efforts have been carried out to draw attention to their possible non-target effects against mosquito’s natural enemies and other aquatic organisms (Benelli Citation2015, Citation2016; Muthukumaran et al. Citation2015). For a long time, biological organisms have been used in mosquito control programs, such as the kinds of Bacillus, which is well-known for its effect on mosquito larvae. Among them, Bacillus sphaericus and Bacillus thuringiensis are effective against Culex mosquitoes (Soni and Prakash Citation2014). The increase of resistant insects to chemical pesticides and toxicity problems has drawn the researcher’s attention to the need for development of new insecticides like silver nanoparticles (Panneerselvam et al. Citation2013; Roni et al. Citation2015; Santhosh et al. Citation2015; Shah et al. Citation2015). Bacillus amyloliquefaciens is one of the most common bacterial endophytes, which infected many plants and it has gained considerable attention due to its beneficial effect of improving plant disease resistance, where, crude lipopeptides from cultures of B. amyloliquefaciens showed antagonistic activity against three kinds of pathogen. However, the feeding larvae test of fall armyworms (Spodoptera fruigiperda) did not show a significant difference in weight gains between larvae fed with leaves dipped in crude lipopeptides and with control leaves (Chen et al. Citation2013; Li et al. Citation2015). Biocontrol-active isolates of B. amyloliquefaciens and B. subtilis produce various antimicrobial CLP, including surfactin, iturin A, and fengycin. Besides, limited on insect pests, the metabolites have antifungal and antibacterial activities, which affect bacterial motility and biofilm formation (Chowdhury et al. Citation2015; Nam et al. Citation2016).
Plant disease control is important for agriculture in the twemty-first century. Microorganisms are associated with several devastating diseases in economically important crops worldwide, and were responsible for significant losses to farmers. Among the microorganisms, bacterial leaf blight of rice, caused by Xanthomonas oryzae pv. oryzae (Xoo), is responsible for huge economic loss. It was known to occur in all rice growing areas and is exceptionally severe in Asia. It has been reported that BLB extremely affect yield losses up to 50 or 60% in tropical regions throughout Asia (Hop et al. Citation2014; Lee et al. Citation2008, Citation2013). Therefore, reducing the disease year-to-year and minimizing the rate of epidemics are the main issue to sustaining rice productivity. Nowadays, we have a big problem in controlling disease because most of the bacteria have developed resistance to bactericidal. The current control measures were concentrated mostly on preventing dissemination of disease to uninfected plants. Recent researches drew a great attention on the nanoparticles for controlling plant diseases.
The synthesized AgNPs proved excellent antimicrobial activity against varieties of gram-negative and gram-positive pathogenic microorganisms. In addition, the AgNPs showed the potential application of antibacterial agent against multidrug-resistant bacteria (Du et al. Citation2016; Kim et al. Citation2009). However, recently those microorganisms have been discovered as the prospective biofactory for the synthesis of metallic nanoparticles such as silver, gold and cadmium (Gopinath and Velusamy Citation2013; Mubarak Ali et al. Citation2012; Pawar et al. Citation2016). Several of researchers have been experimenting on the microbes especially fungi like Cladosporium tropicum, Aspergillus niger, Pencillium sp. but few experiments have been reported using bacteria like Bacillus thuringiensis, to synthesize silver nanoparticles so as to dynamically control the mosquito larvae and pupae (Banu et al. Citation2014; Dhanasekaran and Thangaraj Citation2013; Padman et al. Citation2014; Soni and Prakash Citation2012). Therefore, we adopted an approach involving bio-reduction of silver ions using bacteria to highlight the advantages such as no need to use high pressure, energy, temperature, and toxic chemicals for such a synthesis.
In the current research, we focused on mycosynthesis of silver nanoparticles using two kinds of bacteria, Bacillus amyloliquefaciens (D29) and Bacillus subtilis (A15) to study the larvicidal and pupicidal effects of bacteria-AgNPs as a capping approach against the spread of vector mosquito and also pathogenic bacteria to rice plants.
Materials and methods
Isolation and definition of the bacteria
Seven biocontrol agents including four strains of Bacillus amyloliquefaciens A1, A3, A13, and D29 (Access No. KF112077, JN661697, JN700138, and JF460733), respectively, two strains of B. methylotrophicus A2 and H8 (Access No. JF899259 and HQ662588), respectively, and one strain of B. subtilis A15 (Access No. EF423590). Besides, rice pathogen, X. oryzae pv. oryzae (Xoo) strain PXO99 were provided by the Institute of Biotechnology, Zhejiang University, China (El-Shakh et al. Citation2015). Briefly, the Bacillus strains were prepared by streaking on Luria–Bertani (LB) agar medium and the plates were incubated at 28 °C for 24 h. Then, Bacillus strains were inoculated in LB broth medium, incubated at 28 °C for 12 h with constant shaking at 200 rpm. The bacterial suspension centrifuged at 8000g for 10 min and bacterial pellets harvested and suspended with sterile saline buffer solution to obtain the final concentration of (108 cfu/mL) (Thompson et al. Citation1996). Totally, seven strains were insulated and used for nanoparticles synthesis. Based on the rapid reduction of Ag metal ions, B. amyloliquefaciens (D29) and B. subtilis (A15) strains were chosen and used for further characterization.
Extracellular synthesis silver nanoparticles
In order to screen an effective strain for the synthesis of silver nanoparticles, all the isolated bacterial strains were freshly inoculated in LB broth medium and incubated at 28 °C for 12 h. The pure culture of Bacillus strain was then grown in an Erlenmeyer flask containing [LB Broth (Hi-Media)] and incubated at 28 °C for 24 h on an Orbit Incubator Shaker at 180 rpm. After the incubation period, the culture supernatant was obtained by centrifugation at 10,000 rpm for 8 min. To synthesis AgNPs, 115 mL aqueous solution of 3 mM (AgNO3) (Laboratory of Urban Entomology, Institute of Insect Science, Zhejiang University, Zhejiang, China) (0.051 g/100 mL) was treated with 35 mL of culture supernatant solution in a 250 mL Erlenmeyer flask at 200 rpm in 29 °C for 48 h and preserved under the dark conditions. The supernatant (without an addition of AgNO3) was saved as a control. Hence, the bio-reduction was observed by optical color change and sampling an aliquot (4 mL) of the solution after 24 h and measured in an UV-vis spectrophotometer. On the basis of the fast reduction of AgNO3 into AgNPs, a capable bacterial strain was chosen and used for further characterization.
Characterization of Bacillus-AgNPs
Two milliliters of AgNPs, which was formed using the cell free supernatant was diluted with 2 mL Milli-Q water and then characterized by UV-2550 spectra (UV-Visible Spectrophotometer Shimadzu, Japan) at a resolution of 1 nm in the range of 200–750 nm. The FT-IR analysis was accomplished with cell free culture and reduced silver nanoparticles were recorded under identical conditions in the range of 500–4000 cm−1 region at a resolution of 4 cm−1 using Fourier transform infrared spectrometer (Vector 22, Bruker, Germany). The synthesized silver nanoparticles samples (1 mg) were mixed with KBr (300 mg) to make a pellet by pressing with the hydraulic pellet press. The values of the peaks observed pointed to the functional groups responsible for synthesized AgNPs. The quality and formation of compounds were tested using Siemens X-ray diffractometer (XRD) analysis. Where, XRD pattern was measured by drop coated film of dried powder of silver nanoparticles onto glass slides. The operation conditions were at a voltage of 45 keV and a current of 20 mA with Cu-Kα radiation as an X-ray source in the range of 20–80° at the 2θ angle. Further, the morphology of the synthesized AgNPs was measured by Scanning Electron Microscopic (SEM) using TM-1000, Hitachi, Japan. Thin film of the sample was prepared on a carbon coated copper grid by simply dropping a very small amount of the sample on the grid. The film on the SEM grid was then allowed to dry by putting the grid under a mercury lamp for 5 min.
The structural characterization of AgNPs was carried out by Transmission Electron Microscopy (TEM) (JEM-1230, JEOL, Akishima, Japan). The extra sample was removed from carbon-coated copper grid using the cone of a blotting paper and sample was placed on the carbon-coated copper grid to make a thin film of the sample and then it was kept in a grid box sequentially. The instrument was equipped with an energy dispersive spectrum (EDS) to confirm the presence of silver metal. The morphology and size of the synthesized silver nanoparticles were further characterized by the atomic force microscopy (AFM) images. The microscopic images were recorded with silicon cantilever with force constant 0.22–0.77 N/m, tip height 10–12 nm in the contact mode.
Larvicidal and pupicidal activity
Larvae and pupae collection
Culex pipiens pallens eggs were collected from the laboratory of Urban Entomology, Institute of Insect Sciences, Zhejiang University. To develop the colony after hatching, the larvae were kept in plastic and enamel trays containing tap water. First instar larvae were fed with the small quantity of rat food even up to the fourth instar form. The pupae converted were separated manually and relocated by transport tool into glass beaker (100 mL) containing distilled water. The beaker was introduced into cages for the emergence of adult mosquitoes. Cotton soaked in 5% glucose solution was used as glucose meal for adult mosquitoes and periodically they were provided with a mouse to serve as a blood source for laying eggs (Mohan et al. Citation2006). Second and third instars were used directly and first instars were reared up to fourth instar in the laboratory before using them in the experiment.
Larvicidal and pupicidal assay
With slight modifications, standard methods for testing the toxicity of biologically synthesized nanoparticle and the susceptibility of the mosquito to insecticides were performed as specified by WHO (Citation2004). The setup was maintained at 27 ± 1 °C and 70 ± 4% RH, a photoperiod of 14:10 L:D. The desired concentrations such as 2.5, 5, 7.5, and 10 ppm put in a 250 mL beaker (Borosil) were prepared using deionized water as a solvent. The AgNPs toxicity test was made by using 20 larvae of each instar (I-IV instar) and pupae into 200 mL of double sterilized distilled water. Each test including a set of control group (cell free supernatant) was tested with four replicates for each individual concentration. The effectiveness of silver nanoparticles as mosquito larvicidal and pupicidal were determined from all the larvae (I-IV instar) and pupae by exposing them to different time periods. The AgNPs toxicity was assessed against the larvae of Cx. pipiens pallens at 24, 48, and 72 h of exposure. The number of dead larvae and pupae were counted with four replicates and corrected using the Abbott formula (Abbott Citation1925).
In vitro, the effects of nanoparticles, Bacillus strains against Xanthomonas oryzae pv. Oryzae
The inhibitory effect of AgNPs was tested alone and combinations with Bacillus spp. against the pathogen by agar diffusion technique according to the method reported by Monteiro et al. (Citation2005) with some modifications. The treatments in this experiment include A15 and D29 individuals and A15-AgNPs and D29-AgNPs. Briefly, 200 mL pathogen (108 CFU/mL), obtained from 24 h old cultures in NB media, was added to 10 mL LB medium in a Petri dish, which had already solidified. The upper agar medium was allowed to solidify and air dried. Different concentrations of suspensions of (108 CFU/mL) antagonistic strains and AgNPs were placed in equidistance to each other on the seeded medium on the agar surface and incubated at 28 ± 2 °C for 48 h. Antibacterial activity was measured as the diameter of inhibition zone around the colony of bacterial strains. This experiment was repeated twice with three replicates for each treatment in a completely randomized design.
Statistical analysis
The percentage of mortality for larvae and pupae treated with AgNPs were subjected to probit analysis (Finney Citation1971) for calculating LC50, LC90 statistics at 95% confidence limits of lower and upper values. Chi-square values were calculated using the SPSS Statistical Software Package version 16.0 (SPSS Inc., Chicago, IL). Individual comparisons between mean values were performed by using the least significant differences (LSD). Results with P < .05 were considered to be statistically significant.
Results and discussion
Characterization and biological synthesis of AgNPs
UV–vis spectroscopy
The effective consolidation of B. amyloliquefaciens and B. subtilis with 3 mM AgNO3 was approved by the color change from light yellow to dark brown ( and ). The existence of silver nanoparticles was confirmed and measured by using UV spectrophotometer. The formation was shown at absorption peak of 405 nm and 420 nm for B. amyloliquefaciens and B. subtilis, respectively, after 24 h of incubation time. The control cell-free supernatant (without an addition of AgNO3) exhibited surface plasmon resonance (SPR) peak at 210 nm, which is unique for reducing proteins (). In contrast, previous reports indicated that silver nanoparticles have free electrons, which create a surface plasmon resonance at 406, 416, 430, and 448 nm (Banu et al. Citation2014; Du et al. Citation2016; Gopinath and Velusamy Citation2013; Sundaravadivelan and Padmanabhan Citation2014; Wang et al. Citation2015). There is an inverse relationship between increasing SPR peaks and decreasing the symmetry of the nanoparticles. Further, variation particles might give two or more bands of surface plasmon resonance depending on the particle shape (Kanchana et al. Citation2011). The cleansed pellets were collected, then freeze-dried, powdered and characterized by FTIR, SEM, TEM, XRD, and EDX analyses.
FT-IR analysis
Studies of the infrared spectrum were performed to verify the potential mechanism behind the formation of AgNPs and provide information about the functional groups. The typical spectra of AgNPs using cell-free supernatant of B. amyloliquefaciens (D29) were presented in . The peaks were shown at 3384.91, 1633.91, 1359.54, 1064.84, and 528.96 cm−1. The very strong broad absorption peaks at 3384.91 correspond to strong stretching vibrations of (O–H); 1633.91 cm−1 that was associated with stretching vibration of C-H and some variable stretching and bending peaks were observed, which might be from AgNO3 solution. The strong interaction of water with Ag surface might be the reason for the O–H stretching and C = C, =C–H, C = O, N–H functional groups, which may be present between the amino acid residues and protein synthesized during microbial AgNPs. The IR spectrum of synthesized AgNPs by B. subtilis (A15) was shown in . The peaks at 3386.75, 1634.43, 1395.58, 1079.35, and 532.60 cm−1 were distinctive. The comparison between the two kinds of AgNPs synthesized by B. amyloliquefaciens and B. subtilis shows that there were minor changes in these values 3384.91, 3386.75 indicating the hydroxyl functional group. In addition to the absorption bands, slight changes were observed in all the peak sites for the synthesis of silver nanoparticles with the possible reason of the cover enveloping protein on the nanoparticle surface.
Figure 2. The FT-IR spectra of AgNPs biosynthesized by cell-free supernatant of Bacillus amyloliquefaciens (D29) and Bacillus subtilis (A15).
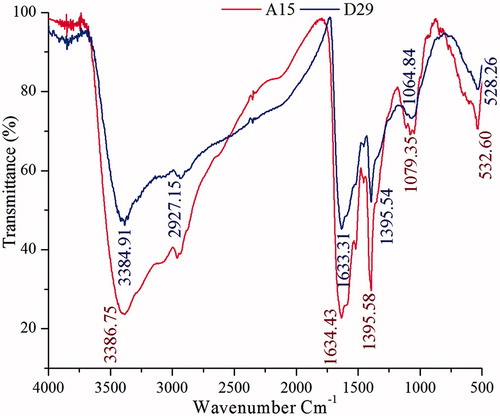
A bacterial cell-free extract was used for the silver nanoparticle synthesis and it is suggested that the extracellular polymeric substance (EPS) plays a major role in the silver nanoparticle formation. EPS are the microbially produced organic compounds that constitutes polysaccharides, proteins, nucleic acids, uronic acids, lipids, and functional groups such as carboxyl, phosphoric, amine, and hydroxyl groups. Proteins have suggested playing key role in the biomediated synthesis of nanoparticles (Padman et al. Citation2014; Sanghi and Verma Citation2009).
Hence, with the noticeable infrared spectrum results, we can conclude that the protein in the cell-free supernatant might be responsible for the bio-reduction besides steadiness of AgNPs. Previous studies mentioned that protein can easily tie silver nanoparticles through free amino groups of cysteine residues (Gole et al. Citation2001). According to previous reports (Janeiro et al. Citation2005; Udayasoorian et al. Citation2011) participation of other possible functional biomolecules such as enzymes, esters, anhydrides, and alkynes in the cell wall of bacteria showed the ability to synthesize the silver nanoparticles.
SEM and X-ray diffraction analysis
Scanning electron microscopy images provide further understanding about morphology and size details of AgNPs. The silver nanoparticles (freeze-dried) were measured under a HITACHI SU-8010 SEM at 3 kV and and ) gives a clear picture of the nanoparticles synthesized by B. amyloliquefaciens and B. subtilis. Most of SEM images of AgNPs observed from the micrograph were spherical with the size ranging from 15.9 to 80 nm. In addition, synthesis of similar-sized particles from bacteria Bacillus megaterium and Pseudomonas mandelii (46.10 to 80.62 nm) were reported in literature (Banu and Balasubramanian Citation2015; Mageswari et al. Citation2014).
Figure 3. SEM images of AgNPs synthesized by reducing 3 mM AgNO3 using cell free supernatant of B. amyloliquefaciensD29 (A) and B. subtilisA15 (B); XRD patterns of synthesized AgNPs (C and D).
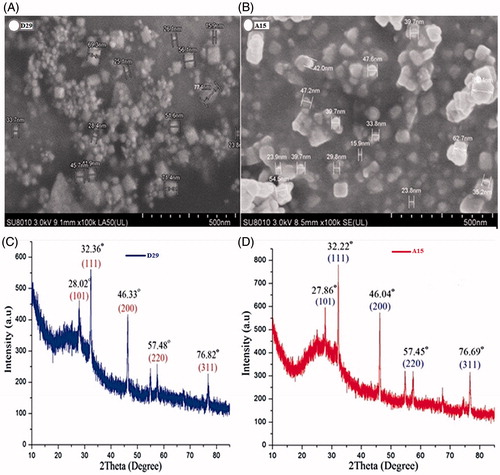
The crystalline nature of synthesized silver nanoparticles was examined by X-ray diffraction technique. The XRD analysis showed the diffraction peaks at 2θ = 38.27°, 46.27°, 64.64°, and 77.66° which exemplifies the (111), (200), (220), and (311) Bragg’s reflection of the face-centered cubic structure of silver nanoparticles ( and ). Amongst the seven strains experimented, the silver nanoparticles from B. amyloliquefaciens and B. subtilis cell-free spectrums confirmed the existence of silver and then it was used for further tests. Hence, the results suggest that the crystallization of bio-organic compounds happened on the surface of AgNPs and the same has been shown by other studies (Velu et al. Citation2015).
TEM and EDX spectrum analyses
The spherical form of synthesized silver nanoparticles using free cell supernatant from two types of bacteria was confirmed by transmission electron microscope (). These results are similar to the studies of Lallawmawma and his colleagues (Citation2015) who described the shape of synthesized AgNPs as spherical.
Figure 4. (A) TEM images of AgNPs synthesized by reducing 3 mM AgNO3 using cell free supernatant of B. subtilis (A15) and B. amyloliquefaciens (D29), (B) EDX patterns of synthesized AgNPs.
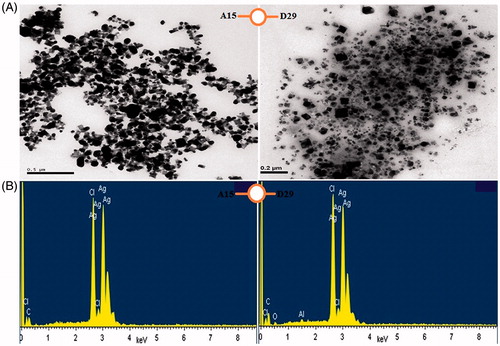
The existence of silver element in the silver nanoparticles was confirmed by EDX instrument. This indicates the formation of the silver nanoparticles in cell-free supernatant from bacteria D29 and A15. Where, the strong peak of silver ions was observed at 3 KeV, which confirmed the reduction of silver ions Ag+ to Ag0 (). The same bank peak (3 keV) has been reported by other researchers (Suganya et al. Citation2014).
In the biomediated synthesis silver nanoparticle (), AgNO3 ionizes to Ag+ and NO3- in solution, followed by the reduction of Ag+ to metallic silver (Ag0). EPS is not active cells but have electrons (Laspidou and Rittmann Citation2002). The electrons from the EPS could donate electrons to Ag+, reduce them to metallic silver and stabilize as nanoparticles (Padman et al. Citation2014).
Toxicity of Bacillus-AgNPs to mosquito larvae and pupae
In the present report, the LC50 and LC90 values for B. amyloliquefaciens-AgNPs against the first to fourth instar larvae and pupae of vector mosquito were 0.72, 0.73, 0.69, 1.16, and 4.18 ppm and LC90 values were 2.90, 2.25, 3.19, 4.58, and 56.59 ppm after 72 h (); LC50 and LC90 values for B. subtilis-AgNPs were 0.60, 0.62, 0.21, 0.28, and 3.46 ppm and LC90 values were 2.05, 1.87, 1.60, 4.14, and 34.08 ppm after 72 h (). The χ2 value was significant at P ≤ .05. Recently, significant effects on different vector mosquitoes have been reported using silver nanoparticles. Though, the cell-free supernatant has less or no effect on the larval instar and pupae of C. pipiens pallens as compared to the AgNPs treatments that have stronger lethal effect on mosquito larvae and pupae. The regression equation suggested that the linear relationship between the rates of mortality and doses of silver nanoparticles have positive relationship between the higher doses of silver nanoparticles and the larval body's absorption of high quantity of nanoparticles that caused their death at the end. The laboratory studies on Bacillus megaterium-AgNPs were carried out for its insecticidal activity against Culex quinquefasciatus and Aedes aegypti at a different concentration, where all larval stage of Cx. quinquefasciatus and Ae. aegypti were found to be more susceptible to the synthesized silver nanoparticles (Banu and Balasubramanian Citation2015; Deepak et al. Citation2016; Roni et al. Citation2015).
Table 1. Larval and pupal toxicity of silver nanoparticles synthesized using cell free supernatant of B. amyloliquefaciens (D29) against C. pipiens pallens.
Table 2. Larval and pupal toxicity of silver nanoparticles synthesized using cell-free supernatant of B. subtilis (A15) against C. pipiens pallens.
Likewise, LC50 for first (0.079), second (0.084), third (0.087), fourth (0.068) instar larvae and pupae (0.026) of Aedes aegypti were observed when exposed to AgNPs produced from extracellular filtrate of Trichoderma harzianum (Sundaravadivelan and Padmanabhan Citation2014). The recorded studies were very few in the synthesis of silver nanoparticles using bacteria against the pests. The researchers (Banu and Balasubramanian Citation2015, Banu et al. Citation2014) have reported that synthesis of silver nanoparticles using B. thuringiensis and B. megaterium and AgNPs have been effective against Ae. aegypti and Cx. quinquefasciatus. The reason for larval mortality might be due to the internal toxic effects of small particles inside cuticle. The cross movement of tiny particles targeting individual cell and preventing molting and other physiological processes that led to mortality of insects have been described elsewhere (Santhosh et al. Citation2015; Sap-Iam et al. Citation2010). Most likely, this is the first investigation with synthesized AgNPs using B. amyloliquefaciens and B. subtilis against larval instar and pupae of Cx. pipiens pallens. The microbe-mediated silver nanoparticles have a rapid effect on vector mosquito population, thus achieving the bacteria-mediated synthesis of AgNPs to be used as vector control strategy.
Morphological studies in fourth instar and pupae
The fourth instar larvae of the mosquito Cx. pipiens pallens, when treated with silver nanoparticles synthesized cell-free supernatant for both types of bacteria showed some morphological changes compared to the control. The structure changes were noted in the thorax and abdomen, led to significant damage in the anal papillae region as well as cuticle layer. These structural distortions in the anal papillae and cuticle may have close relation with the death of exposure larval by silver nanoparticles. As well as, larvae which were to have the ability to transformation to the pupal stage have severe distortions in the head, thorax, and abdomen. In addition, the pupae lost the ability to breathe because of the lack of a trumpet (). Structural deformations of anal papillae were regarded one of the most important reasons of the treated mosquito larvae's death (Perumalsamy et al. Citation2010).
Figure 6. (A) Mortality of Cx. pipiens pallens mosquito larvae (fourth instar) after 24 h exposure of AgNPs synthesized using cell free supernatant of B. subtilis and B. amyloliquefaciens. (B) No mortality was observed in control larvae. (C) Light micrographs showing morphological characteristics of treated larvae which represent morphological changes in the head, thorax, abdomen and anal papillae region. (D) No morphological changes were observed in the head, thorax and abdomen region of control larvae. (E) The treated pupae showed considerable morphological changes in the whole body spatially in the cuticle layer and trumpet. (F) The control pupae showed no morphological disruption.
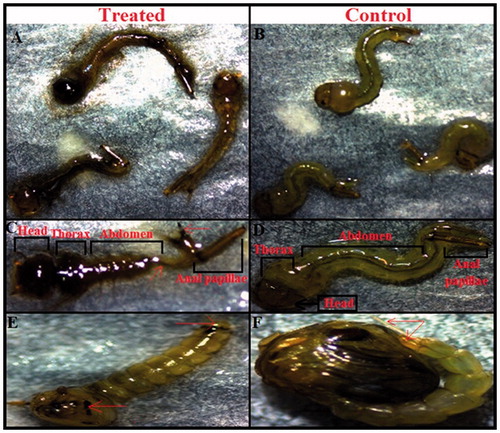
According to the oxidation state of the silver atom is 1, 2, 3, and 4. In the first, silver nanoparticles connected with the -NH of acetamide of the chitin and also can be connected with the oxygen atom, which made the surface of the insect very weak due to the inhibition of hydrogen bond formation. Inside the insect, the free-NH of the imidazole ring can also be connected with the silver atom made another lean and kill the insect (). The mechanism of the insect’s death lies on the ability of nanoparticles to penetrate the larval membrane, this penetration may promote silver ion to enter the cell membrane, which led to the death of the insect (Choi et al. Citation2008; Kvitek et al. Citation2008).
Antimicrobial efficiency of nanoparticles and Bacillus strains
The results showed that the different concentrations of AgNPs and Bacillus strains (5, 10, 15, and 20 μL) significantly disrupt the growth of the pathogen compared with untreated control double distilled water (). The highest concentration (20 μL) of treatments D29-AgNPs and A15-AgNPs gave the highest means of inhibition diameter of 25.00 and 23.33 mm, respectively, but using Bacillus strains (D29 and A15) without silver ion give us lower inhibition zone 16.70 and 14.90 mm, respectively, of efficacy against the pathogen growth (). This clear zone indicates the antimicrobial efficiency of AgNPs and Bacillus strains. The inhibition growth of pathogen indicates the release of silver ion from the AgNPs with the ability of Bacillus strains as bio-control agents and together diffusion into the agar layer, preventing the growth of pathogen colonies in the agar medium. The mechanism of antagonistic effect in the plate may be due to antibiosis or production of siderophores or both of them (Lemessa and Zeller Citation2007). These results are similar with previous studies conformed the antagonistic activity of Bacillus strains against deferent phyto-pathogens (Almoneafy et al. Citation2012; El-Shakh et al. Citation2015). The antibacterial activity of AgNPs may be because of close attachment of the nanoparticle surface with microbial cells. It is reported that during anti-bacterial effect bio-silver highly interacted with sulfur and phosphorus, which are found in abundance throughout the cell membrane and utilize these essential elements for displaying antibacterial properties (Guzman et al. Citation2012). Furthermore, synthesized silver nanoparticles successfully controlled the human pathogenic and fish pathogenic bacteria in vitro (Rawani et al. Citation2013).
Figure 8. Zones of inhibition of reaction mixture containing silver nanoparticles and B. subtilis A15 and B. amyloliquefaciens D29 against Xanthomonas oryzae pv. oryzae growth on LBA medium.
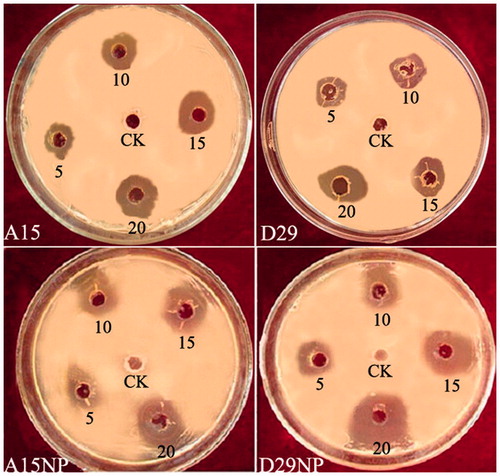
Figure 9. In vitro inhibitory effect (mm) of two bacterial strains (A15 and D29) and (AgNPs) against Xanthomonas oryzea growth on Luria-Bertani agar (LBA) medium after 48 h incubation at 28 ± 2 °C in laboratory. Columns with the different letters indicate significant differences (P < .05) among treatments, Error bars in each column represent the standard error within same treatment.
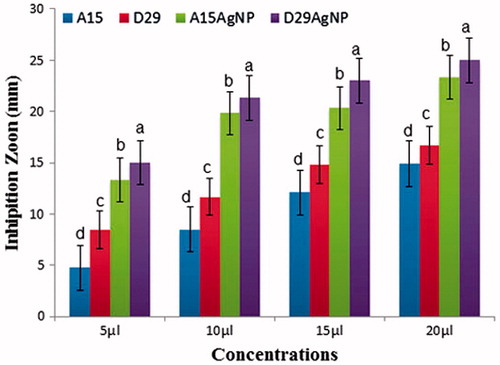
Conclusion
The synthesized silver nanoparticles by the cell-free supernatant from B. subtilis (A15) and B. amyloliquefaciens (D29) were regarded as one of the strongest compounds to control the pests. Both types of bacteria have shown a great ability to convert the silver-ion-charged to silver-ion-free to make this process handy and cost-effective to produce on a large scale/commercial level. As far as we know, this is the first study on the synthesis of silver nanoparticles using B. subtilis and B. amyloliquefaciens to control the mosquito colonies of Culex pipiens pallens, and having antibacterial activities. Where, this compound is non-harmful to humans, clean and simple (Sundaravadivelan and Padmanabhan Citation2014). As a result, our study recommends expanding the use of synthesized bacteria-AgNPs in mosquito and pathogenic bacteria control programs.
Ethical statement
All applicable international, national, and/or institutional guidelines for the care and use of animals were followed.
Disclosure statement
The authors declare that they have no conflict of interest.
References
- Abbott W. 1925. A method of computing the effectiveness of an insecticide. J Econ Entomol. 18:265–267.
- Almoneafy AA, Xie G, Tian W, Xu L, Zhang G, Ibrahim M. 2012. Characterization and evaluation of Bacillus isolates for their potential plant growth and biocontrol activities against tomato bacterial wilt. Afr J Biotechnol. 11:7193–7201.
- Banu AN, Balasubramanian C. 2015. Extracellular synthesis of silver nanoparticles using Bacillus megaterium against malarial and dengue vector (Diptera: Culicidae). Parasitol Res. 114:4069–4079.
- Banu AN, Balasubramanian C, Moorthi PV. 2014. Biosynthesis of silver nanoparticles using Bacillus thuringiensis against dengue vector, Aedes aegypti (Diptera: Culicidae). Parasitol Res. 113:311–316.
- Benelli G. 2015. Research in mosquito control: current challenges for a brighter future. Parasitol Res.114:2801–2805.
- Benelli G. 2016. Plant-mediated biosynthesis of nanoparticles as an emerging tool against mosquitoes of medical and veterinary importance: a review. Parasitol Res. 115:23–34.
- Benelli G, Mehlhorn H. 2016. Declining malaria, rising of dengue and Zika virus: insights for mosquito vector control. Parasitol Res. 115:1747–1754.
- Chen YT, Yuan Q, Shan LT, Lin MA, Cheng DQ, Li CY. 2013. Antitumor activity of bacterial exopolysaccharides from the endophyte Bacillus amyloliquefaciens sp. isolated from Ophiopogon japonicus. Oncol Lett. 5:1787–1792.
- Choi O, Deng KK, Kim NJ, Ross L, Surampalli RY, Hu Z. 2008. The inhibitory effects of silver nanoparticles, silver ions, and silver chloride colloids on microbial growth. Water Res. 42:3066–3074.
- Chowdhury SP, Hartmann A, Gao X, Borriss R. 2015. Biocontrol mechanism by root-associated Bacillus amyloliquefaciens FZB42 - a review. Front Microbiol. 6:780.
- De-Jian S, Xu LD, Ji HD. 2013. The history of the elimination of lymphatic filariasis in China. Infect Dis Poverty. 2:30.
- Deepak P, Sowmiya R, Ramkumar R, Balasubramani G, Aiswarya D, Perumal P. 2016. Structural characterization and evaluation of mosquito-larvicidal property of silver nanoparticles synthesized from the seaweed, Turbinaria ornata (Turner) J. Agardh 1848. Artif Cells Nanomed Biotechnol. 6:1–9.
- Dhanasekaran D, Thangaraj R. 2013. Evaluation of larvicidal activity of biogenic nanoparticles against filariasis causing Culex mosquito vector. Asian Pac J Trop Dis. 3:174–179.
- Du J, Singh H, Yi TH. 2016. Biosynthesis of silver nanoparticles by Novosphingobium sp. THG-C3 and their antimicrobial potential. Artif Cells Nanomed Biotechnol. 5:1–7.
- El-Shakh AS, Kakar KU, Wang X, Almoneafy AA, Ojaghian MR, Li B, Anjum SI, Xie G-L. 2015. Controlling bacterial leaf blight of rice and enhancing the plant growth with endophytic and rhizobacterial Bacillus strains. Toxicol Environ Chem. 97:766–785.
- Finney DJ. 1971. Probit analysis. 3rd ed. London: Cambridge University Press, pp. 68–72.
- Gole A, Dash C, Ramakrishnan V, Sainkar S, Mandale A, Rao M, Sastry M. 2001. Pepsin-gold colloid conjugates: preparation, characterization, and enzymatic activity. Langmuir. 17:1674–1679.
- Gopinath V, Velusamy P. 2013. Extracellular biosynthesis of silver nanoparticles using Bacillus sp. GP-23 and evaluation of their antifungal activity towards Fusarium oxysporum. Spectrochim Acta Part A Molec Biomolec Spectrosc. 106:170–174.
- Guzman M, Dille J, Godet S. 2012. Synthesis and antibacterial activity of silver nanoparticles against gram-positive and gram-negative bacteria. Nanomed Nanotechnol BiolMed. 8:37–45.
- Hop DV, Phuong HT, Quang ND, Ton PH, Ha TH, Hung NV, et al. 2014. Biological control of Xanthomonas oryzae pv. oryzae causing rice bacterial blight disease by Streptomyces toxytricini VN08-A-12, isolated from soil and leaf-litter samples in Vietnam. Biocontrol Sci. 19:103–111.
- Janeiro P, Corduneanu O, Oliveira AM. 2005. Chrysin and (±)‐taxifolin electrochemical oxidation mechanisms. Electroanalysis. 17:1059–1064.
- Kanchana A, Agarwal I, Sunkar S, Nellore J, Namasivayam K. 2011. Biogenic silver nanoparticles from Spinacia oleracea and Lactuca sativa and their potential antimicrobial activity. Digest J Nanomat Biostruct. 6:1741–1750.
- Kim SW, Kim KS, Lamsal K, Kim Y-J, Kim SB, Jung M, et al. 2009. An in vitro study of the antifungal effect of silver nanoparticles on oak wilt pathogen Raffaelea sp. J Microbiol Biotechnol. 19:760–764.
- Kvitek L, Panáč A, Soukupova J, Kolar M, Vecerova R, Prucek R, Holecova M, Zboril R. 2008. Effect of surfactants and polymers on stability and antibacterial activity of silver nanoparticles (NPs). J Phys Chem C. 112:5825–5834.
- Lallawmawma H, Sathishkumar G, Sarathbabu S, Ghatak S, Sivaramakrishnan S, Gurusubramanian G, Kumar NS. 2015. Synthesis of silver and gold nanoparticles using Jasminum nervosum leaf extract and its larvicidal activity against filarial and arboviral vector Culex quinquefasciatus Say (Diptera: Culicidae). Environ Sci Pollut Res Int. 22:17753–17768.
- Laspidou CS, Rittmann BE. 2002. A unified theory for extracellular polymeric substances, soluble microbial products, and active and inert biomass. Water Res. 36:2711–2720.
- Lee KJ, Kamala-Kannan S, Sub HS, Seong CK, Lee GW. 2008. Biological control of Phytophthora blight in red pepper (Capsicum annuum L.) using Bacillus subtilis. World J Microbiol Biotechnol. 24:1139–1145.
- Lee KJ, Park SH, Govarthanan M, Hwang PH, Seo YS, Cho M, et al. 2013. Synthesis of silver nanoparticles using cow milk and their antifungal activity against phytopathogens. Mater Lett. 105:128–131.
- Lemessa F, Zeller W. 2007. Screening rhizobacteria for biological control of Ralstonia solanacearum in Ethiopia. Biol Control. 42:336–344.
- Li H, Soares MA, Torres MS, Bergen M, White JF. 2015. Endophytic bacterium, Bacillus amyloliquefaciens, enhances ornamental hosta resistance to diseases and insect pests. J Plant Interact. 10:224–229.
- Mageswari A, Subramanian P, Ravindran V, Yesodharan S, Bagavan A, Rahuman AA, Karthikeyan S, Gothandam KM. 2014. Synthesis and larvicidal activity of low-temperature stable silver nanoparticles from psychrotolerant Pseudomonas mandelii. Environ Sci Pollut Res Int. 22:5383–5394.
- Mohan L, Sharma P, Srivastava C. 2006. Evaluation of Solanum xanthocarpum extract as a synergist for cypermethrin against larvae of the filarial vector Culex quinquefasciatus (Say). Entomol Res. 36:220–225.
- Monteiro L, Mariano LR, Souto-Maior AM. 2005. Antagonism of Bacillus spp. against Xanthomonas campestris pv. campestris. Braz Arch Biol Technol. 48:23–29.
- Mubarak Ali D, Gopinath V, Rameshbabu N, Thajuddin N. 2012. Synthesis and characterization of CdS nanoparticles using C-phycoerythrin from the marine cyanobacteria. Mater Lett. 74:8–11.
- Muthukumaran U, Govindarajan M, Rajeswary M, Hoti SL. 2015. Synthesis and characterization of silver nanoparticles using Gmelina asiatica leaf extract against filariasis, dengue, and malaria vector mosquitoes. Parasitol Res. 114:1817–1827.
- Nam HS, Oh BJ, Kim YC. 2016. Biological control potential of Bacillus amyloliquefaciens KB3 isolated from the feces of Allomyrina dichotoma larvae. Plant Pathol J. 32:273–280.
- Padman AJ, Henderson J, Hodgson S, Rahman PK. 2014. Biomediated synthesis of silver nanoparticles using Exiguobacterium mexicanum. Biotechnol Lett. 36:2079–2084.
- Panneerselvam C, Murugan K, Kovendan K, Kumar PM, Subramaniam J. 2013. Mosquito larvicidal and pupicidal activity of Euphorbia hirta Linn. (Family: Euphorbiaceae) and Bacillus sphaericus against Anopheles stephensi Liston. (Diptera: Culicidae). Asian Pac J Trop Med. 6:102–109.
- Pawar O, Deshpande N, Dagade S, Waghmode S, Nigam JP. 2016. Green synthesis of silver nanoparticles from purple acid phosphatase apoenzyme isolated from a new source Limonia acidissima. J Exp Nanosci. 11:28–37.
- Perumalsamy H, Chang KS, Park C, Ahn YJ. 2010. Larvicidal activity of Asarum heterotropoides root constituents against insecticide-susceptible and -resistant Culex pipiens pallens and Aedes aegypti and Ochlerotatus togoi. J Agric Food Chem. 58:10001–10006.
- Rawani A, Ghosh A, Chandra G. 2013. Mosquito larvicidal and antimicrobial activity of synthesized nano-crystalline silver particles using leaves and green berry extract of Solanum nigrum L. (Solanaceae: Solanales). Acta Tropica. 128:613–622.
- Roni M, Murugan K, Panneerselvam C, Subramaniam J, Nicoletti M, Madhiyazhagan P, et al. 2015. Characterization and biotoxicity of Hypnea musciformis-synthesized silver nanoparticles as potential eco-friendly control tool against Aedes aegypti and Plutella xylostella. Ecotoxicol Environ Saf. 121:31–38.
- Sanghi R, Verma P. 2009. Biomimetic synthesis and characterisation of protein capped silver nanoparticles. Biores Technol. 100:501–504.
- Santhosh SB, Ragavendran C, Natarajan D. 2015. Spectral and HRTEM analyses of Annona muricata leaf extract mediated silver nanoparticles and its larvicidal efficacy against three mosquito vectors Anopheles stephensi, Culex quinquefasciatus, and Aedes aegypti. J Photochem Photobiol B Biol. 153:184–190.
- Sap-Iam N, Homklinchan C, Larpudomlert R, Warisnoicharoen W, Sereemaspun A, Dubas S. 2010. UV irradiation-induced silver nanoparticles as mosquito larvicides. J Appl Sci. 10:3132–3136.
- Shah AT, Din MI, Bashir S, Qadir MA, Rashid F. 2015. Green synthesis and characterization of silver nanoparticles using Ferocactus echidne extract as a reducing agent. Analyt Lett. 48:1180–1189.
- Soni N, Prakash S. 2012. Efficacy of fungus mediated silver and gold nanoparticles against Aedes aegypti larvae. Parasitol Res. 110:175–184.
- Soni N, Prakash S. 2014. Microbial synthesis of spherical nanosilver and nanogold for mosquito control. Ann Microbiol. 64:1099–1111.
- Suganya G, Karthi S, Shivakumar MS. 2014. Larvicidal potential of silver nanoparticles synthesized from Leucas aspera leaf extracts against dengue vector Aedes aegypti. Parasitol Res. 113:875–880.
- Sundaravadivelan C, Padmanabhan MN. 2014. Effect of mycosynthesized silver nanoparticles from filtrate of Trichoderma harzianum against larvae and pupa of dengue vector Aedes aegypti L. Environ Sci Pollut Res Int. 21:4624–4633.
- Thompson D, Clarke B, Kobayashi D. 1996. Evaluation of bacterial antagonists for reduction of summer patch symptoms in Kentucky bluegrass. Plant Dis. 80:856–862.
- Udayasoorian C, Kumar R, Jayabalakrishnan M. 2011. Extracellular synthesis of silver nanoparticles using leaf extract of Cassia auriculata. Dig J Nanomater Biostruct. 6:279–283.
- Velu K, Elumalai D, Hemalatha P, Janaki A, Babu M, Hemavathi M, Kaleena PK. 2015. Evaluation of silver nanoparticles toxicity of Arachis hypogaea peel extracts and its larvicidal activity against malaria and dengue vectors. Environ Sci Pollut Res Int. 22:17769–17779.
- Wang C, Kim YJ, Singh P, Mathiyalagan R, Jin Y, Yang DC. 2015. Green synthesis of silver nanoparticles by Bacillus methylotrophicus, and their antimicrobial activity. Artif Cells Nanomed Biotechnol. 44:1127–1132.
- WHO. 2004. Estimated incidence, prevalence and TB mortality. Geneva: WHO. Available from: http://www.who.int/mediacentre/factsheets/fs104/en
- WHO. 2014. Lymphatic filariasis. Fact Sheet No 102. Available from: http://www.searo.who.int/thailand/factsheets/fs0012/en/
- WHO. 2015. Lymphatic filariasis fact sheet. Available from: http://www.who.int/mediacentre/factsheets/fs102/en/