Abstract
Mucosal administration of vaccine is most prevalent way to induce desired immunity against various types of antigen and microbial in central and in addition, the peripheral blood in most external mucosal surface. Mucosal delivery of vaccine provides both humoral and cellular responses against mucosal infection. Mucosa, which are exposed to heavy loads of commensal and pathogenic microorganism, are one of the main region where infections are built up, also, thus have frontline status in immunity, making mucosa perfect site for vaccines application. The nasal route is favoured over parenteral route due to ease of administration, protection of antigen from degradation and induces sIgA which is not produced by systemic immunity. Natural and synthetic polymers are utilized to get nanoparticles carrier systems for development of nasal mucosal antibodies. The present review summarized the recent development in the field of vaccine delivery by means of mucoadhesive polymeric carriers. This review also describes the recent patent conceded for mucosal immunization utilizing these polymeric carriers.
Introduction
Mucosal immunization is a needle-free method of vaccine delivery. Mucosal surfaces cover ∼400 m2 of the human body surface; furthermore, work both as physical obstructions and in insusceptible guard against pathogen intrusion [Citation1]. The versatile invulnerable framework is intended to recognize antigens, pathogens and antibodies that enter the body through mucosal surfaces from those that are presented specifically into tissues or the circulation system by infusion on the other hand damage. It is turning out to be progressively obvious that nearby mucosal safe reactions are imperative for insurance against illness: for instance, mucosal antibodies against Vibrio cholerae microorganisms and hepatitis B [Citation2]. Studies show that acceptance of systemic immune through parenteral immunization can impact only systemic infection, but it fails to give the mucosal surfaces. Mucosal vaccine administration can induce immune responses at both systemic and mucosal sites. The induction of mucosal response leads to production of secretory IgA (sIgA) which cannot be produced by systemic immunity [Citation3].
This mucosal-associated lymphoid tissue (MALT) lies along the mucosa of the respiratory, gastrointestinal, and genitourinary tracts as totals as bronchus-related lymphoid tissue (BALT), gut-associated lymphoid tissue (GALT) and nasal-associated lymphoid tissue (NALT) [Citation4]. The GALT is represented by the Peyer’s patches (PP), the appendix and small solitary lymphoid nodules connected with microfold (M) cells, antigen-exhibiting cells, microphages and dendritic cells; CD4+ and CD8+ T cells and B cells. These are the effector organs, which evoke mucosal insusceptible reaction. The major characteristic of mucosal immunity is to deliver local insusceptible respond as sIgA which check the contamination of epithelial host [Citation5]. Mucosal surfaces are grouped into two sorts in view of their distinct attributes. Sort I mucosal surfaces are found in the gut alimentary, respiratory and female upper reproductive system, whereas sort II mucosal surfaces are found in the visual, mouth alimentary and female lower reproductive system. Sort I mucosal surfaces have a basic columnar epithelial layer in which IgA is the real immunoglobulin (Ig) isotype. Interestingly, sort II mucosal surfaces have a stratified squamous epithelial layer, and the real Ig isotype is IgG [Citation6] ().
Table 1. Comparison of parenteral and mucosal administration of vaccine.
The mucosal invulnerable framework has three fundamental capacities:
(i) To secure the mucosal layers against colonization and intrusion by possibly risky organisms that might be experienced.
(ii) To forestall take-up of undegraded antigens including outside proteins got from ingested nourishment, airborne matter and commensal microorganisms, and
(iii) To keep the advancement of conceivably hurtful insusceptible reactions to these antigens in the event that they do achieve the body inside.
Role of M cells in mucosal immunization
M cells have unique ability to take up antigen from lumen of small intestine via endocytosis, phagocytosis and transcytosis [Citation7]. Antigen delivered to antigen-presenting cells (APCs). M cells are specific epithelial cells shaping part of the follicle-related epithelium. As opposed to their neighbouring columnar epithelial cells, M cells are morphologically special because of the non-attendance of surface microvilli which are normal for the intestinal epithelial cells [Citation8]. Rather, the apical layer of the M cell has a microfold (or membranous) topography for the successful take-up of ingested antigens from luminal destinations. Like other epithelial cells, M cells frame tight intersections to keep up a hindrance work. The basolateral film of M cells is invaginated, and structures many “pockets”, which harbour penetrating lymphocytes. The arrangement of these “pockets” enormously diminishes the intracellular separation that antigens need to travel and permits M cells to quickly transport (inside 10–15 min) antigenic materials to the basolateral layer. The larger part of GALT is composed into totals of lymphoid follicles called as PP [Citation9]. Antigens, particulate carriers, microscopic organisms, infections and protozoa are transported into PP by specific epithelial cells called M cells. The take-up and transport of antigens by M cells is identified with two viewpoints; in the first, antigen will most likely escape degradation and second, the antigen will be discharged into a situation rich in immunocompetent cells. Thus, the take-up by M cells permits the conveyance of in place antigen into the safe inductive environment of the PP and limits the entrance of antigens to option ranges of the digestive tract, where suppressor T cells prevail. Thus, antigen in the wake of being taken up by M cells incites strong immune response, whereas the antigens that are taken up by common enterocytes will probably actuate systemic tolerance [Citation10].
The induction of potent immune responses
Taking after intranasal inoculation, humoral- and cell-insusceptible reactions can be created. Mucoadhesive particles, for example, poly(lactic-co-glycolic acid) (PLGA), chitosan (CS), hyaluronan, and so on and particulates bearer frameworks indicate impressive bioadhesion to the mucosal surface and they could subsequently be viable for the intercession of mucosal connection and maintenance of micro sized and nanosized carrier(s) to the nasal mucosal surface [Citation11]. Thereby subsequent transcytosis of these carrier systems could ensure the delivery the antigen to the NALT where the effective localization of antigens to the APCs is possible via phagolysosomal pathway of antigen uptake, which subsequently presents the antigen in association with MHC II and elicits humoral immune response against the antigen (Pathway I, ). This mucosal conveyance of antigen could likewise be compelling in conveying the antigen to the cytosol of APCs show in the NALT. In cytosol, proteasomes interceded preparing of antigens happens and therefore these peptides are transported to the lumen of endoplasmic reticulum (ER) by transporters of antigen presentation (TAP). Inside the ER, peptides connect with MHC I molecules, which therefore transported, by means of Golgi complex, to the plasma film. This film bound complex initiates CD8+ cells, and induces cell immune response (Pathway II, ) bringing about the arrangement of cytotoxic T lymphocytes (CTL) [Citation12,Citation13].
Novel adjuvants for mucosal immunization
Several groups have attempted to develop adjuvant-free nasal vaccines, mucosal adjuvant for bacteria origin like cholera toxins (CT) and heat labile enterotoxin (LT) [Citation14]. Most protein antigens are poor immunogens, when given mucosally, as well as regularly initiate resistance rather than immunity. Although some microbial segments, for example, the CT or the non-toxic binding parts of these toxins (CT-B) have been observed to be intense mucosal immunogens evoking strong secretory and circulating antibodies. Notwithstanding being a good mucosal immunogens, CT is an intense adjuvant [Citation15,Citation16].
Other various approaches have been applied to improve their immunogenicity, which include PLGA microparticles, CS bioadhesive polysaccharides, liposomes and genetically modified whole virus.
Mechanisms of mucosal immune response against infection
The prime aim of an effective vaccine is to identify the immunogens and is processed in such a way that the vaccine induces the sustain immune response in mucosal and systemic tissues. In order to prevent intracellular pathogen mucosal immunizer IgA and IgG act to incite the cell-related responses with the CD8 + cytotoxic and CD4 + T cells [Citation17]. GALT, an immunological hindrance, secures the intestinal mucosa. It is an epithelial single layer hindrance, in which enterocytes mixed by the mucus discharging goblet cells. The GALT comprises of bunch of lymphoid follicles known as PP, and serves as mucosal inductive sites for an immune response. The antigen is presented by the APCs to B and T lymphocytes. To recognize the antigen, the Toll-like receptors are available at the surface of APCs, this prompt to the incitement of T cells (CD4 + T-assistant cells and CD8 + CTL) and IgA-precursor cells in PP and the spreading of B cells and T cells to mucosal effectors cells/tissue, for example, mesenteric lymph nodes and lamina propria, in this part the lymphocyte will additionally separate and maturate, and in next part they will move into systemic circulation through the thoracic duct and to organ including the liver and spleen [Citation18]. Different events involved in era of immune responses upon mucosal vaccination are represented as .
Figure 2. Schematic representation of pathways eliciting a local mucosal response and a systemic response through NALT and nasal mucosa. APCs: antigen-presenting cells (macrophages, dendritic cells); M: microfold epithelial cell; NALT: nasal-associated lymphoid tissue; PCLN: posterior cervical lymph nodes; SCLN: superior cervical lymph node.
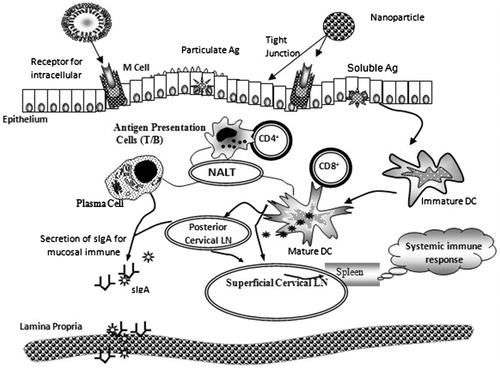
Extra adjuvants like alum alongside particles have been utilized to enhance the immune response while inoculating with polymeric particle formulation [Citation20]. Immunization with poly(lactic acid) (PLA) particles evoked fundamentally higher anti-TT antibody titers as compared to PLGA particles. These perceptions can be clarified on the premise that PLA is more hydrophobic compared to PLGA due to the presence of an additional -CH3 group in lactic acid. In view of this hydrophobicity factors, APCs are specially pulled in towards PLA particles and this helps in inspiring enhanced immune response. The superiority of hydrophobic polymer particles in enhancing immunogenicity of polymer-entrapped antigen has been accounted for reported for Influenza A vaccines [Citation21] for ovalbumin [Citation22] and TT [Citation23].
A single-shot vaccine can be made utilizing microspheres as adjuvants or vehicles, from which the encapsulated antigens are discharged under control over a prolong period by controlling the polymer degradation rate, would be of extraordinary advantage for developing countries in which health conditions are poor and drop-out rates between the first and the last immunization are particular tissue, cell type or sub-cellular compartment [Citation24]. For example, the antigen-examining M cells might be focused on particulate frameworks or lections [Citation25]. Vaccine viability could be improved by anchoring M-cell particular ligands (e.g. lectins) on to the bioactive carriers. The most generally concentrated particular bioadhesives are lectin-like molecules of plant or bacterial origin. Lectins are proteins or glycoproteins of non-immunological origin that attached to sugar structures particularly and with moderately high affinities [Citation26]. The mucosal surfaces are exceedingly glycosylated and along these link for particular ligands. Besides, there is proof that lectins might be translocated over the epithelium and induce immune responses to conjugated or co-administered antigens. Lectin that binds particularly to the epithelial cells might be utilized to target conjugated antigens or might be joined to microparticles and other conveyance frameworks, for example, liposomes to improve association with epithelia [Citation27].
Bovine serum albumin (BSA) was chosen as a model antigen, though, recombinant hepatitis B surface antigen (HBsAg) was picked as hopeful antigen for novel carrier-based vaccine development.
Change in immune response from physical blend of various particles can be credited to the combined adjuvant effects of both sorts of particles. The appearance of more particles gives a higher surface range which may help in adsorbing the burst – discharged antigens from the particles and introduce these surface adsorbed antigens through MHC II pathways to enhance immune response [Citation28]. Blend, that is, mixtures of PLA polymer particles, due to the high adsorption of protein into the surface and higher hydrophobicity, evoked much higher antibody response.
Poly(lactide-co-glycolide) PLGA polymer is biocompatible and biodegradable. It has been utilized as basic material with a well-established safety profile in people. Controlled release drug delivery systems utilizing this biomaterial have been approved for human use [Citation29]. A few reviews have demonstrated that PLGA micro- and nanoparticles can be utilized to balance immune responses against encapsulated antigens because to their capacity to efficiently target professional APCs and to encourage appropriate processing and presenting antigens to T cells [Citation30,Citation31].
Role of mucoadhesive polymers in mucosal delivery
In the mid of 1980s, the possibility of mucoadhesive, was exhibited into the controlled medication conveyance. Mucoadhesive is characterized as the capacity of a material to stick to the mucosal layer [Citation33]. Nonetheless, during mucus secretion, they would definitely be pushed facilitate from the surface of the mucosa and in the end be evacuated by the luminal substance. Larger particles could be pulled from the mucosal surface because of their individual weight, or expelled during the motility of the stomach.
Various polymers used for mucosal administration of vaccines
Biodegradable polymers are having many advantages as effective/particular conveyance bearers for proteins and peptides [Citation36]. The primary criteria managing polymer qualification for mucosal delivery conveyance have been bioavailability, biocompatibility, straightforward and degeneration rate, which give a maintained arrival of antigen. Polymeric materials used for production of microparticles/nanoparticles incorporate synthetic or natural polymers. Polyesters, alone or in combination with different polymers, are the most generally utilized for the formulation of nanoparticles. Recently, formulation based on natural polymers has been produced. Up to now, CS is the most esteemed, unquestionably because of its penetrability enhancer capacities and to its properties, permitting the preparation of organic solvent-free mucoadhesive particles [Citation37] ().
Table 2. Classification of mucoadhesive polymers.
Natural polymers
Chitosan and alginate
Until now, most hydrophilic and macromolecules drug-like peptides, protein and polysaccharides need to injected due to low absorption at mucosal sites. Polymers, for example, alginate and CS have been portrayed as biocompatible, biodegradable and mucoadhesive, empowering various pharmaceutical and biomedical applications [Citation38] including the design of controlled release device. CS is cationic polysaccharides obtained by the deacetylation of chitin, compound of exoskeletons in crustaceans. CS [α(1-4) 2-amino 2-deoxy β-D glucan] is a mucopolysacharide closely related to cellulose, whose chemical properties are determined by the molecular to molecular weight and degree of deacetylation for weight, degree of deacetylation and viscosity. It was firstly portrayed by Rouget in 1859; however, it took until 1894 preceding it was formally named by Hoppe-Seyler [Citation39] regarding mucosal drug delivery, and CS appear mucoadhesive properties [Citation40]. In addition, connection of the positively charged amino group at the C-2 position of CS with the negatively charged sites on the cell surfaces and tight intersections permits paracellular transport of extensive hydrophilic compound by too opening the tight intersections of mucosal layer hindrances [Citation41].
Alginate is an anionic polymer involved varying extends of 1,4-connected β-D-mannuronic corrosive (M), α-L-guluronic acid (G), and alternating (MG) blocks. Alginate is a safe, non-immunogenic, biodegradable, and biocompatible and mucoadhesive polymer which makes it reasonable possibility for mucosal protein/antigen conveyance [Citation42].
Patents
In Illum et al.’s study, their patent is directed towards a novel nasal vaccine composition that uses the cationic polysaccharide, CS as a delivery system. CS is a polysaccharide comprising copolymers of glucosamine and N-acetyl glucosamine. It can be concluded that nasal administration of CS-antigen nasal vaccines induced significant serum IgG responses and sIgA levels. Animals vaccinated via the nasal route with various CS-antigen vaccines were also found to be protected against the appropriate challenge [Citation43].
In Sava et al.’s study, their patent relates to microcapsules with a double layer of natural polysaccharides CS and alginate-gelified and stabilized by means of a divalent ion containing inside at least one biologically active substance [Citation44].
In Fernandez et al.’s study, their patent relates to nanoparticle systems for the release of biologically active molecules formed by the CS polymer or its derivatives, chemically modified with polyethylene glycol and cross-linked with a cross-linking agent. These systems are particularly valuable for pharmaceutical composition, immunizations and cosmetic formulation [Citation45].
Gelatin
Gelatin is a natural and biodegradable protein and, it is well known that gelatin is a product of the structural and chemical degradation of collagen. It is a different mixture of single- or multi-stranded polypeptides made mainly of glycine, proline and hydroxyproline remains and is deteriorate in vivo into component amino acids. PEGylation of the particles fundamentally improves their flow time in the bloodstream circulation system and builds their take-up into cells by endocytosis. Antibody-altered gelatin nanoparticles have been utilized for focused take-up by lymphocytes [Citation46].
Patent
Published in PCT application US2000/U38843, the present invention is directed to the use of gelatins in vaccines. The gelatin of the present invention is recombinant gelatin consisting of polypeptides of uniform molecular weight, said gelatin being a homogenous mixture of identical molecules. Therefore, in one embodiment, the present invention provides a vaccine formulation comprising the recombinant gelatin. In a preferred embodiment, the recombinant gelatin is human gelatin [Citation47].
Lawter invented a formulation containing tetracycline and at least one cationic polymer and/or mucoadhesive material like gelatin and formulated different dosage forms liquid or solid dosage forms, such as mouth rinse or tablet, causes prolonged retention of the tetracycline in the mucosa for oral cavity [Citation48].
Hyaluronic acid
Hyaluronic acid is a polymer, composed of two sugars that our cells produce throughout their lives (N-acetyl-glucosamine-6-phosphate and glucuronic acid). Bioadhesive polymer got from hyaluronic acid has been appeared to be an effective delivery system for i.n. vaccines. The utilization of hyaluronic acid polymers provides a protected and powerful approach for improving the immunogenicity of a wide assortment of antigens [Citation49].
Patent
In O’Hagan et al.’s study, their patent provides a successful strategy to eliciting an immune response in a mammalian subject utilizing mucosal immunization and hyaluronic acid conveyance methods. The present development depends on the discovery that the mucosal conveyance of hyaluronic acid derivatives, for example, esterified hyaluronic acid polymers and auto-cross-linked hyaluronic acid polymers, in mix with an antigen of interest, and alternatively an adjuvant, act to improve the immunogenicity of the antigen co-administered therewith [Citation50].
Polyphosphazenes and polyanhydrides
Polyphosphazenes are a one of a kind class of degradable polymers in that their backbone is totally inorganic comprising of phosphorous and nitrogen bonded directly through rotating single and double bonds. Polyanhydrides are a class of surface dissolving polymers that contain two carbonyl gatherings bound together by an ether bond and have been solely studied for biomedical applications [Citation51]. One especially exceptional property of polyanhydrides is that the degradation of the anhydride bond is very subject to polymer backbone chemistry. While polyphosphazenes and polyanhydrides has been extensively investigated for drug delivery applications [Citation52].
Patent
In Andrianov et al.’s study, their patent was disclosed that an intradermally administered product comprising an antigen and an adjuvant comprising a polyphosphazene polyelectrolyte capable of eliciting an immune response, in a human or in an animal, against the antigen [Citation53].
Pollulan
Pullulan is water-soluble, linear polysaccharides that consist of three α-1,4-linked glucose molecules polymerized by α-1,6 linkages on the terminal glucose. Pullulan is a fermentation product of the yeast Aureobasidium pullulans [Citation54].
Patent
Ni and Yates in their innovation revealed herein relate to the delivery of physiologically active agents to the tissues or body fluids of animals, including humans. The composition of the invention can be administered to the animal and its tissues and body fluids in the form or liquids, or solids, or powders comprising microspheres or microparticles of selected size ranges [Citation55].
A recent patent has described that bioactive agent can be encapsulated in the microparticles composed of biodegradable polymer pullulan. It was mentioned in one embodiment of the patent that these medical articles can be useful for delivering bioactive agents to a treatment site within a body, such as the eye [Citation56].
Synthetic polymers
PLGA and PLA
Polyester PLGA is a copolymer of PLA and poly(glycolic acid) (PGA). PLGA has been among the most appealing polymeric hopefuls used to manufacture gadgets for medication conveyance. PLGA is biocompatible and biodegradable shows an extensive variety of disintegration times has tunable mechanical properties and in particular is a FDA-approved polymer. Specifically, PLGA has been widely considered for the advancement of gadgets for controlled conveyance of little particle medications, proteins and different macromolecules in business utilize and in research. PLGA has indicated tremendous potential as a medication conveyance transporter. PLGA is most prominent among the others available biodegradable polymers on account of its long clinical experience, and potential outcomes for supported medication conveyance for sustained drug delivery [Citation57]. Following mucosal organization, PLGA micro/nanoparticles are taken up by M cells and permit ricted T cells. PLGA-based carrier systems may therefore be important mucosal adjuvants for the induction of cellular immune responses to intracellular pathogens. PLG microsphere in the intestine is that antigens have a tendency to be discharged from the polymer network with an underlying subsequent burst. For successful immunization, the particle should retain its cargo until it achieves PP resigns in the ileum, or ideally until uptake by M cells have been accomplished. A few methodologies including polymeric coatings or encapsulation are right now being assessed for the improvement of mucosal conveyance of vaccines [Citation58].
Patents
In Sokoll et al.’s study, their patent coordinated towards the creation of a novel and significant polymer that has properties suitable for manufacturing by different procedures into microparticles and microspheres. In this innovation, alteration of existing processing procedures results in significant improvement in encapsulation efficiencies [Citation59].
In Reid et al.’s study, their patent relates to a novel pharmaceutical composition, a microcapsule/sphere formulation, which involves an antigen encapsulated inside a biodegradable polymeric matrix such as poly(DL-lactide-co-glycolide) (DL-PLG), wherein the relative proportion between the lactide and glycolide component of the DL-PLG is inside the range of 40:60 to 0:100, and its utilization, as a vaccine, in the effective pretreatment of animals (including humans) to prevent intestinal infections caused by a virus or bacteria [Citation60].
Poly(ɛ-caprolactone)
Poly-ɛ-caprolactone (PCL) is a one of the biodegradable polymers, which has a long time of degradation and these incorporate polymers such as PGA, poly-lactide (PLLA) and their copolymer. It is a semicrystalline polymer due to its regular structure, and its melting temperature is above body temperature (59–64 °C), but its Tg is −60 °C, so in the body the semicrystalline structure of PCL results in high durability, because the amorphous domains are in the rubbery state. PCL is prepared by ring opening polymerization of ɛ-caprolactone using a catalyst for example stannous octoate. Since PCL degrades slowly, it has been utilized in blends and copolymers with other biodegradable polymers [Citation61].
Patents
Grandfils et al. reported that biopolymer nanoparticles made out of aliphatic polyester polymer, for example, polycaprolactone, PLA and PLGA, can be appropriately used for preserving the activity of drug administered while in the meantime controlling the release of the medication from the particles [Citation62].
Furthermore, patent depicted the utilization of polymeric microparticles including biodegradable polymers with the goal of immunization. It is revealed in this innovation that polymer microparticles involving a biodegradable polymer, for example, poly(alpha-hydroxy corrosive), polyhydroxy butyric corrosive, polycaprolactone, polyorthoester, polyanhydride and polycyanoacrylate are pharmaceutically appropriate excipient with the goal of immunization and can be utilized reasonably for such reason [Citation63].
Poly(alkyl cyanoacrylate)
An alternative hydrophobic microparticulate dosage form can be produced by utilizing poly(alkyl cyanoacrylates) also referred to as simply poly(cyanoacrylates) (PCAs). Hydrolysis of PCL yields 6-hydroxycaproic acid, which enters the citric acid cycle and is metabolized. As mentioned previously, PCL degrades slowly and being used in blends and copolymers with other biodegradable polymers [Citation61]. The rate of degradation of PCAs is much faster than PLGAs which do not necessitate the overloading of the body with excess polymer that is still present long after initial treatment [Citation64].
The rate of degradation of PCAs is significantly greater than PLGAs which do not necessitate the overloading of the body with excess polymer that is still present long after initial treatment PCAs is a class of polymers that undergo polymerization under mild conditions. PCAs have been widely investigated for the delivery of bio macromolecules. Because to their properties, cyanoacrylates can easily be formed into two types of particles: spheres [Citation65] or capsules [Citation66], both of which can be used to deliver bio macromolecules.
Patents
Bobo et al. mentioned the novel compositions of cyanoacrylic acid polymers and monomers, and uses thereof. In certain embodiments, a cyanoacrylic acid monomer of the present invention may incorporate a cyanoacrylic acid core bound to a biocompatible substituted organic residue that, upon formation of a polymer and subsequent biodegradation of such polymer, results in biocompatible degradation products [Citation67].
Miscellaneous
Agents, for example, hydroxypropylcellulose (HPC), carbopol, carboxymethylcellulose and polyacrylic acid have all demonstrated guarantee as mucoadhesive agents for potential use in pulmonary, and additionally nasal, conveyance, either alone, in combination with another carrier or fused into the structure of the carrier itself. Carbopol polymers are high molecular weight acrylic acid chains, usually cross-linked, and are available as powders or liquids.
Patents
Gerber invented lecithin- and carbopol-based mucoadhesive immunization arrangement that demonstrated tough mucoadhesive and adsorptive properties of vaccine antigen onto mucosal surfaces [Citation68].
See and See have also been described a method that comprises orally administering lyophilized multilamellar liposomes containing the antigen with acrylic polymers and copolymers [Citation69].
Surface-modified microspheres mediated mucosal immunization
In recent years, PLGA and PLA microparticles used for administration of vaccine antigens have gotten much consideration [Citation70,Citation71]. The mucoadhesive PLGA microspheres that were modified with CS were effectively created to enhance nasal vaccine delivery. CS is a mucoadhesive polysaccharide of marine origin and is drawing in expanded consideration as an authority specialist for mucosal immunization conveyance, since it is viewed as protected and has been appeared to advance transmucosal ingestion. Nasal or pulmonary delivery of CS has been appeared to improve systemic and mucosal antibody responses to various antigens in mice. These studies demonstrated that, taking after nasal administration, antigens encapsulating in PLGA microspheres evoke a higher insusceptible response than plan antigen. The molecule size of single microspheres was over the range of 1–10 microns. Particles under 10 microns size were effective in focusing on the APCs at mucosal surfaces [Citation72]. To clarify this positive response, it was hypothesized that encapsulating antigens are tested by particular cells, like the microfold (M) cells, which overlie the NALT, and then transported to the underlying APCs. NALT is a composed framework comprising B cells, T cells and APCs (dendritic cells and macrophages), which are secured by an epithelial layer containing M cells [Citation73] ().
The surface-modified nanoparticles also increase cellular antigen uptake and elicits the responses for longer period of time and restrict the adhesion of bacterial toxin to the surface of mucosa [Citation74]. In the development of mucosal vaccine, the greatest obstruction is need to cross the mucosal epithelial hindrance, and proficient presentation of the antigen to bodily fluid mucus immune system, also to overcome the natural tolerance at mucosal surface [Citation75]. Solution of such issues was proposed by advancement of surface-modified nanocarrier-based conveyance framework system. Transport of non-changed and surface-modified nanocarriers and resulting immunological responses are introduced in .
Figure 3. Schematic representation of targeting potential of surface-modified over non-modified nanocarriers [Citation19].
![Figure 3. Schematic representation of targeting potential of surface-modified over non-modified nanocarriers [Citation19].](/cms/asset/4ba9d412-71a9-4a45-8aeb-02dd8998fc98/ianb_a_1345927_f0003_b.jpg)
Ligand-decorated polymeric carrier systems for mucosal delivery
Improving the definite binding of particulates to particulate cells in mucosa using focusing on ligands and subsequent translocation/take-up by cells of gastrointestinal covering is another approach for the successful mucosal immunization conveyance [Citation76]. M cells have unique ability to take up antigen from lumen of small intestine via endocytosis, phagocytosis, transcytosis and antigen deliver to APCs for their activation. These components make M cells as the promising focus for immunization conveyance purposes.
Lectins
Lectins like molecules of plant or microbial origin, and in addition biotechnologically produced subordinates of such molecules, have interesting qualities to control the binding, up-take and intracellular directing of macromolecules and in addition colloidal carrier system. Interestingly to other mucoadhesive polymers, lectin binds specifically to epithelial cells instead of to the mucus gel layer. Lectins have the capacity to recognize and bind with particular sugar moieties. The sugar-binding moiety of most lectins is just a little part of the lectin, that is, a wide portion of lectin is not included in the acknowledgement and binding to the receptor [Citation77]. Lectins cause agglutination because of their capacity to cross-link sugar containing macromolecules [Citation78]. Lectins can possibly be used in Nasal Drug Delivery, particularly where internalization of the drug encapsulated nanoparticles is of special significance, for example, DNA delivery [Citation79].
Patents
Segal and Young in their invention described a multifunctional molecule, for example, a combination polypeptide, including an initial part which is capable of binding to an antigen bearing target and a second part which is capable of binding to a cell [Citation80].
In Kido et al.’s study, their patent provides an antigen-drug vehicle empowering transnasal, transmucosal and transdermal administrations, a method for inducing mucosal immunity and humoral immunity using the antigen-drug vehicle in particular, an AD vehicle enabling a switch from induction of specific generation of both of IgA and IgG antibodies and a transnasal vaccine, a mucosal vaccine, a therapeutic/prophylactic operator for sensitivity and so forth utilizing the AD vehicles [Citation81] ().
Table 3. List of patent on biodegradable/mucoadhesive polymers used for vaccine delivery.
Monoclonal antibody
The utilization of antibodies and monoclonal antibodies has been proposed for particular focusing within the gastrointestinal tract especially M cells. New approaches in monoclonal counter acting agent innovation are empowering the change of new method for passive immunization of mucosal surfaces. Ferritin-loaded liposomes conjugated to IgA were also additionally researched for mucosal immunization by mean of the rectum. It was observed that the presence of IgA on the liposome surface increased the uptake of conjugates by the PP and the nearby rectal/colon immune response to ferritin about 5-fold over uncoated liposomes [Citation82].
Patents
In Chatterjee et al.’s study, this disclosure outlines a specific monoclonal against idiotype antibody, assigned 1A7. This immune response has been set up as being fit of producing an anti GD2 response. It has all the attractive properties that accommodate for escaping and is appropriate for treating GD2 associated disease [Citation83].
In Koff et al.’s study, the present innovation is based, in part, on applicant’s discovery that immunizations with antigen–antibody complexes elicit neutralizing antibody responses. Broadly neutralizing antibodies, if passively administered to monkeys, ensure against an HIV-equivalent virus (SIV/HIV chimera, e.g. SHIV). The identification of antigens that bind the neutralizing antibodies remains challenging, especially illustrating the preferred conformation of the antigen [Citation84].
In Fox et al.’s study, their invention describes the advancement and utilization of antibodies within the digestive tract. Antibodies are described that are utilized to treat disorders associated with altered permeability of the digestive tract. Antibodies are described with increased stability within the environment of the digestive tract. Antibodies are described with enhanced penetrability to a compromised digestive tract [Citation85].
Invasin-mediated uptake
Invasions, virulent factor generally connected with the pathogenic bacteria cell wall, have also been explored as an alternative means to exploit M cells for enhanced uptake [Citation86]. Pathogens of interest for the purpose are Yersinia, Listeria, Salmonella, and Shigella, as they possess extracellular protein, invasion, which gives one of the most direct and efficient host cell invasion. These biomolecules encourages bacterial passage into cell and lymphatic system by binding to a subset of beta1 integrin receptors situated on apical membrane of intestinal M cells. Young and co-workers beautifully describe invasin-mediated internalization process [Citation87]. For this purpose, micro/nanoparticles coated with invasion of various pathogens like Yersinia enterocolitica, Y. pseudotuberculosis, Salmonella typhimurium, Salmonella enteritidis and Reovirus were evaluated. Reovirus type 1 is another ligand, which selectively adheres to, and endocytosed by intestinal M cells [Citation88]. Proteolytic processing of native Reovirus type 1 is required for adhesion to murin M cells and this is dependent on retention of modified σ1 and/or product of μ1 outer capsid protein.
Patent
In one patent, the strategy for the generation of very highly purified invasin proteins is disclosed. It was disclosed in this development that invasion can be utilized as an insusceptible immune-adjuvant to induce a safe immune response and for administration of drugs ().
Table 4. List of patent on ligands decorated system for vaccine delivery.
Conclusions
Mucosal immunization conveyance holds promise in generation of humoral as well as cellular responses. Biodegradable polymer-based systems have indicated assurance to the loaded antigen and help in inciting immune response to the desired level. Mucoadhesive polymeric system additionally upgrade possibility of controlled conveyance of antigenic protein for longer time and accordingly create significant antibody titer level at mucosal sites, that is, site of contamination. Recent understanding of ligand receptor cooperation has cleared new means of nasal immunization involving focusing of antigen to the pre-identified localization within the immune inductive sites including M cells. Exploitation of M cells focusing on ligands on securing to the surface of such carriers is emerging strategy. The successful mucosal inoculation conveyance of antigen should be done by ligand modified mucoadhesive/biodegradable polymer(s) that achieves particular, proficient and strengthen humoral, mucosal and cellular immune response. Biodegradable polymer-based delivery by chemical alteration is one of the ultimate decisions for effective uptake of protein. These delivery systems, specially based on PLGA/PLA/PCL, offer preferences like better antigen stability and prolonged release pattern. These polymeric particulate systems offers several advantages of stabilized antigens, their enhanced antigenicity, reproducible immune memory, probability of alteration for desired goals, site particulate focusing and prolonged release pattern.
Disclosure statement
The authors have declared no conflict of interest.
References
- Ogra PL. Mucosal immunity: some historical perspective on host-pathogen interactions and implications for mucosal vaccines. Immunol Cell Biol. 2003;81:23–33.
- Artis D. Epithelial-cell recognition of commensal bacteria and maintenance of immune homeostasis in the gut. Nat Rev Immunol. 2008;8:411–420.
- Holmgren J, Czerkinsky C, Eriksson K, et al. Mucosal immunisation and adjuvants: a brief overview of recent advances and challenges. Vaccine. 2003;21:S89–S95.
- Simecka JW. Mucosal immunity of the gastrointestinal tract and oral tolerance. Adv Drug Deliv Rev. 1998;34:235–259.
- de Magistris MT. Mucosal delivery of vaccine antigens and its advantages in pediatrics. Adv Drug Deliv Rev. 2006;58:52–67.
- Iwasaki A. Mucosal dendritic cells. Annu Rev Immunol. 2007;25:381–418.
- Kato T. Structure and function of intestinal mucosal epithelium. In: Pearay L. Ogra, Jiri Mestecky, Michael E. Lamm, et al., editors. Handbook of Mucosal Immunology. 1994; p. 11–26.
- Corr SC, Gahan CC, Hill C. M-cells: origin, morphology and role in mucosal immunity and microbial pathogenesis. FEMS Immunol Med Microbiol. 2008;52:2–12.
- Regoli M, Bertelli E, Borghesi C, et al. Three‐dimensional (3D‐) reconstruction of M cells in rabbit Peyer’s patches: definition of the intraepithelial compartment of the follicle‐associated epithelium. Anat Rec. 1995;243:19–26.
- Owen RL, Ermak TH, editors. Structural specializations for antigen uptake and processing in the digestive tract. Springer Semin Immunopathol. 1990;12:139–152.
- Lee Y-R, Lee Y-H, Kim K-H, et al. Induction of potent antigen-specific cytotoxic T cell response by PLGA-nanoparticles containing antigen and TLR agonist. Immune Netw. 2013;13:30–33.
- Gerelchuluun T, Lee Y-H, Lee Y-R, et al. Dendritic cells process antigens encapsulated in a biodegradable polymer, poly(D,L-lactide-co-glycolide), via an alternate class I MHC processing pathway. Arch Pharm Res. 2007;30:1440–1446.
- Rosalia RA, Cruz LJ, van Duikeren S, et al. CD40-targeted dendritic cell delivery of PLGA-nanoparticle vaccines induce potent anti-tumor responses. Biomaterials. 2015;40:88–97.
- O’Hagan DT, MacKichan ML, Singh M. Recent developments in adjuvants for vaccines against infectious diseases. Biomol Eng. 2001;18:69–85.
- Lycke N. Recent progress in mucosal vaccine development: potential and limitations. Nat Rev Immunol. 2012;12:592–605.
- Pavot V, Rochereau N, Genin C, et al. New insights in mucosal vaccine development. Vaccine. 2012;30:142–154.
- Neutra MR, Kozlowski PA. Mucosal vaccines: the promise and the challenge. Nat Rev Immunol. 2006;6:148–158.
- Nugent J, Po A, Scott E. Design and delivery of non-parenteral vaccines. J Clin Pharm Ther. 1998;23:257–285.
- Mishra N, Singh D, Sharma S, et al. Surface modified nanoparticulate carrier constructs for oral mucosal vaccine delivery. CDD. 2014;11:729–743.
- Raghuvanshi RS, Katare YK, Lalwani K, et al. Improved immune response from biodegradable polymer particles entrapping tetanus toxoid by use of different immunization protocol and adjuvants. Int J Pharm. 2002;245:109–121.
- Hilbert AK, Fritzsche U, Kissel T. Biodegradable microspheres containing influenza A vaccine: immune response in mice. Vaccine. 1999;17:1065–1073.
- Nakaoka R, Inoue Y, Tabata Y, et al. Size effect on the antibody production induced by biodegradable microspheres containing antigen. Vaccine. 1996;14:1251–1256.
- Raghuvanshi OS, Panda AK, Rajeev S. Formulation and characterization of immunoreactive tetanus toxoid biodegradable polymer particles. Drug Deliv. 2001;8:99–106.
- Lavelle E. Targeted delivery of drugs to the gastrointestinal tract. Crit Rev Ther Drug Carrier Syst. 2001;18:341–386.
- Jepson MA, Clark MA, Hirst BH. M cell targeting by lectins: a strategy for mucosal vaccination and drug delivery. Adv Drug Deliv Rev. 2004;56:511–525.
- Goldstein IJ. What should be called a lectin? Nature 1980;285:66.
- Russell-Jones G. The potential use of receptor-mediated endocytosis for oral drug delivery. Adv Drug Deliv Rev. 1996;20:83–97.
- Calis S, Jeyanthi R, Tsai T, et al. Adsorption of salmon calcitonin to PLGA microspheres. Pharm Res. 1995;12:1072–1076.
- Periti P, Mazzei T, Mini E. Clinical pharmacokinetics of depot leuprorelin. Clin Pharmacokinet. 2002;41:485–504.
- Okada H, Toguchi H. Biodegradable microspheres in drug delivery. Crit Rev Ther Drug Carrier Syst. 1995;12:1–99.
- Audran R, Peter K, Dannull J, et al. Encapsulation of peptides in biodegradable microspheres prolongs their MHC class-I presentation by dendritic cells and macrophages in vitro. Vaccine. 2003;21:1250–1255.
- Vyas Suresh P , PN. Gupta Implication of nanoparticle/microparticles in mucosal vaccine delivery. Exp Rev Vaccines. 2007;6:401–418.
- Andrews GP, Laverty TP, Jones DS. Mucoadhesive polymeric platforms for controlled drug delivery. Eur J Pharm Biopharm. 2009;71:505–518.
- Shojaei AH, Li X. Mechanisms of buccal mucoadhesion of novel copolymers of acrylic acid and polyethylene glycol monomethylether monomethacrylate. J Control Release. 1997;47:151–161.
- Smart JD. The basics and underlying mechanisms of mucoadhesion. Adv Drug Deliv Rev. 2005;57:1556–1568.
- Mishra N, Goyal AK, Khatri K, et al. Biodegradable polymer based particulate carrier (s) for the delivery of proteins and peptides. AIAAMC. 2008;7:240–251.
- Mishra N, Goyal AK, Tiwari S, et al. Recent advances in mucosal delivery of vaccines: role of mucoadhesive/biodegradable polymeric carriers. Exp Opin Therap Pat. 2010;20:661–679.
- Ilium L. Chitosan and its use as a pharmaceutical excipient. Pharm Res. 1998;15:1326–1331.
- van der Lubben IM, Verhoef JC, Borchard G, et al. Chitosan and its derivatives in mucosal drug and vaccine delivery. Eur J Pharm Sci. 2001;14:201–207.
- He P, Davis SS, Illum L. In vitro evaluation of the mucoadhesive properties of chitosan microspheres. Int J Pharm. 1998;166:75–88.
- Pan Y, Li Y-j, Zhao H-y, et al. Bioadhesive polysaccharide in protein delivery system: chitosan nanoparticles improve the intestinal absorption of insulin in vivo. Int J Pharm. 2002;249:139–147.
- Tafaghodi M, Tabasi SS, Jaafari M. Formulation, characterization and release studies of alginate microspheres encapsulated with tetanus toxoid. J Biomater Sci. 2006;17:909–924.
- Illum L, Chatfield SN. Vaccine compositions including chitosan for intranasal administration and use thereof. US 7,332,3183; 2008.
- Sava G, Zorzin L. Polysaccharide double-layer microcapsules as carriers for biologically active substance oral administration. US 2006228422; 2006.
- Fernandez MA, Janes K, Csaba N. Nanoparticles of chitosan and polyethylene glycol as a system for the administration of biologically-active molecules. US 20080095810; 2008.
- Balthasar S, Michaelis K, Dinauer N, et al. Preparation and characterisation of antibody modified gelatin nanoparticles as drug carrier system for uptake in lymphocytes. Biomaterials. 2005;26:2723–2732.
- Olsen D, Yang C, Bodo M, et al. Recombinant collagen and gelatin for drug delivery. Adv Drug Deliv Rev. 2003;55:1547–1567.
- Lawter JR. Inventor. Mucosal Therapeutics Llc, assignee. Mucoadhesive tetracycline formulations. United States patent application US 12/122,232. 2008.
- Singh M, Briones M, O’Hagan DT. A novel bioadhesive intranasal delivery system for inactivated influenza vaccines. J Control Release. 2001;70:267–276.
- O’Hagan D, Pavesio A. Use of hyaluronic acid polymers for mucosal delivery of vaccine antigens and adjuvants. US Patent No. 6,824,793;2005.
- Kumar N. Polyanhydrides: an overview, 54 ADV. Adv Drug Deliv Rev. 2002;54:889–910.
- Andrianov AK, Payne LG. Protein release from polyphosphazene matrices. Adv Drug Deliv Rev. 1998;31:185–196.
- Andrianov AK, Decollibus DP, Gillis HA, et al. Immunostimulating polyphosphazene compounds for intradermal immunization. US 2009041810; 2009.
- Wolf BW, Garleb KA, Choe YS, et al. Pullulan is a slowly digested carbohydrate in humans. J Nutr. 2003;133:1051–1055.
- Ni Y, Yates KM. Inventors. Carrington Laboratories, Inc., assignee. Delivery of physiological agents with in-situ gels comprising anionic polysaccharides. United States patent US Patent No. 7,494,669. 2009.
- Varner S, Beeley N, Chudzik S, Burkstrand M. Inventors; Varner Signe E, Beeley Nathan RF, Chudzik Stephen J, Burkstrand Michael J, assignee. In vivo formed matrices including natural biodegradale polysaccharides and ophthalmic uses thereof. United States patent application US 11/524,925. 2006.
- O’Hagan D, Singh M, Gupta RK. Poly(lactide-co-glycolide) microparticles for the development of single-dose controlled-release vaccines. Adv Drug Deliv Rev. 1998;32:225–246.
- Delgado A, Lavelle E, Hartshorne M, et al. PLG microparticles stabilised using enteric coating polymers as oral vaccine delivery systems. Vaccine. 1999;17:2927–2938.
- Sokoll KK, Chong P, Klein MH. Biodegradable targetable microparticle delivery system. US 20050163745; 2005.
- Reid RH, Boedeker EC, van Hamont JE, Setterstrom JA. Vaccines against diseases caused by enteropathogenic organisms using antigens encapsulated within biodegradable-biocompatible microspheres. Google US 20030161889; 2003.
- Langer R, Peppas NA. Advances in biomaterials, drug delivery, and bionanotechnology. AIChE J. 2003;49:2990–3006.
- Grandfils C, Jerome R, Nihant N, Teyssie P. Biocompatible and biodegradable nanoparticles designed for proteinaceous drugs absorption and delivery. US 5,962,566; 1999.
- O’Hagan D. Immunogenic compositions containing microparticles comprising adsorbed toxoid and polysaccharide-containing antigens. US 20050118275A1; 2005.
- Lherm C, Muller RH, Puisieux F, et al. Alkylcyanoacrylate drug carriers: II. Cytotoxicity of cyanoacrylate nanoparticles with different alkyl chain length. Int J Pharm. 1992;84:13–22.
- Couvreur P, Roland M, Speiser P. Inventors; Biodegradable submicroscopic particles containing a biologically active substance and compositions containing them. United States patent US 4,329,332. 1982.
- Fallouh NA, Roblot-Treupel L, Fessi H, et al. Development of a new process for the manufacture of polyisobutylcyanoacrylate nanocapsules. Int J Pharm. 1986; 28:125–132.
- Bobo JS, Quintero JA, Jonn JY, et al. Inventors; Closure Medical Corporation, assignee. Biocompatible polymers and adhesives: compositions, methods of making and uses related thereto. United States patent US 7,255,874. 2007.
- Gerber JD. Adjuvant composition for mucosal and injection delivered vaccines. US 6,676,958; 2004.
- See JR, See DM. Method for inducing a systemic immune response to an antigen. US 6,015,576; 2000.
- Jiang W, Gupta RK, Deshpande MC, et al. Biodegradable poly(lactic-co-glycolic acid) microparticles for injectable delivery of vaccine antigens. Adv Drug Deliv Rev. 2005;57:391–410.
- Alpar HO, Somavarapu S, Atuah K, et al. Biodegradable mucoadhesive particulates for nasal and pulmonary antigen and DNA delivery. Adv Drug Deliv Rev. 2005;57:411–430.
- Alonso MJ, Gupta RK, Min C, et al. Biodegradable microspheres as controlled-release tetanus toxoid delivery systems. Vaccine. 1994;12:299–306.
- Kuper CF, Koornstra PJ, Hameleers DM, et al. The role of nasopharyngeal lymphoid tissue. Immunol Today. 1992;13:219–224.
- Hobson P, Barnfield C, Barnes A, et al. Mucosal immunization with DNA vaccines. Methods. 2003;31:217–224.
- Chen W, Patel GB, Yan H, et al. Recent advances in the development of novel mucosal adjuvants and antigen delivery systems. Human Vaccines. 2010;6:706–714.
- Lavelle E, Grant G, Pusztai A, et al. The identification of plant lectins with mucosal adjuvant activity. Immunology. 2001;102:77–86.
- Lehr C-M. Lectin-mediated drug delivery: the second generation of bioadhesives. J Control Release 2000;65:19–29.
- Vasir JK, Tambwekar K, Garg S. Bioadhesive microspheres as a controlled drug delivery system. Int J Pharm. 2003;255:13–32.
- Ugwoke MI, Agu RU, Verbeke N, et al. Nasal mucoadhesive drug delivery: background, applications, trends and future perspectives. Adv Drug Deliv Rev. 2005;57:1640–1665.
- Segal AH, Young E. Lectin compositions and methods for modulating an immune response to an antigen. US 20040241137; 2004.
- Kido H, Mizuno D. Antigen-drug vehicle enabling switch from selective production of IgA antibody to production of both of IgA and IgG antibodies and transnasal/mucosal vaccine using the same. US 20090130131; 2009.
- Vyas SP, Gupta PN. Implication of nanoparticles/microparticles in mucosal vaccine delivery. Expert Rev Vaccines. 2007;6:401–418.
- Chatterjee M, Foon KA, Chatterjee SK. Monoclonal antibody 1A7 and use for the treatment of melanoma and small cell carcinoma. US 7,517,967; 2009.
- Koff W. Antigen-Antibody Complexes as HIV-1 Vaccines. WO09058989; 2009.
- Fox BS. Antibody therapy for modulating function of intestinal receptors and methods of treating diabetes and obesity. WO09020748; 2009.
- Henderson B, Poole S, Wilson M. Bacterial modulins: a novel class of virulence factors which cause host tissue pathology by inducing cytokine synthesis. Microbiol Rev. 1996;60:316–341.
- Young VB, Falkow S, Schoolnik GK. The invasin protein of Yersinia enterocolitica: internalization of invasin-bearing bacteria by eukaryotic cells is associated with reorganization of the cytoskeleton. J Cell Biol. 1992;116:197–207.
- Clark MA, Hirst BH, Jepson MA. Lectin-mediated mucosal delivery of drugs and microparticles. Adv Drug Deliv Rev. 2000;43:207–223.
- Makin JC, Bacon AD. Influenza vaccine composition with chitosan adjuvant. US 6,534,065; 2003.
- del Guidice G, Baudner B. Mucosal vaccines with chitosan adjuvant and meningococcal antigens. US 20060051378; 2006.
- Garg N, Mangal S, Khambete H, et al. Mucosal delivery of vaccines: role of mucoadhesive/biodegradable polymers. DDF. 2010;4:114–128.
- Illum LC, Steven N. Vaccine composition including chitosan for intranasal administration and use thereof. US 7,323,183; 2008.
- Kjems J, Howard K, Alan B, et al. Dehydrating chitosan nanoparticle. WO09006905; 2009.
- Alonso FJ, Janes K, Csaba N. Nanoparticles of chitosan and polyethylene glycol as a system for administration of biologically active molecules. US 2008095810; 2008.
- Fernandez A, Jose M, Kevin J. Nanoparticles of chitosan and polyetyleneglycol as a system for administration of biologically active molecules. US 20080095810; 2008.
- Guidice GD, Banden B. Mucosal vaccines with chitosan adjuvant and meningococcal antigens. US 20060051378; 2006.
- Del GG, Barbara B. Mucosal vaccines with chitosan adjuvant and meningococcal antigens. US 20060051378; 2006.
- Del G, Giuseppe CS, Barbara CS, O’Hagan DT. Mucosal vaccines with chitosan adjuvant and meningococcal antigens. EP1506008; 2009.
- Rao KK. Polymerized solid lipid nanoparticles for oral or mucosal delivery of therapeutics protein and peptides. US 20080311214; 2008.
- Jeong S, Young KI, Chan P. Vaccine compositions using antigens encapsulated within alginate microsphere for oral administration and preparation process thereof. WO01000233; 2001.
- Mohapatra S, Lee DW. Controlled and sustained generally transfer mediated by thiol modified polymers. WO2007086923; 2007.
- Scott TL, Brown LR, Riske FJ, et al. Inventors; Epic Therapeutics, Inc., assignee. Sustained release microspheres. United States patent US 6,458,387. 2002.
- del Guidice G, Baudner B. Inventors; Novartis Ag, University Leiden, assignee. Mucosal vaccines with chitosan adjuvant and meningococcal antigens. United States patent US 8,926,992. 2015.
- Hargis BM, Pumford NR, Morgan M, et al. Inventors; The Board of Trustees of the University of Arkansas, assignee. Novel mucosal adjuvants and delivery systems. United States patent application US 14/439,536. 2013.
- Hargis BM, Pumford NR, Morgan M, et al. Inventors; The Board of Trustees of the University of Arkansas, assignee. Novel Mucosal Adjuvants and Delivery Systems. United States patent US 20,170,143,823. 2017.
- Hilgers L. Polyanionic polymers as adjuvant for mucosal immunization. US 2003021793; 2003.
- King M. Use of charged dextran as a mucoactive agent and methods and pharmaceutical compositions relating thereof. US 20040224922; 2004.
- Wang D, Erlanger , Bernard F. Methods relating to immunilogical dextran protein conjugate. US 6,287,568; 2001.
- Singh M, O’Hagan D. Use of bioadhesive and adjuvants for mucosal delivery of antigens. US 2005281843; 2005.
- O’Hagan DT. Immunologenic compositions containing microparticles comprising absorbed toxoid and polysaccharides containing antigens. US 20050118275A1; 2005.
- O’Hagan D, Singh M, Klinman DM. Immunogenic compositions containing anthrax antigen, biodegradable polymer microparticles and polynucleotide containing immunological adjuvant; US 2008037784; 2008.
- Gerber JD. Inventor. Advanced Bioadjuvants, Llc, assignee. Adjuvant composition for mucosal and injection delivered vaccines. United States patent US 6,676,958. 2004.
- McCluskie MJ, Davis HL. Inventors; Ottawa Hospital Research Institute, assignee. Methods and products for inducing mucosal immunity. United States patent US 8,574,599. 2013.
- Baker JR, Bielinska A, Myc A. Inventors; The Regents of the University of Michigan, assignee. Nanoemulsion vaccines. United States patent application US 11/929,295. 2007.
- Chang RC, Kivirikko KI, Neff TB, et al. Inventors; FibroGen, Inc., assignee. Recombinant gelatins. United States patent US 6,992,172. 2006.
- Langer RS, Elisseeff JH, Anseth K, Sims D. Inventors; Massachusetts Institute of Technology, University Technology Corporation, The General Hospital Corporation, assignee. Semi-interpenetrating or interpenetrating polymer networks for drug delivery and tissue engineering. United States patent US 6,224,893. 2001.
- Berkland CJ, Shi L. Inventors; The University of Kansas, assignee. Nanoclusters for delivery of therapeutics. United States patent US 7,651,770. 2010.
- O’Hagan DT, Singh M, Klinman DM. Inventors. Immunogenic compositions containing anthrax antigen, biodegradable polymer microparticles, and polynucleotide-containing immunological adjuvant. United States patent US 9,107,813. 2015.
- Wu C, Ghayur T, Dixon RW, Salfeld JG. Inventors; Abbott Laboratories, assignee. Dual variable domain immunoglobulin and uses thereof. United States patent US 7,612,181. 2009.
- O’Hagan D, Van Nest G, Ott GS, et al. Inventors; Novartis Vaccines, Diagnostics, Inc., assignee. Use of microparticles with adsorbed antigen to stimulate immune responses. United States patent US 7,597,908. 2009.
- Baker JR Jr, Bielinska AU, Mank N, et al. Inventors; The Reagents of the University of Michigan, assignee. Immunogenic compositions comprising nanoemulsion and hepatitis b virus immunogen and methods of using the same. United States patent US 9,415,006. 2016.
- Orlowski M. Inventor; Eurocor GmbH, assignee. Coated expandable system. United States patent application US 12/527,741. 2008.
- Parmely M, Medhekar J. Therapeutics alkaline protease composition and use in facilitating the transport of antigens across the gastrointestinal mucosal lining. US 20090068174; 2009.
- O’Hagan D, Lavelle EC. Plant lectins as mucosal adjuvants. US 20050169938; 2005.
- Newell M, Karen NE, Villobos-Menvey E. Composition of UCP inhibitors, Fas antibody a fatty acid metabolism inhibitor and a glucose metabolism inhibitor. US 7,510,710; 2010.
- Sorio C. Antibody and method for identification of dendritic cells. US 20090131346; 2009.
- Sims JE, O’Neal LF. Methods for treating disease with an IL-IR antibody. US 20090022733; 2009.
- Readett D, Robot J. Jungnelius. Anti-Ctla-4 antibody and Cpg-Motif containing synthetic oligodeoxynucleotide combination therapy for cancer treatment. US 20090117132; 2009.
- Wu C, Ghayur T, Dixon RW, Salfeld JG. Inventors; Abbott Laboratories, assignee. Dual variable domain immunoglobulin and uses thereof. United States patent US 8,258,268. 2012.
- Allan CB. Stabilized high concentration Anti-Integrin alphavbeta3 antibody formulation. US 20090053238; 2009.
- Fox BS. Antibody therapy for modulating function of intestinal receptors. W009058989; 2009.
- Gillies SD, Lan Y, Holden S. Enhancement of antibody-cytokine fusion protein mediated immune response by combinated treatment with immunocytokine uptake enhancing agents. US 7,517,526; 2009.
- Gelfand J A. Engineered antibody-stress protein fusions. US 8,143,387. 2012.