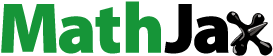
Abstract
Recently, there has been an upsurge in the use of naturally available fuels for solution combustion synthesis (SCS) of nanoparticles. Although many reports suggest that these biofuels pose less harm to the environment, their strategic advantages and reliability for making NPs has not been discussed. In the present work, we try to address this issue using plant extracts as biofuels for the SCS of zinc oxide nanoparticles as a model system. In the present work, combustion synthesis of ZnO NPs using lactose and aqueous leaf extracts of Abutilon indicum, Melia azedarach, Indigofera tinctoria as biofuels has been carried out. A comparative analysis of the obtained powders has been conducted to understand the strategic advantages of using plant extracts over a chemical as combustion fuel for the synthesis of zinc oxide nanoparticles. The X-ray diffractograms of the samples revealed the presence of Wurtzite hexagonal structure with varying crystallite sizes. Morphological studies indicated that samples prepared using biofuels had smaller diameter than those prepared using lactose as fuel. Surface characteristics of the samples were measured by X-ray photoelectron spectroscopy. Qualitative phytochemical screening of aqueous leaf extracts revealed the presence of many phytochemicals in them, which might be responsible for combustion. Gas chromatography mass spectrum was carried out to detect the phytochemicals present in the aqueous extracts of the leaves. Further, anticancer evaluation carried out against DU-145 and Calu-6 cancer cells indicated higher anticancer activity of zinc oxide nanoparticles prepared using biofuels. The results of blood haemolysis revealed the biocompatibility of zinc oxide nanoparticles at lower concentrations. In conclusion, we propose that multiple other studies would be required in order to vindicate the potential advantages of using naturally available fuels in SCS.
Introduction
Zinc oxide (ZnO) is an inorganic antimicrobial agent which is generally recognized as safe (GRAS) under US-FDA listings, to human beings and animals. Controllable synthesis of ZnO nanomaterials of desired size and shapes has been the subject of investigation by researchers in recent days because it has been found that the properties of ZnO nanoparticles (NPs) are size and morphology dependent. ZnO NPs have shown wide variety of applications in the field of catalysis [Citation1], textiles [Citation2] cosmetics [Citation3], drug delivery [Citation4], cancer therapy [Citation5], antivirals [Citation6,Citation7], etc. Along with the basic properties of ZnO, fine particles of ZnO have deodorizing and antibacterial action and for that reason are added into various materials including cotton fabric, rubber, and food packaging. ZnO NPs have found good application in bio molecular detection, diagnostics, and micro-electronics. Recently antidiabetic activity of ZnO NPs has also been reported [Citation8].
Self-propagating high temperature solution combustion synthesis (SCS) is a novel technique that has been used successfully for the preparation of ceramic, phosphor materials. This method has been employed to prepare LiNiO2, LiCoO2, LiMn2O4, ZnO, CuO NPs using various organic compounds such as sugar, citric acid, lactose, glycine as fuels [Citation9–12].
Synthesis of nanomaterials by biological approach is cheaper, environment friendly and innovative. Biosynthesis of NPs has been started as they possess advantages over physical and chemical processes. It was observed that many a times, chemical methods lead to the presence of some of the toxic chemicals absorbed on the surface of NPs that may have adverse effects in medical applications [Citation13]. This problem can be overcome by synthesizing nanomaterials by green methods [Citation14]. Green synthesis provides advancement over chemical and physical methods as it is environment friendly and free from toxic chemicals [Citation15]. Green synthesis approach for the synthesis of metal NPs has several other advantages over other methods such as it is very simple, clean, efficient, economically cheap as bio-resources are used that can act as reducing agent as well as stabilizing agent and capping agent. It is a non-toxic method due to very less or non-consumption of hazardous materials on the surface of nanomaterials, it does not need any external ligand or capping or stabilizing agent for NPs [Citation16–22]. In addition to all this biosynthesized NPs are usually biocompatible and highly applicable for biomedical applications [Citation16,Citation17,Citation18]. Green synthesis of different NPs by plants such as Acalypha indica, Rosa hybrid, Marraya koenigii, Tanacetum vulgar, Punica granatum, Tamarindus indica, Prunus japonica, Couroupita guianensis, Borago officinalis, etc., has been reported [Citation23–28]. This begs a question; whether the use of naturally occurring phytochemicals pose any strategic advantage over using chemically purified counterparts, other than just being cheaper or naturally available?
Abutilon indicum L (A. indicum) is a herb found throughout India. It is a small shrub in the malvaceae family. This herb is used in ayurvedic preparations to treat hemorrhoids, diabetes, etc. [Citation29]. Melia azedarach L (M. azedarach) belongs to the family meliaceae and is popularly known as Indian lilac. This plant is traditionally used as anthelmintic, antilithic diuretic, emmenagouge and astringent agents. Many studies have reported the analgesic, antiviral, anti-malarial, antibacterial, antifeedant, anticancer and anti-fertility activity of this plant [Citation30]. Indigofera tinctoria L (I. tinctoria) is a widely distributed shrub found throughout India, belongs to the family Fabaceae. It has been showed that, this plant possesses anti-microbial, anti-cancer, nervous disorders, bronchitis, liver ailments and anticardiovascular activities [Citation31].
In recent days NPs have gained much importance for their implications in cancer therapy. Studies have shown that ZnO NPs cause cytotoxicity to many types of cells such as human kidney cells, human heapatocytes, embryonic kidney cells, HepG2, MDA-MB-231, DU-145, Calu-6, A549, PC-3 HT29, Caco-2 and MCF7 cell lines of epithelial origin, glioma cells, etc. [Citation32–38]. In our previous work we have shown the anti-tubercular activity of ZnO NPs synthesized by SCS using lemon juice as biofuel [Citation33,Citation38]. Although many reports suggest that these "bio" fuels pose less harm to the environment, their strategic advantages and reliability for making NPs has not been discussed so far. As the interaction of NPs with biomolecules is an expanding area of research, which is still largely unexplored yet, the present study reports the synthesis of ZnO NPs by simple, cost-effective SCS using lactose and the aqueous leaf extracts of A. indicum, M. azedarach, I. tinctoria as biofuels and investigations of their anticancer activity was examined on human prostate carcinoma cell line DU-145 and lung cancer cell line Calu-6 by MTT [3-(4,5-dimethylthiazol-2-yl)-2,5-diphenyltetrazolium bromide] assay. In vitro haemolysis assay was carried out to assess the biocompatibility of ZnO NPs. Biological activity of ZnO NPs prepared by SCS using biofuels was compared extensively with that of the ZnO NPs prepared using lactose as fuel.
Materials and methods
Materials
Zinc nitrate hexahydrate [Zn(NO3)2.6H2O), AR 99% SD Fine, India], lactose monohydrate [C12H22O11.H2O, AR SD Fine, India], Dulbecco's modified eagle's medium [DMEM, Gibco, USA], Dimethyl sulfoxide [DMSO, C2H6SO, AR 99% Merck, India], MTT [C18H16BrN5S, 97.5%, Sigma Aldrich, USA], Triton X-100 [C14H22O(C2H4O)n (n = 9−10), Sigma Aldrich, USA] were used as such without further purification.
Plant collection
Leaves of A. indicum, M. azedarach were collected in and around Vidyaranyapura, Bengaluru and I. tinctoria leaves were collected in Hunasamarnahalli, Bengaluru (GPS position of the location where the leaves were collected has been provided in Supplementary file Figure 9). Leaves were washed individually under running tap water to remove any traces of soil, dust particles, etc. Then washed with distilled water and dried in shade at room temperature. The dried leaves were ground well mechanically to get the fine powder.
Preparation of aqueous extracts
Twenty grams of the leaves of each plant was subjected to soxhlet extraction separately for 72 h using 125 ml of double distilled water. The aqueous solution obtained was filtered with Whatman No.1 filter paper. Filtrate was collected and used for the preparation of NPs.
Synthesis by solution combustion method
Using lactose as fuel
The ratio of oxidizer to fuel needed for stoichimetric mixture was calculated as reported in the literature [Citation11,Citation12]. 5.0 g of zinc nitrate hexahydrate [Zn(NO3)2.6H2O] and 1.25 g of lactose monohydrate [C12H22O11. H2O] were dissolved in 20 ml of double distilled water. The crystallizing dish containing this mixture was placed in a preheated muffle furnace at 375 ± 10 °C. Within a short while the solution boiled to form a transparent gel. As the reaction initiated, a flame appeared which witnessed the combustion of the fuel. ZnO thus synthesized was labelled as ZnO (LA).
Green synthesis using the aqueous leaf extracts of A. indicum, M. azedarach and I. tinctoria as biofuels
Five grams of Zn(NO3)2.6H2O and different aliquots (12, 15, 18 ml) of the aqueous leaf extract of A. indicum were mixed thoroughly for about 10 min by adding 20 ml of double-distilled water. Then the reaction mixture was placed in a preheated muffle furnace at 375 ± 10 °C. Within a short while the solution boiled to form a gel followed by decomposition with the evolution of gases. Then the gel got transformed in to a white solid.
The method was standardized using different aliquots of the leaf extracts of M. azedarch and I. tinctoria. The above procedure was repeated separately with 15, 18 and 21 ml of the aqueous extracts of M. azedarach leaves and 10, 12.5 and 15 ml of the aqueous extracts of I. tinctoria leaves.
ZnO NPs thus prepared above were ground in to fine powders and characterized using various methods. The samples were labeled as ZnO (AI-1, AI-2, AI-3), ZnO (MA-1, MA-2, MA-3) and ZnO (IT-1, IT-2, IT-3) for ZnO powders prepared by using the aqueous leaf extracts of A. indicum (12 ml, 15 ml, 18 ml), M. azedarach (15 ml, 18 ml, 21 ml) and I. tinctoria (10 ml, 12.5 ml, 15 ml) as fuels, respectively.
Characterization techniques
The phase identity and crystalline size of ZnO NPs were studied by powder X-ray diffraction using PANalytical X’pert diffractometer with Cu Kα radiation (λ = 1.5418Å) as the source. The surface morphology of the samples was studied by scanning electron microscopy (SEM) performed on HITACHI S3400N (Singapore). The particle shape and size were determined by transmission electron microscopy (TEM) carried out on Philips CM200. Surface characteristics of the samples were measured by X-ray photoelectron spectroscopy (XPS) (PHI 5000 Versa Probe II, USA) equipped with a mono-chromatic Al Kα (1486.6 eV) X-ray source and a hemispherical analyzer. The weight change with heat of the samples was observed by thermo gravimetric analysis (TGA) on Perkin Elmer Simultaneous Thermal Analyzer (STA 8000, USA). Brunauer-Emmett-Teller (BET) surface area measurements were carried out on Micromeritics ASAP 2020 with N2 adsorption-desorption isotherms. Degassing condition was as follows. Outside the instrument and one hour in-situ degassing at 120 °C. To understand which components of the leaf extracts are responsible for combustion, phytochemical examinations were carried out for all the extracts as per the standard methods [Citation39–41]. To find out the phytochemicals present in the aqueous extracts of the leaves, gas chromatography mass spectrum (GCMS) was carried out on Jeol spectrometer (Model: Accu TOF GCV, Japan). Split ratio of the GC-MS analysis was 20:1. Column temperature program was as follows: Initial temperature 80 °C for 5 min. Ramp: 10 °C/min to 260 °C. Again isothermal for 5 min, then ramp at 30 °C/min to 280 °C, isothermal for 5 min. Solvents used were methyl alcohol, ethyl alcohol and DMSO in equal volumes. Column used was HP5, 30 m long, 0.25 mm id and 0.25 μm film thicknesses. The injector temperature was 250 °C, while the detector temperature was 280 °C. Helium was used as carrier gas at 1 ml/min.
Evaluation of anticancer activity by MTT assay
Anticancer activity of ZnO NPs was carried out by MTT assay as reported in our earlier studies [Citation33,Citation38]. Cell lines DU-145 and Calu-6 (procured from ATCC) of 80% confluent were trypsinized. The viable 50,000 cells/well were seeded in a 96 well plate and incubated for 24 h at 37 °C, 5% CO2 incubator. ZnO NPs from 0–300 μg/mL in DMEM without fetal brovine serum were incubated for 24 h. After incubation with ZnO NPs the media was removed from the wells and 100 μl/well of the MTT (5 mg/10 ml of MTT in 1 × phosphate buffered saline, the solution was filtered through 0.2 μM filter) working solution was added and incubated for 3 to 4 h. After incubation with MTT reagent, the media was removed from the wells and 100 μL of DMSO was added to rapidly solubilize formazan and absorbance was measured at 590 nm. Percent of inhibition was calculated as [100-(As/Ac) × 100] and cell viability was calculated as [As ×100/Ac], where As and Ac are absorbance of sample and control, respectively.
In vitro cytotoxicity by blood haemolysis
The haemolysis activity test was performed against ZnO NPs by following the procedure of Dhaneswar Das et al. [Citation42]. In brief, to 9 ml of the blood sample collected from a sheep, 1 ml of 3.8% sodium citrate was mixed. This inhibits the coagulation of blood. The sample was centrifuged at 3000 rpm for 5 min. The supernatant which was having platelet poor plasma was discarded. The pellet containing RBC was suspended in 10 ml of phosphate buffer saline (PBS) of pH 7.4. The cells were suspended in PBS to obtain a uniform suspension of cells. ZnO NPs with different concentrations of 0.25, 0.5, 1.0, 2.5, 5.0 mg/mL were taken in different test tubes. To all the test tubes 2 ml of erythrocyte suspension was added and the test tubes were inverted. The tubes were then gently shaken to retain the contact of the blood with ZnO NPs and incubated at 37 °C for 90 min. The samples were centrifuged at 3000 rpm for 5 min to pellet out the RBC cells. The supernatant was then separated and the absorbance was measured at 540 nm against a PBS blank solution. The percentage of haemolytic index (%) was calculated based on the formula shown below [Citation43]
where, A is the absorbance value. Triton X-100 and phosphate buffer saline (PBS) served as positive and negative controls, respectively.
Results and discussions
Crystal structure
shows the crystal structure of the as-prepared ZnO NPs using lactose and different volumes of aqueous extracts of the leaves of A. indicum, M. azedarach and I. tinctoria. The data were analyzed using Origin 8.1 Software (Origin Lab Corporation, USA). The PXRD pattern of ZnO NPs synthesized using lactose is shown in , ZnO NPs synthesized using 12 ml, 15 ml, 18 ml of the aqueous leaf extract of A. indicum is shown in ) respectively, ZnO NPs synthesized using 15 ml, 18 ml, 21 ml of the aqueous leaf extract of M. azedarach is shown in ) respectively and ZnO NPs synthesized using 10 ml, 12.5 ml, 15 ml of the aqueous leaf extract of I. tinctoria is shown in ) respectively All the diffractograms agree with the standard JCPDS pattern of zincite [36–1451]. This confirms the hexagonal structure of ZnO with lattice parameters a = 3.24982 Å and c = 5.2066 Å. The broadening of the diffraction peaks indicates that the crystal size is small. The crystallite size was calculated using the Scherrer equation, D = k λ/β Cos θ, where D is the crystallite size, k is the Scherrer constant (0.9), λ is the X-ray wavelength, θ is the Bragg angle and β is the corrected half-peak width of the sample. The average crystallite sizes of all ZnO NPs prepared are given in . The observed increase in crystallite size with the increase in fuel/oxidant ratio might be due to increase of flame temperature which assists the crystal growth [Citation44]. Among the green synthesized ZnO samples, ZnO (AI-2), ZnO (MA-2) and ZnO (IT-2), prepared using moderate volume of plant extracts were selected for further characterization and bio-studies.
Figure 1. Powder XRD pattern of (a) ZnO (LA), (b) ZnO (AI-1), (c) ZnO (AI-2), (d) ZnO (AI-3), (e) ZnO (MA-1), (f) ZnO (MA-2), (g) ZnO (MA-3), (h) ZnO (IT-1), (i) ZnO (IT-2) and (j) ZnO (IT-3).

Table 1. Average crystallite size of ZnO NPs.
Product formation mechanism
Zn(NO3)2 and the fuel (lactose, aqueous extract of A. indicum, M. azedarach and I. tinctoria) were mixed in distilled water. When this mixture is heated to 375 ± 10 °C, initially the wet powder undergoes thermal dehydration followed by decomposition of zinc nitrate and fuel. Then it ruptures in to a flame and yields porous, agglomerated powders. The reaction was self-propagating and the temperature produced was sustained for a length of few seconds.
The time span required for completion of the above reactions was about 5 min. Probable reaction mechanisms of formation of ZnO by SCS using lactose and biofuels are given as below
Morphology
The surface morphology of the as prepared ZnO (LA), ZnO (AI-2), ZnO (MA-2) and ZnO (IT-2) was studied using the SEM as shown in ), respectively. The SEM micrographs of ZnO powders show that the morphology of all the samples is more or less spherical. Micrographs reveal that besides the spherical crystals the powders also contain voids and pores, the reason for which can be traced to the large amounts of hot gases that escape out of the reaction mixture during combustion. It is through pores of various sizes and shapes that the crystallites are interlinked to one another.
) shows the TEM images of ZnO (LA), ZnO (AI-2), ZnO (MA-2) and ZnO (IT-2), respectively. The TEM study was carried out to understand the crystalline characteristics of the NPs. The images of ZnO (LA), ZnO (AI-2) and ZnO (IT-2) confirm that the particles are spherical and the images of ZnO (MA-2) confirm that the particles are of non-uniform thickness with spherical and cube shape. The particle size of ZnO (AI-2), ZnO (MA-2) and ZnO (IT-2) were found to be in the range of 15–30 nm, 11–20 nm, 8–20 nm and 11–22 nm, respectively. The average particle size of ZnO (LA), ZnO (AI-2), ZnO (MA-2) and ZnO (IT-2) by histogram was found to be 26, 15, 12 and 21 nm, respectively.
XPS measurements
The XPS reference spectra obtained for ZnO (LA), ZnO (AI-2), ZnO (MA-2) and ZnO (IT-2) are shown in ), respectively. All the samples showed strong characteristic peaks for Zn and O with trace quantities of carbon and nitrogen in the sample. The presence of carbon could be attributed to the presence of trace contaminants either in the XPS instrument or due to the remnants of the combustion synthesis. However, the absence of nitrogen and sulphur along with other contributing elements characteristic of biofuel/plant extracts could signify the role of plant extracts purely in the process of ZnO NPs preparation and none in their anticancer properties. The trace quantities of nitrogen seen were removed in subsequent cleaning procedures before the samples were subject to any further experimentation. It is important to also note that no changes in the Znp3/2 binding energies across all the samples suggest a strong crystallite formation in all the samples. These values correlate to previously described ZnO NPs which further confirm the formation of the samples without any crystalline defects (High resolution spectra of the elements Zn, C, O and N has been shown in Supplementary file as Figure 10).
Surface area measurements
The BET surface area values are presented in . These results show that the surface area of ZnO (MA-2) > ZnO (IT-2) > ZnO (AI-2) > ZnO (LA). The results indicate the higher surface area to volume ratio of ZnO NPs prepared using biofuels over ZnO NPs prepared using lactose as fuel. The different phytocomponents present in the leaves extracts which act as fuels in the combustion process might be responsible for this. These results indicate the higher surface area of ZnO NPs prepared using biofuels over ZnO NPs prepared using conventional chemical fuels by SCS.
Table 2. BET surface area values of ZnO NPs.
TG analysis
TG curves of ZnO (LA), ZnO (AI-2), ZnO (MA-2) and ZnO (IT-2) are shown in ), respectively. The temperature scale for the measurement was from 29 °C to 1185 °C. All the samples show that the weight decreased continuously up to 1185 °C. The total weight loss for ZnO (LA), ZnO (AI-2), ZnO (MA-2) and ZnO (IT-2) were 1.011%, 6.819%, 3.495% and 4.08%, respectively. The microvoids in the samples could be the reason in allowing weight loss over a period of 1185 °C. A continuous decrease in weight was observes as the TG analysis was carried out in N2 ambient. Oxygen out-diffusion from ZnO matrix probably has resulted in the formation of oxygen deficient ZnO compound (ZnO1-δ). Two characteristic weight loss peaks were seen, one at 600 °C and the other at 1100 °C. First one could be the formation of CO2 and the other one could be the microvoids losing air. The weight loss also might be due to thermal desorption of water and carbon dioxide from the surface of ZnO particles. Furthermore, no obvious peaks were seen in the curves as shown in ), which may indicate the formation of hexagonal wurtzite structure of ZnO due to temperature released during combustion process. In all the curves no transition was seen anywhere. TGA results also indicate that all the four samples absorb N2 and slowly release them over a period of time. This is a direct indication that the NPs are not only pure but also porous in nature [Citation25]. Thus TGA studies confirm the absence of any reminder from the plant extracts after the combustion process.
Qualitative phytochemical analysis of the aqueous extracts of leaves of A. indicum, M. azedarach and I. tinctoria
The results of qualitative phytochemical analysis of aqueous leaf extracts of A. indicum, M. azedarch and I. tinctoria are given in . These confirm the presence of many phyto-components in the aqueous extracts of the leaves. The results are almost in agreement with the literature [Citation45–48]. Carbohydrates, reducing sugars which are present in the plant extracts might be responsible for combustion in green synthesis.
Table 3. Results of phytochemical screening of the aqueous leaf extracts of A. indicum, M. azedarach and I. tinctoria.
GC-MS analysis
GC-MS of the leaves of A. indicum, M. azedarach and I. tinctoria are presented in ), respectively. (Mass spectra of the leaf extracts of A. indicum, M. azedarch and I. tinctoria are provided in the Supplementary file). The fragmentation patterns of the mass spectra were compared with those of the known compounds stored in the National Institute of Standards and Technology (NIST) research library. The phytocomponents detected in the GC-MS analysis are presented in . The identification of photochemical compounds was based on peak area, retention time, molecular weight and molecular formula. It is to be noted that these phytochemicals do not contribute to the anticancer property as they are not present in their original phase in the finished product (mass spectra of the aqueous extracts of the leaves are shown in Supplementary file as Figure 11).
Figure 6. GC-MS analysis of the leaf extracts of (a) A. Indicum, (b) M. azedarach and (c) I. tinctoria.
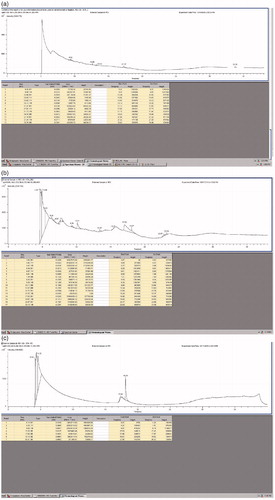
Table 4. Results of GC-MS analysis of the leaf extracts of A. indicum, M. azedarach and I. tinctoria.
Anticancer activity
Results of MTT assay in DU-145 and Calu-6 cells indicate that all the samples of ZnO NPs induced dose dependent toxicity in both the cells. Overall, the samples prepared using aqueous leaf extracts as fuels exhibited better activity than the sample prepared using a chemical as fuel. IC50 values (the concentration of ZnO NPs needed to inhibit cell growth by 50%) for cytotoxicity test of DU-145 and Calu-6 were derived from a nonlinear regression analysis (curve fit) based on sigmoid dose response curve (variable) and computed using Graph Pad Prism 5 (Graphpad, San Diego, CA). Determination of IC50 values of ZnO (LA), ZnO (IT-2), ZnO (MA-2) and ZnO (IT-2) on DU-145 are shown in ) respectively and the determination of IC50 values of ZnO (LA), ZnO (IT-2), ZnO (MA-2) and ZnO (IT-2) on Calu-6 are shown in ), respectively. The IC50 values for DU-145 and Calu-6 after 24 h are presented in . Among all the samples, ZnO (MA-2) prepared using the aqueous extract of leaves of M. azedarach showed higher anticancer activity in both DU-145 and Calu-6 cells. The marked cytotoxicity against DU-145 and Calu-6 cancer cells suggests an exciting potential for ZnO NPs prepared using a biofuel as novel alternatives to cancer chemotherapy. The higher anticancer activity of ZnO NPs synthesized using bio-fuels over the anticancer activity of ZnO NPs synthesized using a chemical as fuel might be attributed to their higher surface area to volume ratio.
Figure 7. Determination of IC50 values of (a) ZnO (LA), (b) ZnO (AI-2), (c) ZnO (MA-2) and (d) ZnO (IT-2) on DU-145 cell lines [data represent mean ± SD (standard deviation)].
![Figure 7. Determination of IC50 values of (a) ZnO (LA), (b) ZnO (AI-2), (c) ZnO (MA-2) and (d) ZnO (IT-2) on DU-145 cell lines [data represent mean ± SD (standard deviation)].](/cms/asset/0c4d2482-a0ae-487a-a592-0e98dc83bc78/ianb_a_1351982_f0007_c.jpg)
Figure 8. Determination of IC50 values of (a) ZnO (LA), (b) ZnO (AI-2), (c) ZnO (MA-2) and (d) ZnO (IT-2) on Calu-6 cell lines (data represent mean ± SD).
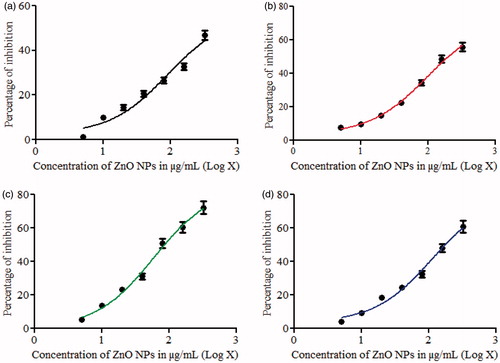
Table 5. IC50 (μg/mL) of ZnO NPs on DU-145 and Calu-6 cells.
Literature shows that ZnO NPs induce cytotoxicity in a cell specific and proliferation dependent manner by rapidly dividing cancer cells being the most susceptible and quiescent cells being the least sensitive [Citation49,Citation50]. However, the anticancer activity of ZnO NPs, in particular, the mechanism of apoptosis in cancer cells due to ZnO NPs is still not clear. These results are promising and hence the internal mechanism needs to be discovered in the longer run.
Haemolysis analysis
Haemolysis is basically related to the release of haemoglobin into the plasma due to the damage of erythrocyte membrane. If haemolysis occurs to a greater extent it can lead to dangerous pathological conditions. Therefore, all biomedical products should be tested for haemolytic properties [Citation51]. The results of cytotoxicity studies by haemolysis assay are presented in . Since 5% haemolysis is considered as permissible limit for biomaterials, up to a concentration of 2.5 mg/mL of ZnO NPs can be taken for haemolysis activity [Citation52]. Therefore, these results indicate that ZnO NPs synthesized using different fuels in our studies are biocompatible in nature at their lower concentration. These results are in well agreement with the literature [Citation42]. Hence, within the limitation of this study it can be concluded that the concentrations of ZnO NPs employed in the present anticancer studies are biocompatible.
Table 6. Haemolysis of blood by ZnO NPs.
Conclusions
ZnO NPs were prepared by low-cost SCS using lactose and aqueous extracts of the leaves of A. indicum, M. azedarach and I. tinctoria as fuels. The samples were characterized by PXRD, SEM, TEM, XPS, TGA and BET. Anticancer activity by MTT assay demonstrates that ZnO NPs induce dose-dependent toxicity in DU-145, Calu-6 cells. Haemolysis studies confirm that ZnO NPs are bio compatible at lower concentrations. It is evident from the studies that the ZnO NPs prepared using biofuels exhibited higher anticancer activity than the ZnO NPs prepared using lactose as reducing agent by SCS. This study successfully demonstrates the convenient utilization of biofuels to get potential anticancer and bio compatible ZnO NPs. These results indicate that plant extracts could indeed be more advantageous than a chemical such as lactose for the preparation of ZnO NPs by SCS method. It also raises the question whether the use of commercially available chemical reagents should indeed be considered for large scale production of NPs. However, more studies in this direction would be required for needful justifications/assumptions.
Supplementary_file.doc
Download MS Word (1.2 MB)Acknowledgements
The authors G. K. Prashanth, Dr. G. M. Krishnaiah, H. M. Sathyananda, Dr. H. G. Nagendra and Dr. C. Rajendra Singh thank the Management of Sri KET and Principal of Sir MVIT for the support and encouragement extended towards this project. The authors thank Prof. Avinash V. Mahajan, IITB for PXRD measurements. The authors acknowledge SAIF, IITB for TEM and GCMS, CIF, Pondicherry University for SEM, IIT Kanpur for Thermogravimetric and National Center for Nanosciences and Nanotechnology, University of Mumbai for XPS measurements.
Disclosure statement
The authors declare that they have no conflict of interest.
References
- Kumar R, Kumar G, Akhtar MS, et al. Sonophotocatalytic degradation of methyl orange using ZnO nano-aggregates. J Alloys Compounds. 2015;629:167–172.
- Petkova P, Francesko A, Perelshtein I, et al. Simultaneous sonochemical-enzymatic coating of medical textiles with antibacterial ZnO nanoparticles. Ultrasonics Sonochem. 2016;29:244–250.
- Lu P-J, Huang S-C, Chen Y-P, et al. Analysis of titanium dioxide and zinc oxide nanoparticles in cosmetics. J Food Drug Analysis. 2015;23:587–594.
- Xiong H-M. ZnO nanoparticles applied to bioimaging and drug delivery. Adv Mater Weinheim. 2013;25:5329–5335.
- Padalia H, Chanda S. Characterization, antifungal and cytotoxic evaluation of green synthesized zinc oxide nanoparticles using ziziphus nummularia leaf extract. Artif Cells Nanomed Biotechnol. 2017. DOI:10.1080/21691401.2017.1282868
- Antoine TE, Mishra YK, Trigilio J, et al. Prophylactic, therapeutic and neutralizing effects of zinc oxide tetrapod structures against herpes simplex virus type-2 infection. Antiviral Res. 2012;96:363–375.
- Yadavalli T, Shukla D. Role of metal and metal oxide nanoparticles as diagnostic and therapeutic tools for highly prevalent viral infections. Nanomed Nanotechnol Biol Med. 2017;13:219–230.
- Bayrami A, Parvinroo S, Habibi-Yangjeh A, et al. Bio-extract-mediated ZnO nanoparticles: microwave-assisted synthesis, characterization and antidiabetic activity evaluation. Artif Cells Nanomed Biotechnol. 2017. DOI:10.1080/21691401.2017.1337025
- Nehru LC, Sanjeeviraja C. ZnO nanoparticles by citric acid assisted microwave solution combustion method. J Ceramic Processing Res. 2013;6:712–716.
- Rao M, Liebenow C, Jayalakshmi M, et al. High-temperature combustion synthesis and electrochemical characterization of LiNiO2, LiCoO2 and LiMn2O4 for lithium-ion secondary batteries. J Solid State Electrochem. 2001;5:348–354.
- Patil KC, Aruna SC, Mimani T. Combustion synthesis: an update. Curr Opin Solid State Mater Sci. 2002;6:507–512.
- Patil KC, Hegde MS, Tanu R, Aruna ST. 2008. Chemistry of Nanocrystalline Oxide Materials. Singapore: World Scientific.
- Parashar UK, Saxena PS, Srivastava A. Bioinspired syntheis of silver nanoparticles. Digest J Nanomater Biostruct. 2009;4:159–166.
- Begum NA, Mondal S, Basu S, et al. Biogenic synthesis of Au and Ag nanoparticles using aqueous solutions of Black Tea leaf extracts. Colloids Surf B Biointerfaces. 2009;71:113–118.
- Forough M, Farhad K. Biological and green synthesis of silver nanoparticles. Turkish J Env Sci. 2010;34:281–287.
- Mukherjee S, Sushma V, Patra S, et al. Green chemistry approach for the synthesis and stabilization of biocompatible gold nanoparticles and their potential applications in cancer therapy. Nanotechnology. 2012;23:455103.
- Mukherjee S, Vinothkumar B, Prashanthi S, et al. Potential therapeutic and diagnostic applications of one-step in situ biosynthesized gold nanoconjugates (2-in-1 system) in cancer treatment. RSC Adv. 2013;3:2318–2329.
- Mukherjee S, Chowdhury D, Kotcherlakota R, et al. Potential theranostics application of biosynthesized silver nanoparticles (4-in-1 system). Theranostics. 2014;4:316–335.
- Lee J, Kim HY, Zhou H, et al. Green synthesis of phytochemical-stabilized Au nanoparticles under ambient conditions and their biocompatibility and antioxidative activity. J Mater Chem. 2011;21:13316–13326.
- Shankar SS, Ahmad A, Pasricha R, et al. Bioreduction of chloroaurate ions by geranium leaves and its endophytic fungus yields gold nanoparticles of different shapes. J Mater Chem. 2003;13:1822–1826.
- Ahmad A, Senapati S, Khan MI, et al. Extra-/intracellular biosynthesis of gold nanoparticles by an alkalotolerant fungus, Trichothecium sp. J Biomed Nanotechnol. 2006;1:47–53.
- Patra S, Mukherjee S, Barui AK, et al. Green synthesis, characterization of gold and silver nanoparticles and their potential application for cancer therapeutics. Mater Sci Engg C. 2015;53:298–309.
- Noruzi M, Zare D, Khoshnevisan K, et al. Rapid green synthesis of gold nanoparticles using Rosa hybridapetal extract at room temperature. Spectrochimica Acta Part A: Mol Biomol Spectrosc. 2011;79:1461–1465.
- Montes MO, Mayoral A, Deepak FL, et al. Anisotropic gold nanoparticles and gold plates biosynthesis using alfalfa extracts. J Nanopart Res. 2011;13:3113–3121.
- Prashanth GK, Prashanth PA, Bora U, et al. In vitro antibacterial and cytotoxicity studies of ZnO nanopowders prepared by combustion assisted facile green synthesis. Karbala Int J Modern Sci. 2015;1:67–77.
- Saravanakumar A, Peng MM, Ganesh M, et al. Low-cost and eco-friendly green synthesis of silver nanoparticles using Prunus japonica (Rosaceae) leaf extract and their antibacterial, antioxidant properties. Artif Cells Nanomed Biotechnol. 2016;45:1165–1171.
- Sathishkumar G, Logeshwaran V, Sarathbabu S, et al. Green synthesis of magnetic Fe3O4 nanoparticles using Couroupita guianensis Aubl. fruit extract for their antibacterial and cytotoxicity activities. Artif Cells Nanomed Biotechnol. 2017. DOI:10.1080/21691401.2017.1332635
- Singh H, Du J, Yi T-H. Green and rapid synthesis of silver nanoparticles using Borago officinalis leaf extract: anticancer and antibacterial activities. Artif Cells Nanomed Biotechnol. 2016. DOI:10.1080/21691401.2016.1228663
- Yoganarasimhan SN. Medicinal plants of India. Bangalore (India): Cyber Media; 2010.
- Xiao J, Zhang Q, Gao Y-Q, et al. Secondary metabolites from the endophytic Botryosphaeria dothidea of Melia azedarach and their antifungal, antibacterial, antioxidant and cytotoxic activities. J Agric Food Chem. 2014;62:3584–3590.
- Vijayan M, Jacob K, Govindaraj Y. Antibacterial activity and mutagenicity of leaves of Indigofera tinctoria Linn. J Exp Integr Med. 2012;2:263–269.
- Abdolmohammadi MH, Fallahian F, Fakhroueian Z, et al. Application of new ZnO nanoformulation and Ag/Fe/ZnO nanocomposites as water-based nanofluids to consider in vitro cytotoxic effects against MCF-7 breast cancer cells. Artif Cells Nanomed Biotechnol. 2017. DOI:10.1080/21691401.2017.1290643.
- Prashanth GK, Prashanth PA, Gadewar M, et al. In vitro antibacterial and anticancer studies of ZnO nanoparticles prepared by sugar fueled combustion synthesis. Adv Mat Lett. 2017;8:24–29.
- Kang T, Guan R, Chen X, et al. In vitro toxicity of different-sized ZnO nanoparticles in Caco-2 cells. Nanoscale Res Lett. 2013;8:496.
- Prashanth GK, Prashanth PA, Nagabhushana BM, et al. In vitro antimicrobial, antioxidant and anticancer studies of ZnO nanoparticles synthesized by precipitation method. Adv Sci Engng Med. 2016;8:306–313.
- Guan R, Kang T, Lu F, et al. Cytotoxicity, oxidative stress and genotoxicity in human hepatocyte and embryonic kidney cells exposed to ZnO nanoparticles. Nanoscale Res Lett. 2012;7:602.
- Prashanth GK, Prashanth PA, Yadavalli T, et al. ZnO nanopellets have selective anticancer activity. Mater Sci Eng C. 2016;62:919–926.
- Prashanth GK, Prashanth PA, Bora U, et al. Antitubercular activity of ZnO nanoparticles prepared by solution combustion synthesis using lemon juice as bio-fuel. Mater Sci Eng C. 2017;75:1026–1033.
- Kokate CK. Practical pharmacognosy. New Delhi (India): Vallabh Prakashan; 2000.
- Harbone JB. Phytochemical methods. London (UK): Chapman and Hall; 1999.
- Tiwari P, Kumar B, Kaur M, et al. Phytochemical screening and extraction: a review. Internationale Pharmaceutica Sciencia. 2011;1:98–106.
- Das D, Nath BC, Phukon P, et al. Synthesis of ZnO nanoparticles and evaluation of antioxidant and cytotoxic activity. Colloids Surf B Biointerfaces. 2013;111:556–560.
- Mishra A, Chaudhary N. Study of povidone iodine loaded hydrogels as wound dressing material. Trends Biomater Artificial Organs. 2010;23:122–128.
- Salunkhe AB, Khot VM, Phadatare MR, et al. Combustion synthesis of cobalt ferrite nanoparticles-Influence of fuel to oxidizer ratio. J Alloys Compounds. 2012;514:91–96.
- Saraswathi R, Lokesh Upadhyay P, Venkatakrishnann R, et al. Phytochemical investigation, analgesic and anti inflamaatory activity of Abutilon Indicum Linn. Int J Pharmacy Pharm Sci. 2011;3:154–156.
- Bhargava S. Phytochemical screening and evaluation of its repellent activity of Melia azedarach L. Indochemical J Pharm Res. 2013;3:4310–4318.
- Ahmed MF, Rao AS, Ahemad SR, et al. Phytochemical studies and antioxidant activity of M. azedarach leaves by DPPH scavenging assay. Int J Pharm Appl. 2012;3:271–276.
- Chopra C. A review of medicinal plants from India. Indian J Pharmacol. 1956;8:416–420.
- Premanathan M, Karthikeyan K, Jeyasubramanian K, et al. Selective toxicity of ZnO nanoparticles toward Gram-positive bacteria and cancer cells by apoptosis through lipid peroxidation. Nanomedicine: Nanotechnol Biol Med. 2011;7:184–192.
- Hanley C, Layne J, Punnoose A, et al. Preferential killing of cancer cells and activated human T cells using ZnO nanoparticles. Nanotechnology. 2008;19:295103.
- Dobrovolskaia M, Clogston J, Neun B, et al. Method for analysis of nanoparticle hemolytic properties in vitro. Nano Lett. 2008;8:2180.
- Singhal JP, Ray AR. Synthesis of blood compatible polyamide block copolymers. Biomaterials. 2002;23:1139–1145.