Abstract
Transplantation of stem cells using biocompatible nanofibrous scaffolds is a promising therapeutic method for treating Diabetic Mellitus. The aim of this study was to derive insulin-producing cells (IPCs) from conjunctiva-derived mesenchymal stem cell (CJMSCs) and to compare the functionality of differentiated IPCs in a three-dimensional (3D) culture with 2D. Furthermore, the effects of hydrophobicity of scaffold on IPCs differentiation were examined. Scanning electron microscopy (SEM), quantitative real times PCR (qPCR), Immunostaining and flow cytometry were used to analyze fabricated scaffold and the presence of IPCs. Functional maturity of differentiated cells was determined by measuring insulin release and the creation of IPCs was confirmed via gene and protein expression. In this study, the induced CJMSCs were morphologically similar to pancreatic islet-like cells. The expression of the islet-associated genes (glucagon, insulin and Pdx-1) and the insulin release (2.5-fold) in 3D-cultured cells was significantly higher than the 2D. The expression of IPCs genes was significantly higher in CJMSCs differentiated on plasma-treated nanofibers compared to those on untreated scaffolds. In conclusion, the results show that CJMSCs might be a new source for Diabetic Mellitus therapy and the nanofibrous scaffold could be used as a potential cell carrier for islet tissue engineering.
Introduction
Diabetes mellitus can arise from autoimmune obliteration of pancreatic beta-cells (Type 1) or from a lack of insulin insufficiency or resistance (Type 2) [Citation1]. For treatment of diabetes mellitus administration of insulin is the gold standard. However, this treatment does not cure the disease and the patient is exposed to complications arisen from hyperglycemia in the short and long time such as, severe dehydration, ketoacidosis, neuropathy, blood vessel degeneration and blindness [Citation2]. Therefore, there is a great need to progress alternative promising approach to treating diabetes mellitus [Citation3]. Although islet transplantation has been revealed to renovate normoglycemia [Citation4], a limited source of human islet tissues, cost, and surgical complications prevents this treatment from being used in patients with type 1 diabetes mellitus [Citation5].
Due to this disadvantage, many researchers have made efforts to investigate alternate cell sources for treating diabetes mellitus. To date, differentiation of different cell sources such as embryonic stem cells (ESC), hepatic oval cells, cells within spleen, pancreas [Citation6], bone marrow [Citation7] and adipose tissue [Citation8], into insulin-producing cells; IPCs) have been reported. However, despite their differentiating potency, the clinical use of these cells has presented problems including risk of rejection after cell transplantation, the ethical/legal issues, risk of teratoma formation and painful cell harvesting procedures [Citation9,Citation10]. The previous study showed that two dimensional cell culture is not promising for upholding the islet functionality, due to significant role of interactions between islets and extracellular matrix (ECM) in survival [Citation11], proliferation, insulin secretion and spherical morphology of islet cells [Citation12]. The microenvironment is a critical factor in the differentiation, survival of cells and directly impacts on cell function. Tissue engineering via combination of cells and biomaterials (as 3D) try to generate insulin-producing biohybrid pancreatic construct. 3D culture is important in cell engineering for the gaining of mature cells [Citation13] by improving cell–cell and cell–matrix interactions (as in vivo microenvironment) [Citation14] that directly impacts on cell function. The purpose of this study was to generate insulin-producing islet-like clusters from conjunctiva-derived mesenchymal stem cells (CJMSCs) using appropriate growth factors and to compare the generation of IPCs in 3D scaffold and on two-dimensional (2D) culture system.
Materials and methods
Cell isolation and differentiation into mesenchymal cells
CJMSCs were isolated according to a protocol adapted by Nadri et al. [Citation15,Citation16]. In brief, 2–3 mm2 of conjunctiva biopsy was treated in supplemented hormonal epithelial medium (SHEM). The stromal tissue slices were cultured in DMEM/F-12 supplemented with 5 mg/mL insulin, 4 ng/mL basic-FGF, 10 ng/mL human LIF and 10% knockout serum, and was incubated. The isolated cells were treated with osteogenic (DMEM including 10 nM dexamethasone (Sigma), 50 mg/mL ascorbic acid 2-phosphate, 10 mM b-glycerophosphate (Sigma)), adipogenic (DMEM including 100 nM dexamethasone (Sigma), 50 mg/mL indomethacine (Sigma)), chondrogenic (DMEM including bone morphogenetic protein-6 (BMP-6), 10 ng/mL transforming growth factor- sz3 (Sigma), 10−7 M dexamethasone (Sigma), 50 mg/mL insulin–transferrin–selenium (Gibco) and 50 mg/mL ascorbate-2-phosphate (Sigma)) medium for 21 days.
Fabrication of electrospun nanofibrous scaffolds
Nanofibrous PCL scaffolds were fabricated using an electrospinning machine [Citation17]. PCL (12% w/v) was dissolved in the chloroform/DMF solution. A plasma generator (40 kHz frequency; Diener Electronics, Germany) was used for surface modification of the nanofibers. Pure oxygen was introduced into the reaction chamber of the system at 0.4 mbar pressure and purged for 5 min.
Scanning electron microscopy (SEM)
The cell–scaffold constructs were fixed in glutaraldehyde, dehydrated, dried and sputter coated with gold. Constructs were observed using a scanning electron microscope (Seron technology, AIS2100).
Differentiation of CJMSCs into functional islet-like cells
The cells (CJMSCs) were cultured in Dulbecco’s modified Eagle’s medium (DMEM)–high glucose, 5 mmol/l β-mercaptoethanol (Sigma) and moved into cell culture dishes for 1 day. The cells were cultured in DMEM–high glucose supplemented with 10 ng/ml epidermal growth factor (EGF; Chemicon), 10 ng/ml basic fibroblast growth factor (Invitrogen), 2% B27 supplement (Stemcell Technologies Inc.), 1 mmol/l β-mercaptoethanol and 10 mmol/l nicotinamide (Sigma) for 6 days. Next, the cells induced with DMEM–high glucose plus 10 ng/ml EGF, 50 ng/ml Activin A (Sigma), 10 mmol/l nicotinamide, 2% B27, and 1 mmol/l β-mercaptoethanol, for 6 days with medium changes every 2 days. In final step, the cells were treated with DMEM–low glucose containing 10 ng/ml EGF, 10 nmol/l exendin-4 (Sigma), 2% B27 and 1 mmol/l β-mercaptoethanol for 3 days.
ELISA assay
The quantities of insulin in supernatants secreted from differentiated cells (in 2 and 3D culture systems) and cells (undifferentiated cells) were determined by ELISA using a commercially available ELISA kit (AccuBind Rapid insulin test, Monobind Inc., Lake Forest, CA) according to the manufacturer’s instructions after treatment with different concentration of glucose (5, 15 and 25 mM) for 1 h. Determinations were carried out in triplicate.
Immunofluorescent staining and flow cytometry analysis
The cells were fixed and permeabilized (with Triton X-100). Then the cells were reacted with primary antibodies for insulin (abcam), C-peptide (abcam), Glucagon (abcam) and Pdx-1 (abcam) at 4 °C overnight, and subsequently reacted with secondary antibody (the FITC-conjugated anti mouse IgG (Sigma)) at room temperature(RT) for 3 h. The cells were incubated with diaminobenzidine (DAB) solution (Sigma) for 30 s and then observed by Inverted Fluorescence Microscope. Furthermore, the cells that reacted with primary and secondary antibody were analyzed by flow cytometry machines.
Real-time polymerase chain reaction
Total RNA was extracted using GeneMATRIX Universal RNA Purification Kit (eurx®) and random hexamer primed cDNA synthesis was carried out using PrimeScript™ RT reagent Kit (Perfect Real Time) (Takara, Japan). The cDNA was used for 40 cycles PCR in Applied Biosystems™ Real-Time PCR Instruments. The cycling parameters for qPCR were as follows: 10 min at 95 °C for initial denaturation, followed by 40 cycles of 15 s at 95 °C and 30 s at 58 °C, 30 s at 72 °C, and finally, melting of PCR products at 60–95 °C to confirm PCR specificity using melting curve analysis. The primers are presented in .
Table 1. Primer sequences employed in this project.
Results
Cell culture of conjunctiva mesenchymal stem cells
The spindle-shaped mesenchymal stem cells from human conjunctiva tissue were isolated by adhesion of their onto the tissue-culture polystyrene (TCPS) plates. After 2 weeks, the cells with spindle-shaped morphology appeared on TCPS (Supplementary Figure 1a). To approve their mesenchymal nature, the cells were incubated with osteo-, adipo- and chondro-induction media, and their differentiation was approved via alizarin red (for osteogenic differentiation), oil red (for adipogenic differentiation) and alcian blue (for chondrogenic differentiation) staining (Supplementary Figure 1b–d).
Morphological changes of CJMSCs differentiation
Although under differentiation, these spindle-like cells changed rapidly into round, circular shape and formed clusters, these cluster-like cells are maintained in all steps of differentiation. Cellular aggregation occurred after induction with β-mercaptoethanol in the first step of differentiation and complete aggregates with core dense of cells were formed in differentiation (). In the next step of differentiation, the rate of cell migration from main cluster (with denser core) increased, the small clusters gradually were separated from main cluster and the center of main cluster changed into denser core. Meanwhile, some new islet-like cell aggregates started to appears. In inversed microscope, undifferentiated cells were typical of adherent spindle and fibroblast-like cells and did not form any cluster. SEM studies indicated that PCL-based nanofibers resulted in a scaffold composed of uniform fibers and the cells in the 3D (under differentiation media) showed round-shaped cluster morphology similar to pancreatic islet ().
Figure 1. Morphologic changes in the cells on TCPS at different time periods of differentiation. (a) Day 0, the cells were spindle shaped before induction (original magnification 100×); (b) Day 1, the induced cells turned shorter and changed into round cells and islet-like cell (ILC) clusters appeared (original magnification 100×); and (c) Day 6, the center of large cell cluster was dense and its peripheral migrated to outer part of cluster; (d) large ILC separated to small cell clusters. Scale bar 100 uM.
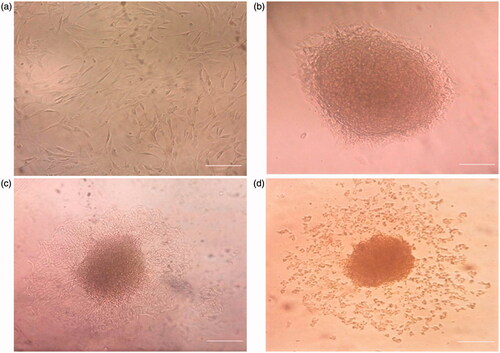
Contact angle analysis
The contact angle obtained from the untreated PCL scaffold was 120 and for the plasma treated scaffolds with zero water contact angle and rapid absorbance of the water droplet. This confirmed that the surface of scaffold was hydrophilic.
Immunofluorescence staining and flow cytometry analysis
The result of fluorescent microscopy showed that mature islet cell proteins such as insulin, c-peptide, Glucagon and PDX-1 proteins were detected in the CJMSCs cultured on either nanofiber scaffold (as 3D experimental culture) or TCPS dishes (as 2D experimental culture) () after treatment with induction medium for 16 days. No positive cells were recognized for these markers among our untreated CJMSCs (data not shown).
Figure 3. Immunostaining of CJMSCs-derived IPCs. The cells were seeded on 3D scaffold and TCPS were then maintained in induction medium for 16 days and were analyzed for expression of insulin, c-peptide, glucagon and Pdx-1 markers. Cells were co-stained with 4,6-diamidino-2-phenylindole to visualize nuclei (blue). Scale bar 100 μM).
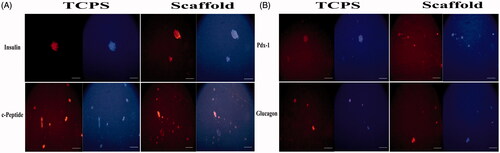
Furthermore, the treated cells in 2 D experimental culture group were analyzed for the expression of a four main markers in pancreatic differentiation pathway using flow cytometry. As shown in , nearly 27.1%, 23.8%, 20.6% and 22.6% of treated cells expressed insulin, c-peptide, glucagon and Pdx-1 proteins, respectively.
Insulin release in response to glucose stimulation
The cultured CJMSCs in the control groups showed very low release of insulin in the presence of glucose challenge. Elisa analysis showed no significant differences between 2D, 3D and cell control in a 5 and 15 mM glucose concentration. Insulin secretion of the 3D culture of CJMSCs-derived cluster cells significantly increased in a 25 mM glucose (2.5-fold; p < .01) compared to the 2D culture of CJMSCs-derived cluster cells ().
Figure 5. In vitro functional (insulin ELISA) assay in response to different concentrations of glucose.
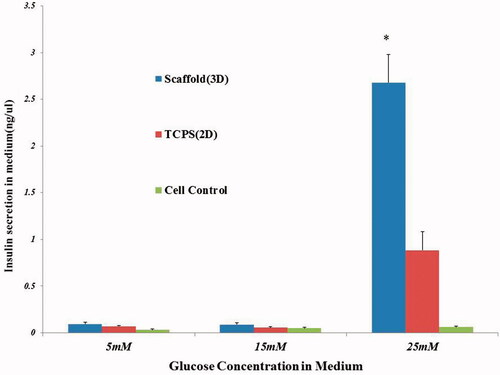
Gene expression
Gene expression profiles for islet cell differentiation were studied using a quantitative real-time RT-PCR (qPCR).
Although Pdx-1, glucagon, and insulin gene expressions were higher in differentiated CJMSCs compared to untreated cells, we did not detect any expression of Pdx-1, glucagon and insulin genes in undifferentiated CJMSCs (control).
At the end of days 7 and 16 differentiation, RT-qPCR was used for analysis of differentiation rate on the plasma treated PCL scaffold (p-PCL) and TCPS ().
Figure 6. Gene expression profile of cells differentiated on P-PCL and TCPS on day 7 and 16. The cells were maintained in induction medium for 7 and 16 days and analyzed for expression of pancreatic markers. The column ratio is the expression rate of genes compared with untreated cells. GAPDH is shown as a control for RNA sample quality. Rest software was used for gene expression analysis using real-time PCR data. Asterisks show significance expression rate. *p ≤ .05.
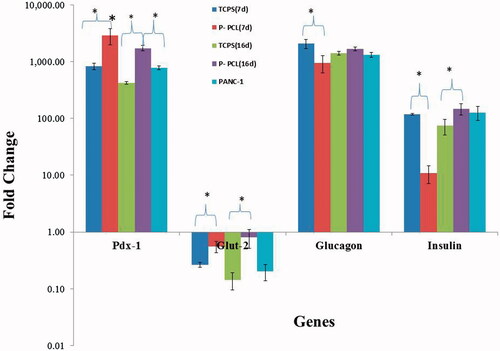
qPCR analysis demonstrated that Pdx-1 gene expression was higher in CJMSCs differentiated on fibrous scaffold compared to TCPS dishes and positive pancreatic cell line (PANC-1).
Pdx-1 gene expression was significantly higher in CJMSCs differentiated on fibrous scaffold (1.679 fold; p ≤ .002) and TCPS (1.969 fold; p ≤ .01) after day 7 of differentiation compared to fibrous scaffold and TCPS after 16 days. qPCR analysis showed that Glucose transporter 2 (GLUT2) gene expression was significantly higher in CJMSCs differentiated on fibrous scaffold (2.08 fold; p ≤ .001; and 5.696 fold; p ≤ .005) after day 7 and 16 of differentiation compared to TCPS, respectively. Insulin (1.995-fold; p ≤ .001) gene expressions were higher in cells on fibrous scaffolds after day 16 of differentiation.
As shown in , Pdx-1(1.828-fold; p ≤ .014) and Glut-2 (2.184; p ≤ .01) gene expressions were higher in cells on plasma treated scaffold after day 16 of differentiation compared to Untreated scaffold. However, we did not find any significant difference in insulin expression between untreated cells compared with treated ones.
Figure 7. Gene expression profile of cells differentiated on plasma treated PCL and untreated PCL scaffold on day 7 and 16. The cells were maintained in induction medium for 7 and 16 days and analyzed for expression of pancreatic markers. The column ratio is the expression rate of genes compared with cells differentiated on TCPS. GAPDH are shown as a control for RNA sample quality. Rest software was used for gene expression analysis using real-time PCR data. Asterisks show significance expression rate. *p ≤ .05.
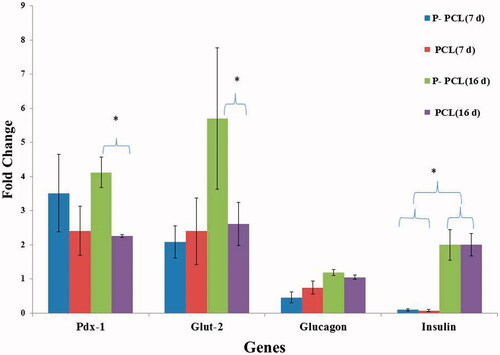
Discussion
This research was assumed to study suitable stem cell substrate interactions for islet tissue engineering. Despite the fact that the cells from pancreatic tissue are considered to be a well-accepted source of stem cells for islet regeneration, the clinical use of these cells has presented problems [Citation18,Citation19]. Due to these results, many researchers have attempted to investigate alternate cell sources. In this respect, the recognition of an available stem cell source would propose the advantage of autologous stem cell therapies. In this research, we examined islet differentiation of mesenchymal stem cells, isolated from human conjunctiva stromal tissue.
The phenotypic characterization of these mesenchymal stem cells has been well recognized. The CJMSCs have a spindle-shaped morphology and are capable of multiple mesodermal lineage differentiation. In addition, CJMSCs can also be induced to express neuronal genes consistent with a neurogenic phenotype and were differentiated into mesenchymal lineages (osteogenic, adipogenic and chondrogenic) and maintained these capacities up to passage 10. High proliferation capacity, clonogenic potential, spindle-shaped morphology, differentiation into mesenchymal lineages and neuronal cells of CJMSCs could guide researchers to use CJMSCs as a model source for clinical trial in the future [Citation15]. The most imperative properties of CJMSCs were their expression of Oct-4, Rex-1 and Nanog (as undifferentiated stem cells markers) and lineage-specific markers like neurocyte [Citation16].
Stem cells from various sources [Citation6–8,Citation20] have been differentiated to IPCs. The simplicity of availability and surgical procedure for accessing conjunctiva tissue makes it an attractive source as autograft for clinical application without any ethical issues [Citation21]. The plasticity of MSCs for differentiating into islet-like cells has been reported [Citation9,Citation22]. In this experiment, CJMSCs expressed islet genes after treatment with induction medium.
On the basis of this, in the present study, in the first step, the cells were incubated with β-mercaptoethanol for induction of cell aggregation and spherical morphology. CJMSCs are a type of cells with robust adhesion affinity to cell culture plate. In this study after the induction of cells with β-mercaptoethanol, cell aggregations were seen on TCPS as an adherent cluster. However, cell aggregations (cell clusters) were efficiently induced into functional pancreatic islet-like cell clusters due to the fact that cell–cell contact between pancreatic beta-cells is vital for upholding their survival and increasing insulin secretion [Citation23]. However, cells in cell clusters, affected by adjacent cells via paracrine, simply harmonized each other and differentiated [Citation8]. Cell clusters proved that the environment within the cell clusters was able to promote cell differentiation.
In this project, we used reagent factors including bFGF, EGF, betacellulin and Activin A for islet differentiation. bFGF and EGF play a key role in cellular proliferation, IPC differentiation and cell survival. They improve the development of functional beta cells and play a significant role in inducing beta cell neogenesis [Citation24]. bFGF, secreted by endocrine precursor cells, was found to be the most effectual chemo-attractant in the clustering of pancreatic cells [Citation25]. Activin proteins, as members of the transforming growth factor-beta (TGF-β) superfamily, can enhance glucose-stimulated insulin secretion in beta-cells [Citation26] and proliferation of beta- and ductal-cells [Citation27,Citation28]. The high expression of endocrine genes such as Pdx-1, insulin and glucagon, after induction by activin-A in mouse ESC have been documented [Citation29]. Beta-cellulin, either alone or together with Activin-A, has resulted in differentiation of stem cells (bone marrow, liver and pancreatic origins) into IPCs [Citation30,Citation31].
The high amount of antioxidants present in the B27, as neuronal supplements, could help to support more healthy microglia and neuronal cells in culture [Citation32]. In the present study, we used B27 medium for differentiation of CJMSCs into IPCs. The latest study showed that neural-positive cells have capability to generate cell clusters, pancreatic islet-like cell clusters and functional islet-like cell clusters [Citation33]. In the early days of differentiation, cells look like cluster-like morphology and the size of ILCs enhanced. However, in the final day of differentiation, small islet clusters are separated from larger islets with regard to higher oxygen consumption and reduced necrosis [Citation34]. We approved a four-stage protocol with growth factor in serum-free high-glucose medium for islet differentiation of cells. Various researchers have reported that serum-free condition and high-glucose medium increase the differentiation of stem cells to insulin-producing cells.
The connection between islets and extracellular matrix (ECM) is vital for islet survival and function [Citation11,Citation12]. In monolayer culture of islet-like cells, low cell–matrix connection between cells and less spherical morphology of cells were reported. In the present study, the CJMSCs were induced to cluster formation and their differentiation capacity into IPCs were examined using qPCR, immunofluorescence assay and insulin secretion. Our results showed that CJMSCs were differentiated into IPCs. The differentiated CJMSCs also expressed different IPCs markers, in gene and protein levels, such as Pdx-1, glucagon, glut-2 and insulin [Citation12,Citation23]. Pdx1 (pancreatic and duodenal homeobox 1) is essential for pancreatic development (β-cell maturation and duodenal differentiation) [Citation35]. Pdx1 appears to play a role in the fating of endocrine cells, encoding for somatostatin and insulin, β-cell differentiation and β-cell survival [Citation36]. Glucagon was produced in the pancreas tissue (alpha cells). Glut-2, a marker for beta-cell maturity [Citation37], is a transmembrane carrier protein that facilitate glucose movement(controlled glucose uptake) across cell membranes [Citation38]. In pancreatic beta-cells, the capability of beta-cells to secretion of insulin is dependent on the presence of glut-2 [Citation37].
Concerning our results, we propose CJMSCs as a more desirable cell type for islet transplantation studies compared to bone marrow-derived MSCs (BMMSCs). The clinical use of BMMSCs has presented difficulties including painful aspiration and contamination with non-MSCs [Citation16] and also the differentiation rate of BMMSCs towards IPCs is 18.40% in the adherent culture [Citation39]. However, conjunctival biopsy is an easy procedure and the method for the isolation of CJMSCs is simple, no additional growth factors are required for maintaining proliferation capacity and can be easily followed by a beginner researcher [Citation40]. The results indicate that induction in plastic dishes can greatly promote CJMSCs to form pancreatic islet-like cell clusters. The islet-like cell clusters from CJMSCs expressed more Pdx-1 (22.6%), c-peptide (23.8%) and glucagon (20.6%) proteins and they secreted relatively higher insulin (27.1%).
We documented insulin secretion from differentiated CJMSCs into islet-like cells after the cells were exposed to 25 mM glucose. However, the previous study showed that glucose concentration (at 20–30 mM) is a potent stimulator for beta cells by secreting insulin in vitro [Citation10]. Insulin is created by the pancreatic islets (beta cells) that controls carbohydrate, protein and fat metabolism [Citation41].
This study, for the first time, confirmed the differentiation of adult CJMSCs into IPCs in vitro. However, further research including xeno or autologous cell transplantation is required to clarify the functionality of CJMSCs for the treatment of diabetic mellitus. Overall, the expression of islet-beta cell markers in induced CJMSCs suggests conjunctiva stromal-derived cells as a new source of multipotent MSCs for Diabetes mellitus.
More studies have revealed the role of Extra Cellular Matrix (ECM) in islet survival [Citation42,Citation43]. Three-dimensional (3D) culture is critical for mimicking the role of native ECM for proposing long-term islet survival and function [Citation44]. The present study develops the values of tissue engineering via combination of cells, biodegradable polymeric scaffolds and growth factors to produce insulin-producing biohybrid pancreatic construct. In the present study, CJMSCs were cultured on PCL nanofibrous scaffold (by electrospinning), and subsequently, differentiation of these cells into IPCs were examined.
In the present study, in the two steps of differentiation process, the expression rate of pancreatic genes was examined. Our results indicated some differences among the CJMSCs differentiated on fibrous scaffold from day 7 and 16 of differentiation. Compared to day 7, the higher expression of insulin was shown on fibrous scaffolds after day 16 of differentiation.
The pancreatic islet is composed of multiple cell types, including insulin-secreting β-cells, glucagon-secreting α-cells and somatostatin-secreting δ-cells. In human islets, the α-cells are not limited in the periphery but are rather dispersed throughout the islet [Citation45]. In the present study, differentiated CJMSCs expressed other pancreatic endocrine hormones like glucagon in addition to insulin. However, Islet morphology and paracrine interactions among alpha and beta are the main principles in determining functioning of ILCs. However, researchers have shown that 3D cultures improve contacts between beta cells and could enhance beta-cell survival and function [Citation43].
The results of this study demonstrated that 3D culture by using PCL can excellently increase differentiation of CJMSCs into IPCs. In this study, the existence of IPCs was established by morphology analysis, expression of islet specific genes and insulin secretion. The induced CJMSCs were morphologically similar to pancreatic islet-like cells. In agreement with the previous study that 3D dextran/gelatin scaffold was used for differentiation of adipose stem cells to islet-like clusters, our results showed that PCL promotes differentiation of a glucose-responsive beta-cell cluster from CJMSCs. In the present study, a significant increase in the secretion of insulin upon glucose stimulation in PCL fibrous scaffold (3D) CJMSCs-derived IPCs in comparison to TCPS (2D culture) was recognized. This finding designates that mature IPCs can be created from CJMSCs in 3D cultures.
However, future experiments including autologous cell transplantation are required to elucidate whether 3D conditions have inherent benefits in producing matured beta-cells from CJMSCs for the treatment of DM patients.
ILCs require an optimum microenvironment for maintaining its spherical architecture, viability and function, which could be provided by a favorable scaffold. Surface modification of biomaterials scaffold by ECM, laminin [Citation46] or physical plasma modification has a serious role in the differentiation of stem cells. Plasma surface modification is one of the methods of introducing functional groups (hydroxyl and carboxyl groups) to provide scaffolds with more hydrophilic character [Citation47]. Researchers have reported that scaffolds with hydrophilic surfaces show better cell adhesion and growth [Citation48]. However, there are not a lot of reports and research on the effect of hydrophilicity of scaffold on IPCs formation from stem cells. In the present study, we differentiated CJMSCs on both hydrophilic or hydrophobic scaffold and the rate expression of some genes involved in islet-like cells differentiation were examined. The result of contact angle showed that after plasma treatment the scaffold was hydrophilic. The results of this research showed that the wettability of scaffolds could affect the expression of some pancreatic genes in differentiated CJMSCs. The inhibitory effect of hydrophobic surface of substrate on the differentiation of cells has been established [Citation49]. CJMSCs differentiated on hydrophilic nanofibrous scaffolds demonstrate a higher rate of pancreatic differentiation, including Pdx-1 and glut-2 than hydrophobic nanofibrous scaffolds. In this study, the rate of CJMSCs differentiation for hydrophilic PCL nanofiber was higher than hydrophobic fibers. It might be that the high number of contact and surface energy and strong cell adhesion on scaffold influence the expression of genes. However, further research is required to approve the hypothesis about influence of surface energy on nanofibrous scaffold on the biochemical pathways and cellular signaling mechanisms that control cell differentiation. It seems that coating scaffold by proteins such as fibronectin, laminin and ECM was involved in pancreatic gene expression [Citation46]. The results suggested that surface hydrophilicity/hydrophobicity of the nanofibrous scaffold plays an essential role in CJMSCs pancreatic differentiation. The result suggests that the scaffold effectively mimics the role of ECM by providing a positive environment for IPCs. The positive environment obtainable by the scaffold is due to the presence of biodegradable polymer, the hydrophilicity domains, the lack of toxic agents and good porosity. The PCL scaffold is more favorable for maintaining the morphology, thereby sustaining the survival and function of IPCs. To our awareness, this is the first report indicating the enhanced insulin production of IPCs derived from conjunctiva mesenchymal stem cells on a 3D scaffold. It seems that cell–cell interaction in 3D matrix scaffold is essential for islet viability.
Conclusion
The present study has demonstrated the potential of CJMSCs to differentiate into mature IPCs on 2D culture and 3D scaffold using a four-stage protocol. IPCs on PCL scaffold exhibited higher levels of secreted insulin in response to glucose concentration compared with TCPS. The PCL scaffold reported in this study exhibited positive impact on islet survival and function in vitro. The overall findings signify that CJMSCs cultured on PCL scaffold in the existence of induction factors could be a favored method to produce viable IPCs, thus a promising strategy for islet culture for pancreatic tissue engineering. However, the results of this study demonstrated that the PCL scaffold can improve the differentiation of IPCs from human CJMSCs. It is suggested that adult conjunctiva mesenchymal stem cells are capable of differentiating into functional pancreatic cell phenotypes.
This could be an alternative therapeutic approach for diabetes mellitus in the future. The present report deals with only in vitro studies, and more detailed investigations about the signaling cues from the scaffold should be made in the future for in vivo experiments.
Nadri-et_al_supplemental_figure.jpg
Download JPEG Image (1.6 MB)Disclosure statement
No potential conflict of interest was reported by the authors.
Additional information
Funding
References
- Gan MJ, Albanese-O'Neill A, Haller MJ. Type 1 diabetes: current concepts in epidemiology, pathophysiology, clinical care, and research. Curr Probl Pediatr Adolesc Health Care. 2012;42:269–291.
- Kar P, Holt RI. The effect of sulphonylureas on the microvascular and macrovascular complications of diabetes. Cardiovasc Drugs Ther. 2008;22:207–213.
- Amer LD, Mahoney MJ, Bryant SJ. Tissue engineering approaches to cell-based type 1 diabetes therapy. Tissue Eng Part B Rev. 2014;20:455–467.
- Shapiro AM, Lakey JR, Ryan EA, et al. Islet transplantation in seven patients with type 1 diabetes mellitus using a glucocorticoid-free immunosuppressive regimen. N Engl J Med. 2000;343:230–238.
- Bonner-Weir S, Taneja M, Weir GC, et al. In vitro cultivation of human islets from expanded ductal tissue. Proc Natl Acad Sci USA. 2000;97:7999–8004.
- Zulewski H, Abraham EJ, Gerlach MJ, et al. Multipotential nestin-positive stem cells isolated from adult pancreatic islets differentiate ex vivo into pancreatic endocrine, exocrine, and hepatic phenotypes. Diabetes. 2001;50:521–533.
- Phadnis SM, Joglekar MV, Dalvi MP, et al. Human bone marrow-derived mesenchymal cells differentiate and mature into endocrine pancreatic lineage in vivo. Cytotherapy. 2011;13:279–293.
- Timper K, Seboek D, Eberhardt M, et al. Human adipose tissue-derived mesenchymal stem cells differentiate into insulin, somatostatin, and glucagon expressing cells. Biochem Biophys Res Commun. 2006;341:1135–1140.
- Chen LB, Jiang XB, Yang L. Differentiation of rat marrow mesenchymal stem cells into pancreatic islet beta-cells. World J Gastroenterol. 2004;10:3016–3020.
- Aloysious N, Nair PD. Enhanced survival and function of islet-like clusters differentiated from adipose stem cells on a three-dimensional natural polymeric scaffold: an in vitro study. Tissue Eng Part A. 2014;20:1508–1522.
- Bosco D, Meda P, Halban PA, et al. Importance of cell-matrix interactions in rat islet beta-cell secretion in vitro: role of alpha6beta1 integrin. Diabetes. 2000;49:233–243.
- Stendahl JC, Kaufman DB, Stupp SI. Extracellular matrix in pancreatic islets: relevance to scaffold design and transplantation. Cell Transplant. 2009;18:1–12.
- Takeuchi H, Nakatsuji N, Suemori H. Endodermal differentiation of human pluripotent stem cells to insulin-producing cells in 3D culture. Sci Rep. 2014;4:4488.
- Grayson WL, Ma T, Bunnell B. Human mesenchymal stem cells tissue development in 3D PET matrices. Biotechnol Prog. 2004;20:905–912.
- Nadri S, Kazemi B, Eslaminejad MB, et al. High yield of cells committed to the photoreceptor-like cells from conjunctiva mesenchymal stem cells on nanofibrous scaffolds. Mol Biol Rep. 2013;40:3883–3890.
- Nadri S, Soleimani M, Kiani J, et al. Multipotent mesenchymal stem cells from adult human eye conjunctiva stromal cells. Differentiation. 2008;76:223–231.
- Nadri S, Nasehi F, Barati G. Effect of parameters on the quality of core-shell fibrous scaffold for retinal differentiation of conjunctiva mesenchymal stem cells. J Biomed Mater Res A. 2017;105:189–197.
- van der Windt DJ, Bottino R, Kumar G, et al. Clinical islet xenotransplantation: how close are we? Diabetes. 2012;61:3046–3055.
- Ryan EA, Lakey JR, Rajotte RV, et al. Clinical outcomes and insulin secretion after islet transplantation with the Edmonton protocol. Diabetes. 2001;50:710–719.
- Massumi M, Hoveizi E, Baktash P, et al. Efficient programming of human eye conjunctiva-derived induced pluripotent stem (ECiPS) cells into definitive endoderm-like cells. Exp Cell Res. 2014;322:51–61.
- Chandra V, Swetha G, Muthyala S, et al. Islet-like cell aggregates generated from human adipose tissue derived stem cells ameliorate experimental diabetes in mice. PLoS One. 2011;6:e20615.
- Bai C, Gao Y, Li Q, et al. Differentiation of chicken umbilical cord mesenchymal stem cells into beta-like pancreatic islet cells. Artif Cells Nanomed Biotechnol. 2015;43:106–111.
- Habener JF, Kemp DM, Thomas MK. Minireview: transcriptional regulation in pancreatic development. Endocrinology. 2005; 146:1025–1034.
- Suarez-Pinzon WL, Lakey JR, Brand SJ, et al. Combination therapy with epidermal growth factor and gastrin induces neogenesis of human islet {beta}-cells from pancreatic duct cells and an increase in functional {beta}-cell mass. J Clin Endocrinol Metab. 2005;90:3401–3409.
- Hardikar AA, Marcus-Samuels B, Geras-Raaka E, et al. Human pancreatic precursor cells secrete FGF2 to stimulate clustering into hormone-expressing islet-like cell aggregates. Proc Natl Acad Sci USA. 2003;100:7117–7122.
- Florio P, Luisi S, Marchetti P, et al. Activin A stimulates insulin secretion in cultured human pancreatic islets. J Endocrinol Investig. 2000;23:231–234.
- Brun T, Franklin I, St-Onge L, et al. The diabetes-linked transcription factor PAX4 promotes {beta}-cell proliferation and survival in rat and human islets. J Cell Biol. 2004;167:1123–1135.
- Liu J, Liu Y, Wang H, et al. Direct differentiation of hepatic stem-like WB cells into insulin-producing cells using small molecules. Sci Rep. 2013;3:1185.
- Park MK, Han C, Lee KH, et al. Effects of activin A on pancreatic ductal cells in streptozotocin-induced diabetic rats. Transplantation. 2007;83:925–930.
- Zalzman M, Anker-Kitai L, Efrat S. Differentiation of human liver-derived, insulin-producing cells toward the beta-cell phenotype. Diabetes. 2005;54:2568–2575.
- Sun Y, Chen L, Hou XG, et al. Differentiation of bone marrow-derived mesenchymal stem cells from diabetic patients into insulin-producing cells in vitro. Chin Med J (Engl). 2007;120:771–776.
- Brewer GJ, Torricelli JR, Evege EK, et al. Optimized survival of hippocampal neurons in B27-supplemented Neurobasal, a new serum-free medium combination. J Neurosci Res. 1993;35:567–576.
- Zhang Y, Dou Z. Under a nonadherent state, bone marrow mesenchymal stem cells can be efficiently induced into functional islet-like cell clusters to normalize hyperglycemia in mice: a control study. Stem Cell Res Ther. 2014;5:66.
- Lehmann R, Zuellig RA, Kugelmeier P, et al. Superiority of small islets in human islet transplantation. Diabetes. 2007;56:594–603.
- D'Amour KA, Bang AG, Eliazer S, et al. Production of pancreatic hormone-expressing endocrine cells from human embryonic stem cells. Nat Biotechnol. 2006;24:1392–1401.
- Johnson JD, Ahmed NT, Luciani DS, et al. Increased islet apoptosis in Pdx1+/- mice. J Clin Investig. 2003;111:1147–1160.
- Zhao FQ, Keating AF. Functional properties and genomics of glucose transporters. Curr Genomics. 2007;8:113–128.
- Guillam MT, Hummler E, Schaerer E, et al. Early diabetes and abnormal postnatal pancreatic islet development in mice lacking Glut-2. Nat Genet. 1997;17:327–330.
- Tang DQ, Cao LZ, Burkhardt BR, et al. In vivo and in vitro characterization of insulin-producing cells obtained from murine bone marrow. Diabetes. 2004;53:1721–1732.
- Nadri S, Yazdani S. Isolation and expansion of mesenchymal stem cells from human conjunctival tissue. Curr Protocols Stem Cell Biol. 2015;33:1F.14.1–8.
- Sonksen P, Sonksen J. Insulin: understanding its action in health and disease. Br J Anaesth. 2000;85:69–79.
- Mooranian A, Negrulj R, Takechi R, et al. Electrokinetic potential-stabilization by bile acid-microencapsulating formulation of pancreatic beta-cells cultured in high ratio poly-L-ornithine-gel hydrogel colloidal dispersion: applications in cell-biomaterials, tissue engineering and biotechnological applications. Artif Cells Nanomed Biotechnol. 2017. DOI:https://doi.org/10.1080/21691401.2017.1362416
- Weber LM, Hayda KN, Anseth KS. Cell-matrix interactions improve beta-cell survival and insulin secretion in three-dimensional culture. Tissue Eng Part A. 2008;14:1959–1968.
- Cheng JY, Raghunath M, Whitelock J, et al. Matrix components and scaffolds for sustained islet function. Tissue Eng Part B Rev. 2011;17:235–247.
- Brissova M, Fowler MJ, Nicholson WE, et al. Assessment of human pancreatic islet architecture and composition by laser scanning confocal microscopy. J Histochem Cytochem. 2005;53:1087–1097.
- Daoud J, Petropavlovskaia M, Rosenberg L, et al. The effect of extracellular matrix components on the preservation of human islet function in vitro. Biomaterials. 2010;31:1676–1682.
- Kaur S, Ma Z, Gopal R, et al. Plasma-induced graft copolymerization of poly(methacrylic acid) on electrospun poly(vinylidene fluoride) nanofiber membrane. Langmuir ACS J Surf Colloids. 2007;23:13085–13092.
- Rupp F, Scheideler L, Olshanska N, et al. Enhancing surface free energy and hydrophilicity through chemical modification of microstructured titanium implant surfaces. J Biomed Mater Res A. 2006;76:323–334.
- Bhang SH, Lim JS, Choi CY, et al. The behavior of neural stem cells on biodegradable synthetic polymers. J Biomater Sci Polymer Ed. 2007;18:223–239.