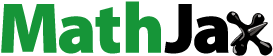
Abstract
The present study was conducted for the optimization of transethosomes formulation for dermal fisetin delivery. The optimization of the formulation was carried out using “Box–Behnken design”. The independent variables were Lipoid S 100, ethanol and sodium cholate. The prepared formulations were characterized for vesicle size, entrapment efficiency and in vitro skin penetration study. The vesicles–skin interaction, confocal laser scanning microscopy and dermatokinetic studies were performed with optimized formulation. Results of the present study demonstrated that the optimized formulation presented vesicle size of 74.21 ± 2.65 nm, zeta potential of −11.0 mV, entrapment efficiency of 68.31 ± 1.48% and flux of 4.13 ± 0.17 µg/cm2/h. The TEM image of optimized formulation exhibited sealed and spherical shape vesicles. Results of thermoanalytical techniques demonstrated that the prepared transethosomes vesicles formulation had fluidized the rigid membrane of rat’s skin for smoother penetration of fisetin transethosomes. The confocal study results presented well distribution and penetration of Rhodamine B loaded transethosomes vesicles formulation up to deeper layers of the rat’s skin as compared to the Rhodamine B-hydro alcoholic solution. Present study data revealed that the developed transethosomes vesicles formulation was found to be a potentially useful drug carrier for fisetin dermal delivery.
Introduction
The non-melanoma skin cancers comprise of the cancers affecting to the skin apart from melanoma, counting basal cell carcinoma and squamous cells carcinoma as the most commonly diagnosed and malignant one [Citation1]. Among which squamous cells carcinoma is regarded as the most malignant because of the invasive nature which can lead to metastasis and end up in death of the patient [Citation2]. Solar ultraviolet radiation (UV) is considered as the most prominent contributing factor of aforementioned cutaneous malignancies [Citation3]. Alterations in life style, dietary habits and environmental conditions also can arise as risk factors of skin malignancies [Citation4,Citation5]. As the uses of synthetic agents are associated with overriding concerns of high toxicity, expensiveness and accessibility, there is call for natural agents which can circumvent these problems [Citation6]. Lots of such phytochemicals obtained from plants have exhibited their potential in chemoprevention of cancer. The natural flavonoid fisetin has been found abundantly in various vegetables and fruits like cucumber, onion, persimmon, apple and strawberry [Citation7]. Fisetin is endowed with anti-oxidative, anti-inflammatory, anti-viral, pro-apoptotic and neuroprotective ability [Citation8–10]. Topical application of fisetin has proven to an effective mean for management of skin cancer on mice by actively suppressing inflammation and DNA damage induced by solar UV B radiation [Citation11]. Fisetin has shown its potential in induction of cell cycle arrest and apoptosis in human epidermoid carcinoma A431 cells and impeded the invasion of human melanoma cells to the dermis [Citation12,Citation13].
Utilization of topical route by using novel formulation is preferred as a better option for managements of skin cancers and other skin ailments as skin is always regarded as a potential pathway for chemicals into our body [Citation14]. Fisetin exhibits lower water solubility which will make it difficult for fisetin for deeper skin penetration and deposition. Albeit this could be overcome by formulating fisetin in novel formulations known with superior drug loading, efficient penetration ability and skin targeting upon dermal application. The phospholipids-based colloidal drug delivery systems have proven to be a promising choice for dermal delivery of actives due to the biocompatible and biodegradable nature of phospholipids. Nevertheless, the stratum corneum layer of skin was found to be hindering the passage of drugs through the skin owing to its resistive nature. The scientists have trawled through various strategies to surmount the stratum corneum barrier for effective dermal delivery of drugs. The first such approach was unveiled with the development of liposomal dermal delivery system of triamcinolone [Citation15]. But the lack of flexible nature of liposome impeded the deeper skin penetration and made it to remain confined in the upper layers of the skin [Citation16,Citation17]. The advent of deformable liposomes by the Cevc and blume solved the issues related to the rigid membrane nature of liposomes [Citation18]. The presence of edge activators in the deformable liposomes apart from phospholipid and water makes the vesicles more flexible by destabilizing the lipid bilayers [Citation19–22]. This will pave the way for deeper skin penetration of entrapped drug [Citation23,Citation24]. Following deformable liposomes, Touitou et al. developed a new elastic vesicular system named as ethosomes [Citation25]. The presence of ethanol in the ethosomes apart from phospholipid and water makes it distinct from liposome. The interaction of ethanol with the lipids of both skin and vesicles had made the vesicles more flexible due to the ethanol inherent ability to fluidize the lipid membranes [Citation26–29]. This ethanol characteristic had led to augment the passage of drugs entrapped in ethosomes through stratum corneum barriers. The transethosomes presented the advantages of both ethosomes and deformable liposome as it was composed of both ethanol and edge activators [Citation30]. Furthermore, transethosomes presented better penetration ability and deposition characteristics [Citation31]. The current study was focused on the development of transethosomes formulation of fisetin. The developed formulation was optimized by design expert software using vesicles size, entrapment efficiency and flux as dependent variables. Thereafter, the optimized formulation was analyzed for the morphology, zeta potential, skin penetration competence, skin interaction and dermatokinetic properties.
Materials and methods
Materials
Fisetin was purchased from Xi’an XIAOCAO Botanical Development Co., Ltd. (China). Lipoid S 100 was obtained from Lipoid GmbH (Ludwigshafen, Germany). Sodium cholate was purchased from Thomas Baker (Mumbai, India). Ethanol (absolute alcohol 99.9%) was purchased from Brampton, Ontario L6T 3Y4 (Canada). Chloroform, sodium hydroxide and potassium dihydrogen orthophosphate were purchased from Merck (Mumbai, India). Carbopol 934, triethanolamine and polyethylene glycol-400 were purchased from SD fine chemicals (Mumbai, India). All other chemicals and solvents used were of analytical grade. Water for high performance liquid chromatography (HPLC) was used for all experiments.
Formulation of fisetin transethosomes
For the preparation of transethosomes of fisetin, specified quantity of Lipoid S 100, sodium cholate and fisetin was dissolved in a mixture of methanol:chloroform (1:2, v/v) in round bottom flask. Then the organic solvents were removed under vacuum with reduced pressure using rotary evaporator till the formation of thin layer of lipid mixture on the inner lines of round bottom flask. The deposited thin lipid film was made free of traces of solvents by keeping it in vacuum for 4 h. The dried deposited lipid film was rehydrated with hydroethanolic solution for 1 h and kept under refrigerator to attain sufficient swelling. Then prepared dispersions were subjected to ultra-sonication using “titanium probe, Ultrasonicator (UP100H, Hielscher Ultrasonics GmbH, Berlin)” for 4 min and sonicated vesicles were extruded through polycarbonate membrane to obtain transethosomes vesicles. The transethosomes were evaluated based on their vesicles size, zeta potential, entrapment efficiency, flux and morphological characters. A three-factor, three levels Box–Behnken design was utilized and chosen independent and dependent variables were presented in .
Table 1. Independent variables with their levels and dependent variables with their constraints in Box–Behnken design for the preparation of fisetin transethosomes.
Box–Behnken design for optimization of transethosomes
The potential parameters which could influence the desirable properties of transethosomes for dermal delivery were ascertained by initial screening trials. These trials had revealed the facts that the three factors such as concentration of lipid S 100, ethanol and sodium cholate could directly influence the essential characteristics of transethosomes required for efficient dermal delivery. Based on these observations, constraints were set on each factor up to which they could influence the formulation characteristics. Thereafter, a three factor, three levels – Box–Behnken design was utilized for the optimization of transethosomes formulation using Design expert 11.0.3.0 software (Stat-Ease Inc., Mineapolis, USA). The design was composed of 15 experimental runs () with the computer generated quadratic model as follows.
where Y is the measured response associated with each factor level combination; b0 is constant; b1, b2, b3 are linear coefficients, b12, b13, b23 are interaction coefficients among three factors, b11, b22, b33 are quadratic coefficients of observed experimental values and X1, X2 and X3 are mentioned levels of independent variables. The chosen independent variables were Lipoid S 100 (X1), ethanol (X2) and sodium cholate (X3). The corresponding dependent variables were vesicle size (Y1), entrapment efficiency (Y2) and flux (Y3) [Citation32,Citation33].
Table 2. Observed response in Box–Behnken design for optimization of fisetin transethosomes formulation.
Transethosomes vesicles size, polydispersity index and zeta potential
The particle size, polydispersity index and zeta potential of the transethosomes were determined using the Malvern zetasizer (Malvern Instruments, Worcestershire, UK) at 25 ± 1 °C. For the analysis, the samples were diluted in Milli-Q water, and the determinations were performed in triplicate.
Entrapment efficiency
The ultra-centrifugation method was utilized for the estimation of entrapment efficiency of developed formulations [Citation34]. The samples were subjected to centrifugation at 25,000 RPM for 3 h at 4 °C in centrifuge machine (REMI cooling centrifuge, Mumbai). The sediment which were settled down as a layer of vesicles were disrupted with Triton X 100 (0.1%) and filtered to find out the amount of fisetin in transethosomes vesicles by HPLC. The supernatant was also collected after suitable dilution with appropriate medium and fisetin content was quantified by applying following equation.
Briefly, the quantification of drug was performed using HPLC machine connected with UV detector. The mobile phase was comprised of methanol and water (2% glacial acetic acid) in a ratio of 85:15 which pumped through Shiseido C18 column at a flow rate of 1 ml/min. UV detector was set at a wavelength of 363 nm.
In vitro skin penetration study
In vitro skin penetration study was conducted on rat skin using an automated transdermal diffusion cell sampling system (SFDC 6, LOGAN instrument, NJ) equipped with Franz diffusion cells having an effective penetration area of 1.76 cm2 [Citation35–37]. For the preparation of rat skin, hairs from skin were removed using electric clipper followed by surgical removal of subcutaneous tissues. The adhering fat on the dermis side of skin was wiped out with isopropyl alcohol. The skin was wrapped in aluminum foil after washing with phosphate buffer saline and stored in deep freezer at −20 °C till use. On the day of experiment, skin was thawed and mounted on Franz diffusion cells in such a manner that the stratum corneum side facing donor compartment and dermis side facing receiver compartment [Citation38]. The receiver vehicle (phosphate buffer saline pH 7.4 containing 0.5% of Tween 80) was maintained at 32 ± 1 °C and agitated with the help of magnetic bead at 600 rpm [Citation39]. Transethosomes formulation was applied in non-occlusive manner on the donor compartment of skin. Aliquots of 0.5 ml from receiver compartment was withdrawn through the sampling port at specific time intervals of 0, 1, 2, 4, 6, 8, 12 and 24 h followed by immediate replenishment with receptor vehicle. The samples were analyzed for drug content by HPLC.
Transethosomes morphology
Morphological analysis of prepared transethosomes was performed with transmission electron microscopy (TEM-Tecnai, G20, Philips scientific, the Netherlands). One drop of diluted sample was placed on copper grid and allowed to dry. The dried sample was then stained with 2% phosphotungstic acid. Finally, the sample on the copper grid was analyzed by transmission electron microscopy.
Transethosomes–skin interaction study
The transethosomes skin interaction was analyzed using differential scanning calorimeter (DSC) (Pyris 6 DSC, Perkin Elmar, Waltham, MA) and FTIR (Perkin, Elmer, Germany). The freshly prepared rat skin samples were mounted on two Franz diffusion cells containing vehicle (phosphate buffer saline pH 7.4 containing 0.5% of Tween 80). In one Franz cell, optimized transethosomes formulation was applied to skin sample and penetration study was carried out for 12 h. The other skin sample mounted on Franz cell was left untreated (control). Then skin samples from both the Franz cell were removed and washed with water, dried and cut into small piece and sealed in aluminum DSC pan. DSC analysis was carried out from 30 to 300 °C at a rate of 10 °C/min. The data analysis was performed by using software Pyris (Perkin-Elmer, Waltham, MA). For FTIR analysis, the skin samples were subjected to FTIR scanning in the range of 400–4000 cm−1 using FTIR instrument.
Confocal laser scanning microscopy study
Rhodamine B dye-loaded transethosomes formulation and hydro alcoholic solutions were applied non-occlusively and homogeneously on the excised rat abdominal skin mounted on two separate Franz diffusion cells and left for 8 h at 32 °C. The skin treated with formulation and hydro alcoholic solutions containing Rhodamine B were washed with distilled water to remove the excess of formulation or hydro alcoholic solution. The skin samples were cut into small sections and mounted on glass slide. The slide with upward facing stratum corneum was observed under Leica confocal laser scanning microscope (Leica TC SPE-IIw, DMI 4000 RGBV Leica Microsystems, Germany). The optical scanning of skin was done through z-axis of a confocal microscope with 5 µm increments. The Argon laser beam of 488 nm was utilized for the optical excitation and the subsequent detection of fluorescence emission was carried out above 532 nm. Leica Application suite Advance Fluorescence software was used for depth measurement [Citation40].
Dermatokinetic study
Since prepared transethosomes formulation was insufficiently viscous and could be therefore quickly removed from the rat skin. Therefore, the optimized transethosomes was converted into gel formulation. For the preparation of gel formulation, Carbopol 934 was used as gelling agent. Initially, the carbopol 934 was slowly mixed with distilled water to get the polymer dispersion and then kept aside in dark to allow to polymer to complete swell. Polyethylene glycol 400 (15% w/w) and chlorocresol (0.1%) were added slowly. The carbopol dispersion was neutralized with the incorporation of triethanolamine to get a clear viscous gel. Then the optimized transethosomes formulation was slowly added into preformed gel with stirring [Citation41,Citation42].
The dermatokinetic study was performed to analyze the concentration of drug in different layers of Wistar rat skin. For the study, fisetin transethosomes gel formulation was applied to rat skin mounted in Franz diffusion cell and investigation was carried out as discussed under in vitro skin penetration study. In contrast to penetration study, the whole rat skin was removed from the Franz cell at the respective sampling times [Citation43,Citation44]. Then the separated skin sample was then washed with normal saline to make it free of any adhering formulation and dipped in water maintained at 60 °C for 2–3 min. The skin sample was then separated into different layers such as epidermis and dermis with the aid of forceps. The separated skin layers were chopped into small pieces and soaked in methanol (5 ml) for 24 h for easy extraction of fisetin. The methanolic extract of fisetin was further passed through membrane filter and the fisetin content was estimated by HPLC. Fisetin concentration per cm square of skin versus time was plotted for epidermis and dermis separately. The PK solver software was utilized to evaluate the different dermatokinetic parameters such as Tskin max, Cskin max, AUC0–8 h and Ke.
Result and discussion
Optimization of transethosomes by Box–Behnken design
The Box–Behnken design had generated 15 experimental runs for formulations development with three center points. The responses obtained from these runs were shown in . The values of three dependent variables namely vesicle size (Y1), entrapment efficiency (Y2) and flux (Y3) were fall in the range of 41.35 to 184.18 nm, 39.23 to 80.14% and 2.34 to 5.46 µg/cm2/h, respectively. The quadratic model was found to be the most fitted model for the responses of all the 15 formulations. The values of R2, SD and %CV of each of the three responses are shown in . The effect of independent variables on vesicles size, entrapment efficiency and flux is presented by the three-dimensional graph (), and quantitatively compared the resultant experimental values of the responses with that of the predicted values.
Figure 1. 3D-response surface plot showing effect of independent variables on (A) vesicles size (B) entrapment efficiency and (C) flux.
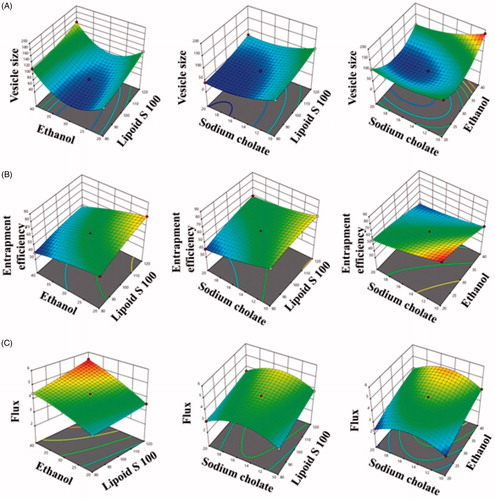
Figure 2. Linear correlation plots (A, C, E) between actual and predicted values and the corresponding residual plots (B, D, F) for various responses.
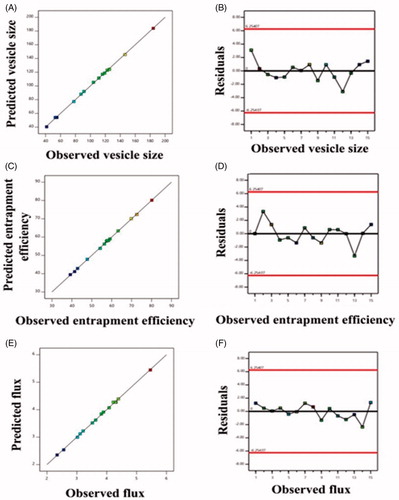
Table 3. Summary of results of regression analysis for responses Y1, Y2 and Y3 for fitting to quadratic model.
Response 1 (Y1): effect of independent variables on vesicles size
The average vesicle size of all 15 experimental runs was found to be 99.30 with values lying between the minimum and maximum of 41.35 nm and 184.14 nm, respectively.
(1)
(1)
As per the polynomial EquationEquation (1)(1)
(1) , the Lipoid S 100 was found to be showing a positive effect and sodium cholate was exhibiting a negative effect on vesicle size.
It was observed that the vesicles size of transethosomes was increased with increasing in the concentration of Lipoid S 100. It was observed that vesicles size was increased from 87.42 ± 0.84 nm (formulation 11) to 122.53 ± 1.05 nm (formulation 3) when Lipoid S 100 was increased from 80 mg (formulation 11) to 120 mg (formulation 3). Similar results were seen with formulation 6 (vesicles size 119.14 ± 4.27 nm) and formulation 8 (vesicles size 146.38 ± 3.92 nm) and . Results of the present study were in agreement with the findings of other researchers [Citation25,Citation45].
The effect of sodium cholate is clearly seen in . It was observed that sodium cholate presented inverse effect on vesicles size. Vesicles size of prepared transethosomes was decrease on increasing the sodium cholate from 10 mg to 20 mg. Formulation 5 having sodium cholate 10 mg presented vesicles size of 91.35 ± 1.61 nm while formulation 15 presented vesicles size of 41.35 ± 0.31 nm which comprised of 20 mg of sodium cholate. Similarly, formulation 9 (sodium cholate 10 mg) presented vesicles size of 116.21 ± 1.57 nm while formulation 10 (sodium cholate 20 mg) exhibited vesicles size of 77.85 ± 1.28 nm. Similar results were obtained for formulation 1 with formulation 13, and formulation 2 with formulation 12 .
Response 2 (Y2): effect of independent variables on entrapment efficiency
The entrapment efficiency of all the formulation was lying in between a minimum and maximum value of 39.23 ± 0.68% and 80.14 ± 0.53% with the average value of 57.24% ().
(2)
(2)
It was observed from EquationEquation (2)(2)
(2) that the concentration of Lipoid S 100 was found to be showing a positive effect on entrapment efficiency while the other two variables ethanol and sodium cholate was found to exhibiting a negative effect on entrapment efficiency of the drug. The gradual increase of entrapment efficiency with increase in the concentration of Lipoid S 100 might be due to the inherent lipophilic character of fisetin as the lipophilic drug would be attracted towards the lipophilic phase and get deposited over there.
The formulation 3 containing 120 mg of Lipoid S 100 had exhibited entrapment efficiency of 72.62 ± 2.72% on comparison with the formulation 11 possessing 80 mg of Lipoid S 100 showing an entrapment efficiency of 56.34 ± 1.21%. Similar results were observed between formulation 9 (entrapment efficiency 69.81 ± 1.43%) and formulation 5 (entrapment efficiency 63.25 ± 1.26%). Similar results were obtained for formulation 10 and 15 ().
Ethanol presented inverse effect on the entrapment efficiency of fisetin in transethosomes. Formulation 11 (ethanol 20%) presented entrapment efficiency of 56.34 ± 1.21% while the formulation 6 having ethanol 40% presented entrapment efficiency of 39.23 ± 0.68%. Similar results were obtained for formulation 1 and 2. Formulation 1 (ethanol 20%) presented entrapment efficiency of 80.14 ± 0.53% and formulation 2 (ethanol 40%) presented entrapment efficacy of 54.21 ± 1.14%. Similar results were obtained between formulation 13 (entrapment efficiency 57.41 ± 0.75%) and formulation 12 (entrapment efficiency 42.87 ± 0.64) (). This could be due that at higher ethanol percentage; vesicles become leakier [Citation28].
Entrapment efficiency also decreased on increasing the concentration of sodium cholate (). Formulation 5 (sodium cholate 10 mg) presented entrapment efficiency of 63.25 ± 1.26% while formulation 15 (sodium cholate 20 mg) presented entrapment efficiency of 41.39 ± 0.79%. Similar results were obtained between formulation 1 (entrapment efficiency 80.14 ± 0.53%) and formulation 13 (entrapment efficiency 57.41 ± 0.75%). Alike data were obtained between formulation 2 (entrapment efficiency 54.21 ± 1.14%) and formulation 12 (entrapment efficiency 42.87 ± 0.64%). The decrease in entrapment efficiency with increasing in the concentration of sodium cholate is could be due to the coexistence of micelle structure with vesicles in the formulation; micelles usually exhibit low entrapment efficiency as compared to vesicles [Citation46].
Response 3 (Y3): effect of independent variables on flux
The average flux value of all the observed 15 formulations was found to be 3.72 µg/cm2/h with the minimum and maximum values as 2.34 ± 0.08 µg/cm2/h and 5.46 ± 0.23 µg/cm2/h, respectively ().
(3)
(3)
The concentration of ethanol and phospholipid had shown positive effect towards flux (EquationEquation (3))(3)
(3) . It was observed that formulation 3 (Lipoid S 100, 120 mg) exhibited higher flux of 3.52 ± 0.17 µg/cm2/h as compared to the formulation 11 (Lipoid S 100, 80 mg) which presented flux of 3.21 ± 0.11 µg/cm2/h. Similar results were observed for formulation 9 (Lipoid S 100 = 120 mg) as compared to the formulation 5 (Lipoid S 100 = 80 mg). Similar results were also observed between formulation 10 and formulation 15 (). The increase in flux on increasing the phospholipid content of vesicle could be due to the property of phospholipid to augment the partition of vesicles via the skin lipid barrier.
Similarly, ethanol also had shown positive effect on the fisetin flux across the rat’s skin. It was observed the formulation 6 (ethanol 40%) presented flux of 4.39 ± 0.07 µg/cm2/h as compared to the formulation 11 (ethanol 20%). Likewise, formulation 8 (ethanol 40%) shown flux of 5.46 ± 0.23 µg/cm2/h as compared to formulation 3 which contained ethanol 20% (). The increase in flux on increasing in ethanol could be due to the well-known penetration enhancer characteristic of ethanol. Being a penetration enhancer, ethanol reduced the transition temperature of the lipid and imparts flexibility in the vesicles and allows the vesicles in the penetration across the skin [Citation25]. Furthermore, ethanol might have interacted with polar head groups of the lipid molecules of intercellular lipid multilayers present in stratum corneum and induce fluidization of the stratum corneum and could improve the penetration of flexible vesicles through it [Citation47].
We have observed a gradual increase in flux with the increase in sodium cholate concentration from 10 to 15 mg (). This was followed by a steady decrease in flux for formulation containing 15 to 20 mg of sodium cholate (). The increase in fisetin flux with increase in the concentration of sodium cholate could be due to the capability of sodium cholate to impart flexible to the vesicles by altering the packing characteristics of the bilayer. This allows the vesicles to squeeze through the skin pores even smaller than the vesicles diameter. At higher sodium cholate concentration (from 15 mg to 20 mg) the flux decreased. This could be due to the formation of micelle structures at higher concentration of sodium cholate. Hence it is advisable to use optimum concentration of sodium cholate for the formulation of transethosomes vesicles.
Further in this study, the point prediction method of Box–Behnken design was utilized for the optimization of transethosomes formulation. The formulation composition with Lipoid S 100 (115.12 mg), ethanol (27.32%) and sodium cholate (13.75 mg) was found to fulfill requisites of an optimum formulation.
The optimized formulation had shown the vesicles size of 74.21 ± 2.65 nm (), entrapment efficiency of 68.31 ± 1.48% and flux of 4.13 ± 0.17 µg/cm2/h. These values are found in proximity to the predicted values for vesicle size (76.43 nm), entrapment efficiency (66.42%) and flux (4.26 µg/cm2/h) generated by the Design Expert software. Furthermore, the polydispersity index and zeta potential () value of optimized formulation was found to be 0.130 ± 0.001 and −11.0 mV, respectively. The optimized formulation so produced was further evaluation for vesicles morphology, vesicles-skin interaction study, confocal laser scanning microscopy and dermatokinetic study.
Transethosomes morphology
The TEM analysis image of optimized transethosomes formulation revealed that the fisetin loaded vesicles are well-identified sealed structure with uniform size distribution and spherical in shapes () [Citation48].
Transethosomes − skin interaction
In this study, rat skin treated with the transethosomes formulation was analyzed based on the endotherms of Tm and their corresponding enthalpy ΔH in DSC thermogram and compared with the DSC thermogram of untreated rat skin. The untreated rat skin presented Tm and ΔH values of 102.12 °C and 5017.507 J/g, respectively (). The transethosomes treated skin sample had shown a substantial decline in the values of Tm and ΔH. The Tm value of rat skin treated with formulation was reduced to 80.38 °C, while the ΔH value had drop sharply to 637.46 J/g in comparison to the untreated control skin (). It was reported that the disruption of skin lipid bilayer could be detected by the shift in Tm to a lower value and the fluidization of lipid bilayer is denoted by a decline in ΔH value.
Figure 4. DSC thermogram of rats skin (A) untreated and (B) treated with fisetin transethosomes optimized formulation.
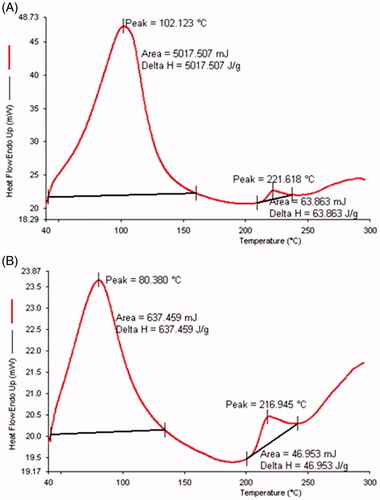
The molecular organization of lipid matrixes of stratum corneum could be evaluated with the help of FTIR by the presence of bands at various wave numbers. The appearance of bands at specific wave numbers indicating the vibration of lipids and proteins molecules of stratum corneum. The lipid hydrocarbon peaks are distinguished from other by their symmetric and asymmetric stretching vibrations of CH2 bonds with values as 2929 cm−1 and 2851 cm−1, respectively. The stratum corneum proteins were showing bands for the amide I (C=O stretching) and amide II (C–N stretching) bonds stretching vibrations at a wave number of 1647 cm−1 and 1550 cm−1, respectively. The treatment of rat skin with fisetin optimized transethosomes formulation led to a reduction on the peak intensity of asymmetric and symmetric stretching vibrations of CH2 in comparison to untreated skin (. This implies the extraction of stratum corneum lipids on treatment of transethosomes formulation. The significant reduction of peaks intensity at 1647 cm−1 and 1550 cm−1 of treated skin could be due to the interaction of applied formulation with skin proteins. The blue shift of CH2 stretching bands in treated skin also indicated the distortion lipid bilayer structure of rat skin [Citation49,Citation50].
Confocal laser scanning microscopy
The results of confocal laser scanning microscopy exhibited that the hydro alcoholic solution of Rhodamine B remained confined in the upper layers of skin and show penetration of dye fluorescence up to the depth of 30 µm only (). On the other hand, Rhodamine B-loaded transethosomes well absorbed in the skin with deeper penetration of probe up to 70 µm (). The higher fluorescence intensity in the middle region of the skin indicates that the formulation was retained in the lower epidermal region in a higher amount after crossing the upper epidermis. This is desirable for many skin diseases including basal cell carcinoma and squamous cell carcinoma as they are situated in this lower epidermal region of skin [Citation50]. Hence it was concluded that the prepared transethosomes were effectively delivered Rhodamine B into the deeper layers of the rat’s skin.
Dermatokinetic
The relative concentration of fisetin in rat’s skin dermis and epidermis at different time intervals is depicted in . The observed values were analyzed statistically by means of one factor ANOVA and are shown in . The skin treated with transethosomes gel had shown significantly higher CSkin max and AUC0–8 in the epidermis and dermis as compared to the conventional gel (). The high retention of transethosomes gel could be due to the potency of vesicles to augment the partitioning through the skin lipid bilayers. The TSkin max of transethosomes gel in epidermis was found to be comparable to the conventional gel. While the TSkin max obtained in dermis treated with transethosomes gel is found earlier than that of conventional gel.
Table 4. Dermatokinetic parameters of fisetin conventional and transethosomes gel.
Conclusion
Fisetin-loaded transethosomes formulations were optimized using Box–Behnken design. Optimized formulation presented vesicle size in nano range and good entrapment efficiency with reasonable flux for fisetin dermal delivery. Confocal study had shown better penetration of Rhodamine B loaded fisetin transethosomes across rat’s skin than control solution. Further dermatokinetic study revealed better penetration of fisetin transethosomes gel than fisetin conventional gel. Present results demonstrate that the developed transethosomes formulation is a potentially useful drug carrier for dermal delivery of fisetin.
Disclosure statement
No potential conflict of interest was reported by the authors.
References
- Afaq F. Natural agents: cellular and molecular mechanisms of photoprotection. Arch Biochem Biophys. 2011;508:144–151.
- Cohen JL. Actinic keratosis treatment as a key component of preventive strategies for nonmelanoma skin cancer. J Clin Aesthet Dermatol. 2010;3:39–44.
- Bowden GT. Prevention of non-melanoma skin cancer by targeting ultraviolet-B-light signalling. Nat Rev Cancer. 2004;4:23–35.
- Kurundkar D, Srivastava RK, Chaudhary SC, et al. Vorinostat, an HDAC inhibitor attenuates epidermoid squamous cell carcinoma growth by dampening mTOR signaling pathway in a human xenograft murine model. Toxicol Appl Pharmacol. 2013;266:233–244.
- Tang X, Zhu Y, Han L, et al. CP-31398 restores mutant p53 tumor suppressor function and inhibits UVB-induced skin carcinogenesis in mice. J Clin Investig. 2007;117:3753–3764.
- Syed DN, Suh Y, Afaq F, et al. Dietary agents for chemoprevention of prostate cancer. Cancer Lett. 2008;265:167–176.
- Arai Y, Watanabe S, Kimira M, et al. Dietary intakes of flavonols, flavones and isoflavones by Japanese women and the inverse correlation between quercetin intake and plasma LDL cholesterol concentration. J Nutr. 2000;130:2243–2250.
- Syed DN, Afaq F, Maddodi N, et al. Inhibition of human melanoma cell growth by the dietary flavonoid fisetin is associated with disruption of Wnt/beta-catenin signaling and decreased Mitf levels. J Investig Dermatol. 2011;131:1291–1299.
- Khan N, Afaq F, Khusro FH, et al. Dual inhibition of phosphatidylinositol 3-kinase/Akt and mammalian target of rapamycin signaling in human nonsmall cell lung cancer cells by a dietary flavonoid fisetin. Int J Cancer. 2012;130:1695–1705.
- Hou DX, Fukuda M, Johnson JA, et al. Fisetin induces transcription of NADPH:quinone oxidoreductase gene through an antioxidant responsive element-involved activation. Int J Oncol. 2001;18:1175–1179.
- Pal HC, Athar M, Elmets CA, et al. Fisetin inhibits UVB-induced cutaneous inflammation and activation of PI3K/AKT/NFκB signaling pathways in SKH-1 hairless mice. Photochem Photobiol. 2015;91:225–234.
- Pal HC, Sharma S, Elmets CA, et al. Fisetin inhibits growth, induces G(2)/M arrest and apoptosis of human epidermoid carcinoma A431 cells: role of mitochondrial membrane potential disruption and consequent caspases activation. Exp Dermatol. 2013;22:470–475.
- Pal HC, Sharma S, Strickland LR, et al. Fisetin inhibits human melanoma cell invasion through promotion of mesenchymal to epithelial transition and by targeting MAPK and NFkappaB signaling pathways. PLoS One. 2014;9:e86338.
- Sabitha M, Sanoj Rejinold N, Nair A, et al. Development and evaluation of 5-fluorouracil loaded chitin nanogels for treatment of skin cancer. Carbohydr Polym. 2013;91:48–57.
- Mezei M, Gulasekharam V. Liposomes – a selective drug delivery system for the topical route of administration. Lotion dosage form. Life Sci. 1980;26:1473–1477.
- Verma DD, Verma S, Blume G, et al. Particle size of liposomes influences dermal delivery of substances into skin. Int J Pharm. 2003;258:141–151.
- Manosroi A, Jantrawut P, Manosroi J. Anti-inflammatory activity of gel containing novel elastic niosomes entrapped with diclofenac diethylammonium. Int J Pharm. 2008;360:156–163.
- Cevc G, Blume G. Lipid vesicles penetrate into intact skin owing to the transdermal osmotic gradients and hydration force. Biochim Biophys Acta. 1992;1104:226–232.
- Ahad A, Raish M, Ahmad A, et al. Development and biological evaluation of vesicles containing bile salt of telmisartan for the treatment of diabetic nephropathy. Artif Cells Nanomed Biotechnol. 2018. Forthcoming. doi:10.1080/21691401.2018.1430700
- Ahad A, Al-Saleh AA, Al-Mohizea AM, et al. Formulation and characterization of novel soft nanovesicles for enhanced transdermal delivery of eprosartan mesylate. Saudi Pharm J. 2017;25:1040–1046.
- Ahad A, Al-Saleh AA, Al-Mohizea AM, et al. Formulation and characterization of Phospholipon 90 G and tween 80 based transfersomes for transdermal delivery of eprosartan mesylate. Pharm Dev Technol. 2017. Forthcoming. doi:10.1080/10837450.2017.1330345
- Ahad A, Aqil M, Kohli K, et al. Formulation and optimization of nanotransfersomes using experimental design technique for accentuated transdermal delivery of valsartan. Nanomedicine. 2012;8:237–249.
- Cevc G. Transfersomes, liposomes and other lipid suspensions on the skin: permeation enhancement, vesicle penetration, and transdermal drug delivery. Crit Rev Ther Drug Carrier Syst. 1996;13:257–388.
- El Maghraby GM, Williams AC, Barry BW. Interactions of surfactants (edge activators) and skin penetration enhancers with liposomes. Int J Pharm. 2004;276:143–161.
- Touitou E, Dayan N, Bergelson L, et al. Ethosomes – novel vesicular carriers for enhanced delivery: characterization and skin penetration properties. J Control Release. 2000;65:403–418.
- Godin B, Touitou E. Erythromycin ethosomal systems: physicochemical characterization and enhanced antibacterial activity. Curr Drug Deliv. 2005;2:269–275.
- Ahad A, Raish M, Al-Mohizea AM, et al. Enhanced anti-inflammatory activity of carbopol loaded meloxicam nanoethosomes gel. Int J Biol Macromol. 2014;67:99–104.
- Ahad A, Aqil M, Kohli K, et al. Enhanced transdermal delivery of an anti-hypertensive agent via nanoethosomes: statistical optimization, characterization and pharmacokinetic assessment. Int J Pharm. 2013;443:26–38.
- Ahad A, Aqil M, Kohli K, et al. Nano vesicular lipid carriers of angiotensin II receptor blocker: anti-hypertensive and skin toxicity study in focus. Artif Cells Nanomed Biotechnol. 2016;44:1002–1007.
- Song CK, Balakrishnan P, Shim CK, et al. A novel vesicular carrier, transethosome, for enhanced skin delivery of voriconazole: characterization and in vitro/in vivo evaluation. Colloids Surf B Biointerfaces. 2012;92:299–304.
- Abdulbaqi IM, Darwis Y, Khan NA, et al. Ethosomal nanocarriers: the impact of constituents and formulation techniques on ethosomal properties, in vivo studies, and clinical trials. Int J Nanomedicine. 2016;11:2279–2304.
- Javed MN, Kohli K, Amin S. Risk assessment integrated QbD approach for development of optimized bicontinuous mucoadhesive limicubes for oral delivery of rosuvastatin. AAPS PharmSciTech. 2018;19:1377–1391.
- Alam MS, Garg A, Pottoo FH, et al. Gum ghatti mediated, one pot green synthesis of optimized gold nanoparticles: investigation of process-variables impact using Box-Behnken based statistical design. Int J Biol Macromol. 2017;104:758–767.
- Imam SS, Ahad A, Aqil M, et al. Formulation by design based risperidone nano soft lipid vesicle as a new strategy for enhanced transdermal drug delivery: in-vitro characterization, and in-vivo appraisal. Mater Sci Eng C Mater Biol Appl. 2017;75:1198–1205.
- Ahad A, Aqil M, Ali A. The application of anethole, menthone, and eugenol in transdermal penetration of valsartan: enhancement and mechanistic investigation. Pharm Biol. 2016;54:1042–1051.
- Ahad A, Aqil M, Kohli K, et al. Interactions between novel terpenes and main components of rat and human skin: mechanistic view for transdermal delivery of propranolol hydrochloride. Curr Drug Deliv. 2011;8:213–224.
- Ahad A, Aqil M, Kohli K, et al. Design, formulation and optimization of valsartan transdermal gel containing iso-eucalyptol as novel permeation enhancer: preclinical assessment of pharmacokinetics in Wistar albino rats. Expert Opin Drug Deliv. 2014;11:1149–1162.
- Ahad A, Aqil M, Kohli K, et al. Role of novel terpenes in transcutaneous permeation of valsartan: effectiveness and mechanism of action. Drug Dev Ind Pharm. 2011;37:583–596.
- Chen Y, Wu Q, Song L, et al. Polymeric micelles encapsulating fisetin improve the therapeutic effect in colon cancer. ACS Appl Mater Interfaces. 2015;7:534–542.
- Kamran M, Ahad A, Aqil M, et al. Design, formulation and optimization of novel soft nano-carriers for transdermal olmesartan medoxomil delivery: in vitro characterization and in vivo pharmacokinetic assessment. Int J Pharm. 2016;505:147–158.
- Ahad A, Al-Saleh AA, Al-Mohizea AM, et al. Pharmacodynamic study of eprosartan mesylate-loaded transfersomes Carbopol((R)) gel under Dermaroller((R)) on rats with methyl prednisolone acetate-induced hypertension. Biomed Pharmacother. 2017;89:177–184.
- Ahad A, Aqil M, Ali A. Investigation of antihypertensive activity of carbopol valsartan transdermal gel containing 1,8-cineole. Int J Biol Macromol. 2014;64:144–149.
- Iqbal B, Ali J, Baboota S. Silymarin loaded nanostructured lipid carrier: from design and dermatokinetic study to mechanistic analysis of epidermal drug deposition enhancement. J Mol Liq. 2018;255:513–529.
- Negi P, Singh B, Sharma G, et al. Biocompatible lidocaine and prilocaine loaded-nanoemulsion system for enhanced percutaneous absorption: QbD-based optimisation, dermatokinetics and in vivo evaluation. J Microencapsul. 2015;32:419–431.
- Dubey V, Mishra D, Dutta T, et al. Dermal and transdermal delivery of an anti-psoriatic agent via ethanolic liposomes. J Control Release. 2007;123:148–154.
- El Zaafarany GM, Awad GA, Holayel SM, et al. Role of edge activators and surface charge in developing ultradeformable vesicles with enhanced skin delivery. Int J Pharm. 2010;397:164–172.
- Kirjavainen M, Monkkonen J, Saukkosaari M, et al. Phospholipids affect stratum corneum lipid bilayer fluidity and drug partitioning into the bilayers. J Control Release. 1999;58:207–214.
- Elsayed MM, Abdallah OY, Naggar VF, et al. Deformable liposomes and ethosomes: mechanism of enhanced skin delivery. Int J Pharm. 2006;322:60–66.
- Vaddi HK, Ho PC, Chan YW, et al. Terpenes in ethanol: haloperidol permeation and partition through human skin and stratum corneum changes. J Control Release. 2002;81:121–133.
- McGillis ST, Fein H. Topical treatment strategies for non-melanoma skin cancer and precursor lesions. Semin Cutan Med Surg. 2004;23:174–183.