Abstract
In recent years, artemisinin (ART) and its derivatives have highlighted according to their effects on highly aggressive cancers, as well as treatment of malaria and leishmaniasis, besides presenting anti-inflammatory and antibacterial activity. It has also been shown that ART compounds have the ability to modulate the immune response by regulating cell proliferation and cytokine release. These effects may be beneficial and improve the treatment of cancer and parasitic diseases by increasing therapeutic success, but it has some pharmacological limitations such as low bioavailability, short half-life and limited tissue access. Nanotechnology has been explored during the last decades, notably in the design of drug carrier systems which includes polymeric, lipid and inorganic nanoparticles, cyclodextrins inclusion complexes, liposomes, carbon nanotubes, among others. These nanostructured drug delivery systems bring benefits both increased therapeutic efficacy and reduced toxicity. This review article aims to give an overview of the current progress in nanostructured drug carriers used for encapsulation of ART and its derivatives yielding examples of successful outcomes. The data collection suggests future applications of ART and derivatives encapsulated in nano delivery systems in clinical trials and prospects for use of ART loaded nanosystems in immunomodulatory responses.
Graphical Abstract

Introduction
At present, various immunosuppressive drugs are routinely used to treat related diseases. A large number of plants contain substances with potential to be used in new drug preparations [Citation1], among which we can highlight Artemisia annua L., belonging to the family Asteraceae. A. annua contains an endoperoxide sesquiterpene lactone called artemisinin (ART), with the relevance to humanity by the awarding of the Nobel Prize for 2015 to Youyou Tu [Citation2]. ART derivatives as dihydroartemisinin (DHA), artesunate (AS) and artemether (ARTE) also presented biological effects.
These substances have been found to have a range of properties, including anti-ulcerous [Citation3], antinociceptive [Citation4], antifungal [Citation5], antibacterial [Citation6], anti-HIV [Citation7] and anticancer [Citation8]. Li and coworkers showed that ART enhances the signalling of GABA and prevents the secretion of glucagon, resulting in glucose homeostasis [Citation9]. Despite its efficacy, its use is still limited due to low solubility in water, resulting in weak and erratic absorption after oral administration. Additionally, ART has a short half-life and high first-passage metabolism [Citation10].
The association of ART and its derivatives and nanocarriers has been shown to be very advantageous in the several applications of these drugs. Accordingly, some physicochemical and pharmacokinetic problems can be solved by improving the efficacy of these drugs in the treatment of infectious diseases and cancer [Citation11]. Here, we highlight recent developments of nanocarriers containing ART and its derivatives, strategies for improved in vitro and in vivo efficacy and their potential to treat disorders, including cancer and parasitic diseases. Also, we provided a prospection of future use of nanocarriers containing ART family drugs improving immunomodulatory effects of these compounds beyond antimalarial activity.
Immunomodulatory effects of ART compounds
ART drugs accomplish immunosuppressive activities mainly through the inhibition of pathogenic T-cell activation, suppression of B cells, reduction in IgG and IgE immunoglobulins and inhibition of MAPKs signalling pathway (phosphorylation of ERK1/2, Jnk and P38) [Citation12]. Additionally, another mechanism reported is expanding of regulating T cells by increasing immune tolerance and activation of cytotoxic T cells (). Likewise, it was reported in vitro inhibition of IL-1β, IL-6 and IL-8, stimulated with TNF-α in a rheumatoid arthritis model mitigating the progress of severe inflammatory conditions [Citation9].
Figure 1. Schematic representation of immunomodulatory suppressive effects of artemisinin in B and T cells. (a) The expansion of regulating T cells, increasing immune tolerance and activation of cytotoxic T cells and (b) Inhibition of Th1 and Th17 responses, decrease the release of matrix metalloproteinases (MMPs) and TNF-α; Inhibition of IgG production by B cells. Adapted from Shakir and co-workers [Citation18].
![Figure 1. Schematic representation of immunomodulatory suppressive effects of artemisinin in B and T cells. (a) The expansion of regulating T cells, increasing immune tolerance and activation of cytotoxic T cells and (b) Inhibition of Th1 and Th17 responses, decrease the release of matrix metalloproteinases (MMPs) and TNF-α; Inhibition of IgG production by B cells. Adapted from Shakir and co-workers [Citation18].](/cms/asset/dce14f2d-9b77-420e-9f2d-cc1cc4998053/ianb_a_1505739_f0001_c.jpg)
The immunomodulatory effect of ART compounds in autoimmune diseases (as systemic lupus erythematosus and multiple sclerosis) is connected to the inhibition of Th1 and Th17 responses decreasing the release of matrix metalloproteinases and TNF-α by macrophages, following the inhibition of generation of IgG immunoglobulins by B cells. Either, increases in IL-4 and IL-10 levels were detected [Citation13]. Humoral immune responses, such as allergic diseases and contact hypersensitivity, the T cells can mediate inflammatory reaction. ART compounds were capable to modulate T cell responses (Th1, Th2, Th17 and Treg) (). Equally, these drugs might inhibit the production of IgE immunoglobulin by the B cells via downregulation of PI3K/AKT pathway or block IgE-induced mast cell degranulation [Citation14].
In the immune response, the use of ART compounds ensures more selective treatment through activated pathogenic T cells inhibition solely, preventing reaching other cells in the body. However, ART has many advantages modulating multiple pathways of the immune system also being candidates to treat inflammation and autoimmune diseases.
Harnessing nanotechnology for drug delivery systems
Nanotechnology involves the study of particulate matter, natural or synthetic origin, with number size distribution and one of its dimensions between 1 and 100 nm, displaying unique properties that allow new applications, namely developing nanostructured drug delivery systems (NDDS). However, this concept can be expanded to particles <1000 nm, which reach a biological system and exhibit different properties when compared to the same material on a non-nanometric scale [Citation15]. The key points are to improve the solubility of drugs and their bioavailability as well as to protect against degradation, increasing the half-life and its therapeutic efficacy [Citation16].
According to the composition, NDDS are classified into polymeric, lipid and inorganic carriers and may assume structures such as matrix, vesicular, self-assembled or emulsions (). In the literature, several studies have evaluated the use of different types of NDDS with ART or its derivatives in cancer, malaria and visceral leishmaniasis models. Generally, these studies reported an improvement in pharmacological activity, but only a few studies have done a more detailed study of the immune response pattern promoted by the drug, is possible through the enhancement of the therapeutic value promoted by the NDDS.
Figure 2. Different types of NDDS used to encapsulate artemisinin and its derivatives. (a) magnetic nanoparticles; (b) fullerenes (C60); (c) nanostructured lipid carriers; (d) solid lipid nanoparticles; (e) carbon nanotubes; (f) polymeric micelles; (g) nanocapsules; (h) nanospheres and (i) liposomes.
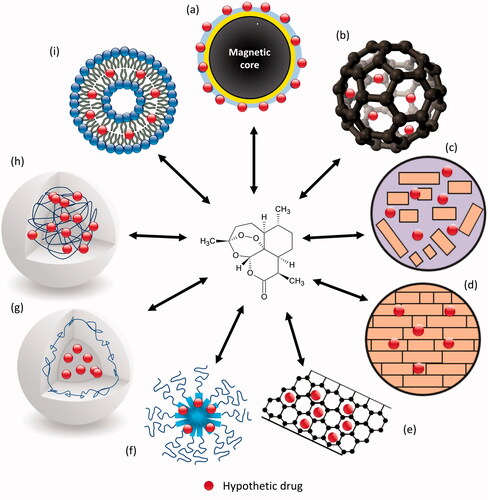
Polymeric carriers
Polymer-based nanocarriers are produced with biodegradable and biocompatible polymers, which can have the natural or synthetic origin. The most common polymers used are aliphatic polyesters (poly(lactic-co-glycolic, PLGA), poly(ε-caprolactone, PCL)), chitosan and polyethyleneglycol (PEG) [Citation17]. Depending on the nanocarrier, the interaction between polymer and drug is through matrix entrapment or adsorption.
Micelles
Polymeric micelles (PMs) are colloidal self-assembled spherical nanocarriers involving non-covalent interactions, composed of amphiphilic block polymers (generally PEG or polyethylene oxide and acyl chains) in aqueous solution. These block polymers assemble a hydrophobic core carrying poorly water-solubility drugs, surrounded by a hydrophilic shell with a typical size range of 20–100 nm [Citation18].
PMs usually presented long blood circulation because of the stealth ability provided by the hydrophilic corona, passive targeting by EPR effect and active targeting through surface functionalization with antibodies and peptides presenting wide application in cancer therapy [Citation11].
Arteether (ARE), a potent anticancer drug, was encapsulated in pH-sensitive polyurethane nanomicelles (PU NMs). ARE loaded PU NMs inhibited 4T1 cell line, reduced tumour growth in breast cancer model and increased INF-γ levels, indicating there was a stimulus of Th1 immune response by nanosystem containing ARE [Citation19]. Manjili and coworkers prepared nanomicelles of PCL-PEG-PCL triblock copolymers containing ART. The pharmacokinetic study in rats showed improved bioavailability of ART by intravenous administration of the PMs formulation compared to the free drug as well as toxicity specific for 4T1 and MCF7 tumour cell lines [Citation20]. Another study with the same nanocarrier, its antiplasmodial effect was evaluated in animal malaria model. PCL-PEG-PCL micelles containing ART provided an appropriate drug delivery to Plasmodium berghei infected erythrocytes, reducing parasitemia. These results make PMs containing ART a candidate for malaria treatment [Citation21].
The incorporation of ART compounds in PMs has been shown promising in both in vitro and in vivo evaluations (). However, PMs’ performance is limited by phenomena as burst effect and rate of biodegradation and drug.
Table 1. Summary of the main works with polymeric nanocarriers and artemisinin and its derivatives.
Polymeric nanoparticles
Polymeric nanoparticles (PNs) are submicron colloidal structures and depending on their composition, they can have two distinct arrangements: nanospheres (NSs) or nanocapsules (NCs). NSs are matrix-type structures with molecularly dispersed drugs, whereas NCs have a vesicular shape where the drug is confined in the inner compartment, enclosed by a polymeric membrane. PNs also increase the therapeutic value of drugs and reduce their systemic toxicity [Citation17].
Concerning efficacy and bioavailability, PNs show a remarkable improvement in intravenous and oral routes. In addition, they deliver pharmaceutical compounds to a target tissue at a specified concentration and conferring stability to volatile active ingredients [Citation22]. Also, PNs are able to offer multiple drug delivery, long circulation (stealth PNs), better stability, narrow size distribution, high drug loading and control of drug release by diffusion through polymer or erosion of the matrix [Citation23].
Presently, there are several studies involving ART compounds and polymeric nanoparticles (), both in vitro and in vivo. Tran and coworkers employed chitosan-coated lipid nanocapsules with AS aiming drug sustained release profile and enhanced cellular uptake increasing cytotoxicity. The results showed evident antitumor activity against breast cancer lines (MCF-7, MDA-MB-231), compared with free AS [Citation24]. Chitosan magnetic nanoparticles containing ART were assayed in breast cancer mice model. The magnetic property could direct the NP to the target tissue through the application of the external magnetic field, where high doses of ART were released, reducing tumour growth [Citation25].
Likewise, PLGA nanoparticles have been extensively studied as an NDDS system for ART drugs. Want and coworkers performed two studies in visceral leishmaniasis model. At the first study, ART entrapped PLGA NPs demonstrated reduction of drug toxicity in murine macrophages and infected macrophages as well as amastigotes mediated by NO production [Citation26]. In the second study, the same ART-PLGA NPs systems were tested in mice infected. It was observed suitable immunomodulation of the drug with high delayed-type hypersensitivity (DTH) response, increase of INF-γ and IL-2 and reduction of IL-4 and IL-10 indicating Th1 immune response pattern resulting in clearance of the parasite [Citation27]. Other studies conducted with PLGA NPs and other ART derivatives revealed high cytotoxicity in SCC-7, MCF-7 and HepG2 cell lines with growth inhibition and induced apoptosis, compared with free drugs [Citation28,Citation29].
Similarly, protein-polymeric nanoparticles loading ART derivatives have also been addressed in these same pharmacological performance models, as previously mentioned. Ibrahim and coworkers employed the in vivo malaria model to evaluate the performance of albumin NPs containing ART drug. NPs were administrated intravenously and revealed 96% of parasitemia inhibition, prolonging the mean survival time of infected mice [Citation30]. Albumin NPs were also applied for AS encapsulation. The comparison with free AS, the nanosystems presented cytotoxicity and significant apoptotic effects indicating activated mitochondrial-mediated cell apoptosis [Citation31]. Herein, PNs indicate their potential to encapsulate ART and its derivatives and the use in cancer and protozoal diseases with high therapeutic efficacy ().
Lipid carriers
The versatile lipid carriers have attracted attention as drug vehicles because of their physiological compatibility. Cholesterol, phospholipids, triglycerides, fatty acids, among others, are examples of raw materials used in lipid carriers [Citation17]. Several advantages are associated with lipid nanocarriers as sustained release of hydrophobic drugs, lower incidence of matrix erosive phenomena, simple and less expensive manufacturing compared with polymeric carriers. The main lipid carriers systems for controlled drug release are liposomes, solid lipid nanoparticles and nanostructured lipid carriers [Citation32].
Liposomes
Spherical lipid vesicles, namely liposomes, are structures composed by one or more concentric phospholipid bilayer enclosing an aqueous inner core. These structures are formed according to self-assembled of phospholipids in an aqueous medium, forming vesicles. The classical lipid film hydration method described for producing liposomes can furnish three different types of structures: multilamellar vesicles (MLVs) with mean diameter >500 nm; large unilamellar vesicles (LUVs) exhibiting diameters greater than 100 nm and small unilamellar vesicles (SUVs) with diameters not much larger than 100 nm [Citation33]. These vesicles are commonly prepared with amphiphilic lipids as phosphatidylcholines, phosphatidylethanolamines and phosphatidylserines. The cholesterol is used to enhance bilayer fluidity [Citation33].
Liposomes encapsulating ART drugs have been described to enhance therapeutic effectiveness in malaria and cancer treatment (). In fact, using MCF-7 cell lines, PEGylated liposomes with and DHA exhibited higher efficacy than free drug solutions, confirming the modified release of ART compounds of the vesicles [Citation34]. Conventional liposomes have been reported to be useful to deliver ART and its derivatives with antitumoral purposes [Citation35]. Want and coworkers studied immunomodulation effects of liposomes containing ART in visceral leishmaniasis model. It was observed a reduction of infected macrophages as well as amastigotes, improving the disease progression. Also, it was verified the positive DTH with an increase of Th1 cytokines levels [Citation36]. The presence of transferrin in ART-liposomes improved the cytotoxic effects of the drug, confirmed by the synergism between iron and ART and the increase of transferrin receptor overexpressed on the surface of HCT-8 cells, allowing a more effective targeting of the liposomes [Citation37]. Addition of mannose onto the surface of the liposomes may also target the vesicles to a target site. Kang and coworkers developed mannosylated liposomes containing DHA. The nanosystem was able to inhibit tumor growth above 88% in colorectal cancer model, indicating the elevation of the apoptosis phenomenon in tumor lines [Citation38].
Table 2. Summary of the main works with lipid nanocarriers and artemisinin and its derivatives.
Nevertheless, liposomal formulations were quite effective mainly in the treatment of parasitic diseases and tumours, suitable for long blood circulation or surface-modified with targeting moieties.
Solid lipid nanoparticles and nanostructured lipid carriers
At 1990s, another type of lipid nanocarriers has arisen as a potential drug delivery system, the solid lipid nanoparticles (SLNs) [Citation32]. SLNs are spherical matrix lipid structures used to encapsulate hydrophobic drugs and have emerged as alternative carriers to liposomes and nanoemulsions. SLNs are composed of lipids generally solid both room and body temperature such as high purity fatty acids, waxes, triglycerides, among others, and surfactants, ranging size from 50 to 1000 nm [Citation39]. These particles show biocompatibility, high physicochemical stability when compared to liposomes, lack of toxicity and provide sustained release as well as easy industrial scale-up. However, some disadvantages are related to nanocarriers as crystallization of lipids culminating drug expulsion during storage [Citation32]. To overcome these problems, a second generation of lipid nanoparticles called nanostructured lipid carriers (NLCs) has been created. The NLCs are composed of both solid and liquid lipids and an aqueous phase containing surfactants [Citation40]. The oil incorporation promotes imperfections in crystallization of solid lipids, leading to lipid lattices with many spaces.
A few types of research reported in the literature involving SLN or NLC and ART drugs (). ARE drug was incorporated in SLN aiming to improve pharmacokinetics parameters, which was confirmed with enhancing of absorption, compared with free drug [Citation41]. In malaria infected-mice, DHA-loaded SLN presented antimalarial efficacy in vitro and in vivo models, compared with free DHA [Citation42]. Another approach that has been used in the treatment of malaria is the combination of two drugs such as ARTE and lumefantrine (LMF). In two studies, this co-loading system was used in malaria models. A first study, Parashar and coworkers demonstrated that the NLC system containing ARTE and LMF was able to reduce parasitemia and increased the survival of infected mice [Citation43]. Another study, conducted by Prabhu and coworkers, it was demonstrated that the nanosystem ARTE-LMF-NLC was able to revert the symptoms of cerebral malaria and kill the parasites, with a survival of all animals tested with complete clearance of the parasite given once a day to infected mice [Citation44].
As seen, lipids NPs have innumerous advantages of application for ART and its derivatives. However, there is a tendency in the increase of the use of the NLC because of its physicochemical advantages which reflect in the improvement of in vitro and in vivo performance of these NDDS.
Carbon-based nanoparticles
Carbon nanomaterials have mechanical, electronic, optical and chemical interesting properties for many applications. Among them, carbon nanotubes (CNTs) and fullerenes are the ones that have most attention and recent studies have shown the high potential of these materials for medical applications [Citation45]. The CNTs are cylindrical large molecules in hexagonal array of low-dimensional sp2 carbon materials. The structure consists of single or multiple curved graphene sheets, being single wall (SWCNTs) and multiple wall (MWCNTs), which still differ in diameter [Citation46]. The fullerenes have a hollow spherical structure, where the carbon atoms are arranged in polycyclic rings [Citation47]. A past few years, CNTs and fullerenes have attracted considerable interest in drug delivery. These interests include a high drug loading, intrinsic stability and structural flexibility; hence, the bioavailability of the drug carried [Citation46].
Carbon-based nanoparticles were evaluated as potential candidates for ARTs delivery (). Zhang and coworkers tested HA-grafted MWCNTs containing ART aiming antitumoral applications. The presence of transferrin ligand in nanosystems enhanced cytotoxicity and presented synergistic antitumor effects in MCF-7 cells and tumour-bearing murine model [Citation48]. In another study, Zhang and coworkers used the same strategy in HA-grafted fullerenes containing AS. The transferrin moiety was capable to transfer synchronously the drug and iron ions into the tumour, enhancing antitumor effects [Citation49]. The strategy of use transferrin ligands was also employed to graphene oxide nanoparticles with DHA. Liu and coworkers developed a smart system to deliver DHA and iron aiming to target tumour cells with overexpressed transferrin receptor. This approach leads to a burst of intracellular ROS and enhances the DHA cytotoxicity in the tumor xenograft animal model [Citation50].
Table 3. Summary of the main works with carbon-based and magnetic nanoparticles and artemisinin and its derivatives.
As seen, carbon-based nanoparticles have been used to incorporate ART compounds. However, possible inherent toxic effects of extending the use of CNTs must be summarily investigated to ensure the safety.
Magnetic nanoparticles
The magnetic NPs (MNPs) are composed of different magnetic oxides, such as iron, zinc, manganese and cobalt, being the most common the iron oxides magnetite (Fe3O4) and maghemite (Fe2O3). Each NP becomes an electrically charged single domain that exhibits superparamagnetic characteristics, behaving as a giant paramagnetic atom that replies quickly to a magnetic field but with almost zero residual coercivity and residual magnetism [Citation51]. The ability to interact with an external magnetic field allows these particles to be transported to targets of interest because the tissues are permeable to the magnetic field and these particles do not retain remaining magnetization [Citation52]. Surface modifications including proteins or antibodies, PEGylation and inorganic layers (silica, alumina) allow biocompatibility of magnetic NPs, demonstrating a wide field of biomedical applications and have been used in the treatment of cancer, as carriers of chemotherapy. In addition, the presence of iron core, magnetic NPs are suitable for magneto-hyperthermia treatment [Citation51,Citation52].
ART and its derivatives were loaded in MNPs with antitumoral purposes (). The novel pH-sensitive MNPs, namely metal-organic-frameworks (MOFs), have arisen as a new approach as drug delivery systems. Multifunctional nanocarriers FCA@mSiO2 could serve as both iron and ART reservoirs. The pH-responsive systems were capable to enhance HeLa cells killing and co-delivery inside the cells improved the destruction of cancer cells [Citation53]. Chen and coworkers prepared pH-responsive Fe3O4@MnSiO3-FA NPs aiming the efficiently deliver of ART and Mn2+ into tumours in mice models. Manganese ions released in acidic environments and intracellular lysosomes could interact with ART to kill cancer cells [Citation54]. The magnetic titanium nanocomposites coated with hyaluronic acid (HA-TiO2-IONPs) containing ART exhibited high cytotoxicity in MCF-7 lines and combined with laser irradiation provided best anti-tumour efficacy [Citation55].
The encapsulation of ART and its derivatives into magnetic NPs enhanced their biological activity and are quite promising for antitumor therapy; however, further studies are needed to clarify the efficacy of these new systems.
Conclusions and future perspectives
Because of the many advantages of nanoparticles, the world scientific production had reported their use in the past years and clearly indicates the evolution of this technology. To assess this growth, we performed a search in the PubMed database using the term “nanotechnology” in the subject field. This led to the recovery of 65.167 records between January 2007 and December 2017. Over this period, the number of publications per year increased from 348 in 2007 to 759 in 2017 (). We also conducted a more specific search on the database, including the word “ART” () along with “immunology” () or association with drug delivery systems. It is interesting that in the last 10 years we have observed a great increase in the search for immunological modulation using ART. We also analysed the types of nanocarriers that have published articles about ART involving the immunology. The ranking of the eight main nanotechnologies is presented in .
Figure 3. Number of reports over 10 years using the terms: (a) “Nanotechnology”; (b) “Artemisinin”; (c) “Artemisinin” and “Immunology” and (d) type of nanocarriers used to encapsulate artemisinin drugs with terms “Artemisinin” and “Drug Delivery”.
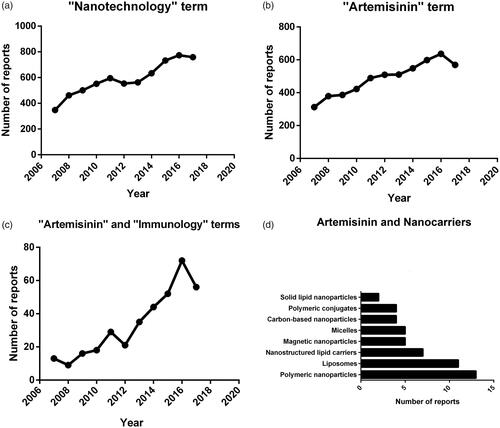
In this article, we perform a review of the association between ART compounds and NDDS. The nanomaterials have been shown to be very advantageous in the several applications of these drugs. We noticed that the lipid carriers are the most used followed by the polymeric nanoparticles and liposomes. Accordingly, some physicochemical and pharmacokinetic problems were solved (low water solubility, poor bioavailability and short half-life), improving the efficacy of these drugs in the treatment of infectious diseases and cancer. Several articles have addressed pharmacological improvement with the use of NPs. However, there are few types of research on the performance of NDDS containing ART and its derivatives in immunomodulation. The nanoencapsulation of the ARTs allows the improvement of the bioavailability of the drugs, reaching the target sites with the same efficiency. Thus, the immunomodulatory mechanisms of this class of drugs can be evidenced in detail next years.
New strategies may be employed to enhance the application of NDDS for ARTs. The use of chitosan-coated nanoparticles may be an alternative to improve the bioavailability of ART because chitosan coating has mucoadhesive properties interacting with mucus layer, increasing the residence time at the site of absorption which helps to modulate the drug release achieving an adequate drug concentration at specific sites in immune system. Moreover, the conjugation of specific antibodies with nanocarriers may be a viable alternative to improve the efficacy of ART compounds. Although, in recent years, our knowledge of ART and its derivatives associated with drug delivery systems has improved, new researches are needed to investigate the effects of nanotechnology on the cellular and molecular immune response of CD4 and CD8 T cells. Further studies evaluating ARTs-loaded nanosystems and immunomodulatory effects are required to improve our understanding of their effects on the immune system.
Geolocation information
São Paulo State, Brazil.
Acknowledgments
The authors would like to thank the agencies São Paulo Research Foundation (Fapesp), Minas Gerais Research Foundation (Fapemig), National Council for Scientific and Technological Development (CNPq) and Coordination for Improvement of Higher Education Personnel (CAPES).
Disclosure statement
The authors report no conflict of interest.
References
- Atanasov AG, Waltenberger B, Pferschy-Wenzig E-M, et al. Discovery and resupply of pharmacologically active plant-derived natural products: a review. Biotechnol Adv. 2015;33:1582–1614.
- Dias PC, Foglio MA, Possenti A, et al. Antiulcerogenic activity of crude ethanol extract and some fractions obtained from aerial parts of Artemisia annua L. Phytother Res. 2001;15:670–675.
- Foglio MA, Dias PC, Antônio MA, et al. Antiulcerogenic activity of some sesquiterpene lactones isolated from Artemisia annua. Planta Med. 2002;68:515–518.
- Favero F. d F, Grando R, Nonato FR, et al. Artemisia annua L.: evidence of sesquiterpene lactones’ fraction antinociceptive activity. BMC Complement Altern Med. 2014;14:266.
- Santomauro F, Donato R, Sacco C, et al. Vapour and liquid-phase Artemisia annua essential oil activities against several clinical strains of candida. Planta Med. 2016;82:1016–1020.
- Tajehmiri A, Issapour F, Moslem MN, et al. In vitro antimicrobial activity of Artemisia annua leaf extracts against pathogenic bacteria – semantic scholar. ASB 2014;6:93–97.
- Lubbe A, Seibert I, Klimkait T, et al. Ethnopharmacology in overdrive: the remarkable anti-HIV activity of Artemisia annua. J Ethnopharmacol. 2012;141:854–859.
- Humphreys C, Cooper AJ, Barbu E, et al. Artemisinins as potential anticancer agents: uptake detection in erythrocytes using Fourier transform infrared spectroscopy and cytotoxicity against bladder cancer cells. J Clin Pathol. 2016;69:962–967.
- Li J, Casteels T, Frogne T, et al. Artemisinins target GABAA receptor signaling and impair α cell identity. Cell 2017;168:86–100.e15.
- Titulaer HAC, Zuidema J, Lugt CB. Formulation and pharmacokinetics of artemisinin and its derivatives. Int J Pharm. 1991;69:83–92.
- Aderibigbe BA. Design of drug delivery systems containing artemisinin and its derivatives. Molecules. 2017;22:323.
- Hou L, Huang H. Immune suppressive properties of artemisinin family drugs. Pharmacol Ther. 2016;166:123–127.
- Shakir L, Hussain M, Javeed A, et al. Artemisinins and immune system. Eur J Pharmacol. 2011;668:6–14.
- Shi C, Li H, Yang Y, et al. Anti-inflammatory and immunoregulatory functions of artemisinin and its derivatives. Mediators Inflamm. 2015;2015:(Article ID 435713):435713–435717.
- Vivek R, Rejeeth C, Thangam R. Targeted nanotherapeutics based on cancer biomarkers. In: Grumezescu AM, organizador. Multifunctional systems for combined delivery, biosensing and diagnostics [Internet]. Elsevier; 2017 [citado 11 de abril de 2018]. p. 229–44. Disponível em: https://doi.org/10.1016/B978-0-323-52725-5.00012-5
- Kapadia CH, Perry JL, Tian S, et al. Nanoparticulate immunotherapy for cancer. J Control Release. 2015;219:167–180.
- Estanqueiro M, Amaral MH, Conceição J, et al. Nanotechnological carriers for cancer chemotherapy: the state of the art. Colloids Surf B. 2015;126:631–648.
- Thipparaboina R, Chavan RB, Kumar D, et al. Micellar carriers for the delivery of multiple therapeutic agents. Colloids Surf B. 2015;135:291–308.
- Jabbarzadegan M, Rajayi H, Mofazzal Jahromi MA, et al. Application of arteether-loaded polyurethane nanomicelles to induce immune response in breast cancer model. Artif Cells Nanomedicine Biotechnol. 2017;45:808–816.
- Manjili HK, Malvandi H, Mousavi MS, et al. In vitro and in vivo delivery of artemisinin loaded PCL-PEG-PCL micelles and its pharmacokinetic study. Artif Cells Nanomedicine Biotechnol. 2018;46:926–936.
- Ramazani A, Keramati M, Malvandi H, et al. Preparation and in vivo evaluation of anti-plasmodial properties of artemisinin-loaded PCL-PEG-PCL nanoparticles. Pharm Dev Technol. 2017;1–10.
- Masood F. Polymeric nanoparticles for targeted drug delivery system for cancer therapy. Mater Sci Eng C Mater Biol Appl. 2016;60:569–578.
- Pérez-Herrero E, Fernández-Medarde A. Advanced targeted therapies in cancer: drug nanocarriers, the future of chemotherapy. Eur J Pharm Biopharm off J Arbeitsgemeinschaft Pharm Verfahrenstechnik EV. 2015;93:52–79.
- Tran TH, Nguyen TD, Poudel BK, et al. Development and evaluation of artesunate-loaded chitosan-coated lipid nanocapsule as a potential drug delivery system against breast cancer. AAPS PharmSciTech. 2015;16:1307–1316.
- Natesan S, Ponnusamy C, Sugumaran A, et al. Artemisinin loaded chitosan magnetic nanoparticles for the efficient targeting to the breast cancer. Int J Biol Macromol. novembro De. 2017;104:1853–1859.
- Want MY, Islamuddin M, Chouhan G, et al. A new approach for the delivery of artemisinin: formulation, characterization, and ex-vivo antileishmanial studies. J Colloid Interface Sci. 2014;432:258–269.
- Want MY, Islamuddin M, Chouhan G, et al. Therapeutic efficacy of artemisinin-loaded nanoparticles in experimental visceral leishmaniasis. Colloids Surf B. 2015;130:215–221.
- Wang L, Wang Y, Wang X, et al. Encapsulation of low lipophilic and slightly water-soluble dihydroartemisinin in PLGA nanoparticles with phospholipid to enhance encapsulation efficiency and in vitro bioactivity. J Microencapsul. 2016;33:43–52.
- Tran TH, Nguyen TD, Van Nguyen H, et al. Targeted and controlled drug delivery system loading artersunate for effective chemotherapy on CD44 overexpressing cancer cells. Arch Pharm Res. 2016;39:687–694.
- Ibrahim N, Ibrahim H, Sabater AM, et al. Artemisinin nanoformulation suitable for intravenous injection: preparation, characterization and antimalarial activities. Int J Pharm. 2015;495:671–679.
- Liu R, Yu X, Su C, et al. Nanoparticle delivery of artesunate enhances the anti-tumor efficiency by activating mitochondria-mediated cell apoptosis. Nanoscale Res Lett. 2017;12:403.
- Naseri N, Valizadeh H, Zakeri-Milani P. Solid lipid nanoparticles and nanostructured lipid carriers: structure, preparation and application. Adv Pharm Bull. 2015;5:305–313.
- Manaia EB, Abuçafy MP, Chiari-Andréo BG, et al. Physicochemical characterization of drug nanocarriers. Int J Nanomedicine. 2017;12:4991–5011.
- Righeschi C, Coronnello M, Mastrantoni A, et al. Strategy to provide a useful solution to effective delivery of dihydroartemisinin: development, characterization and in vitro studies of liposomal formulations. Colloids Surf B. 2014;116:121–127.
- Chen H-J, Huang X-R, Zhou X-B, et al. Potential sonodynamic anticancer activities of artemether and liposome-encapsulated artemether. Chem Commun. 2015;51:4681–4684.
- Want MY, Islammudin M, Chouhan G, et al. Nanoliposomal artemisinin for the treatment of murine visceral leishmaniasis. Int J Nanomedicine. 2017;12:2189–2204.
- Leto I, Coronnello M, Righeschi C, et al. Enhanced efficacy of artemisinin loaded in transferrin-conjugated liposomes versus stealth liposomes against HCT-8 colon cancer cells. Chem Med. 2016;11:1745–1751.
- Kang X-J, Wang H-Y, Peng H-G, et al. Codelivery of dihydroartemisinin and doxorubicin in mannosylated liposomes for drug-resistant colon cancer therapy. Acta Pharmacol Sin. 2017;38:885–896.
- Jain P, Rahi P, Pandey V, et al. Nanostructure lipid carriers: a modish contrivance to overcome the ultraviolet effects. Egypt J Basic Appl Sci. 2017;4:89–100.
- Beloqui A, Solinís MÁ, Rodríguez-Gascón A, et al. Nanostructured lipid carriers: promising drug delivery systems for future clinics. Nanomedicine Nanotechnol Biol Med. 2016;12:143–161.
- Dwivedi P, Khatik R, Khandelwal K, et al. Pharmacokinetics study of arteether loaded solid lipid nanoparticles: an improved oral bioavailability in rats. Int J Pharm. 2014;466:321–327.
- Omwoyo WN, Melariri P, Gathirwa JW, et al. Development, characterization and antimalarial efficacy of dihydroartemisinin loaded solid lipid nanoparticles. Nanomedicine Nanotechnol Biol Med. 2016;12:801–809.
- Parashar D, Aditya NP, Murthy RSR. Development of artemether and lumefantrine co-loaded nanostructured lipid carriers: physicochemical characterization and in vivo antimalarial activity. Drug Deliv. 2016;23:123–129.
- Prabhu P, Suryavanshi S, Pathak S, et al. Nanostructured lipid carriers of artemether-lumefantrine combination for intravenous therapy of cerebral malaria. Int J Pharm. 2016;513:504–517.
- Cancino J, Marangoni VS, Zucolotto V. Nanotechnology in medicine: concepts and concerns. Quím Nova. 2014;37:521–526.
- Kushwaha SKS, Ghoshal S, Rai AK, et al. Carbon nanotubes as a novel drug delivery system for anticancer therapy: a review. Braz J Pharm Sci. 2013;49:629–643.
- Montellano A, Da Ros T, Bianco A, et al. Fullerene C60 as a multifunctional system for drug and gene delivery. Nanoscale. 2011;3:4035–4041.
- Zhang H, Ji Y, Chen Q, et al. Enhancement of cytotoxicity of artemisinin toward cancer cells by transferrin-mediated carbon nanotubes nanoparticles. J Drug Target. 2015;23:552–567.
- Zhang H, Hou L, Jiao X, et al. Transferrin-mediated fullerenes nanoparticles as Fe(2+)-dependent drug vehicles for synergistic anti-tumor efficacy. Biomaterials. 2015;37:353–366.
- Liu L, Wei Y, Zhai S, et al. Dihydroartemisinin and transferrin dual-dressed nano-graphene oxide for a pH-triggered chemotherapy. Biomaterials. 2015;62:35–46.
- Wahajuddin null, Arora S. Superparamagnetic iron oxide nanoparticles: magnetic nanoplatforms as drug carriers. Int J Nanomedicine. 2012;7:3445–3471.
- Mohammed L, Gomaa HG, Ragab D, et al. Magnetic nanoparticles for environmental and biomedical applications: a review. Particuology. 2017;30:1–14.
- Chen J, Guo Z, Wang H-B, et al. Multifunctional mesoporous nanoparticles as pH-responsive Fe2+ reservoirs and artemisinin vehicles for synergistic inhibition of tumor growth. Biomaterials. 2014;35:6498–6507.
- Chen J, Zhang W, Zhang M, et al. Mn(II) mediated degradation of artemisinin based on Fe3O4@MnSiO3-FA nanospheres for cancer therapy in vivo. Nanoscale. 2015;7:12542–12551.
- Zhang H, Zhang H, Zhu X, et al. Visible-light-sensitive titanium dioxide nanoplatform for tumor-responsive Fe2+ liberating and artemisinin delivery. Oncotarget. 2017;8:58738–58753.
- Prabhu P, Suryavanshi S, Pathak S, Patra A, Sharma S, Patravale V, 2016. Nanostructured lipid carriers of artemether-lumefantrine combination for intravenous therapy of cerebral malaria. Int. J. Pharm. 513;504–517. https://doi.org/10.1016/j.ijpharm.2016.09.008