Abstract
Acute alcohol intoxication is a central nervous system disease that accounts for a large number of hospital admissions. In the present study, we have explored the role of GINS complex subunit 2 (GINS2) in acute alcohol intoxication and alcohol-induced brain injury. We began by determining that GINS2 mRNA expression was significantly increased in the serum of patients with alcohol abuse. We then found that GINS2 is increased in mouse brains after alcohol consumption. To explore the role of GINS2 in alcohol-induced microglia function, we knocked down GINS2 in mouse microglia and then treated the cells with alcohol. Knockdown of GINS2 significantly increased alcohol-induced ROS production and the oxidative stress marker malondialdehyde. To explore if GINS2 is involved in alcohol-induced microglia apoptosis, we examined cell viability in GINS2 knockdown cells by TUNEL staining and caspase activity assays. Consistently, results showed that alcohol-induced cell apoptosis was promoted by knockdown of GINS2. Finally, we assessed expression levels of inflammatory factors in GINS2 knockdown microglial cells as well as the effects of GINS2 knockdown on NF-κB signalling. Inflammatory factors were stimulated by alcohol and further promoted by GINS2 knockdown, and GINS2 knockdown promoted alcohol-induced NF-κB activity in microglia.
Introduction
Alcohol consumption is deeply entrenched in societies throughout the world as an accepted cultural practice as well as one of the greatest causes of morbidity and mortality [Citation1,Citation2]. Its negative effects are wide ranging, impacting communities and the individual’s health both chronically and acutely [Citation3]. In the United States, excessive alcohol consumption accounts for 1 in 10 deaths among working-age adults and is a leading cause of premature mortality [Citation4]. In the present study, we have explored the role of GINS complex subunit 2 (GINS2) in acute alcohol intoxication and alcohol-induced brain injury. Ultimately, we have determined that GINS2 plays a crucial protective role in microglia against alcohol-induced brain injury and NF-κB-mediated inflammation.
The central nervous system (CNS) largely mediates both chronic and acute alcohol intoxication [Citation5]. Chronic alcohol intoxication causes altered excitatory and inhibitory signalling cascades as well as neuroinflammatory responses that ultimately results in lasting structural brain abnormalities [Citation6,Citation7]. Acutely, alcohol intoxication causes dramatic impairments in attention, visuomotor control, short-term memory, mood, and cognitive performance [Citation8]. While the short- and long-term effects of alcohol consumption on cognition and behaviour have been extensively studied, more research at the genetic and molecular levels are necessary for clinically assessing and mediating the harmful outcomes. There are currently no clinically reliable objective biomarkers of alcohol abuse or alcoholism, but investigating the molecular and genetic underpinnings of alcohol pathology – as we have in this study – promises to improve diagnosis and treatment of alcohol abuse [Citation9].
Some areas of the brain are more susceptible to the harmful effects of alcohol than others. For example, the hippocampus, white matter, cerebellum, prefrontal cortex and glial cells are especially susceptible to damage [Citation10]. Alcohol’s effects also vary based on age, with greatly noticeable differences between the developing and developed brain [Citation11,Citation12]. However, consistent across both adolescent and adult brains is the significant effect alcohol has on neuroinflammation and subsequent neurotoxicity [Citation13,Citation14]. Acute alcohol intoxication induced neuroimmune gene expression which increases oxidative stress and subsequent neurodegeneration [Citation15]. This alcohol-induced, aberrant molecular activity is part of a complex proinflammatory pathway that includes enhanced microglia and astrocyte activation as well as increased NF-κB signalling, which upregulates transcription of cytokines, oxidases, and proteases [Citation16,Citation17].
Activation of proinflammatory monocytes is a hallmark of alcohol-induced neurodegenerative processes [Citation18,Citation19]. Microglia, which are the brain’s resident macrophage that develops from monocytes, can function as pro- or anti-inflammatory mediators in response to CNS immune insults [Citation20,Citation21]. While microglia can help establish cell homeostasis following alcohol exposure [Citation22], they also exacerbate oxidative stress and neuroinflammation [Citation23,Citation24]. Acute alcohol intoxication activates microglia almost immediately, resulting in NF-κB signalling and increases in several cytokines, including interleukin-1β (IL-1β) [Citation25] and tumour necrosis factor (TNF)-α [Citation26]. In this study, we therefore focused on the effects of acute alcohol intoxication on microglia, specifically how their functions are impacted by knockdown of GINS complex subunit 2 (GINS2), which we determined was upregulated by alcohol in rodent brain tissues in a 2015 study [Citation27]. Ultimately, we established that GINS2 knockdown increases alcohol-induced brain inflammation, oxidative pressure, microglia endoplasmic reticulum (ER) stress, microglia apoptosis, and NF-κB activity.
Materials and methods
Human samples
The study was approved by the Ethics Committee of the Fourth Affiliated Hospital of Harbin Medical University. This study includes 10 men with alcohol abuse who were hospitalized in the Neurology Department of the Fourth Affiliated Hospital of Harbin Medical University. Written informed consent was received from all patients. Fresh serum samples were collected from all participants and frozen in liquid nitrogen and then stored at −80 °C.
Animals
This study was approved by the Institutional Animal Care and Use Committee (IACUC) of Harbin Medical University. 36 C57BL/6 mice (5–6 weeks old) were randomly divided into four groups. Alcohol consumption followed a two-bottle choice-drinking in the dark paradigm [Citation28,Citation29]. For 3 weeks, the animals received 0% or 15% alcohol from separate bottles during a 3 h period starting 3 h into the dark cycle to avoid side preference. The groups were pair-fed diets with equal amounts of water. Study mice were sacrificed under anaesthesia and brain tissues were excised for analysis.
Culture of primary microglial cells
Mouse primary microglial cell cultures were prepared as previously described [Citation27,Citation30].
RT-PCR
RT-PCR were performed as previously described [Citation31]. Sense and antisense primer sequences were ATF4 sense 5′-GCCGGTTTAAGTTGTGTGCT-3′; ATF4 antisense 5′-CTGGATTCGAGGAATGTGCT-3′; CHOP sense 5′-CGGAACCTGAGGAGAGAGTG-3′; CHOP antisense 5′-CGTTTCCTGGGGATGAGATA-3′; GRP78 sense 5′-CGACCTGGGGACCACCTACT-3′; GRP78 antisense 5′-TTGGAGGTGAGCTGGTTCTT-3′; GINS2 sense 5′-AATGCCCAGCCCTTACTA-3′; GINS2 antisense 5′-GGATTTCGTCTGCCTTCG-3′; IL-1β sense 5′-AATCTCACAGCAGCACATCAA-3′; IL-1β antisense 5′-AGCCCATACTTTAGGAAGACA-3′; IL-4 sense 5′-AACGAGGTCACAGGAGAAGG-3′; IL-4 antisense 5′-TCTGCAGCTCCATGAGAACA-3′; IL-6 sense 5′-TGGAGTCACAGAAGGAGTGGCTAAG-3′; IL-6 antisense 5′-TCTGACCACAGTGAGGAATGTCCAC-3′; IL-10 sense 5′-ATAACTGCACCCACTTCCCA-3′; IL-10 antisense 5′-GGGCATCACTTCTACCAGGT-3′; TNF-α sense 5′-CCCCTCAGCAAACCACCAAGT-3′; TNF-α antisense 5′-CTTGGGCAGATTGACCTCAGC-3′; IFN-γ sense 5′-TTCTTCAGCAACAGCAAGGC-3′; IFN-γ antisense 5′-TCAGCAGCGACTCCTTTTCC-3′; β-actin sense 5′-CCACACCCGCCACCAGTTCG-3′ and β-actin antisense 5′-CCCATTCCCACCATCACACC-3′.
Western blot
We performed western blot as previously described [Citation31]. Antibodies against GINS2, ATF4, CHOP, GRP78, p65, Histone H1 and β-actin were purchased from Santa Cruz Biotechnologies (Santa Cruz). Protein levels of Histone H1 and β-actin were used as loading controls.
ROS measurement
Intracellular ROS production was used to measure oxidative pressure. The microglial cells (1 × 105) were seeded in six-well plates and resuspended in 10 μM DCF-DA for 30 min at 37 °C after treatment. Flow cytometry was then used to analyse ROS levels in the stained cells.
Malondialdehyde and superoxide dismutase measurement
The kits for Malondialdehyde (MDA) levels and superoxide dismutase (SOD) activity measurement (Beyotime, Beijing) were used according to the manufacturer’s instructions.
TUNEL staining
TUNEL staining was performed as previously described [Citation31].
Caspase activity assays
Caspase activity assays were performed as previously described [Citation31].
Immunofluorescent staining
Immunofluorescent staining was performed as previously described [Citation31].
Statistical analysis
Result analysis were showed as mean ± SD from at least three separate experiments. One-way ANOVA was used to analyse differences between groups. p < .05 was considered statistically significant.
Results
Treatment with alcohol induces GINS2 production in microglial cells and brain tissues
From our previous microarray data, we found that GINS2 was upregulated by alcohol in rodent brain tissues [Citation27]. Therefore, in the present study we examined GINS2 mRNA expression in the serum of patients with alcohol abuse and found that GINrS2 expression was significantly increased (). To investigate whether GINS2 plays a role in alcohol-induced brain injury, we analysed GINS2 expression in the brains of mice fed with alcohol. We found that GINS2 mRNA and protein level is increased in mouse brains after alcohol consumption (). In addition, there was no difference in weight gain between the control and alcohol-feed groups (). To assess the effects of alcohol on microglia, we treated primary microglial cells with different concentrations of alcohol and then analysed GINS2 expression 12 h post-treatment. GINS2 expression was significantly higher after alcohol treatment in a dose-dependent manner (F)). Together, these results indicate that alcohol increases GINS2 expression in brain tissues and primary microglia cells.
Figure 1. Treatment with alcohol induces GINS2 production in rodent brain tissues and microglial cells. (A) RT-PCR analysis of GINS2 mRNA expression in the serum of 10 healthy people and 10 patients with alcohol abuse. (B) RT-PCR analysis of GINS2 mRNA expression in the brains of mice fed with alcohol (0% or 15%) for 3 weeks. (C) Western blot analysis of GINS2 protein expression in the brains of mice fed with alcohol (0% or 15%) for 3 weeks. (D) Body weight gain in mice fed with alcohol (0% or 15%) for 3 weeks. (E) RT-PCR analysis of GINS2 mRNA expression in mouse microglial cells treated with alcohol (0, 25, 50 or 75 mM). (F) Western blot analysis of GINS2 protein expression in mouse microglial cells treated with alcohol (0, 25, 50 or 75 mM). *p < .05 compared with the control group.
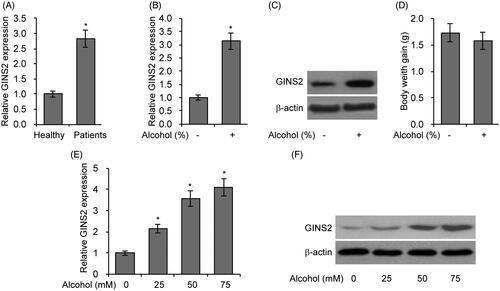
GINS2 knockdown enhances oxidative pressure induced by alcohol
To explore the role of GINS2 in alcohol-induced microglial cell function, we knocked down GINS2 gene in mouse microglial cells and then exposed the cells to 75 mM alcohol. Knockdown efficiency was confirmed by western blotting (). Because prior research has established a close relationship between oxidant stress and alcohol-induced brain injury [Citation32], we used flow cytometry to assess the effect of alcohol treatment on intracellular ROS levels. As shown in , GINS2 knockdown significantly increased alcohol-induced ROS production. To further assess oxidative pressure, we assessed levels of MDA, a degradation product of membrane lipid oxidation. GINS2 knockdown significantly enhanced alcohol-induced MDA production (). We then examined levels of the endogenous anti-oxidative enzyme SOD. GINS2 knockdown significantly decreased the amount of free SOD in alcohol treated microglial cells (). Overall, these results indicate that reduced GINS2 expression increases alcohol-induced oxidative pressure in microglial cells.
Figure 2. GINS2 knockdown enhances alcohol-induced oxidative pressure. (A) GINS2 protein expression measured by western blotting after GINS2 siRNA transfection. (B) ROS production levels measured by DCF-DA staining and flow cytometric analyses in mouse microglial cells treated or not treated with alcohol (75 mM) after GINS2 siRNA transfection. (C) MDA levels in mouse microglial cells treated or not treated with alcohol (75 mM) after GINS2 siRNA transfection. (D) SOD levels in mouse microglial cells treated or not treated with alcohol (75 mM) after GINS2 siRNA transfection. *p < .05 compared with the control group not treated with alcohol.
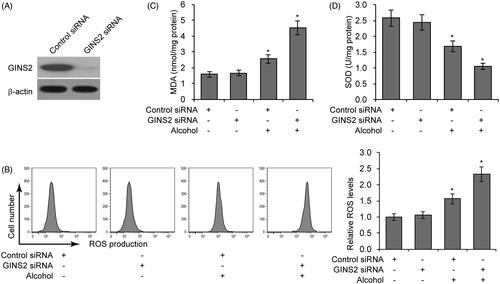
GINS2 knockdown increases ER stress in microglial cells treated with alcohol
Prior research has determined that endoplasmic reticulum (ER) stress is highly associated with alcohol-induced brain cell injury [Citation33,Citation34]. We therefore examined three biomarkers of ER stress (ATF4, CHOP and GRP78) in microglial cells. As shown in , treatment with alcohol promoted expression of ATF4, CHOP and GRP78, and GINS2 knockdown further increased the levels of these markers. Taken together, the above findings strongly suggest that GINS2 knockdown enhances alcohol-induced ER stress in microglial cells.
Figure 3. GINS2 knockdown increases ER stress in microglial cells treated with alcohol. (A) RT-PCR analysis of ATF4, CHOP and GRP78 mRNA expression in mouse microglial cells treated or not treated with alcohol (75 mM) after GINS2 siRNA transfection. *p < .05 compared with the control group not treated with alcohol. (B) Western blot of ATF4, CHOP and GRP78 protein expression in mouse microglial cells treated or not treated with alcohol (75 mM) after GINS2 siRNA transfection.
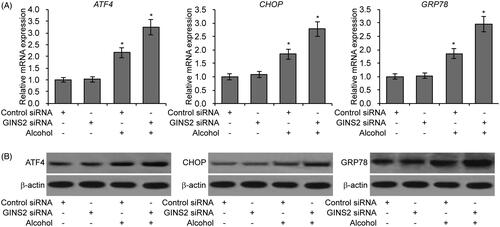
GINS2 knockdown increases alcohol-induced apoptosis in microglial cells
To explore if GINS2 is involved in alcohol-induced apoptosis in microglial cells, we examined cell viability in control and GINS2 knockdown cells. In control cells, cell viability decreased by 50% with alcohol treatment, whereas in GINS2 knockdown cells, cell viability decreased by almost 60% with alcohol treatment (). TUNEL staining, caspase-3 and caspase-9 activity assays consistently showed that alcohol-induced cell apoptosis was promoted by knockdown of GINS2 (). Overall, these results suggest that the presence of GINS2 protects against alcohol-induced apoptosis in microglial cells.
Figure 4. GINS2 knockdown decreases alcohol-induced apoptosis in microglial cells. (A–D) Cell viability (A), caspase-3 activity (B), caspase-9 activity (C) and TUNEL staining (D) assays in GINS2 knockdown microglia treated or not treated with alcohol (75 mM). *p < .01 compared with the control group not treated with alcohol.
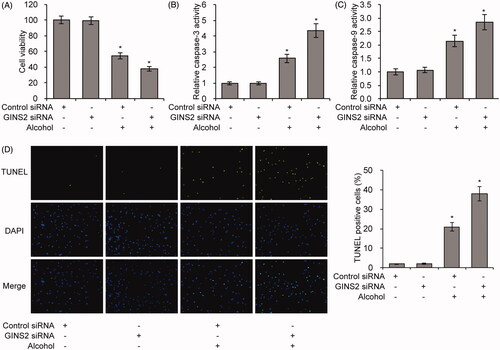
GINS2 regulates NF-κB signalling
To investigate whether GINS2 regulates alcohol-induced rodent brain inflammation, we evaluated expression of inflammatory factors in GINS2 knockdown microglial cells. IL-1β, IL-6, IL-10, TNF-α and IFN-γ expression was stimulated by alcohol and further promoted by GINS2 knockdown (. To determine whether NF-κB signalling is affected by alcohol-induced, GINS2-mediated inflammation, we treated GINS2 knockdown mouse microglial cells with alcohol and extracted nuclear proteins for p65 expression. Alcohol promoted p65 nuclear translocation, which was enhanced by GINS2 knockdown (). NF-κB luciferase activity and cell fluorescence staining assays confirmed that GINS2 knockdown in microglial cells further promoted alcohol-induced NF-κB activity ( and ). These results indicate that GINS2 knockdown increases alcohol-induced brain inflammation and alcohol-induced NF-κB activity.
Figure 5. GINS2 knockdown promotes the expression of pro-inflammatory cytokines in microglial cells treated with alcohol. (A–F) RT-PCR analysis of IL-1β (A), IL-4 (B), IL-6 (C), IL-10 (D), TNF-α (E) and IFN-γ (F) mRNA expression in mouse microglia treated or not treated with alcohol (50 mM) after GINS2 siRNA transfection. *p < .01 compared with the control group not treated with alcohol.
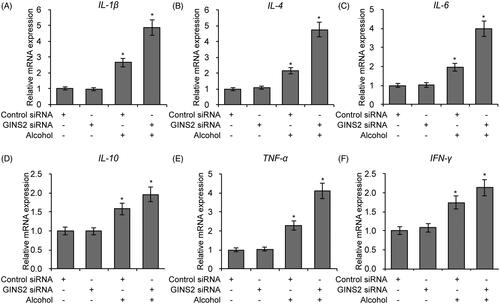
Figure 6. GINS2 regulates NF-κB Signaling. (A–C) Western blot analysis of nuclear p65 protein levels (A), Relative NF-κB luciferase activity (B) and Cell fluorescence staining of p65 (C) in mouse microglia treated or not treated with alcohol (50 mM) after GINS2 siRNA transfection. *p < .01 compared with the control group not treated with alcohol.
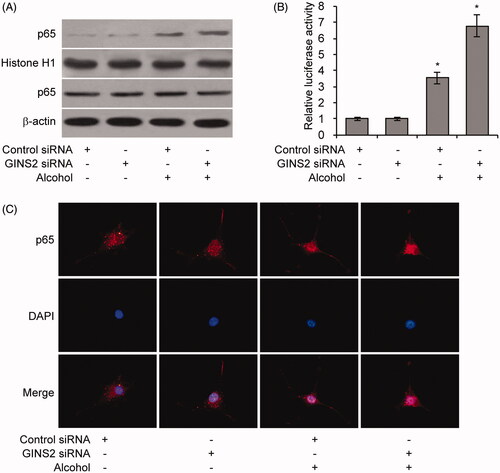
Discussion
Despite its presence in almost all societies, alcohol consumption continues to contribute greatly to the global burden of disease, accounting for around 3.8% of global deaths and 4.6% of global disability-adjusted-life-years [Citation35]. Acute alcohol intoxication occurs following ingestion of significant amounts of alcohol over a short period of time. Symptoms of acute alcohol intoxication vary with severity and can include impaired judgement, memory deficits, inappropriate aggressive behaviour, coma, and other harmful cognitive-behavioural conditions [Citation36]. While alcohol has been extensively studied, especially for its social and economic consequences, the genetic and molecular mechanisms of alcohol’s effects on the CNS have not been fully elucidated. In the present study, using patient serum, an experimental mouse model of acute alcohol intoxication, and primary mouse microglia, we carried out extensive analyses of the effects of GINS2 knockdown on alcohol-induced neuroinflammation and neurotoxicity. Ultimately, our study has established a crucial, protective role for GINS2 in acute alcohol intoxication, thus providing the basis for further research into clinical biomarkers of acute alcohol intoxication and effective clinical treatments.
We focused on the activity of GINS2 because our prior research using microarray data revealed that alcohol upregulates GINS2 expression in rodent brain tissues [Citation27]. GINS was originally identified in 2000 by Lai et al. as an evolutionarily conserved protein product in Caenorhabditis elegans [Citation37], but the first study to focus entirely on the GINS multiprotein complex was conducted by Takayama et al. [Citation38]. In this study, it was determined that GINS forms part of a protein complex required for the initiation of chromosomal DNA replication in yeast. Since then, GINS has been shown to associate with Cdc45 and Mcm2-7 in a helicase complex for leading strand DNA synthesis in human cells [Citation39]. Not only is the GINS complex crucial for normal human physiology in the domain of cell proliferation but it has also been shown to play roles in a variety of pathologies, largely cancers [Citation40]. However, no studies have focused on its function in the CNS or alcohol-induced CNS changes. The present study therefore contributes substantially to the growing body of work concerning GINS2 function in pathology and opens the door for more research into its role in the CNS. Furthermore, this study establishes a previously little explored relationship between GINS2 and oxidative pressure, ER stress, and NF-κB signalling.
Microglia are the resident macrophages of the CNS, and they are highly active in the healthy and pathological CNS, responding to disturbances in blood vessels, neurons, and the blood brain barrier, among many other fundamental CNS components [Citation41]. As the primary immune effectors of the CNS derived from circulating monocytes, microglia act as antigen presenting cells and travel to sites of tissue damage to secrete cytokines and other proinflammatory constituents [Citation42]. They serve crucial, sometimes seemingly contradictory, functions in neurodegenerative diseases [Citation43]. In response to neurological insults, microglia adopt variable phenotypes, which can be anti- or proinflammatory depending on the specific CNS challenge [Citation20]. Additionally, microglia can sometimes be induced to produce ROS that causes neurodegeneration, such as in the case of the organochlorinated pesticide dieldrin [Citation44], or they can scavenge oxygen-derived free radicals, such as during excitotoxin-induced neurodegeneration [Citation45]. In the present study, we have determined that GINS2 knockdown in microglia has a wide range of negative consequences for microglia when treated with alcohol, including increased oxidative pressure, ER stress, and apoptosis. These results strongly suggest that GINS2 serves a protective role in microglia when its expression is induced by alcohol consumption.
Even though it is generally believed that alcohol consumption induces an inflammatory state systemically [Citation46] and in the CNS [Citation19], the effects of acute alcohol intoxication on immune regulation are not entirely understood. Alcohol consumption has been shown to stimulate toll-like receptor 2 (TLR2) and TLR4 expression in microglia, thus increasing ROS, neuronal apoptosis, and proinflammatory mediators [Citation47]. Furthermore, in a rodent model of binge drinking, alcohol exposure increased hippocampal microglia activation, thus inducing a heightened state of neuroinflammation [Citation48]. NF-κB signalling is integral to alcohol-induced neuroinflammation, as alcohol can directly increase NF-κB transcription of proinflammatory genes, such as through alcohol’s release of the TLR4 agonist High Mobility Group Box 1 [Citation49]. This alcohol-induced proinflammatory signalling can be attenuated, for example, with the anti-inflammatory drug xanthohumol, which inhibits microglial inflammatory responses by reducing NF-κB signalling [Citation50]. By demonstrating that GINS2 knockdown increases alcohol-induced NF-κB signalling, this study indicates that GINS2 expression may induce microglia to serve a more anti-inflammatory role to reduce alcohol-induced neuroinflammation.
Overall, the present study has achieved compelling results regarding the role of GINS2 in acute alcohol intoxication, specifically in microglia integrity and neuroinflammation. The results suggest that GINS2 expression can potentially serve as a biomarker of alcohol-induced neuroinflammation and can help identify microglia that act in an inflammatory capacity. Perhaps most importantly, the results here open new avenues for understanding the complex nature of alcohol-induced neurotoxicity and neuroinflammation.
Disclosure statement
No potential conflict of interest was reported by the authors.
Additional information
Notes on contributors
Chunhua Liu
CL and RW performed the experiments and collected data. CL and YZ analysed the data and wrote the manuscript. YZ designed the work.
Renfu Wang
CL and RW performed the experiments and collected data. CL and YZ analysed the data and wrote the manuscript. YZ designed the work.
Yu Zhang
CL and RW performed the experiments and collected data. CL and YZ analysed the data and wrote the manuscript. YZ designed the work.
References
- Carbia C, Lopez-Caneda E, Corral M. A systematic review of neuropsychological studies involving young binge drinkers. Neurosci Biobehav Rev. 2018;90:332–349. doi: 10.1016/j.neubiorev.2018.04.013. PubMed PMID: 29678643; eng.
- Grant BF, Saha TD, Ruan WJ, et al. Epidemiology of DSM-5 drug use disorder: results from the national epidemiologic survey on alcohol and related conditions-III. JAMA Psychiatry. 2016;73:39–47. doi: 10.1001/jamapsychiatry.2015.2132. PubMed PMID: 26580136; PubMed Central PMCID: PMCPMC5062605. eng.
- Scaglione S, Kliethermes S, Cao G, et al. The epidemiology of cirrhosis in the United States: a population-based study. J Clin Gastroenterol. 2015;49:690–696. doi: 10.1097/mcg.0000000000000208. PubMed PMID: 25291348; eng.
- Stahre M, Roeber J, Kanny D, et al. Contribution of excessive alcohol consumption to deaths and years of potential life lost in the United States. Prev Chronic Dis 2014;11:E109. doi: 10.5888/pcd11.130293. PubMed PMID: 24967831; PubMed Central PMCID: PMCPMC4075492. eng.
- Bjork JM, Gilman JM. The effects of acute alcohol administration on the human brain: insights from neuroimaging. Neuropharmacology 2014;84:101–110. doi: 10.1016/j.neuropharm.2013.07.039. PubMed PMID: 23978384; PubMed Central PMCID: PMCPMC3971012. eng.
- Kubota M, Nakazaki S, Hirai S, et al. Alcohol consumption and frontal lobe shrinkage: study of 1432 non-alcoholic subjects. J Neurol Neurosurg Psychiatry. 2001;71:104–106. PubMed PMID: 11413273; PubMed Central PMCID: PMCPMC1737442. eng.
- Fein G, Sclafani V, Cardenas VA, et al. Cortical gray matter loss in treatment-naive alcohol dependent individuals. Alcohol Clin Exp Res. 2002;26:558–564. PubMed PMID: 11981133; PubMed Central PMCID: PMCPMC2435064. eng.
- Zoethout RW, Delgado WL, Ippel AE, et al. Functional biomarkers for the acute effects of alcohol on the central nervous system in healthy volunteers. Br J Clin Pharmacol. 2011;71:331–350. doi: 10.1111/j.1365-2125.2010.03846.x. PubMed PMID: 21284693; PubMed Central PMCID: PMCPMC3045543. eng.
- Achur RN, Freeman WM, Vrana KE. Circulating cytokines as biomarkers of alcohol abuse and alcoholism. J Neuroimmune Pharmacol. 2010;5:83–91. doi: 10.1007/s11481-009-9185-z. PubMed PMID: 20020329; PubMed Central PMCID: PMCPMC2862254. eng.
- Alfonso-Loeches S, Guerri C. Molecular and behavioral aspects of the actions of alcohol on the adult and developing brain. Crit Rev Clin Lab Sci. 2011;48:19–47. doi: 10.3109/10408363.2011.580567. PubMed PMID: 21657944; eng.
- Clark DB, Thatcher DL, Tapert SF. Alcohol, psychological dysregulation, and adolescent brain development. Alcohol Clin Exp Res. 2008;32:375–385. doi: 10.1111/j.1530-0277.2007.00601.x. PubMed PMID: 18241320; eng.
- Guerri C, Pascual M. Mechanisms involved in the neurotoxic, cognitive, and neurobehavioral effects of alcohol consumption during adolescence. Alcohol 2010;44:15–26. doi: 10.1016/j.alcohol.2009.10.003. PubMed PMID: 20113871; eng.
- Crews FT, Lawrimore CJ, Walter TJ, et al. The role of neuroimmune signaling in alcoholism. Neuropharmacology 2017;122:56–73. doi: 10.1016/j.neuropharm.2017.01.031. PubMed PMID: 28159648; PubMed Central PMCID: PMCPMC5493978. eng.
- Crews FT, Vetreno RP. Neuroimmune basis of alcoholic brain damage. Int Rev Neurobiol 2014;118:315–357. doi: 10.1016/b978-0-12-801284-0.00010-5. PubMed PMID: 25175868; PubMed Central PMCID: PMCPMC5765863. eng.
- Qin L, Crews FT. NADPH oxidase and reactive oxygen species contribute to alcohol-induced microglial activation and neurodegeneration. J Neuroinflammation 2012;9:5. doi: 10.1186/1742-2094-9-5. PubMed PMID: 22240163; PubMed Central PMCID: PMCPMC3271961. eng.
- O’Neill LA, Kaltschmidt C. NF-kappa B: a crucial transcription factor for glial and neuronal cell function. Trends Neurosci. 1997;20:252–258. PubMed PMID: 9185306; eng.
- Zou J, Crews F. CREB and NF-kappaB transcription factors regulate sensitivity to excitotoxic and oxidative stress induced neuronal cell death. Cell Mol Neurobiol. 2006; 26:385–405. doi: 10.1007/s10571-006-9045-9. PubMed PMID: 16633891; eng.
- Marshall SA, McClain JA, Kelso ML, et al. Microglial activation is not equivalent to neuroinflammation in alcohol-induced neurodegeneration: the importance of microglia phenotype. Neurobiol Dis. 2013;54:239–251. doi: 10.1016/j.nbd.2012.12.016. PubMed PMID: 23313316; PubMed Central PMCID: PMCPMC3629000. eng.
- Pradier B, Erxlebe E, Markert A, et al. Microglial IL-1beta progressively increases with duration of alcohol consumption. Naunyn-Schmiedeberg’s Arch Pharmacol. 2018;391:455–461. doi: 10.1007/s00210-018-1475-7. PubMed PMID: 29445825; eng.
- Cherry JD, Olschowka JA, O’Banion M. Neuroinflammation and M2 microglia: the good, the bad, and the inflamed. J Neuroinflammation. 2014;11:98. doi: 10.1186/1742-2094-11-98. PubMed PMID: 24889886; PubMed Central PMCID: PMCPMC4060849. eng.
- Krause DL, Muller N. Neuroinflammation, microglia and implications for anti-inflammatory treatment in Alzheimer’s disease. Int J Alzheimers Dis. 2010;2010. doi: 10.4061/2010/732806. PubMed PMID: 20798769; PubMed Central PMCID: PMCPMC2925207. eng.
- Ahlers KE, Karaçay B, Fuller L, et al. Transient activation of microglia following acute alcohol exposure in developing mouse neocortex is primarily driven by BAX-dependent neurodegeneration. Glia 2015;63:1694–1713. doi: 10.1002/glia.22835. PubMed PMID: 25856413; PubMed Central PMCID: PMCPMC4534325. eng.
- Chastain LG, Sarkar DK. Role of microglia in regulation of ethanol neurotoxic action. Int Rev Neurobiol. 2014;118:81–103. doi: 10.1016/b978-0-12-801284-0.00004-x. PubMed PMID: 25175862; eng.
- Kreutzberg GW. Microglia: a sensor for pathological events in the CNS. Trends Neurosci. 1996;19:312–318. PubMed PMID: 8843599; eng.
- Wu Y, Lousberg EL, Moldenhauer LM, et al. Attenuation of microglial and IL-1 signaling protects mice from acute alcohol-induced sedation and/or motor impairment. Brain Behav Immun. 2011;25:S155–S164. doi: 10.1016/j.bbi.2011.01.012. PubMed PMID: 21276848; eng.
- Lippai D, Bala S, Petrasek J, et al. Alcohol-induced IL-1beta in the brain is mediated by NLRP3/ASC inflammasome activation that amplifies neuroinflammation. J Leukoc Biol. 2013;94:171–182. doi: 10.1189/jlb.1212659. PubMed PMID: 23625200; PubMed Central PMCID: PMCPMC3685015. eng.
- Zhang Y, Wei G, Wang Y, et al. Gene expression profile analysis of rat cerebellum under acute alcohol intoxication. Gene 2015;557:188–194. doi: 10.1016/j.gene.2014.12.032. PubMed PMID: 25527120; eng.
- Zhang Y, Wei G, Di Z, et al. miR-339-5p inhibits alcohol-induced brain inflammation through regulating NF-kappaB pathway [research support, non-U.S. Gov’t]. Biochem Biophys Res Commun. 2014;452:450–456. doi: 10.1016/j.bbrc.2014.08.092. PubMed PMID: 25172655; eng.
- Blednov YA, Harris RA. Metabotropic glutamate receptor 5 (mGluR5) regulation of ethanol sedation, dependence and consumption: relationship to acamprosate actions. Int J Neuropsychopharmacol. 2008;11:775–793. doi: 10.1017/s1461145708008584. PubMed PMID: 18377703; PubMed Central PMCID: PMCPMC2574715. eng.
- Frank MG, Wieseler-Frank JL, Watkins LR, et al. Rapid isolation of highly enriched and quiescent microglia from adult rat hippocampus: immunophenotypic and functional characteristics. J Neurosci Methods. 2006;151:121–130. doi: 10.1016/j.jneumeth.2005.06.026. PubMed PMID: 16125247; eng.
- Zhang Y, Gao W, Yang K, et al. Salt-inducible kinase 1 (SIK1) is induced by alcohol and suppresses microglia inflammation via NF-kappaB signaling. Cell Physiol Biochem. 2018;47:1411–1421. doi: 10.1159/000490831. PubMed PMID: 29929190; eng.
- Finnerty N, O’Riordan SL, Klamer D, et al. Increased brain nitric oxide levels following ethanol administration [research support, non-U.S. Gov’t]. Nitric Oxide: Biol Chem. 2015;47:52–57. doi: 10.1016/j.niox.2015.03.002. PubMed PMID: 25819134; eng.
- George AK, Behera J, Kelly KE, et al. Exercise mitigates alcohol induced endoplasmic reticulum stress mediated cognitive impairment through ATF6-herp signaling. Sci Rep. 2018; 8:5158. doi: 10.1038/s41598-018-23568-z. PubMed PMID: 29581524; eng.
- Reynolds AR, Saunders MA, Prendergast MA. Ethanol stimulates endoplasmic reticulum inositol triphosphate and sigma receptors to promote withdrawal-associated loss of neuron-specific nuclear protein/fox-3. Alcohol Clin Exp Res. 2016;40:1454–1461. doi: 10.1111/acer.13097. PubMed PMID: 27177604; eng.
- Rehm J, Mathers C, Popova S, et al. Global burden of disease and injury and economic cost attributable to alcohol use and alcohol-use disorders. Lancet 2009;373:2223–2233. doi: 10.1016/s0140-6736(09)60746-7. PubMed PMID: 19560604; eng.
- Vonghia L, Leggio L, Ferrulli A, et al. Acute alcohol intoxication. Eur J Intern Med. 2008;19:561–567. doi: 10.1016/j.ejim.2007.06.033. PubMed PMID: 19046719; eng.
- Lai CH, Chou CY, Ch’ang LY, et al. Identification of novel human genes evolutionarily conserved in Caenorhabditis elegans by comparative proteomics. Genome Res. 2000;10:703–713. PubMed PMID: 10810093; PubMed Central PMCID: PMCPMC310876. eng.
- Takayama Y, Kamimura Y, Okawa M, et al. GINS, a novel multiprotein complex required for chromosomal DNA replication in budding yeast. Genes Dev. 2003;17:1153. doi: 10.1101/gad.1065903. PubMed PMID: 12730134; PubMed Central PMCID: PMCPMC196052. eng.
- Kang YH, Galal WC, Farina A, et al. Properties of the human Cdc45/Mcm2-7/GINS helicase complex and its action with DNA polymerase epsilon in rolling circle DNA synthesis. Proc Natl Acad Sci U S A. 2012;109:6042–6047. doi: 10.1073/pnas.1203734109. PubMed PMID: 22474384; PubMed Central PMCID: PMCPMC3341058. eng.
- Ouyang F, Liu J, Xia M, et al. GINS2 is a novel prognostic biomarker and promotes tumor progression in early-stage cervical cancer. Oncol Rep. 2017;37:2652–2662. doi: 10.3892/or.2017.5573. PubMed PMID: 28405687; PubMed Central PMCID: PMCPMC5428920. eng.
- Nimmerjahn A, Kirchhoff F, Helmchen F. Resting microglial cells are highly dynamic surveillants of brain parenchyma in vivo. Science 2005;308:1314–1318. doi: 10.1126/science.1110647. PubMed PMID: 15831717; eng.
- Hanisch UK. Microglia as a source and target of cytokines. Glia 2002;40:140–155. doi: 10.1002/glia.10161. PubMed PMID: 12379902; eng.
- Perry VH, Nicoll JA, Holmes C. Microglia in neurodegenerative disease. Nat Rev Neurol. 2010;6:193–201. doi: 10.1038/nrneurol.2010.17. PubMed PMID: 20234358; eng.
- Mao H, Fang X, Floyd KM, et al. Induction of microglial reactive oxygen species production by the organochlorinated pesticide dieldrin. Brain Res. 2007;1186:267–274. doi: 10.1016/j.brainres.2007.10.020. PubMed PMID: 17999924; eng.
- Lindenau J, Noack H, Asayama K, et al. Enhanced cellular glutathione peroxidase immunoreactivity in activated astrocytes and in microglia during excitotoxin induced neurodegeneration. Glia 1998;24:252–256. PubMed PMID: 9728771; eng.
- Zhang P, Bagby GJ, Stoltz DA, et al. Granulocyte colony-stimulating factor modulates the pulmonary host response to endotoxin in the absence and presence of acute ethanol intoxication. J Infect Dis. 1999;179:1441–1448. doi: 10.1086/314763. PubMed PMID: 10228066; eng.
- Fernandez-Lizarbe S, Montesinos J, Guerri C. Ethanol induces TLR4/TLR2 association, triggering an inflammatory response in microglial cells. J Neurochem. 2013;126:261–273. doi: 10.1111/jnc.12276. PubMed PMID: 23600947; eng.
- Ward RJ, Colivicchi MA, Allen R, et al. Neuro-inflammation induced in the hippocampus of “binge drinking” rats may be mediated by elevated extracellular glutamate content. J Neurochem. 2009;111:1119–1128. doi: 10.1111/j.1471-4159.2009.06389.x. PubMed PMID: 19765190; eng.
- Zou JY, Crews FT. Release of neuronal HMGB1 by ethanol through decreased HDAC activity activates brain neuroimmune signaling. PLoS One. 2014;9:e87915. doi: 10.1371/journal.pone.0087915. PubMed PMID: 24551070; PubMed Central PMCID: PMCPMC3925099. eng.
- Lee IS, Lim J, Gal J, et al. Anti-inflammatory activity of xanthohumol involves heme oxygenase-1 induction via NRF2-ARE signaling in microglial BV2 cells. Neurochem Int. 2011;58:153–160. doi: 10.1016/j.neuint.2010.11.008. PubMed PMID: 21093515; eng.