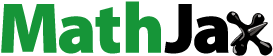
Abstract
The evolution of drug resistance of Candida species to conventional antifungal agents has been a major medical challenge worldwide; attempt to use the potential antifungal agents with appropriate therapy efficacy and minimum effects is considerably growing. This study was conducted to evaluate the use of nanogel as a nanocarrier for pharmaceutical application of farnesol. The nanogels were synthetized using alginate (AL) and chitosan (CS) polymers containing 300 µM of farnesol in the nano-range 42–70 nm size. In vitro release studies indicated that release of farnesol from CS and AL nanogels was as 58% and 37%, respectively. Chitosan nanogel showed more in inhibitory zone as compared to AL nanogel (9 mm). Also, cytotoxicity assay showed no significant difference between control and treatment groups (p>.05). Finally, the effect of nanogels on genes expression of HWP1, SAP6 and Rim101 in Candida albicans ATCC10231 was assessed using real-time polymerase chain reaction (PCR). Expression of HWP1 and SAP6 genes in C. albicans treated with CS nanogel was significantly decreased (p<.01). In general, the obtained finding showed that, CS nanogel contains farnesol with proper antifungal activity and as a new approach used in pharmaceutical applications against C. albicans; however, more studies in vitro and in vivo are needed in the future.
Introduction
Today, the number of immunocompromised (cases due to radio and/or chemotherapy, long-term use of antibiotics corticosteroids, and those who received organ transplant) has been progressively increased [Citation1–3]. On the other hand, emerging resistant Candida species to common antifungal drugs led to the development of new therapeutic strategies, including, liposomes, nano-cochleates, nano-spheres, carbon nanotubes and mucoadhesive nanogels against Candida infection [Citation4]. Recently, the use of natural or bioactive compounds extracted from plants or other microorganisms which have anti-fungal properties with minimum side effects and increase in the antifungal effect for the treatment of invasive fungal infections and candidiasis has been of interest [Citation5,Citation6].
Farnesol is a natural organic compound which is produced from isoprene compounds in plants, animals and fungi [Citation7]. This compound has been suggested to function as an antitumor, apoptosis-promoting effect and inducing growth-inhibitory [Citation8,Citation9]. In fungus such as Candida albicans, farnesol is quorum sensing molecules (QSMs) which can block filamentation and inhibit biofilm formation as well as suppressing the expression of virulence genes as along with biofilm formation [Citation10–14]. The hyphae formation in C. albicans controlled by a network of genes includes aspartyl proteinase 6 (SAP6), Hyphal Wall Protein1 (HWP1) and Rim101 (alkaline-responsive transcriptional factor). The SAP6 gene is a member of aspartyl proteinase family known as secreted proteolytic enzymes. SAP6 and HWP1 genes are expressed strongly in germ tube and hyphal surfaces [Citation15]. Rim101 is a transcription factor that governs virulence in many fungal pathogens. C. albicans Rim101 gene, promotes hyphal formation in response to alkaline growth conditions [Citation16]. The importance of C. albicans hyphae formation and its role on invasion to the host cells have been well documented [Citation17]. Therefore, effective antifungal agent and drug delivery that can prevent C. albicans hyphae form are urgently required. In our previous study by Nikoomanesh et al. [Citation18], we showed that 300 µM concentration of farnesol was prevented from germ tube and hyphae formation of C. albicans. Hence, farnesol was deliberated as an antifungal agent by well-known mechanism. Therefore, hyphae morphology is a new target for novel drug delivery strategy.
Currently, usage of natural polymers is a valuable component for the preparation of mucoadhesive gel due to their biocompatibility and safety. Extensive studies have been focused on the application of mucoadhesive and nanocarriers. As regards the advantages of the nanocarriers drug delivery system, such as bioavailability and promoting biodistribution of drugs, they can be used to overcome therapeutic defects [Citation19]. Nanogels are often synthesized from mucoadhesion polymers [Citation20]. Among the various types of polymers, chitosan (CS) and alginate (AL) are defined as natural base polymers with different application in food and drug industry. Alginate is a polysaccharide polymer that is extracted from a kind of algae which consists of d-mannuronic acid and l-guluronic acid arranged as blocks in the polymeric chain [Citation21]. Chitosan is a copolymer polysaccharide compromised of d-glucosamine and N-acetyl-d-glucosamine prepared from chain by deacetylation of chitin [Citation22].
The present study was performed to design, synthesize and characterize CS and AL nanogels containing farnesol. Finally, the effects of nanogels were examined on gene expression of HWP1, SAP6 and Rim101 as the key genes contribute to hyphae formation in C. albicans in vitro.
Materials and methods
Chemicals
Farnesol purchased from Sigma-Aldrich Company (St. Louis, MO) was prepared in methanol at the concentration of 300 µM. Tetrazolium 3-(4,5-dimethylthiazol-2-yl)-2,5-diphenyltetrazolium bromide) salt was purchased from Sigma-Aldrich (St. Louis, MO). Chitosan (the medium molecular weight (Mw) of 340 g/mol) was purchased from Sigma-Aldrich (St. Louis, MO). Alginate was purchased from Sigma-Aldrich Company (St. Louis, MO). Myristic acid (≥99% purity, MW =228.37 g/mol) was purchased from Sigma-Aldrich (St. Louis, MO). 1-Ethyl-3-(3-dimethylaminopropyl) carbodiimide (EDC; Mw =155.24 g/mol, d = 0.814 g/ml) was purchased from Sigma-Aldrich Company (St. Louis, MO). Intestinal epithelial cell line (SW480) was obtained from Pastor Institute (Tehran, Iran). AMPLIQON master mix (Green High Rox) was purchased from Sinnagene Company (Tehran, Iran). DMEM medium was purchased from Merck Company (Germany). Sabouraud dexterose agar (SDA) and nitrogen base agar (YNB) were purchased from Sigma-Aldrich Company (St. Louis, MO).
Preparation of chitosan and alginate nanogels
Chitosan nano-gel was prepared using self-assembly method with minor modification described previously [Citation23,Citation24]. Briefly, 1 g of CS was dissolved in 100 ml of 1% acetic acid and was added to solution containing 0.1 g myristic in 10 ml ethanol. Then, 0.1 g of EDC as crosslinker and 0.1 g of N-hydroxysuccinimide (NHS) were added, while stirring under hood at room temperature. For preparation of AL nanogel, 1 g of AL was dissolved in 100 ml of water and was stirred, next 100 ml propyl amine and, 0.1 g of EDC as crosslinker and 0.1 g of NHs were added and stirred under hood at room temperature. In the previous study by Nikoomanesh et al. [Citation18], MIC value of farnesol was evaluated. Therefore, farnesol at 300 µM was selected to nanogel synthesis. Farnesol at 300 µM concentration was added to each nanogel and sonicated in water bath (Powersonic S10) with high-power ultrasound at room temperature for 30 min. The pH of CS nanogel was maintained in the range of 4.5–5 and the pH of AL nanogel was maintained between 5 and 5.5.
Physicochemical characterization of synthesized nanogels
Scanning electron microscopy (SEM) was carried out for the analysis of morphology, shape, size and structure of nanogels [Citation24]. For preparing SEM photographs, one drop of each nanogels was dropped onto studs and gold sputtered using an auto sputter coater (BIORAD Polaron Division, Cambridge, MA), then was analyzed with instrument with BELSORP28 Model, Osaka, Japan. Identification of chemical structure and interactions of functional groups of the CS and AL nanogels, were examined using Fourier-transform infrared spectrophotometer (FTIR) test [Citation25]. Spectral scanning was taken in the wavelength region between in the range of 4500 and 500 cm−1. Indeed, the XRD pattern of nanogels was analyzed in the range of 150 < 2θ < 700. In this study, X’pert MPD was used [Citation26].
In vitro experiments
Encapsulation efficiency of farnesol loaded nanogels
To determine their encapsulation efficiency (EE %), previous report was maintained [Citation25]. Briefly, nanogel was first separated by centrifugation at 600 rpm for 10 min at 4 °C from aqueous medium containing nanogels and farnesol. The supernatant was obtained after pelleting out the farnesol-loaded nanogels. Standard curve was plotted using different concentrations of farnesol at 190–195 nm via UV spectrophotometer (Shimadzu Corporation Model UV-2450, Kyoto, Japan). Percentage EE % was determined by using the formula:
Evaluation release of farnesol from nanogels
Release of farnesol was assessed using dialysis method [Citation25]. In brief, the dialysis bag was soaked in distilled water to remove the preservatives and rinsed with phosphate-buffered saline (PBS) solution. Both nanogels containing farnesol were re-dispersed in 3 ml of PBS solution and loaded in the dialysis bag with PBS buffer at pH 7. Time-dependant releasing test was examined for 1–6 days. All sets were incubated at 37 °C under gentle agitation. At definite time intervals, 3 ml of the medium was removed and quantified using spectrophotometer.
Antifungal activity of nanogels contains farnesol
Antifungal activity of nanogels against C. albicans was measured using pure plate method [Citation27]. For this purpose, C. albicans was cultured on sabouraud dextrose agar (SDA) for 48 h and suspension amount of 1 × 103 CFU/ml was prepared 1 ml of suspension was added to 9 ml SDA medium in 45 °C and distributed on plates. Then, two wells with 5 mm diameter were created in the medium. Finally, 500 µl farnesol and nanogels containing farnesol (at 300 µM concentration) were poured in the wells and incubated at 35 °C for 24 h. Thereafter, the inhibitory zone diameter induced by both farnesol and nanogels was measured.
Cytotoxicity assay
The cytotoxicity effect of farnesol and nanogels was considered by colorimetric MTT assay [Citation25]. Epithelial cell line (SW480) was cultured in 500 µl of complete cell culture medium (DMEM) supplemented with 10% foetal bovine serum, 50 µl penicillin/streptomycin (PS), 250 µl l-glutamine and incubated at 37 °C with 5% CO2 for 12, 24, 48 and 72 h. Then, the cells were exposed to nanogels and farnesol for 12, 24, 48 and 72 h. Viability of cells was examined using MTT test at 570 nm using ELISA reader (Star Sate, Germany). The ratio of the absorbance of the treated samples to the absorbance of control (taken as 100%) was expressed as % cell viability. Each experiment was performed triplicate compared to control group (cells without farnesol and nanogels treatments).
Quantitative real-time polymerase chain reaction (qRT-PCR)
The expression of Sap6, HWP1 and Rim101 genes was evaluated using q RT-PCR. Here, fresh culture colonies of C. albicans (103 cells/ml) were counted and treated with a 300 µM concentration of farnesol and nanogels containing farnesol and incubated for 24 h at 37 °C. After this time, yeast cells were harvested and washed with PBS, then, total RNA from treated and non-treated cells was extracted using glass bead and lysis buffer as described previously [Citation28,Citation29]. Then, cDNA was synthesized using vivantis kit (Subang Jaya, Malaysia) as recommended by the manufacturer’s protocol. Real-time PCR was accomplished using AMPLIQON (Real Q plus 2 × master mixes Green High Rox, Sinnacolon Co., Tehran, Iran). In order to analyze PCR performance, the mixture containing 12.5 µl of master mix (Green High Rox), 0.5 µl of each specific primer pmol and 2 µl of cDNA template (10 ng) were adjusted to a final volume of 25 µl using DEPC water [Citation30]. The PCR condition was started at 95 °C for 5 min, followed by 40 cycles of 95 °C for 15 s, 58 °C for 30 s and 72 °C for 30 s. All steps were done in the ABI one step (Applied Biosystems, Rotkreus, Switzerland). The specific primers for HWP1, SAP6 and Rim101 were designed by using Allele ID primer design software (version 7.5) (). The ACT1 gene was used as housekeeping gene for normalization of the RT-PCR data. All the experiments were carried out in duplicates.
Table 1. Primer sequences of genes in quantitative real-time PCR assay.
Statistical analysis
MTT test was analyzed using one-way analysis of variance (ANOVA) and t-tests using SPSS software version 20 (SPSS, Chicago, IL). The mean of the Ct of studies genes before and after treatment by farnesol and nanogel is expressed as a mean ± standard deviation. The levels of ΔCt of the genes before and after treatment with farnesol and nanogel were compared using paired t-test, p value of ≤.05 was considered to be statistically significant. The expression of genes in RT-PCR was analyzed using REST 2009 software (Ver. 2.0.13, Qiagen, Hilden, Germany).
Results
SEM imaging
Morphology structure of CS and AL nanogel containing farnesol was assessed by SEM analysis. As shown in Figure 1(A,B), farnesol loaded in CS nanogel has spherical surface morphology and particle size was 43.5–54.6 nm. Also, AL nanogel showed the spherical surfaces and the particle size was 42.1–70.2 nm.
FT-IR analysis
The FT-IR analysis was carried out to know about the complex formation of major functional groups involved in the loaded of farnesol in CS and AL nanogels. In the CS nanogel loaded farnesol spectrum (), the peaks at 3440 cm−1 show the stretching frequency links of N–H and O–H in CS. Peak at 2945 cm−1 will appear weak and in wide area; the resulting vibration frequency and asymmetric cross-linking in the polymer is aliphatic C–H. Peak at 1700 cm−1 showed the presence of C–O groups. Peak near 1000–1100 is also related to the C–O bond.
Figure 1 Scanning electron microscopic image: (A) chitosan nanogel contain farnesol and (B) alginate nanogel contain farnesol.
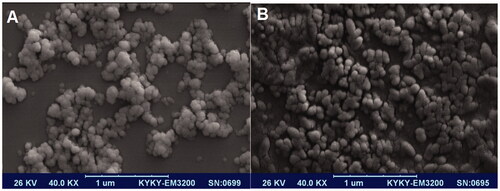
In the AL loaded farnesol spectrum (), the peak in the range of 3400–3443 cm−1 is related to the bonds N–H and O–H. Peaks at 2920 cm−1 are related to vibration frequency C–H carboxylate group in AL. The 1627 cm−1 peak present is related to vibration frequency C–C groups. The peak near 1000–1100 cm−1 is also related to the C–O band, it has been strengthened and all of vibrational bending of the functional groups C–H, CH2 and C–OH are changed in the conditions linked.
Power X-ray diffraction (XRD) spectra of nanogels
XRD technique was used to investigate the crystalline structure of CS, AL, CS containing farnesol and AL containing farnesol. The XRD spectra are as shown in . Comparison of the XRD pattern of CS and nano-gel containing farnesol showed no change in these two patterns. Also, there were no differences in the XRD patterns of the two nanogels, AL and AL-containing farnesol.
Encapsulation and release of farnesol from nanogels
The amount of farnesol fed was 0.5 g equal to 500 µl, the loading percentage for CS nanogel was 88% and for AL nanogel was 74%. The release of farnesol from nanogels was valued at pH 7. It was observed that at pH 7, approximately 58% of the farnesol loaded in CS nanogel was released, whereas when loaded in AL nanogel, 37% of farnesol was released in six days ().
Antifungal activity of nanogels
MIC value of farnesol was examined in our previous experiment. The rate of C. albicans growth was determined by measuring optical density (OD) at 360 nm. Our finding showed farnesol at 300 µM concentration strongly inhibited yeast cell growth ().
TABLE 2. Minimum inhibitory concentration 90 (MIC90) of farnesol on C. albicans (ATCC 10231) growth rate.
Antifungal activity of farnesol and nanogel containing farnesol was used by pure plate method. All experiments were performed in triplicate and mean and SD of value are added in . The finding of pure plate test revealed that at 300 µM concentration of farnesol, inhibition zone was detected at ∼7.0 mm, whereas the inhibition zone of CS nanogel was ∼9.0 mm. Indeed in nanogel AL, inhibition zone was not observed.
TABLE 3. Inhibitory zone of farnesol and nanogels contains farnesol in pure plat assay.
Cytotoxicity assay
Cytotoxicity effect of farnesol and nanogels against SW480 cells was examined using tetrazolium salt (MTT) assay. This experiment was performed in triplicate and the results are analyzed by IBM SPSS Statistics (Armonk, NY), the percentage of cells viability was estimated and the results are shown in . The finding showed that farnesol as well as nanogels has no significant cytotoxicity effects on SW480 cell lines as compared to untreated cells (p<.05). The viability of cells in CS nanogel treated cells was much more than AL nanogel and farnesol treatment.
Figure 6 Cytotoxicity effect of farnesol and farnesol-loaded nanogels on SW480 cells by MTT assay during 12, 24, 48 and 72 h treatment. Results indicated no differences significant cell viability per cent between each of treatment compared to control without treatment. *The difference between groups is statistically significant.
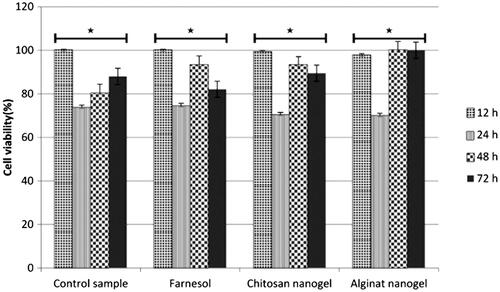
HWP1, SAP6 and Rim101 gene expression pattern in C. albicans
The effect of farnesol, CS and AL nanogels containing farnesol on the expression of HWP1, SAP6 and Rim101 genes was investigated using real-time PCR (). The finding shows that expression of HWP1 and SAP6 genes in C. albicans treated with 300 µM concentration of farnesol, CS and AL nanogels containing farnesol decreased significantly in comparison with un-treated control group (p<.01). However, it was found the significant difference between the expression of SAP6 gene of C. albicans treated with CS nanogel and non-treated Candida was observed. The expression of Rim101 in Candida treated with CS nanogel significantly decreased as compared to the non-treated cells (p<.01), whereas, Rim101 C. albicans treated with farnesol and AL nanogel containing farnesol did not show any change in the gene expression level.
Figure 7 Comparison of gene expression pattern of HWP1, SAP6 and Rim101 of C. albicans compared with ACT1 gene (housekeeping gene) before and after treatment with farnesol and nanogels containing farnesol. Changes in gene expression of HWP1, SAP6 and Rim101 are presented, compared to treatment with farnesol and nanogel. *The difference between groups is statistically significant.
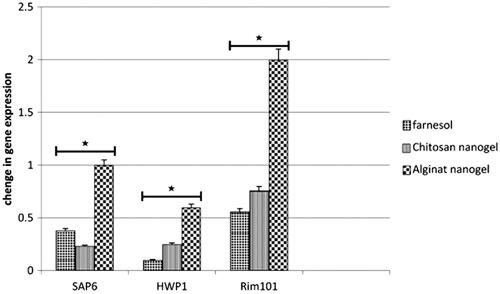
Discussion
C. albicans hyphae formation is the main factor associated with persistent infection. Hyphae form of C. albicans in biofilm architecture plays an important role in the invasion and pathogenesis of Candida species [Citation15]. Farnesol as a QSM is secreted from Candida during biofilm formation and inhibits hyphae formation and biofilm development. Ramage et al. [Citation7] have shown that 300 µM concentration of farnesol had inhibition effect on biofilm formation of C. albicans at 24 h exposure. Mosel et al. [Citation31] have shown that 250 µM farnesol prevented the germ tube formation and suppressed filamentation of Candida in biofilm formation. In the previous study, it has been shown that farnesol in 300 µM concentration can inhibit growth and proliferation of C. albicans. In addition, this QS molecule increases microbial sensitivity to antibiotics. Interaction between farnesol and different antifungal (fluconazole, amphotericin B and micafingin) exerts a synergistic or additive interaction against C. albicans biofilm. The farnesol inhibitory effects can be used as new antifungal agent and hyphal formation of C. albicans is a target for designing natural-based antifungal agents [Citation32,Citation33].
However, farnesol has poor physical properties such as hydrophobic and volatile that make the compound difficult to be used in pharmaceutical [Citation34]. These disadvantages can be conquered to an extent by nanocarrier consisting of polymers with natural based. At present, nanocarrier system was widely used in drug delivery [Citation35]. Nanocarrier system helps in improving bio-distribution of drugs and solubility of hydrophobic compounds, as well as increasing the bioavailability and reducing the toxicity [Citation36]. Nanocarrier technology is a new approach to encapsulation of bioactive compounds such as farnesol to improve the feasible and efficiency of therapeutic. Nanocarrier can be structured by a great range of various material and designs, as natural biomaterials; polysaccharides such as CS and AL are stable, safe, nontoxic, hydrophilic and biodegradable [Citation37].
Today, there are interesting nanocarriers that load natural compounds. Natrajan et al. [Citation25] synthetized alginate–chitosan (CS–AL) as polyelectrolyte complex nanocarrier for the encapsulation of essential oils. Chopra et al. [Citation38] used AL/gum acacia bipolymeric nano-hydrogels as carrier for zinc oxide nanoparticle. In the other study by Emmanuelle et al. [Citation39], they used the AL polymer and Whey protein for oral insulin. Their study showed that the rate of absorption of insulin via intestine epithelial cells increased and protected insulin bioavailability.
With this highlight, for the first time, nanogel was designed and synthesized using natural polymers of CS and AL for loading farnesol in 300 µM concentration. Then, for characteristics of these two nanogels as particle size, farnesol was released from nanogels; growth inhibitory effect and toxicity on the epithelial cell line were investigated and compared.
The finding of SEM image showed that the nanogels have spherical surface morphology and the mean size of CS nanogel was found to be from 43.5 to 54.6 nm and the main size of AL nanogel was obtained in the range 42.1–70.2 nm. Hosseini et al. [Citation40] evaluated CS nanoparticles for encapsulation of oregano essential oil. The obtained nanoparticles exhibited spherical shape, regular distribution and size range of 40–80 nm. de Oliveira et al. [Citation41] have shown that AL/cashew gum has shown nanoparticles size to be in the range of 223–399 nm. The present results showed the successful synthesis.
Assessment of farnesol release rate showed that amount of farnesol loaded in CS nanogel (88%) in six days at pH 7 was released at 58%, whereas, about 37% of farnesol loaded (74%) was released from AL nanogel at pH 7 at the same time. This suggested that the farnesol release of CS nanogel is more than AL nanogel in the same time and pH. As regards the inhibitory effect evaluation, the inhibitory zone of CS nanogel was detected, whereas AL nanogel was not observed. This phenomenon can be related to rate of farnesol released from each of nanogel.
As previously stated, carbohydrate polymer is nontoxic. In this context, cytotoxicity effects of CS and AL nanogel containing farnesol and also farnesol in 300 µM concentration on epithelial cell line SW480 were performed. No significant different was found between experimental groups (nano-gels containing farnesol and farnesol) and control group. In addition, the bioavailability of cells treated with CS nanogel was more than AL nanogel and control group. It is possible that the encapsulation of farnesol decreased its toxicity. The present data showed that the CS nanogel may be a more sufficient compound for pharmaceutical application as compared to AL nanogel. Natrajan et al. [Citation25] indicated that nontoxic nature of nanocarrier (CS–AL) was confirmed with viability of treated cells, which was about 98%.
This study was conducted to investigate and compare the effect of CS and AL nanogel containing farnesol and farnesol pure on the genes expression related to hyphae morphogenesis. Germ tube and hyphae formation were controlled by the network of transcription genes and their targets. It has been well known that SAP6 and HWP1 are the critical genes associated with transcription gene network expressed in germ tube and hyphal form of C. albicans [Citation15]. Hence, transcription factor Rim101 regulated genes are responsible for pH extracellular [Citation16].
Since the role of these genes can be considered in hyphae formation of Candida, therefore, the effect of farnesol and nanogels containing farnesol was assessed on SAP6, HWP1 and Rim101 genes. Quantitative real-time assay revealed the exposure of C. albicans with farnesol at 300 µM concentration and both nanogels significantly decreased the expression of SAP6 and HWP1 genes as compared to untreated Candida (p<.01). Therefore, considering the aforementioned results, the inhibitory effect of farnesol on hyphae and germ tube formation, showed the inhibition of expression of genes related morphogenesis. So far, no more information was declared on the effect of farnesol on the genes which regulate hyphae formation. The finding of the present study is consistent with Nadège Décanis et al. [Citation42] report, which showed that farnesol reduces the expression of SAP4-6 genes significantly. Exposure of yeasts with AL nanogel did not show any change in Rim101 gene expression, in the treatment of C. albicans with AL nanogel containing farnesol; however, in contrast to CS nanogel, it down-regulated the Rim101 expression. The obtained results showed that formulated CS nanogel loaded farnesol improved the inhibitory effect of farnesol against C. albicans and down-regulated SAP6 and Rim101 genes expression as the major gene regulation hyphae morphogenesis.
Conclusions
According to the obtained finding, CS nanogel with nanoscale molecule is the appropriate candidate as well as useful carrier of farnesol with minimum cytotoxicity against Candida infection for future biomedical and pharmaceutical approach. Notably, farnesol and CS nanogel inhibit the key genes contributing to hyphal formation through down-regulation of SAP6 and HWP1 genes. Finally, it has been concluded nanocarrier drug delivery strategy can be a suitable approach for improvement of anti-fungal properties of farnesol.
Acknowledgements
The staff of the Department of Medical Mycology, Faculty of Medical Sciences, Tarbiat Modares University, Tehran, Iran are appreciated for their collaboration.
Disclosure statement
No potential conflict of interest was reported by the authors.
References
- Vandeput P, Selene F, Coste AT. Antifungal resistance and new strategies to control fungal infections. Int J Microbiol. 2012;26:Article ID 713687, 26 pages. http://dx.doi.org/10.1155/2012/713687.
- Hill JA, Ammar R, Torti D, et al. Genetic and genomic architecture of the evolution of resistance to antifungal drug combinations. PLOS Genet. 2013;9:e1003390.
- Perea S, Lopez-Ribot JL, Kirkpatrick WR. Prevalence of molecular mechanisms of resistance to azole antifungal agents in Candida albicans strains displaying high-level fluconazole resistance isolated from human immunodeficiency virus-infected patients. Antimicrob Agents Chemother. 2001;45:2676.
- Zhang H, Zhai Y, Wang J, et al. New progress and prospects: the application of nanogel in drug delivery. Mater Sci Eng C Mater Biol Appl. 2016;60:560–568.
- Tiwari BK, Valdramidis VP, O'Donnell CP, et al. Application of natural antimicrobials for food preservation. J Agric Food Chem. 2009;57:5987–6000.
- Adorjan B, Buchbauer G. Biological properties of essential oils: an updated review. Flavour Fragr J. 2010;25:407–426.
- Ramage G, Saville SP, Wickes BL, et al. Inhibition of Candida albicans biofilm formation by farnesol, a quorum-sensing molecule. Appl Environ Microbiol. 2002;68:5459–5463.
- Joo JH, Jetten AM. Molecular mechanisms involved in farnesol-induced apoptosis. Cancer Lett. 2010;287:123–135.
- Kromidas L, Perrier E, Flanagan J, et al. Release of antimicrobial actives from microcapsules by the action of axillary bacteria. Int J Cosmet Sci. 2006;28:103–108.
- Saville SP, Lazzell AL, Bryant AP, et al. Inhibition of filamentation can be used to treat disseminated candidiasis. Antimicrob Agents Chemother. 2006;50:3312–3316.
- Shareck J, Belhumeur P. Modulation of morphogenesis in Candida albicans by various small molecules. Eukaryotic Cell. 2011;10:1004–1012.
- Hornby JM, Kebaara BW, Nickerson KW. Farnesol biosynthesis in Candida albicans: cellular response to sterol inhibition by zaragozic acid B. Antimicrob Agents Chemother. 2003;47:2366–2369.
- Lu Y, Su C, Unoje O, et al. Quorum sensing controls hyphal initiation in Candida albicans through Ubr1 –mediated protein degradation. Proc Natl Acad Sci USA. 2014;111:1975–1980.
- Mark E, Shirtliff BPK, Roelien AM, et al. Farnesol-induced apoptosis in Candida albicans. Antimicrob Agents Chemother. 2009;53(6):2392–2401.
- Wachtler BWD, Haedicke K, Dalle F, et al. From attachment to damage: defined genes of Candida albicans mediate adhesion, invasion and damage during interaction with oral epithelial cells. PLoS One. 2011;6:e17046.
- Nobile CJNS, Myers CL, Fay AJ, et al. Candida albicans transcription Rim101 mediates pathogenic interactions through cell wall functions. Cell Microbiol. 2008;10:2180–2196.
- Jacobsen ID, Wilson D, Wächtler B, et al. Candida albicans dimorphism as a therapeutic target. Expert Rev anti Infect. 2012;10:85–93.
- Fateme N, Shahla R, Bahareh B, et al. Effect of farnesol on responsive gene expressions in hyphal morphogenesis transformation of Candida albicans. Infect Epedemiol Microbiol. 2018;4:73–77.
- Bilia AR, Guccione B, Isachi C, et al. Essential oils loaded in nanosystems: a developing strategy for a successful therapeutic approach. Evid Based Complement Alternat Med. 2014. Article ID 651593, 14 pages. DOI:10.1155/2014/651593.
- Liu Z, Jiao Y, Wang Y, et al. Polysaccharides-based nanoparticles as drug delivery systems. Adv Drug Deliv Rev. 2008;60:1650–1662.
- Liew CV, Chan LW, Ching AL, et al. Evaluation of sodium alginate as drug release modifier in matrix tablets. Int J Pharm. 2006;309:25–37.
- Rampino A, Borgogna M, Blasi P, et al. Chitosan nanoparticles: preparation, size evolution and stability. Int J Pharm. 2013;455:219–228.
- Chen X-G, Lee CM, Park H-J. O/W emulsification for the self-aggregation and nanoparticle formation of linoleic acid-modified chitosan in the aqueous system. J Agric Food Chem. 2003;51:3135–3139.
- Ziaee M, Moharramipour S, Mohsenifar A. MA-chitosan nanogel loaded with Cuminum cyminum essential oil for efficient management of two stored product beetle pests. J Pest Sci. 2014;87:691–699.
- Natrajan D, Srinivasan S, Sundar K, et al. Formulation of essential oil-loaded chitosan-alginate nanocapsules. J Food Drug Anal. 2015;23:560–568.
- Yahya MZA, Harun MK, Ali AMM, et al. XRD and surface morphology studies on chitosan-based film electrolytes. J Appl Sci. 2006;6:3150–3154.
- Magaldi S, Mata-Essayag S, Hartung de Capriles C, et al. Well diffusion for antifungal susceptibility testing. Int J Infect Dis. 2004;8:39–45.
- Nikoomanesh F, Roudbarmohammadi S, Roudbary M, et al. Investigation of bcr1 Gene Expression in Candida albicans isolates by RT PCR technique and its impact on biofilm formation. IEM. 2016;2:22–24.
- Maryam RSR, Bita B, Zahra F, et al. Identification of Candida species isolated form Iranian women with vaginal candidasis by PCR-RFLP method. Eur J Exp Biol. 2013;3:365–369.
- Rossoni RD, de Barros PP, de Alvarenga JA, et al. Antifungal activity of clinical Lactobacillus strains against Candida albicans biofilms: identification of potential probiotic candidates to prevent oral candidiasis. Biofouling. 2018;34:212–225.
- Mosel DD, Dumitru R, Hornby JM, et al. Farnesol concentrations required to block germ tube formation in Candida albicans in the presence and absence of serum. Appl Environ Microbiol. 2005;71:4938–4940.
- Katragkou A, McCarthy M, Alexander EL, et al. In vitro interactions between farnesol and fluconazole, amphotericin B or micafungin against Candida albicans biofilms. J Antimicrob Chemother. 2015;70:470–478.
- Xia FQ J, Xu W, Zhang Z, et al. In vitro inhibitory effects of farnesol and interactions between farnesol and antifungals against biofilms of Candida albicans resistant strains. Biofouling. 2017;33:283–293.
- Zhu CLWX, Lin ZZ, Xie ZH, et al. Cell microenvironment stimuli-responsive controlled-release delivery systems based on mesoporous silica nanoparticles. J Food Drug Anal. 2014;22:18–28.
- Dugal S, Fernandes A. Formulation and evaluation of a novel mucoadhesive drug delivery system to treat intestinal candidiasis in immunocompromised patients. Res Pharm. 2011;4:10–16.
- Yadav AGM, Jain DK. Nano-medicine based drug delivery system. Pharm Technol Res. 2011;1:13.
- Raemdonck K, Demeester J, De Smedt S. Advanced nanogel engineering for drug delivery. Soft Matter. 2009;5:707–715.
- Chopra M, Bernela M, Kaur P, et al. Alginate/gum acacia bipolymeric nanohydrogels-promising carrier for zinc oxide nanoparticles. Int J Biol Macromol. 2015;72:827–833.
- Deat-Laine E, Hoffart V, Garrait G, et al. Efficacy of mucoadhesive hydrogel microparticles of whey protein and alginate for oral insulin delivery. Pharm Res. 2013;30:721–734.
- Hosseini SF, Zandi M, Rezaei M, et al. Two-step method for encapsulation of oregano essential oil in chitosan nanoparticles: preparation, characterization and in vitro release study. Carbohydr Polym. 2013;95:50–56.
- de Oliveira EF, Paula HCB, de Paula RCM. Alginate/cashew gum nanoparticles for essential oil encapsulation. Colloids Surf B Biointerfaces. 2014;113:146–151.
- Nadège Décanis NT, Alexandra C, Manuel V, et al. Farnesol, a fungal quorum-sensing molecule triggers Candida albicans morphological changes by downregulating the expression of different secreted aspartyl proteinase genes. Open Microbiol J. 2011;5:119–126.