Abstract
Although titanium implants account for a large proportion of the commercial dental market, their bioactivity are inadequate in many applications. A micro- and nano- scale hierarchical surface topography of the implant is suggested for rapid osseointegration from the biomimetic perspective. Moreover, Zinc (Zn) is an essential element in the skeletal system. Thus, a micro/nanostructured TiO2/ZnO coating, produced by micro-arc oxidation, and hydrothermal treatment, and heat treatment, was designed to endow the implant surface with enhanced osteogenic capacity. Physiochemical properties and biological effects of this coating were investigated in our study. The annealed micro/nanostructured TiO2/ZnO coating exhibited higher hydrophilicity and fibronectin adsorption ability compared to the micro-arc oxidation modified TiO2 coating. SaOS-2 cells grown on the annealed micro/nanostructured TiO2/ZnO coating showed increased alkaline phosphatase activity and collagen secretion, and immunofluorescence labeling revealed an upregulation of osteopontin, collagen type ι and osteocalcin. The micro/nanostructure and incorporation of Zn were considered to perform positive effect on the enhanced osteogenic activity of SaOS-2 cells. In conclusion, the micro/nanostructured TiO2/ZnO structure is simple, stable, and easy to produce and scale up, has promising applications in the surface modification of titanium implants.
Introduction
Implant materials need to be tailored to possess both excellent biocompatibility and osteogenic ability. Surface topography is reported to play important roles in mediating the interactions between bone cells and implant, and would eventually influence the osseointegration [Citation1]. Although many studies have demonstrated the important role of the surface structure of titanium in bone regeneration, only limited studies on the controllable fabrication of micro/nanostructure have been reported so far [Citation2,Citation3]. Generally, microscale topography is produced in the current commonly applied implants by various methods [Citation4,Citation5]: machining, acid-etching, grit-blasting, and titanium plasma spraying. It is reported that microstructure surface is beneficial to promote bone-to-implant contact [Citation6]. Nanostructures are also produced on the implant to ensure better adhesion of cells to the implant surface and sufficient stimulation of bone formation [Citation7]. Nanostructure surface can directly interact with some proteins and cell membrane receptors, effectively enhancing cell functions [Citation8]. Micro/nano hierarchical topography on implant materials bear a closer resemblance to the features of the natural extracellular matrix, and may be biologically friendly in multiple cell behaviors [Citation9]. Recently, numerous studies regarding the positive osteogenic effects of hierarchical micro/nanostructure surfaces have been reported [Citation10]. For example, micro/nanostructured bioceramics display the ability to promote the differentiation of human periodontal ligament stem cell but remain a technological challenge, brittleness [Citation11].
Surface engineering approaches that alter the topological chemistry of a substrate could also be employed as an effective tool for directing cell interactions and their subsequent function. In previous studies, various nutrition elements (e.g. Ca, Mg, Zn, and Sr) have been incorporated into biomaterial surface to promote implant osseointegration and shorten the bone healing period. Among these elements, Zn is a trace element in the human body that is second only to iron and essential for the development of skeleton [Citation12]. Zn is also abundant in plasma and bone [Citation13]. Zn participates in bone metabolism, including osteogenesis and bone resorption, and has an effect on both osteoblasts and osteoclasts [Citation14]. The lack of Zn in the human body affects the normal development of bone tissue and may lead to osteoporosis [Citation15]. Oral supplementation of Zn has a positive effect on bone metabolism [Citation16,Citation17]. Zn-containing materials can regulate bone formation by releasing Zn2+ ions. The role of endogenous Zn in protein synthesis in the bone tissues of newborn rats is investigated in previous study [Citation18]. Studies have shown that Zn can increase the expression of osteogenic related genes, and the promotion is significantly concentration-dependent. Zn at low concentration promotes osteogenesis while Zn at high concentration may be toxic [Citation19]. Therefore, controlling the content and release of Zn is pivotal for its application in orthopedic materials.
In the previous study, we developed a novel micro/nanostructured TiO2/ZnO coating for Ti implant surfaces and then employed a simple but effective method to achieve a balance between antibacterial activity and cytocompatibility [Citation20]. The aim of this study was to investigate further cell behavior on the coating, including attachment and differentiation of SaOS-2 osteoblast-like cells on the micro/nanostructured TiO2/ZnO coating to determine whether this material was suitable for clinical applications.
Materials and methods
Preparation and characterization of TiO2/ZnO coating
Titanium Samples (commercially pure titanium Grade 2) with a diameter of 14.5 mm and thickness of 1 mm were polished and then ultrasonically cleaned in acetone, ethanol and deionized water successively, each for 5 min. The micro-arc oxidation (MAO) treatment of the Ti discs was carried out under a pulsed 250 V in positive and 5 V in negative AC field (WHD-20, Harbin, China). 0.1 M calcium acetate monohydrate (Ca(CH3COO)2·H2O), 0.1 M disodium edetate dihydrate (Na2(EDTA)), 0.25 M sodium hydroxide (NaOH), and 0.02 M sodium silicate (Na2SiO3·9H2O) were added into deionized water as electrolyte solution. The duration, frequency and duty of the pulsed AC power were 5 min, 50 Hz, 50%, respectively. Circulating water was employed in the cooling system. After the MAO process, samples were ultrasonically cleaned sequentially in acetone, ethanol and deionized water, each for 5 min. The coating treated with MAO was referred as MAO group. Subsequently, the samples underwent a hydrothermal treatment in 8 ml ammonium hydroxide containing 0.02 M zinc acetate (Zn(CH3COO)2), with a pH value of 12.6, at 200 °C for 4 h. After then, the samples underwent a heat treatment at 450 °C for 3 h following at 700 °C for 3 h. And then an ultrasonic treatment was applied in deionized water for 5 min, which removed the loosely adhered ZnO on the coating (referred as MHTZn group).
Surface morphology were observed by field-emission scanning electron microscopy (SEM; Zeiss, Oberkochen, Germany). Contact angles of water droplets were measured with a contact angle meter (JC2000C1; Powereach, Shanghai, China).
Fibronectin adsorption
Rhodamine-conjugated fibronectin (Sigma; St. Louis, MO, USA) was used for the protein adsorption test. Samples were immersed in 8 μg/ml fibronectin dissolved by Dulbecco's phosphate-buffered saline (DPBS; Welgene, Daegu, Korea) for 4 h at 37 °C. The amount of adsorbed fibronectin was determined from images acquired by fluorescence microscopy (Leica, Wetzlar, Germany) via transforming the fluorescence into an optical density (OD) value using ImageJ2X software.
Evaluation of cellular responses
SaOS-2 osteoblast-like cells were seeded on samples at 3 × 104 per well for adhesion and at 1 × 104 per well for cell differentiation assays. McCoy’s 5 A medium (Gibco, Grand Island, NY, USA) supplemented with 10% fetal bovine serum (Gibco) and 1% penicillin/streptomycin (Gibco; referred to as complete medium) was refreshed every 2 days.
At 4 and 24 h, attached SaOS-2 cells cultured on various coatings were fixed with 4% paraformaldehyde for 30 min and then washed three times with DPBS. Nuclei and cytoskeletal filamentous F-actin in the cells was stained with 4'-6-diamidino-2-phenylindole (DAPI; Sigma) at room temperature for 15 min and with 5 µg/ml phalloidin-tetramethylrhodamine (TRITC; Sigma) at 37 °C for 40 min. Samples were imaged with a confocal laser scanning microscope (Zeiss 710; Zeiss).
Cell Morphologies at 4 and 24 h were also observed under the SEM. Samples were fixed with 2.5% glutaraldehyde at 4 °C overnight. After then, samples were dehydrated by gradient concentration ethanol in pure water (30%, 50%, 70%, 75%, 80%, 85%, 90%, 95%, 100% [v/v]) and tertiary butanol in ethanol (25%, 50%, 75%, 100% [v/v]), each for 10 min. Subsequently, samples underwent a freeze drying process before the observation.
Alkaline phosphatase (ALP) activity at 7 days was measured with an ALP testing kit (Jiancheng Bio-engineering Research Institute of Nanjing, Nanjing, China) and normalized by total protein content, as determined with the Bicinchoninic Acid Assay kit (Beyotime Institute of Biotechnology, Shanghai, China). Collagen secretion was detected by Sirius Red staining after 7 days; images were then taken under a fluorescence microscopy; the dye was dissolved with 0.1 M NaOH/methanol (1:1 v/v) and OD values were determined. Expression of the bone-specific extracellular matrix proteins osteopontin (OPN), collagen type ι (Col-ι), and osteocalcin (OCN) in osteoprogenitors in response to the coatings was evaluated by immunofluorescence analysis. Samples were fixed with 4% paraformaldehyde for 30 min and permeabilized with 0.3% Triton X-100. After blocking with 10% goat serum solution at room temperature for 2 h, samples were incubated with rabbit polyclonal antibodies against OPN and Col-ι and rat polyclonal antibody against OCN (all from Abcam, Cambridge, MA, USA). The cells were then labelled with fluorescein isothiocyanate-conjugated goat anti-rabbit IgG and TRITC conjugated goat anti-rat IgG (both from Sigma). Cell nuclei were stained with DAPI for 30 min at room temperature, and samples were imaged by confocal laser scanning microscopy.
Statistic analysis
Three replicate samples from each group were used in the experiments and the results were expressed as "mean ± standard deviation". Significant differences were calculated using Student’s t test. *p < .05 and **p < .01 were considered statistically significant and highly significant differences between the data.
Results
Morphology of micro/nanostructured TiO2/ZnO coating
showed homogeneous distributed pores ranging from 500 nm to 3 μm which were similar for the MAO and MHTZn surfaces at the microscale. In the further magnified images of MAO and MHTZn surfaces (), nanostructures on the MHTZn surface structure were visible. The MAO coating showed smooth surface in while the MHTZn coating presented granular nanostructure in . The hydrothermal treatment and heat treatment produced nanostructures on the micro-arc oxidation modified surface. The investigation on the properties of the MAO and MHTZn coatings was performed in our previous study [Citation20]. The results suggested that the micro-arc oxidation produced an oxidation layer consisting of anatase and rutile, and the nanostructures formed after hydrothermal treatment and heat treatment were composed of ZnO.
Wettability
The wettability of the two coatings was evaluated by the static contact angle measurement method and displayed in . Surface contact angles of MAO and MHTZn coatings were <90°, indicating that both of the coatings were hydrophilic. The MHTZn coating exhibited higher hydrophilicity than the MAO coating since the contact angle of the MHTZn coating was smaller. The results showed that the introduction of nanostructures could influence the wettability of the materials.
Protein adsorption
Hydrophilicity has an important effect on the adsorption of protein. Fibronectin tends to adsorb on the hydrophilic surface. The amount of adsorbed fibronectin was proportional to the fluorescence intensity of the MAO and MHTZn two groups (), and the intensity was transferred as OD values shown in . Thus, the MHTZn coating exhibited stronger adsorption capacity for fibronectin.
Osteogenic activity
The initial adhesion of SaOS-2 cells on MAO and MHTZn was evaluated by cytoskeletal staining (. At 4 h, most cells flattened on both coatings; in addition, several cells were elongated and extended lamellipodia. At 24 h, cells on the two coatings extended more filopodia although more stress fibers were detected in cells grown on the MHTZn coating, suggesting that cell attachment was enhanced on this material. Cell adhesion was evaluated by counting cell number by staining nuclei with DAPI, as shown in . No difference in the numbers of cells attached to the MHTZn was observed as compared to the MAO coating at either 4 h or 24 h. The organization of cytoskeletal actin filaments was distinct and well-formed and filaments were parallel to each other.
Figure 4. Cytoskeletal and nuclear staining (a) and quantitative analysis (b) of SaOS-2 cells attached to MAO and MHTZn coatings at 4 and 24 h. Values represent mean ± SD (n = 3).
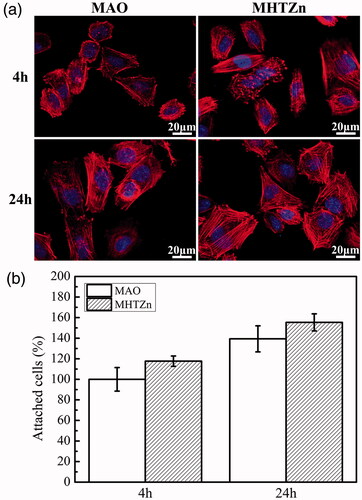
Attached cells were also observed under SEM. At 4 h, cells on the MAO and MHTZn coatings were flatten (). Ruffling membrane and small filopodia were visible on the edges of cells (Inserts of ). These filopodia acted as tentacles to sense the environment. After 24 h, cells extended and lamellipodia assembled (). More and longer filopodia were observed for the MHTZn group. In the insert of , filopodia of cells on the MAO coating tended to enter in the micropores, while in the insert of , filopodia of cells on the MHTZn coating anchored into the pores as well as the surrounding walls.
Figure 5. Morphologies of attached cells on the MAO at 4 h (a) and insert, 24 h (c) and insert; MHTZn at 4 h (b) and insert, 24 h (d) and insert.
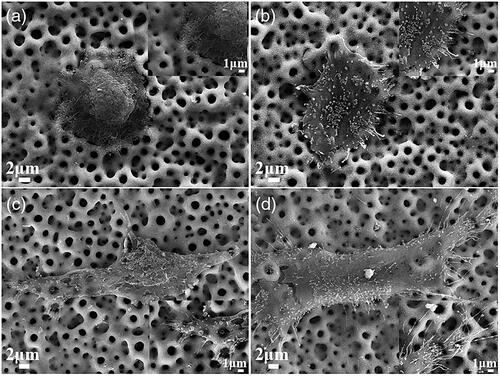
ALP is the most widely used early biochemical marker for osteoblastic activity and is essential for normal bone formation. ALP activity was observed to be higher in the MHTZn group as compared to the MAO group (). Collagen fibers are a major constituent of bone; collagen secretion by cells grown on the two coatings was evaluated with Sirius Red, which stained all types of collagen. The highest collagen secretion was detected on the MHTZn coating (). We also examined the expression of OPN, Col-ι, and OCN markers of terminal osteogenic differentiation by immunofluorescence labelling. Higher fluorescence intensity for all three proteins was observed on the MHTZn coating as compared to the MAO coating (), indicating that the former promoted osteogenesis to a greater degree.
Discussion
Our previous study had incorporated Zn into micro-arc oxidation for its antibiotic properties [Citation20]. Zn is also reported to modulate the expression of transcription factors related to bone resorption and remodeling [Citation21]. Released Zn2+ ions from MHTZn coating may act on Runx2 to directly stimulate bone formation via increased transcription of osteoblast differentiation genes. Zn deficiency leads to decreased Runx2 expression and downregulate of osteoblast-specific genes such as ALP, OPN, OCN, and Col-ι [Citation22]. Zn can activate the extracellular signal-regulated kinase ERK1/2 pathway [Citation23]; that is, cell spreading in conjunction with strong focal adhesion can induce ERK1/2 signaling and consequently, osteogenic differentiation. Other than the effect of Zn2+ ions on bone cells in vitro, the osteogenic capacity in vivo of Zn-containing materials has also been demonstrated. Zn-incorporated hydroxyapatite is reported to exhibit higher osseointegration rate compared to hydroxyapatite coatings used in induced osteoporosis in rats [Citation24]. The osseointegration of titanium implants by incorporating Zn in ceramic coatings is also improved [Citation25]. These studies indicate that MHTZn coating may promote osteogenic differentiation of cells by releasing Zn2+ ions with appropriate concentrations; and the incorporation of Zn2+ ions may increase the binding strength of titanium and titanium alloy implants to bone tissue in vivo.
To endow Ti implant with multifunction, modification of surface chemical properties alone is insufficient; the surface topography should also be taken into account [Citation26]. In our work, a combination of MAO, hydrothermal treatment, and calcination were used to incorporate Zn into the micro/nanostructured TiO2 coating. In addition to inhibit bacterial growth, Zn promoted new bone formation while micro/nanostructures mimic the hierarchical structure of natural bone and thereby altered the local microenvironments of cells. We proposed that the combination of Zn and micro/nanostructured topography influenced the surface properties of implants such as wettability and protein adsorption capacity, and consequently dictated their interactions with cells.
Besides the Zn2+ ions, the surface chemistry, morphology, and wettability of the coating together form the local microenvironments for cell growth and regulate the behavior of bone-related cells and bone tissue healing [Citation4]. Specific proteins in tissue fluids show higher affinity to hydrophilic surfaces than hydrophobic surfaces. The formation of ZnO nanostructure on the surface of MAO coating caused a change in surface wettability. The surface contact angle of the MHTZn coating was smaller than that of the MAO coating, bringing out higher hydrophilicity. Protein adsorption depends on the wettability of a surface; the adsorption of fibronectin, which plays a critical role in cell attachment, increases with higher surface wettability [Citation27]. Thus, the MHTZn coating exhibited stronger fibronectin adsorption ability. The adsorption of proteins on materials directly affected the attachment, proliferation and differentiation of cells [Citation28]. An abundance of absorbed proteins may provide more RGD sequences for increased interaction with special receptors on the cell membrane. Moreover, the microenvironment can modulate the formation of focal adhesions (FAs), and FA signaling transmits force stimuli to the intracellular matrix through the actin cytoskeleton. An increase in protein adsorption may be attributed to the high binding capacity of the micro/nanostructure topography; indeed, nanoscale topography of implant surfaces shows high affinity for extracellular proteins [Citation29]. This may be one of the reasons for the enhanced attachment of cells on the MHTZn coating surface. Cell adhesion and spreading on the implant surface is the first step in osseointegration, which determines the capacity of cells to proliferate and differentiate. In general, elongated cells with many stress fibers generally exhibit good focal adhesion. We observed that cell adhesion was grater on MHTZn than on the MAO surface. Osteoblast differentiation consists of proliferation, matrix maturation, and matrix mineralization stages that are accompanied by the expression of specific marker genes, which is regulated by the bone-specific transcription factor Runt-related homeobox (Runx2) [Citation30]. Huang et al. [Citation31] prepared a series of nanostructures on the surface of micro-arc oxidation coatings by hydrothermal method, and pointed out that the contact area of nanostructures with cells had an important influence on the attachment, proliferation, and differentiation behavior of cells, regulates the differentiation of cells into the osteogenic direction. The introduction of ZnO nanostructures may increase the effective contact area between the cells and the surface, thereby facilitating the expansion of the cells, triggering cytoskeleton reorganization and nuclear deformation, thus promoting DNA synthesis and further promoting cell differentiation. The surface cytoskeleton of the MHTZn coating was polygonal, forming a significant stress fiber, indicating that the cells transmitted mechanical signals into the cells. In addition, it has been reported that micro- and nanostructures have a synergistic effect on cell osteogenesis, and shows enhanced osteogenic activity than that of a single-scale structure [Citation32]. Thus, the micro-nanostructure of the MHTZn coating might produce an osteogenic effect that was superior to a single MAO coating. In conclusion, the microenvironment (including surface structure, released ions, etc.) formed by Zn-containing micro/nanostructures has a pronounced effect on the attachment and differentiation of osteoblasts.
We demonstrated that hydrothermal treatment was an effective method to incorporate Zn into the micro-arc oxidation surface to generate a hierarchical micro/nanostructure. After heat treatment, Zn-incorporated TiO2 coatings served as a stable system to maintain the sustained release of Zn2+ ions [20]. The Zn-incorporated TiO2 coatings described here not only exhibited antibacterial properties [20], but also induced osteogenic activity in osteoblast-like cells. These works highlighted their possibility for promoted implant osseointegration, although in vivo studies were required to further demonstrate the application in clinic.
Conclusions
A micro/nanostructured TiO2/ZnO coating was developed and its in vitro osteogenesis was assessed using osteoblast-like SaOS-2 cells. In vitro biological experiments demonstrated that the micro/nanostructured coating with bioactive Zn2+ ions had significantly promoted cell adhesion and differentiation. The improved osteogenic activity markers including ALP activity, collagen secretion, OPN, Col-ι, and OCN of SaOS-2 cells were attributed to the effects of the bioactive ions and micro/nanostructure. This study provides a promising option for the future surface design of Ti-based implants using surface bioactive Zn2+ ions and micro/nanostructure to enhance osseointegration.
Additional information
Funding
References
- Ogle OE. Implant surface material, design, and osseointegration. Den Clin. 2015;59:505.
- Zhang R, Elkhooly TA, Huang Q, et al. Effects of the hierarchical macro/mesoporous structure on the osteoblast-like cell response. J Biomed Mater Res A. 2018;106:1896.
- Zhang R, Elkhooly TA, Huang Q, et al. A dual-layer macro/mesoporous structured TiO2 surface improves the initial adhesion of osteoblast-like cells. Mat Sci Eng C. 2017;78:443.
- Dohan Ehrenfest DM, Coelho PG, Kang B, et al. Classification of osseointegrated implant surfaces: materials, chemistry and topography. Trends Biotechnol. 2010;28:198.
- Kournetas N, Spintzyk S, Schweizer E, et al. Comparative evaluation of topographical data of dental implant surfaces applying optical interferometry and scanning electron microscopy. Dent Mater. 2017;33:e317.
- Fabbro MD, Taschieri S, Canciani E, et al. Osseointegration of titanium implants with different rough surfaces: a histologic and histomorphometric study in an adult minipig model. Implant Dent. 2017;26:357.
- Salou L, Hoornaert A, Louarn G, et al. Enhanced osseointegration of titanium implants with nanostructured surfaces: an experimental study in rabbits. Acta Biomater. 2015;11:494.
- Bacakova L, Filova E, Liskova J, et al. Chapter 4 – nanostructured materials as substrates for the adhesion, growth, and osteogenic differentiation of bone cells. In: Grumezescu AM, editor. Nanobiomaterials in Hard Tissue Engineering. New York: William Andrew Publishing, 2016. p. 103.
- Moon B, Kim S, Kim H, et al. Hierarchical micro-nano structured Ti6Al4V surface topography via two-step etching process for enhanced hydrophilicity and osteoblastic responses. Mat Sci Eng C. 2017;73:90.
- Xu J, Chen X, Zhang C, et al. Improved bioactivity of selective laser melting titanium: surface modification with micro-/nano-textured hierarchical topography and bone regeneration performance evaluation. Mat Sci Eng C. 2016;68:229.
- Mao LX, Liu J, Zhao J, et al. Effect of micro-nano-hybrid structured hydroxyapatite bioceramics on osteogenic and cementogenic differentiation of human periodontal ligament stem cell via Wnt signaling pathway. Int J Nanomed. 2015;10:7031.
- Chasapis CT, Loutsidou AC, Spiliopoulou CA, et al. Zinc and human health: an update. Arch Toxicol. 2012;86:521.
- Rink L, Gabriel P. Zinc and the immune system. Proc Nutr Soc. 2000;59:541.
- Yamaguchi M, Weitzmann MN. Zinc stimulates osteoblastogenesis and suppresses osteoclastogenesis by antagonizing NF-κB activation. Mol Cell Biochem. 2011;355:179.
- Yamaguchi M. Role of nutritional zinc in the prevention of osteoporosis. Mol Cell Biochem. 2010;338:241.
- Hadley KB, Newman SM, Hunt JR. Dietary zinc reduces osteoclast resorption activities and increases markers of osteoblast differentiation, matrix maturation, and mineralization in the long bones of growing rats. J Nutr Biochem. 2010;21:297.
- Prasad AS. Zinc is an antioxidant and anti-inflammatory agent: its role in human health. Front Nutr. 2014;1:14.
- Wang G, Wan Y, Ren B, et al. Surface Functionalization of micro/nanostructured titanium with bioactive ions to regulate the behaviors of murine osteoblasts. Adv Eng Mater. 2017;19:1700299.
- Yusa K, Yamamoto O, Fukuda M, et al. In vitro prominent bone regeneration by release zinc ion from Zn-modified implant. Biochem Biophys Res Commun. 2011;412:273.
- Zhang R, Liu X, Xiong Z, et al. Novel micro/nanostructured TiO2/ZnO coating with antibacterial capacity and cytocompatibility. Ceram Int. 2018;44:9711.
- Holloway WR, Collier FM, Herbst RE, et al. Osteoblast-mediated effects of zinc on isolated rat osteoclasts: inhibition of bone resorption and enhancement of osteoclast number. Bone. 1996;19:137.
- Kwun I, Cho Y, Lomeda RR, et al. Zinc deficiency suppresses matrix mineralization and retards osteogenesis transiently with catch-up possibly through Runx 2 modulation. Bone. 2010;46:732.
- Huo K, Zhang X, Wang H, et al. Osteogenic activity and antibacterial effects on titanium surfaces modified with Zn-incorporated nanotube arrays. Biomaterials. 2013;34:3467.
- Li X, Li Y, Peng S, et al. Effect of zinc ions on improving implant fixation in osteoporotic bone. Connect Tissue Res. 2013;54:290.
- Yu J, Li K, Zheng X, et al. In vitro and in vivo evaluation of zinc-modified Ca-Si-based ceramic coating for bone implants. Plos One. 2013;8:e57564.
- Cloutier M, Mantovani D, Rosei F. Antibacterial coatings: challenges, perspectives, and opportunities. Trends Biotechnol. 2015;33:637.
- Wei J, Igarashi T, Okumori N, et al. Influence of surface wettability on competitive protein adsorption and initial attachment of osteoblasts. Biomed Mater. 2009;4:45002.
- Kopf BS, Ruch S, Berner S, et al. The role of nanostructures and hydrophilicity in osseointegration: in-vitro protein-adsorption and blood-interaction studies. J Biomed Mater Res. 2015;103:2661.
- Roach P, Farrar D, Perry CC. Surface tailoring for controlled protein adsorption: effect of topography at the nanometer scale and chemistry. J Am Chem Soc. 2006;128:3939.
- Komori T. Regulation of bone development and extracellular matrix protein genes by RUNX2. Cell Tissue Res. 2010;339:189.
- Huang Q, Elkhooly TA, Liu X, et al. Effects of hierarchical micro/nano-topographies on the morphology, proliferation and differentiation of osteoblast-like cells. Colloids Surf B Biointerfaces. 2016;145:37.
- Han G, Müller WE, Wang X, et al. Porous titania surfaces on titanium with hierarchical macro-and mesoporosities for enhancing cell adhesion, proliferation and mineralization. Mat Sci Eng C. 2015;47:376.