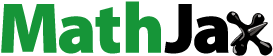
Abstract
Capecitabine (CAP) is an FDA-approved and frequently used chemotherapeutic agent for the treatment of various cancers. However, there are some side effects and chemoresistance limiting its use. Nanotechnological approaches can enhance the efficacy of anticancer drugs. In this study, CAP-loaded nanoniosomes were prepared. Nanoniosomes were prepared by the method of thin film hydration wherein CAP was loaded into the nanoniosomes. Nanoniosomes were then characterized by field emission scanning electron microscopy and (particle) vesicle size analysis. The cytotoxicity effect of the nanoniosomes were evaluated by the 3-(4,5-dimethylthiazol-2-yl)-2,5-diphenyltetrazolium bromide assay. CAP was loaded into the nanoniosomes and loading capacity and entrapment efficiency were determined. The vesicle size of the nanoniosomes was obtained in the nanometer scale, and CAP release profiles from the nanoniosomes were also obtained. Finally, the cytotoxicity effect of CAP and CAP-loaded nanoniosomes were evaluated toward MCF7 and PANC1 cell lines. The nanoniosomes with an amphipathic structure can penetrate into the cells with an enhanced release rate. These caused the toxicity of drug in the nanoniosomes to be higher than the free drug.
Introduction
The rate of occurrence of various cancers has had a steady increase in recent years worldwide [Citation1]. The aetiology of cancer involves smoking, poor diet, physical inactivity, environmental factors and reproductive changes [Citation2]. With increasing longer life expectancy, reports of occurrence of cancers have also increased. It is believed that tumour cells start developing resistance against chemotherapy at the onset of chemotherapy. Therefore, new approaches are required that can kill cancer cells in a way that control and reduce the progression and spreading of these cells in the body [Citation3–5].
Capecitabine (CAP) is an oral fluoropyrimidine carbonate with antineoplastic activity [Citation6] and exerts its effect as an anti-metabolite drug [Citation7]. It is a pro-drug of 5′-deoxy-5-fluorouridine (5′-DFUR) [Citation7] and is transformed to 5-fluorouridine (FU) after ingestion by thymidine phosphorylase present in the tumour tissue [Citation8]. CAP inhibits DNA synthesis thereby slowing down growth of tumour tissue [Citation9]. This drug provides a more convenient regimen than infusional FU with significantly less diarrhoea, stomatitis, nausea and alopecia [Citation10] while it produces side effects like myocardial infarction, angina, hand-foot syndrome, anaemia, thrombocytopenia and hyperbilirubinemia in the conventional dosage form [Citation9]. This can be overcome by delivering the drug to the tumour site by nanovehicles [Citation9].
Limiting factors in traditional drug development are toxicity of the therapeutic substances, low bioavailability and solubility, and so on causing efficacy limiting [Citation9,Citation11]. Developments in nanotechnology can offer novel approaches capable of delivering antineoplastic drugs to cancer cells [Citation5,Citation12]. Engineered nanostructures have been exhibit great potential to carry drugs to their desired site of action and therefore they may provide more effective methods of drug delivery for better treatment [Citation9]. In recent years, different kinds of nanomaterials such as nanotubes, nanorods, nanoparticles (NPs), dendrimers, liposomes and surfactant-based vesicles have offered advantages to target the drugs into various tumours due to small and specific structures leading to amplification of anticancer activity of the routine drugs [Citation13–17]. Such systems are characterized by unique features. Between them, nanoniosomes have been widely employed as drug delivery systems [Citation18]. Nanoniosomes are bilayer vesicles usually obtained by the self-assembly of cholesterol and non-ionic surfactants subsequent by hydration in an aqueous medium [Citation18]. They have the advantages of biodegradability, stability, bioavailability, biocompatibility, low cost and diversity of available surfactants for their design [Citation19–22]. A short summary of some anticancer drugs-affecting cell lines using nanoniosomes as nanovehicles is summarized in .
Table 1. Cytotoxicity effects of some anticancer drugs-loaded niosomes.
There have been some reports on the efficacy of CAP-loaded nanocarriers toward different cancer cell lines. Poly(lactic-co-glycolic acid) (PLGA) NPs-loaded CAP and dextran-spermine-loaded CAP were toxic against HepG2 and U87MG cell lines [Citation9]. The results showed an enhanced cell inhibiting activity, compared to the free drug. In another study [Citation40], CAP was loaded into the magnetic dextran-spermine NPs (DS-NPs). Physicochemical properties, in-vitro release profile and cellular studies of the optimized DS-NPs were evaluated. Drug release at neutral pH after 24 h and acidic pH within 3 h was 56% and 98%, respectively, with an entrapment efficiency of 26.1%. Cytotoxicity assessment exhibited that CAP-loaded DS-NPs was more toxic than the free drug. Pandey et al. [Citation41] prepared CAP-loaded PLGA NPs with a particle size of 179 nm, an entrapment efficiency of 71.3%, a drug release of 81.8% for 72 h, and enhanced cytotoxicity. Solid lipid nanoparticles (SLNs)-loaded palmityl pro-drug analogue of CAP was prepared to assess the toxicity of its colloidal form. The SLNs was evaluated for the toxicity against seven different cells including both normal and cancerous ones. IC50 values obtained for this formulation were 100 μM for the 4T1 and HH cells, and >200 μM (the highest concentration examined) for the other cells [Citation41]. In another study, gold NPs (GNPs) conjugated with CAP to treat liver cancer was synthesized. GNPs facilitated the cancer cells susceptibility to CAP [Citation42]. GNPs facilitated the cancer cells susceptibility to CAP [Citation5]. Payghan et al. have reported the preparation of CAP-loaded nanoerythrosomes by sonication method. The vesicles of the carriers were optimized for morphology, size, size distribution, integrity of membrane, release rate and bioavailability. CAP was targeted by loading on erythrocytes causing increment in the therapeutic index CAP targeting various organs [Citation43]. To the best of our knowledge, there has been no study on the preparation of CAP-loaded nanoniosomes. In the present study, for the first time, the results of niosomal carriers for CAP are reported. The nanoniosomes of CAP were prepared and characterized. Cytotoxicity assays in MCF7 and PANC1 cell lines were performed for comparison of cytotoxicity of CAP and its niosomal formulations.
Experimental section
Materials
CAP was received from Arasto Pharmaceutical Chemicals Inc. (Tehran, Iran). Dimethyl sulfoxide (DMSO), ethanol absolute, chloroform and acetonitrile (HPLC grade) were received from Scharlau (Barcelona, Spain). Span 20, Span 60, Span 65 and Span 80 were purchased from Daejung (Korea). Trypan blue and 3–(4,5-dimethylthiazol-2-yl)-2,5-diphenyltetrazolium bromide (MTT) were received from Sigma (St. Louis, MO, USA). Cholesterol was received from MedChem Express (Shanghai, China). MCF7 (NCBI C135) and PANC1 (NCBI C556) cell lines were prepared from Pasteur Institute Cell Bank (Tehran, Iran). For cell culture, Roswell Park Memorial Institute-1640 (RPMI 1640) was received from Nano Zist Arrayeh (Tehran, Iran). Fetal bovine serum (FBS) was purchased from Gibco (Grand Island, NY, USA). Penicillin-streptomycin solution was from Danesh Azma Cell (Tehran, Iran).
Preparation of nanoniosomes
Blank (b-Span 20, b-Span 60, b-Span 65 and b-Span 80) and CAP-loaded (CAP-Span 20, CAP-Span 60, CAP-Span 65 and CAP-Span 80) nanoniosomes were prepared using the surfactants of Span 20, 60, 65, or 80, and cholesterol. The nanoniosomes were prepared by the thin film hydration method. In a round-bottom flask, appropriate amounts of the non-ionic surfactants (9 mg of Span 20, 10 mg of Span 60, 20 mg of Span 65 or 10 mg Span 80), 10 mg of cholesterol with or without 10 mg of CAP with mole ratios of 1:1:1 were mixed. Then, the required amount of chloroform was added to the mixture to dissolve its contents. Chloroform was evaporated by a rotary evaporator at 60 °C. A thin layer of film was formed on the inner walls of the flask. The resultant film was hydrated by 10 ml of phosphate buffer saline (PBS, pH 7.4) for 30 min at 55 °C. The nanoniosome remained at room temperature for 24 h hydration completion. The nanoniosomes were finally sonicated in an ice bath ultrasound for 30 min to decrease the size of vesicles. Then the nanoniosomes were stored at 4 °C for further studies. The best formulation was selected based on the results of MTT assay. The blank nanoniosomes were also prepared by the same method without using the drug.
Characterization of the nanoniosomes
The droplet size of each nanoniosome was measured by a particle size analyzer (PSA) of Scatterscope, Qudix (South Korea). For recording field emission scanning electron microscopy (FESEM) images from b-Span 20 (as the selected formulation), the sample was diluted 20 times with distilled water and then mixed by a vortex. One drop of the niosomal dispersion was placed on a microscope slide. 1% aqueous solution of phosphotungstic acid dye was employed for staining following by air drying. Excess solution was taken off by a piece of filter paper. Next, they were washed twice with distilled water. Dehydration of the sample was done in an acetone gradient of 35%, 50%, 70%, 80%, 95% and 100%, respectively. After air-drying, the morphological observation of stained sample was performed by field emission scanning electron microscopy TESCAN Mira 3-XMU (Brno, Czech Republic).
In vitro cytotoxicity
The cytotoxicity of the nanoniosomes was evaluated by the MTT-based assay, and compared to free drugs toward MCF7 and PANC1 cell lines. The cells were seeded at dilution of 2 × 104 per well in RPMI supplemented with 10% FBS, and 1% penicillin and streptomycin, at 37 °C in a humid atmosphere containing 5% CO2. Thereafter, the culture was visualized under light microscope to ensure the cell growth and the absence of any other contamination. Then, 10 µL from various concentrations of CAP-loaded nanoniosomes or CAP dissolved in PBS separately were added to attain final concentrations of 1.56–100 µg·mL−1. Untreated cells were used as a control. Plates were incubated for 24 h. Following this, 10 μL of MTT solution (5 mg·mL−1 in PBS) was added per well and the plates were stored for 4 h in dark. After 4 h, all media were removed and 100 µL of DMSO was added to each well to dissolve the formazan crystals. The optical density at 570 nm was read using an ELISA reader of Awareness Technology (Palm City, USA). All experiments were carried out in triplicate. All procedures were performed under sterile conditions.
Drug quantitation by HPLC
Standard stock solutions of CAP were prepared separately in the mobile phase, and standard solutions were prepared by dilution. Using a pre-plotted calibration curve constructed, the concentration of CAP in the samples was determined. Samples of CAP were analyzed using a Waters HPLC (MA, USA) equipped with a UV-vis detector and Eurospher C18 column (5 µm, 4.6 mm × 250 mm) from Knauer (Berlin, Germany). A mixture of methanol–acetonitrile–water at a ratio of 80:18:2, v/v was used as mobile phase in the isocratic condition at a flow rate of 1 ml min−1 for CAP analysis. The separation was carried out at ambient temperature, 20 ± 1 °C. The mobile phase was filtered through a 0.22 µm Millipore membrane filter and degassed by a WiseClean WUC ultrasonic cleaner (Wertheim, Germany) for 5 min before use. The total run time was 4 min with a retention time of 2.7 ± 0.1 min. The solvent flow rate was 1 ml·min−1. A 60 µL volume of samples were injected into the system. Sample detection was carried out at 272 nm. Standard solutions were prepared in the mobile phase as a serial concentration in a range of 30–500 µg·mL−1 of CAP. Standard calibration curves were plotted for CAP, and its concentrations in the samples were determined. All data were expressed as mean ± standard deviation.
Entrapment efficiency and loading capacity
Entrapment efficiency (EE) and loading capacity (LC) were measured using the following equations:
(1)
(1)
1.0 ml of CAP-loaded nanoniosome of 1.0 mg·mL−1 was separated from the free drug in the solution by centrifugation at 2655 g for 15 min. The supernatant was separated. The amounts of free CAP and loaded CAP in the nanoniosomes were determined by HPLC.
In vitro release study
The release studies of niosomal formulations of drugs were performed using dialysis tube containing 2 ml of niosomal dispersion placed into appropriate containers (or small beakers) containing 25 ml of water/ethanol mixture of 70:30 ratios (v/v). Containers (or breakers) were placed in at ambient temperature and agitated with a rotation speed of 100 rpm. Collected samples in predetermined times, were analyzed by HPLC technique according to a method described above. The release of CAP from selected formulation was compared with its release from suspension containing the same quantity of drugs. All experiments were performed in triplicate.
Data analysis
All tests were repeated in triplicate to ensure reproducibility of results, and IC50 values were directly determined by AAT Bioguest online calculator. Statistical analyses were determined by one way Anova with post-hoc Tukey HSD. In addition, the results were expressed as their average and standard deviation.
Results and discussion
Nanotechnology is being carried out in the treatment of different diseases through novel drug delivery systems with different benefits over conventional ones. This study reports that the noisomes are one of favourable vehicles for cancer treatment.
Histograms for size distributions of the nanoniosome vesicles measured by PSA are presented in . Span values for the droplets size of the nanoniosomes were calculated as (D90−D10)/D50, where Di depicts the particle percentage with a size of <i. The median size (D50) of the nanoniosomes and the related span values are reported in . Based on the obtained results, all the nanodroplets of the lipid in the formulations enlarged upon loading the drug. This can be related to the drug–surfactant–cholesterol interactions inside the nanodroplets. The span values also confirm the size distribution of the nanodroplets of the nanoniosomes to be narrow. The prepared nanoniosomes had smaller sizes, compared to other niosomal formulations [Citation22,Citation31,Citation35,Citation44]. Based on the droplet seizes reported in , the relative increment in the droplet sizes of the nanoniosomes upon CAP loading as 94, 63, 80, and 70% for Span 20-, Span 60-, Span 65-, and Span 80-based nanoniosome, can be calculated. Therefore, it can be expected that CAP-Span 20 would have the beset capacity to load CAP and the most pronounced effect on the cancer cell lines (vide also infra). A FESEM image of b-Span 20 is presented in showing the nanodroplets as NPs of 45 ± 13 nm in size. The smaller size obtained from FESEM is related to shrinkage of the nanodroplets upon staining followed by drying in the course of sample preparation. The nanoniosomes can decrease systemic toxicity of drug and increase the drug concentration in cancer cells.
Figure 1. Size distribution diagrams for b-Span 20 (A1), CAP-Span 20 (A2), b-Span 60 (B1), CAP-Span 60 (B2), b-Span 65 (C1), CAP-Span 65 (C2), b-Span 80 (D1), and CAP-Span 80 (D2).
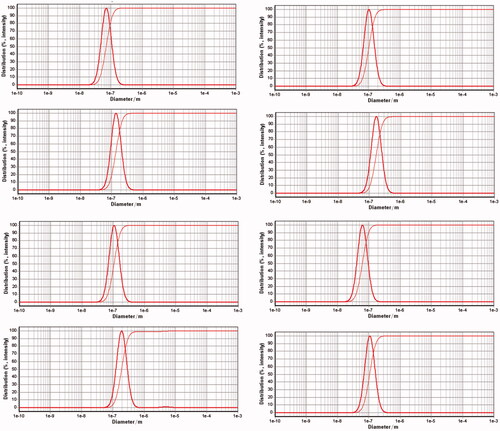
Table 2. Droplet sizes and span values for the nanoniosomes.
To measure EE and LC of the nanoniosomes for CAP, a calibration curve for the drug quantitation was plotted and shown in . According to the obtained results, CAP was determined by a calibration curve with the corresponding regression equation of y = (4482.7 ± 65.9)x − (99116 ± 16957), R2 = 0.9994, in a linear concentration range of 30–500 µg·mL−1, a relative standard deviation (for a concentration of 50 µg·mL−1) of 4.1%, and a limit of detection [as 3×(standard deviation of the blank signal)/(slope of the calibration curve)] of 2.4 µg·mL−1. In the next step, quantities of CAP loading and release into and from the nanoniosomes were determined. Based on the results, EE for CAP-Span 20, CAP-Span 60, CAP-Span 65 and CAP-Span 80 were obtained as 84 ± 6, 77 ± 8, 77 ± 9 and 62 ± 9%, respectively. In addition, LC for CAP-Span 20, CAP-Span 60, CAP-Span 65 and CAP-Span 80 were obtained as 30 ± 5, 23 ± 7, 19 ± 7 and 18 ± 9%, respectively. According to these results, CAP-span 20 was the best formulation.
Drug release is a very important factor in drug delivery systems. Dialysis of the nanoniosomes was performed for drug release at different time intervals. Release patterns of CAP from CAP-loaded nanoniosomes and a CAP solution of 1 mg·mL−1 in PBS are shown in . The release patterns of CAP-Span 60, CAP-Span 65 and CAP-Span 80 were almost similar; an initial burst was followed by slowing down and reaching a constant rate release after 4 h. The rate of CAP release from its solution is the slowest, and CAP-Span 20 represented a very fast release of the loaded drug initially and rapidly reaching steady state after about 1 h. CAP-Span 20 provided a high CAP concentration and released all of its drug content. The fast CAP release from CAP-Span 20 formulation may be due to the solubilizing ability of the nanoniosome, and it can be expected to be highly effective for CAP upon loading into the nanoniosome.
Figure 4. Release patterns of CAP from CAP-loaded nanoniosomes and a CAP aqueous solution in PBS. Inset: enlargement of the main panel.
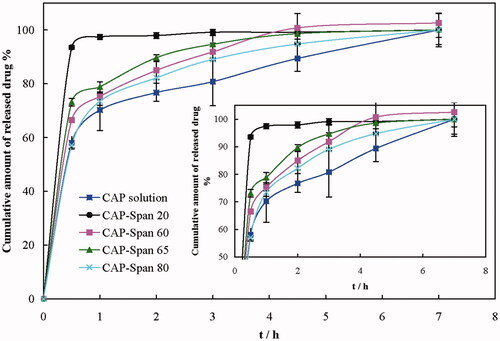
Cytotoxicity of the formulations b-Span 20, b-Span 60, b-Span 65, b-Span 80, CAP-Span 20, CAP-Span 60, CAP-Span 65, CAP-Span 80, and solutions of CAP in PBS against MCF7 and PANC1 cell lines were inspected by the MTT assay. Viability of the control group was taken as 100%. The results are presented as percentage of cell viabilities in . Cell viability in the presence of b-Span 20, b-Span 65 and b-Span 80 were almost >80% (with no significant difference with the control, p > .05). b-Span 60 represented a minor cytotoxicity against both cell lines (with significant differences with the control, p < .01). Therefore, b-Span 20, b-Span 65 and b-Span 80 can be considered as biocompatible, while Span 60-based nanoniosomes demonstrate an inherent toxicity. The results also indicated that there was no significant difference in the viability of MCF7 cells in the presence of 1.6 and 3.1 µg·mL−1 CAP solutions and the control. Slightly higher concentrations of CAP solutions demonstrated significant influence on the cells (p < .05) compared to the control, although the effect of various high-concentrations of CAP solutions was not significantly different (p > .05). Also, there was no significant difference in the viability of PANC1 cells in the presence of 1.6 to 100 µg·mL−1 CAP solutions and the control. As for the cytotoxicity of CAP-loaded nanoniosomes, toxicity of CAP-Span 60 was not considerable compared to b-Span 60 (insignificant difference, p > .05), and b-Span-60 alone showed toxicity on MCF7 and PANC cell viabilities (p < .01). CAP-Span 65 at concentrations lower than 25 µg·mL−1, and CAP-Span 80 at all concentrations did not significantly influence the viability of MCF7 cells, compared to the control, b-Span 65 and b-Span 80. Also, CAP-Span 65 and CAP-Span 80 at concentrations rather than 100 µg·mL−1 did not show significant changes (p < .05) in the PANC1 cell viability, compared to the control (b-Span65 and b-Span 80). On the other hand, the viability of both cell lines in the presence of CAP-Span 20 decreased considerably compared to the control and b-Span 20. Moreover, significant changes in the PANC1 cell viabilities were detected compared to control cells in the presence of CAP-Span 20 at concentrations higher than 1.56 µg·mL−1. Therefore, the highest cytotoxicity was observed for CAP-Span 20 toward both cell lines. Quantitatively, the viability of MCF7 and PANC1 cells in the presence of 100 µg·mL−1 of CAP-Span 20 decreased to 40 and 68%, respectively. Therefore, CAP-Span 20 is more effective in killing MCF7 cells, compared to PANC1 cells. The half maximal inhibitory concentration (IC50) of CAP-Span 20 against MCF7 and PANC1 cell line was obtained as 69.11 and 128.90 µg·mL−1, respectively.
Figure 5. Viability of MCF (A) and PANC (B) cells at different concentrations (in µg·mL−1) of b-Span 20, b-Span 60, b-Span 65, b-Span 80, CAP-Span 20, CAP-Span 60, CAP-Span 65, CAP-Span 80 and solutions of CAP in PBS.
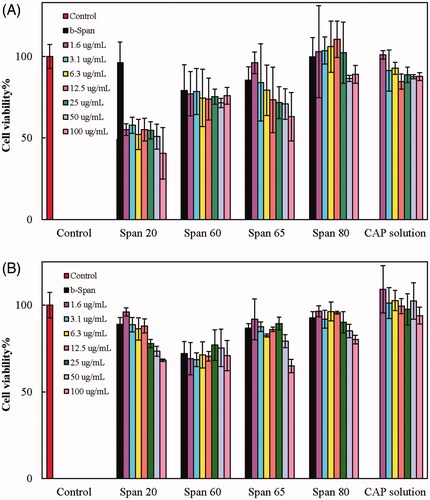
The non-ionic surfactants of Span 20, Span 60, Span 65 and Span 80 have hydrophobic property with hydrophilic–lipophilic balance (HLB) numbers of 8.6, 4.7, 2.1 and 4.3, respectively. Therefore, there is a relationship between the HLB value of Span 20, Span 60, and Span 80 and EE and LC (except Span 65). Niosomal formulations have an amphipathic structure like the bilayer structure of the cell membrane and can easily penetrate into tumours and release the drug directly into the cells. They can block the proliferation of the cells more efficiently, especially at high concentrations.
Conclusion
In recent years, extensive research has been carried out on NPs-based medicine and numerous innovations have been reported for the treatment of cancer using nanotechnology. NPs-based drugs provide enhanced permeability and efficacy, less side effects and may facilitate drug delivery into the cells [Citation41]. In the present study, the average diameter of vesicles, distribution of size, efficiency of loading, release and cytotoxicity effect of niosomal formulations of CAP were compared and compared with the CAP conventional form. Nanoniosome demonstrated reduced cytotoxicity and higher efficacy than the free drug. Niosomal are therefore promising carriers for drug delivery systems.
Acknowledgements
We would like to thank the Research Council of Shiraz University of Medical Sciences [14724] for supporting this research.
Disclosure statement
No potential conflict of interest was reported by the authors.
References
- Abdulla M, Shukla S. Dietary aspects in cancer prevention-A mini-Review. In:Atroshi F, editor. Pharmacology and nutritional Intervention in the treatment of disease. England: InTech; 2014.
- Torre LA, Bray F, Siegel RL, et al. Global cancer statistics, 2012. CA Cancer J Clin. 2015;65:87–108.
- De La Cruz L, Blankenship SA, Chatterjee A, et al. Outcomes after oncoplastic breast-conserving surgery in breast cancer patients: a systematic literature review. Ann Surg Oncol. 2016;23:3247–3258.
- Heidari M, Sattarahmady N, Azarpira N, et al. Photothermal cancer therapy by gold-ferrite nanocomposite and near-infrared laser in animal model. Lasers Med Sci. 2016;31:221–227.
- Tomuleasa C, Soritau O, Orza A, et al. Gold nanoparticles conjugated with cisplatin/doxorubicin/capecitabine lower the chemoresistance of hepatocellular carcinoma-derived cancer cells. J. Gastrointestin. Liver Dis. 2012;21:187–196.
- Van Cutsem E, Findlay M, Osterwalder B, et al. Capecitabine, an oral fluoropyrimidine carbamate with substantial activity in advanced colorectal cancer: results of a randomized phase II study. J Clin Oncol. 2000;18:1337–1345.
- Devanaboyina N, Kishore YS, Pushpalatha P, et al. Development and validation of new RP HPLC method for analysis of capecitabine in pharmaceutical dosage form. Int J Sci Inv Today. 2013;2:21–30.
- Satake H, Iwatsuki M, Uenosono Y, et al. Phase II trial of capecitabine plus modified cisplatin (mXP) as first-line therapy in Japanese patients with metastatic gastric cancer (KSCC1104). Cancer Chemother Pharmacol. 2017;79:147–153.
- Sun SB, Liu P, Shao FM, et al. Formulation and evaluation of PLGA nanoparticles loaded capecitabine for prostate cancer. Int J Clin Exp Med. 2015;8:19670–19681.
- Vincent MD, Breadner D, Soulieres D, et al. Phase II trial of capecitabine plus erlotinib versus capecitabine alone in patients with advanced colorectal cancer. Future Oncol. 2017;13:777–786.
- Chang EH, Harford JB, Eaton MAW, et al. Nanomedicine: past, present and future - a global perspective. Biochem Biophys Res Commun. 2015;468:511–517.
- Sattarahmady N, Azarpira N, Hosseinpour A, et al. Albumin coated arginine-capped magnetite nanoparticles as a paclitaxel vehicle: physicochemical characterizations and in vitro evaluation. J Drug Deliv Sci Technol. 2016;36:68–74.
- Heli H, Mirtorabi S, Karimian K. Advances in iron chelation: an update. Expert Opin Ther Pat. 2011;21:819–856.
- Nazari-Vanani R, Azarpira N, Heli H, et al. A novel self-nanoemulsifying formulation for sunitinib: evaluation of anticancer efficacy. Colloids Surf B Biointerfaces. 2017;160:65–72.
- Negahdary M, Heli H. Applications of nanoflowers in biomedicine. Recent Pat Nanotechnol. 2018;12:22–33.
- Sattarahmady N, Firoozabadi V, Nazari-Vanani R, et al. Investigation of amyloid formation inhibition of chemically and biogenically from Citrus aurantium L. blossoms and Rose damascena oils of gold nanoparticles: toxicity evaluation in rat pheochromocytoma PC12 cells. Int J Biol Macromol. 2018;112:703–711.
- VorinostatKwak TW, Kim DH, Jeong YI, et al. Antitumor activity of vorinostat-incorporated nanoparticles against human cholangiocarcinoma cells. J Nanobiotechnol. 2015;13:60–73.
- Kumar A. Leishmania and leishmaniasis. New York: Springer; 2013.
- Barakat HS, Kassem MA, El-Khordagui LK, et al. Vancomycin-eluting niosomes: a new approach to the inhibition of staphylococcal biofilm on abiotic surfaces. AAPS PharmSciTech. 2014;15:1263–1274.
- Guan L, Liu X, Xiao F, et al. Characterization of elastic niosomes prepared with various nonionic surfactants for lidocaine hydrochloride transdermal delivery. Nanosci Nanotechnol Lett. 2016;8:1033–1039.
- Shaker DS, Shaker MA, Hanafy MS. Cellular uptake, cytotoxicity and in-vivo evaluation of Tamoxifen citrate loaded niosomes. Int J Pharm. 2015;493:285–294.
- Shirsand S, Para M, Nagendrakumar D, et al. Formulation and evaluation of Ketoconazole niosomal gel drug delivery system. Int J Pharm Investig. 2012;2:201–207.
- Asgharkhani E, Azarbayjani A.F, Irani S, et al. Artemisinin-loaded niosome and pegylated niosome: physico-chemical characterization and effects on MCF-7 cell proliferation. J Pharm Investig. 2018;48:251–256
- Zarei M, Norouzian D, Honarvar B, et al. Paclitaxel loaded niosome nanoparticle formulation prepared via reverse phase evaporation method: an in vitro evaluation. Pak J Biol Sci. 2013;16:295–298.
- Kanaani L, Tabrizi MM, Khiyavi AA, et al. Improvement the efficacy of cisplatin by niosome nanoparticles against human breast cancer cell line BT-20: an in vitro study. Asian Pac J Cancer Biol. 2017;2:25–26.
- Sharma PK, Saxena P, Jaswanth A, et al. Novel encapsulation of lycopene in niosomes and assessment of its anticancer activity. J Bioequiv Availab. 2016;8:224–232.
- Jyoti K, Pandey RS, Madan J, et al. Inhalable cationic niosomes of curcumin enhanced drug delivery and apoptosis in Lung cancer cells. Indian J Pharm Educ Res. 2016;50:21–31.
- Babaei M, Ardjmand M, Akbarzadeh A, et al. Efficacy comparison of nanoniosomal and pegylated nanoniosomal Cisplatin on A172 cell line. Tissue Eng Regen Med. 2014;11:350–354.
- Mehrabi MR, Norouzian D, Shokrgozar MA, et al. Pegylated niosomal nanoparticles loaded with vincristine: characterization and in vitro evaluation. Trop J Pharm Res. 2017;16:975–980.
- He RX, Ye X, Li R, et al. PEGylated niosomes-mediated drug delivery systems for Paeonol: preparation, pharmacokinetics studies and synergistic anti-tumor effects with 5-FU. J Liposome Res. 2017;27:161–170.
- Amiri B, Ahmadvand H, Farhadi A, et al. Delivery of vinblastine-containing niosomes results in potent in vitro/in vivo cytotoxicity on tumor cells. Drug Dev Ind Pharm. 2018;44:1371–1376.
- Behroozah A, Mazloumi Tabrizi M, Kazemi SM, et al. Evaluation the anti-cancer effect of PEGylated nano-niosomal gingerol, on breast cancer cell lines (T47D), in-Vitro. Asian Pacific J Cancer Prevention. 2018;19:645–648.
- Sharma V, Anandhakumar S, Sasidharan M. Self-degrading niosomes for encapsulation of hydrophilic and hydrophobic drugs: an efficient carrier for cancer multi-drug delivery. Mater Sci Eng C. 2015;56:393–400.
- Hong M, Zhu S, Jiang Y, et al. Efficient tumor targeting of hydroxycamptothecin loaded PEGylated niosomes modified with transferrin. J Control Release. 2009;133:96–102.
- Palozza P, Muzzalupo R, Trombino S, et al. Solubilization and stabilization of beta-carotene in niosomes: delivery to cultured cells. Chem Phys Lipids. 2006;139:32–42.
- Gude RP, Jadhav MG, Rao SGA, et al. Effects of niosomal cisplatin and combination of the same with theophylline and with activated macrophages in murine B16F10 melanoma model. Cancer Biother Radiopharm. 2002;17:183–192.
- Tavano L, Muzzalupo R, Mauro L, et al. Transferrin-conjugated pluronic niosomes as a new drug delivery system for anticancer therapy. Langmuir. 2013;29:12638–12646.
- Das MK, Kumar R. Development of curcumin nanoniosomes for skin cancer chemoprevention. Int J ChemTech Res. 2015;7:747–754.
- Gaikwad SY, Jagtap AG, Ingle AD, et al. Antimetastatic efficacy of niosomal pentoxifylline and its combination with activated macrophages in murine B16F10 melanoma model. Cancer Biother Radiopharm. 2000;15:605–615.
- Ghadiri M, Vasheghani-Farahani E, Atyabi F, et al. In-vitro assessment of magnetic dextran-Spermine nanoparticles for capecitabine delivery to cancerous cells. Int J Pharm Res. 2017;16:1320–1334.
- Pandey S, Mattha Vijayendra Swamy S, Mansha Ubaid Ulla U, et al. Cell line and augument cellular uptake study of statistically optimized sustained release capecitabine loaded Eudragit S100/PLGA (poly (lacticco-glycolic acid)) nanoparticles for colon targeting. Curr Drug Deliv. 2017;14:887–899.
- Gong X, Moghaddam MJ, Sagnella SM, et al. Lamellar crystalline self-assembly behaviour and solid lipid nanoparticles of a palmityl prodrug analogue of Capecitabine-A chemotherapy agent. Colloids Surf B Biointerfaces. 2011;85:349–359.
- Payghan SA. Design of three-factor response surface optimization of camouflaged capecitabine nanoerythrovesicles. Asian J Pharm. 2016;10:306–321.
- Kakkar S, Kaur IP. Spanlastics - a novel nanovesicular carrier system for ocular delivery. Int J Pharm. 2011;413:202–210.