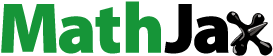
Abstract
Chondroitinase ABCI (cABCI) is a drug enzyme that can be used to treat spinal cord injuries. Due to low thermal stability of cABCI, this enzyme was immobilized on Fe3O4 nanoparticle to increase its thermal stability. The size and morphology, structure and magnetic property of the Fe3O4 nanoparticles were characterized by the analyses of SEM, XRD and VSM, respectively, and FTIR spectroscopy was employed to confirm the immobilization of cABCI on the surface of Fe3O4 nanoparticles. The results indicated that the optimum conditions for pH, temperature, cABCI-to-Fe3O4 mass ratio and incubation time in immobilization process were 6.5, 15 °C, 0.75 and 4.5 h, respectively, and about 0.037 mg cABCI was bound to 1 mg of Fe3O4 nanoparticles at these conditions. The value of Vmax was the same for free and immobilized cABCI, but Km value for immobilized cABCI was 1.6 times higher than that for free one. The storage stability of immobilized cABCI was significantly enhanced at low temperatures, e.g. free cABCI retained 19% of its activity after six days at –20 °C, while the immobilized one retained 96% of its activity. In vitro release of cABCI from Fe3O4 particles showed that about 94% of the enzyme was released after 6 h.
Introduction
Chondroitinase ABC (cABC, EC 4.2.2.4) is a bacterial lyase which is able to split the chain of glycosaminoglycans (GAGs) through a β-elimination reaction of 1,4-hexosaminidic bonds, hence this enzyme can degrade the chondroitin 4-sulphate (C4S), chondroitin 6-sulphate (C6S), dermatan sulphate (DS), and hyaluronic acid and yields unsaturated disaccharides [Citation1,Citation2]. The cABC enzyme produced by the Proteus vulgaris bacterium has two isoforms, i.e. endolytic (cABC I) and exolytic (cABC II), containing 997 and 990 amino acid residues, respectively, and the catalytic efficiency of cABC I is more than cABC II [Citation3–5].
Formation of glial scar is one of the reactive changes occurring following a spinal cord injury (SCI). During this cellular process, the glial cells surround the injury sites of central nervous system (CNS) and can physically prevent the advancement in outgrowth and repair of injured axons [Citation6]. The chondroitin sulphate proteoglycans (CSPGs) consisting of a protein core with one or more GAG chains are one of the chemical factors in the extracellular matrix of the glial scar that can inhibit the axon regrowth and regeneration of CNS [Citation7], and this inhibitory role has been illustrated in the in vitro [Citation8] and in vivo [Citation9] studies. In addition to ability of the cABC enzyme in digesting GAG chains of CSPG molecules, this enzyme has beneficial influences on the growth, sprouting, and plasticity of axons [Citation7]. For this reason, remarkable attention has been attracted to cABC as a potential therapeutic approach in the treatment of SCI in recent years.
Although many studies verified the positive effects of cABC enzyme as a therapeutic strategy, the in vivo utilization of this enzyme has been limited owing to low thermal stability [Citation1]. The activity of cABC rapidly diminishes at 37 °C; hence, local infusion or repeated injections for a period of days to weeks should be provided in the injured sites to retain its enzymatic activity [Citation10,Citation11]. These types of therapeutic methods are susceptible to infection and can lead to the immune system response of the patient body; therefore, increasing thermal stability of cABC is essential. Several methods have been reported in the literature to enhance the thermal stability of enzymes, i.e. utilization of stabilizing additives, mutagenesis, chemical modification and immobilization [Citation1,Citation11–15].
As the enzyme attachment on a carrier reduces the enzyme unfolding, the immobilization method becomes a popular strategy to stabilize the enzyme. To date, various nanoparticles, nanofibers and mesoporous materials have been developed as a carrier due to recent advances in nanotechnology [Citation16–21]. In this study, the magnetite (Fe3O4) nanoparticle was selected as a carrier owing to low toxicity, biocompatibility with biological materials, strong magnetic property, and easy separable by a magnetic field. The Fe3O4 particles were synthesized via co-precipitation method and were used for the chondroitinase ABCI (cABCI) immobilization to stabilize it against thermal inactivation. The analyses of SEM and VSM were used to examine the size, morphology and magnetic response of the particles, and the cABCI immobilization onto Fe3O4 was confirmed by FTIR analysis. At the beginning of this research, the parameters affecting the cABCI immobilization onto Fe3O4 particles, including pH, temperature, cABCI-to-Fe3O4 mass ratio and incubation time were optimized, then a comprehensive study regarding influence of pH, pH-stability, thermal stability, storage stability, and reusability of free and immobilized cABCI was conducted. In addition, the activity and kinetic parameters for both the free and immobilized cABCI were determined and the release of cABCI from Fe3O4 particles in an in vitro medium was explored.
Materials and methods
Materials
All the chemicals were prepared with analytical grade purity. Ammonium hydroxide (NH4OH) 25%, hydrochloric acid (HCl) 37%, iron(II) chloride tetrahydrate (FeCl2·4H2O), iron(III) chloride hexahydrate (FeCl3·6H2O), C4S, kanamycin, KH2PO4, citric acid and glycine were all purchased from Merck (Darmstadt, Germany). Isopropyl-β-d-thiogalactopyranoside (IPTG) and Ni-NTA agarose were provided by Takara (Kyoto, Japan) and Qiagen (Hilden, Germany), respectively.
Synthesis of magnetic nanoparticles
The magnetic nanoparticles (Fe3O4) were synthesized using the chemical co-precipitation method of Fe2+ and Fe3+ ions with the molar ratio of 1:2. In this regard, 0.40 g of FeCl2·4H2O and 1.08 g of FeCl3·6H2O were dissolved in 5 mL of 2.0 M HCl solution and poured into a three-neck round-bottomed flask. The solution was kept under continuous magnetic stirring at 25 °C and 50 mL NH4OH solution (0.7 mM) was added dropwise for 30 min until a black precipitate formed. The precipitate was separated by a vigorous magnet from the solution and washed three times with methanol to remove impurities from Fe3O4 nanoparticles. The particles were finally dried at 50 °C in a vacuum dryer.
Enzyme expression and purification
pET-28a plasmid carrying wild type gene of cABCI was transformed into the BL21 competent cells [Citation1]. Four millilitres of overnight culture was used to inoculate 400 mL of Luria–Bertani (LB) medium containing kanamycin (0.05 mg/mL) at 37 °C. After the optical density at 600 nm reached 0.5, IPTG (0.7 mM) was added and the culture was incubated at 27 °C for 6 h. The cells were harvested by centrifugation at 4000×g for 15 min, and the cell pellet was re-suspended in lysis buffer (50 mM KH2PO4, 300 mM NaCl, 5 mM imidazole; pH 6.8) and disrupted by sonication. Afterward, centrifugation (13,000×g, 15 min, 4 °C) was performed and the supernatant was purified with Ni-NTA column. A further purification step was also performed with centricon and the purity of cABCI enzyme was assessed by the single-band on SDS-PAGE. The concentration of the enzyme was determined according to the Bradford method [Citation22].
Immobilization procedure
To immobilize cABCI enzyme onto magnetic nanoparticles through physical adsorption method, 1 mg of Fe3O4 particles was added to 2.5 mL of phosphate-glycine buffer (50 mM, pH 6.5), then the mixture was sonicated for 30 min to completely disperse the particles in the buffer solution. Afterwards, 0.38 mL of cABCI solution (2 mg/mL in 50 mM phosphate buffer) was added and the reaction mixture was incubated at 15 °C for 4.5 h with shaking. Finally, the Fe3O4 particles bound to cABCI enzyme were separated from the solution using a vigorous magnet, then the supernatant was used to determine the amount of unbound cABCI and the cABCI-bound Fe3O4 was washed three times with phosphate buffer and used to measure the activity of immobilized enzyme.
Activity measurement of cABCI enzyme
The activity of free and immobilized cABCI enzyme was evaluated using UV–vis spectrophotometric method on the basis of product formation. In this regard, the increase in absorbance at 232 nm wavelength as a function of time in phosphate buffer (pH 8, 25 °C) was determined and the molar absorption coefficient (ε) of 3800 M−1 cm−1 was used in the calculations [Citation2]. One unit of cABCI activity was defined as the amount of enzyme releasing 1 µmol unsaturated oligosaccharides per minute under the assay conditions specified. In addition, to calculate the kinetic parameters of the Michaelis–Menten equation, 10 µL of free and immobilized cABCI enzyme with the same concentration (0.04 mg/mL) was added to 340 µL of a phosphate buffer solution containing various concentration of C4S substrate and the initial rate was measured, then the kinetic parameters of Km and Vmax were calculated by a nonlinear regression. All the experiments were implemented at least three times and the standard deviations were ±6%.
Determination of immobilization efficiency
After the immobilization of cABCI onto Fe3O4 particles, the particles were washed three times with the buffer solution to remove the unbound enzyme remained on the carrier surface. Then, the amount of unbound cABCI in the supernatant was measured according to the Bradford method at 595 nm with the bovine serum albumin (BSA) as protein standard. The amount of immobilized cABCI enzyme, A, was determined as follows:
(1)
(1)
where Ci and Cs are the cABCI concentration initially used for the immobilization process and in the supernatant, and Vi and Vs are the volume of initial cABCI enzyme added to the reaction medium and supernatant, respectively.
Characterization
The crystalline structure and phase purity of Fe3O4 nanoparticles was analysed by X-ray diffraction (XRD). The XRD analysis was implemented on a Rigaku X-ray diffractometer using Cu Kα (λ =0.1541 nm) operating at the generator settings of 40 kV/40 mA, and the diffraction data were collected in the range of 2θ =5–80° with the step of 0.02°. The magnetic response of pristine Fe3O4 nanoparticles was analysed by vibrating sample magnetometry (VSM) at room temperature (25 °C). The mass of Fe3O4 sample was measured and the magnetic properties were determined in the magnetic field range of –12,000 to 12,000 Oe (Oersted). The size and morphology of pristine Fe3O4 nanoparticles were characterized by scanning electron microscopy (SEM). The molecular structure of pristine Fe3O4 particles, free cABCI and Fe3O4 particles after cABCI immobilization was characterized using a Perkin-Elmer Fourier Transform Infrared (FTIR) spectrometer over the scan range of 400–4000 cm–1.
Results and discussion
Due to easy separation by a magnetic field and magnetic resonance imaging (MRI) detection of superparamagnetic iron-oxide nanoparticles, Fe3O4 nanoparticle was used as the carrier to immobilize cABCI enzyme. The procedure of the synthesis of Fe3O4 nanoparticles and immobilization of cABCI enzyme on the Fe3O4 particles were detailed in the preceding section and its schematic representation is presented in . After synthesis of the Fe3O4 nanoparticles, the analyses of XRD, VSM and SEM for pristine Fe3O4 particles were conducted and the results are illustrated in , respectively.
shows that the XRD peaks appear at the angles of 2θ = 30.29°, 35.63°, 43.31°, 53.78°, 57.24° and 62.84° corresponding to Bragg’s diffraction planes of (220), (311), (400), (422), (511) and (440), respectively. The position and relative intensity of all XRD peaks could match well to the cubic spinal structure of pure Fe3O4 nanoparticles according to the standard XRD pattern of Fe3O4 nanoparticles (JCPDS card no. 19-0629). shows the magnetization behaviour of pristine Fe3O4 nanoparticle versus magnetic field. The S-shape M–H curve of this figure indicated that the values of saturation magnetization (Ms) and coercivity (Hc) were 66.3 emu/g and 15 Oe, respectively. Therefore, the synthesized Fe3O4 nanoparticles exhibited superparamagnetic behaviour at room temperature and the particles could be easily separated from the solution by a permanent magnet owing to their quick response to an external magnetic field. shows the SEM image of pristine Fe3O4 nanoparticle. The surface morphology of Fe3O4 particles indicated that the formation of the nanoparticles was nearly uniform, and their average size was approximately 30.1 nm.
Enzyme immobilization using physical adsorption method is the simplest way to bind the enzyme molecules to a carrier. This method can be easily implemented by incubating the carrier into an enzyme solution in appropriate experimental conditions, and no additional coupling reagent and modification of enzyme or carrier are required. To confirm the immobilization of cABCI enzyme onto the Fe3O4 nanoparticles, FTIR spectra for the pristine Fe3O4 nanoparticles, free cABCI, and cABCI-bound to Fe3O4 nanoparticles (Fe3O4@cABCI) are obtained and the results are shown in . All the samples were dissolved in phosphate buffer. The peaks observed at 568 cm–1 for pristine Fe3O4 particles, and 561 cm–1 and 613 cm–1 for Fe3O4@cABCI were ascribed to Fe–O bond, whereas the peaks observed at 3484 cm–1 and 3460 cm–1 were ascribed to stretching vibration of O–H adsorbed on the surface of Fe3O4 particles. It can be seen in this figure that the characteristic absorption bands for free cABCI enzyme appear at the wave numbers of 544, 1080, 1639, 2073 and 3474 cm–1. Comparison of FTIR spectra for the free and immobilized cABCI indicated changes in the peaks of functional groups. The FTIR spectrum of Fe3O4@cABCI illustrated the characteristic bands of pristine Fe3O4 nanoparticles in addition to the peaks of free cABCI enzyme, which confirmed the physical binding of cABCI on the surface of Fe3O4 nanoparticles.
Figure 3. (a) FTIR spectra for pristine Fe3O4 nanoparticle, free cABCI enzyme and Fe3O4@cABCI. Influences of (b) solution pH; (c) incubation temperature; (d) cABCI-to-Fe3O4 mass ratio; and (e) incubation time of cABCI and Fe3O4 particles on immobilization efficiency of chondroitinase ABCI enzyme.
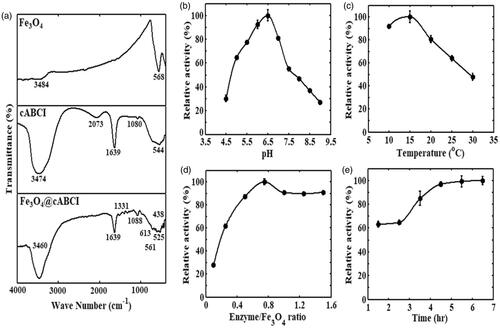
Influence of different parameters on cABCI immobilization
In the immobilization process of cABCI, the influences of solution pH, solution temperature, cABCI-to-Fe3O4 mass ratio, and incubation time of the Fe3O4 nanoparticles and cABCI enzyme on the immobilization efficiency of cABCI were investigated and the results are shown in , respectively. In , equal amount of Fe3O4 particles and cABCI were incubated in 30 mM citric acid–phosphate–glycine buffer in the pH range of 4.5–9.0 and temperature of 15 °C for the duration of 1.5 h. As it can be observed, the activity increases with an increase in pH and reaches the maximum at pH 6.5, but it is reduced for pH > 6.5. Therefore, pH 6.5 was considered as optimum pH for the immobilization process of cABCI onto Fe3O4 nanoparticles. The zeta potential of Fe3O4 nanoparticles as a function of solution pH is illustrated in . The zeta potential at pH value of 2.7 is approximately equal to zero, so the isoelectric point of Fe3O4 nanoparticle is 2.7. Daneshjou et al. [Citation14] reported that the pI of cABCI is 8.5, hence the cABCI enzyme is positively charged and the Fe3O4 nanoparticle is negatively charged at the optimum pH of 6.5 obtained in .
In , an equal amount of cABCI and Fe3O4 particles was incubated in the temperature range of 10–30 °C and pH 6.5 for a period of 1.5 h. It can be observed that the activity of immobilized cABCI boosts at low temperatures and reaches the maximum activity at 15 °C. With a further increase in temperature, the activity of immobilized cABCI reduced in which the activity of immobilized cABCI at 15 °C was two times higher than that at 30 °C. The kinetic energy of particles increases as temperature rises, so the particles move with a greater speed and zig–zag or random motions of the particles increase. Decreasing immobilization efficiency of cABCI at high temperatures may be due to increase in random motions of the particles. indicates that the maximum activity of immobilized cABCI was obtained at 15 °C, so it was considered as optimum temperature for the immobilization process of cABCI onto Fe3O4 nanoparticles.
In , various mass ratios of cABCI enzyme to Fe3O4 particles were considered and the samples were incubated at pH 6.5 and 15 °C for the duration of 1.5 h. As it can be seen, the activity of immobilized cABCI increases up to the mass ratio of 0.75, but it decreases with a further increase in mass ratio. It can be also seen that increasing mass ratio of cABCI-to-Fe3O4 above 1.0 has insignificant influence on the immobilization efficiency. On the basis of the results shown in this figure, the optimum mass ratio of cABCI-to-Fe3O4 was considered to be 0.75. As mass ratio of cABCI to Fe3O4 particles increases, a larger amount of enzyme is available for the enzyme–carrier interaction, so the surface coating of the carrier boosts. In addition, with an increase in cABCI-to-Fe3O4 mass ratio, the enzyme–enzyme interactions increase which leads to formation of multiple layers of cABCI on the carrier surface and consequently the efficiency of immobilization process increases. On the other hand, formation of multiple layers of cABCI enzyme on the surface of Fe3O4 nanoparticles hinders the diffusion of substrate and product, and as a result the immobilization efficiency reduces.
In , cABCI and Fe3O4 particles with a mass ratio of 0.75 at pH 6.5 and 15 °C were incubated for the duration of 1.5–6.5 h. It can be observed that increasing incubation time of cABCI and Fe3O4 particles boosts the activity of immobilized enzyme and then the activity reaches a plateau beyond 4.5 h. This is because the immobilization of cABCI onto Fe3O4 particles becomes saturated after 4.5 h. On the basis of the results illustrated in this figure, the optimum incubation time of cABCI and Fe3O4 nanoparticles was considered to be 4.5 h, because further increase in incubation time slightly affected the immobilization efficiency. After all the factors affecting immobilization efficiency of the cABCI enzyme onto Fe3O4 nanoparticles were optimized, the results indicated that about 0.037 mg cABCI was bound to 1 mg of Fe3O4 particles at optimum conditions.
Activity and stability study
To determine the Michaelis–Menten constants, i.e. Vmax and Km, the initial reaction rate of enzymatic reaction for the free and immobilized cABCI is obtained as a function of substrate concentration in the range of 0–2.6 mg/mL and the results are illustrated in . The cABCI concentration was 0.04 mg/mL and the activity was measured at 25 °C and pH 8. On the basis of the results of this figure, the values of Km were obtained 1.84 and 2.9 mg/mL for the free and immobilized cABCI, respectively, and the Vmax value for both the free and immobilized cABCI was obtained equal to 0.037 µmol/min. In addition, the specific activity of free and immobilized cABCI was 46 and 41 U/mg, respectively. Although the parameter Vmax was the same for both the free and immobilized cABCI, the specific activity for immobilized cABCI was less than that for free one and the value of Km for immobilized cABCI was 1.6 times higher than that for its free counterpart. The lower value for Km and higher value for specific activity of free cABCI indicate that the cABCI attachment onto Fe3O4 nanoparticles deteriorates the orientation of enzyme molecules that leads to a lower available active sites of the enzyme, and as a result the affinity of enzyme to substrate reduces. The kinetic parameters of free and immobilized cABCI are summarized in .
Figure 5. (a) The Michaelis–Menten plot; (b) influence of pH; (c) pH stability; (d) thermal stability; (e) storage stability at –20 °C; (f) storage stability at 4 °C; and (g) storage stability at 37 °C for free (—) and immobilized (––) cABCI enzyme. The activity was measured in 50 mM phosphate buffer at 25 °C and pH 8.
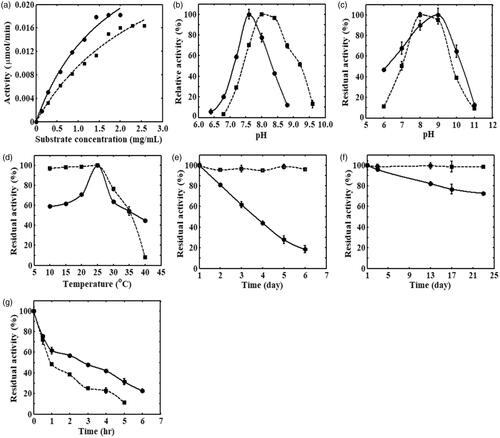
Table 1. Kinetic parameters for free and immobilized cABCI.
The activity of free and immobilized cABCI enzyme in the pH range of 6.4–9.6 at 25 °C is illustrated in . As it can be observed, the maximum activity of free cABCI is obtained at pH 7.6, whereas the maximum activity of immobilized cABCI is obtained at pH 8. The H+ ions can be absorbed from the bulk solution to the surface of Fe3O4 particles causing the pH in the surrounding of cABCI-bound to Fe3O4 nanoparticles becomes lower than that in the bulk solution. Hence, the solution pH should be increased to some extent so that the enzyme molecules be able to function properly. In addition, comparison of the activity of free and immobilized cABCI enzyme indicated that a better tolerance to the variations in solution pH could be seen for free cABCI at pH < 7.8, but the tolerance of immobilized cABCI to the pH variations was higher for pH > 7.8. The conformational changes occurred after the immobilization of cABCI on the carrier surface can explain the lower/higher tolerance of immobilized cABCI in comparison with the free one in the pH range of 6.4–9.6.
The pH-stability of free and immobilized cABCI is investigated in the pH range of 6–11 and the results are illustrated in . The samples were incubated in 50 mM phosphate-glycine buffer at 15 °C for 1.5 h, and the residual activity was measured at 25 °C. The results of pH-stability indicated that the maximum activity of free cABCI was obtained at pH 9, whereas the immobilized cABCI reached the maximum activity at pH 8. It can be also observed that the immobilized cABCI shows a lower activity in comparison with its free counterpart at different pH except pH = 7.5–8.5.
illustrates the thermal stability of free and immobilized cABCI enzyme in the temperature range of 10–40 °C. The free and immobilized samples were incubated in 50 mM phosphate buffer at pH 8 for 1.5 h, then they were placed in an ice jacket for at least 30 min and the residual activity was determined at 25 °C. As it can be seen, the maximum activity of free cABCI is obtained at 25 °C, whereas the activity of immobilized cABCI is nearly constant in the temperature range of 10–25 °C. In addition, the activity of immobilized cABCI was higher than free one for the temperature below 35 °C, but the activity of immobilized cABCI became less than its free counterpart for the temperature above 35 °C. In physical adsorption, the enzyme molecules are attached to the surface of carrier through ionic interaction, hydrogen bonding and van der Waals forces. These bonding forces are weak and slight changes in micro-environment of immobilized enzyme (e.g. temperature change) can affect the linkage of enzyme onto the surface of Fe3O4 nanoparticles. Hence increasing temperature results in detachment of enzyme molecules from the carrier surface, and this phenomenon can explain the decrease observed in thermal stability of the immobilized cABCI in comparison with the free one for the temperature above 35 °C.
depicts the storage stability of free and immobilized cABCI enzyme at three temperatures of –20, 4 and 37 °C, respectively. The free and immobilized enzymes were kept at –20 °C for six days, 4 °C for 23 days, and 37 °C for 6 h, and the sampling was carried out at various time intervals and the residual activity was determined in 50 mM phosphate buffer at pH 8. It can be observed that the free cABCI retains 19% of initial activity after six days at –20 °C and 73% of initial activity after 23 days at 4 °C, whereas 96% of initial activity at –20 °C and 99% of initial activity at 4 °C for immobilized cABCI remains after the same period of time. In addition, after incubation of the samples at 37 °C for 5 h, 31% and 11% of initial activity remains for free and immobilized cABCI, respectively. Therefore, it was obvious that the storage stability of cABCI enzyme at low temperatures, i.e. –20 and 4 °C, was significantly improved on their being immobilized to Fe3O4 nanoparticles owing to rigid bonds of enzyme and carrier preventing thermal inactivation. However, the storage stability of cABCI at 37 °C reduced after being immobilized to Fe3O4 nanoparticles. Due to weak bonding forces formed between enzyme and carrier in physical adsorption method, the cABCI enzyme is separated from the surface of carrier when the temperature rises; consequently, the storage stability of immobilized cABCI reduces at the temperature of 37 °C.
Reusability of immobilized cABCI
After the activity measurement of immobilized cABCI, the immobilized enzyme was separated using a permanent magnet and washed three times with phosphate buffer (50 mM, pH 8), then fresh reaction medium was introduced and determination of activity was repeated again. The activity of the first use was defined as 100% and the activity of subsequent uses was determined. The results indicated that only 30% and 10% of the activity of immobilized cABCI were retained after the second and third uses, respectively. As the forces bound cABCI to Fe3O4 particles are weak, the cABCI molecules are easily detached from the surface of nanoparticles after the repeated uses.
In vitro release of cABCI
Due to the magnetic property of Fe3O4 particles, they can carry drugs to targeted locations under an external magnetic field. The Fe3O4@cABCI was incubated in phosphate buffer (pH 7.5) at 37 °C for about 7 h and the samples were withdrawn at various time intervals, then the nanoparticles were separated from the solution using a vigorous magnet and the amount of cABCI in supernatant was detected at 220 nm. illustrates the release of cABCI from Fe3O4 nanoparticles as a function of time. It can be observed that as time goes on, the release of cABCI enzyme from nanoparticles increases until it reaches a constant value after 6 h. This figure also indicated that the release rate in the first one-hour was steeper than that in the rest six-hour in which 52% of the bound cABCI was released in the first one-hour and 42% was released in the rest six-hour. In physical adsorption of cABCI onto Fe3O4, the enzyme molecules are bound to the carrier surface through hydrogen bonding, ionic interaction and van der Waals force. In aqueous solutions with physiological temperature range, these linkage forces between enzyme and carrier become weaker and the bound cABCI is released from the surface of nanoparticles during a period of time.
Conclusions
The cABCI enzyme is able to cleave the GAG chains of CSPGs; hence, much attention has been attracted to this enzyme as a potential therapy for the treatment of SCI. Due to low thermal stability of this enzyme at normal body temperature and the problems concerning repeated injections in the injured sites, increasing thermal stability of cABC is necessary. Due to easy MRI detection of iron-oxide nanoparticles, Fe3O4 nanoparticle was used to immobilize cABCI enzyme to increase its thermal stability and examine its in vitro release. It was found that the Vmax value was the same for both free and immobilized cABCI, but Km value for immobilized cABCI was higher than free one. The maximum activity of free cABCI was at 25 °C, while the activity of immobilized one was nearly constant in the temperature range of 10–25 °C. The storage stability of Fe3O4@cABCI was significantly higher than its free counterpart at low temperatures of –20 and 4 °C. The results of in vitro release indicated that about 94% of bound cABCI was released during 6 h.
Acknowledgements
The present authors gratefully acknowledge the experimental support by Biochemical and Bioenvironmental Engineering Research Centre (BBRC) and Chemical Engineering Department at Sharif University of Technology, and Tarbiat Modares University (Tehran, Iran). The authors also gratefully thank Dr. B. Dabirmanesh for her help through this research.
Disclosure statement
The authors report no conflict of interest. The authors alone are responsible for the content and writing of the paper.
References
- Nazari-Robati M, Khajeh K, Aminian M, et al. Co-solvent mediated thermal stabilization of chondroitinase ABC I form Proteus vulgaris. Int J Biol Macromol. 2012;50:487–492.
- Yamagata T, Saito H, Habuchi O, et al. Purification and properties of bacterial chondroitinases and chondrosulfatases. J Biol Chem. 1968;243:1523–1535.
- Kheirollahi A, Khajeh K, Golestani A. Rigidifying flexible sites: an approach to improve stability of chondroitinase ABC I. Int J Biol Macromol. 2017;97:270–278.
- Tester NJ, Plaas AH, Howland DR. Effect of body temperature on chondroitinase ABC’s ability to cleave chondroitin sulfate glycosaminoglycans. J Neurosci Res. 2007;85:1110–1118.
- Hamai A, Hashimoto N, Mochizuki H, et al. Two distinct chondroitin sulfate ABC lyases an endoeliminase yielding tetrasaccharides and an exoeliminase preferentially acting on oligosaccharides. J Biol Chem. 1997;272:9123–9130.
- Fawcett JW, Asher RA. The glial scar and central nervous system repair. Brain Res Bull. 1999;49:377–391.
- Bradbury EJ, Carter LM. Manipulating the glial scar: chondroitinase ABC as a therapy for spinal cord injury. Brain Res Bull. 2011;84:306–316.
- Snow DM, Lemmon V, Carrino DA, et al. Sulfated proteoglycans in astroglial barriers inhibit neurite outgrowth in vitro. Exp Neurol. 1990;109:111–130.
- Tang X, Davies JE, Davies SJ. Changes in distribution, cell associations, and protein expression levels of NG2, neurocan, phosphacan, brevican, versican V2, and tenascin-C during acute to chronic maturation of spinal cord scar tissue. J Neurosci Res. 2003;71:427–444.
- Pakulska M, Vulic K, Shoichet M. Affinity-based release of chondroitinase ABC from a modified methylcellulose hydrogel. J Control Release. 2013;171:11–16.
- Lee H, McKeon RJ, Bellamkonda RV. Sustained delivery of thermostabilized chABC enhances axonal sprouting and functional recovery after spinal cord injury. Proc Natl Acad Sci USA. 2010;107:3340–3345.
- Fágáin CO. Understanding and increasing protein stability. Biochim Biophys Acta. 1995;1252:1–14.
- DeSantis G, Jones JB. Chemical modification of enzymes for enhanced functionality. Curr Opin Biotechnol. 1999;10:324–330.
- Daneshjou S, Dabirmanesh B, Rahimi F, et al. Porous silicon nanoparticle as a stabilizing support for chondroitinase. Int J Biol Macromol. 2017;94:852–858.
- Vossoughi A, Matthew HWT. Encapsulation of mesenchymal stem cells in glycosaminoglycans-chitosan polyelectrolyte microcapsules using electrospraying technique: investigating capsule morphology and cell viability. Bioeng Transl Med. 2018;3:265–274.
- Liao MH, Chen DH. Immobilization of yeast alcohol dehydrogenase on magnetic nanoparticles for improving its stability. Biotechnol Lett. 2001;23:1723–1727.
- Jia H, Zhu G, Wang P. Catalytic behaviors of enzymes attached to nanoparticles: the effect of particle mobility. Biotechnol Bioeng. 2003;84:406–414.
- Jia HF, Zhu GY, Vugrinovich B, et al. Enzyme-carrying polymeric nanofibers prepared via electrospinning for use as unique biocatalysts. Biotechnol Prog. 2002;18:1027–1032.
- Kim BC, Nair S, Kim J, et al. Preparation of biocatalytic nanofibers with high activity and stability via enzyme aggregate coating on polymer nanofibers. Nanotechnology. 2005;16:S382–S388.
- Beck JS, Vartuli JC, Roth WJ, et al. A new family of mesoporous molecular-sieves prepared with liquid-crystal templates. J Am Chem Soc. 1992;114:10834–10843.
- Diaz JF, Balkus KJJ. Enzyme immobilization in MCM-41 molecular sieve. J Mol Catal B Enzym. 1996;2:115–126.
- Bradford MM. A rapid and sensitive method for the quantitation of microgram quantities of protein utilizing the principle of protein–dye binding. Anal Biochem. 1976;72:248–254.