Abstract
Glioblastoma Multiform (GBM) known as the most common CNS malignant tumour. Therapy for GBM includes maximum tumour resection and chemotherapy. Recent advances have emphasized the use of nanoparticles, such as zinc oxide nanoparticles (ZnO-NPs). Conjugated ZnO NPs with folic acid (FA) easily pass through cell membrane. In the present study, ZnO NPs-FA applied to GBM U87MG cell line. ZnO NPs-FA synthesized according to the sol-gel method. The GBM U87MG and astrocytes 1321N1 cell lines cultured and divided into control, sham and ZnO NPs-FA groups. MTT assay used for the cell viability, and ROS assay and flow cytometry exploited. The size of nanoparticles was ≤20 nm using TEM and FTIR. After 12 hours, the viability for U87MG cells showed a significant decrease at 1.25 and 2.5 mg/ml concentrations. However, no such results obtained for astrocytes. According to the results, the ROS assay caused a significant increase in GBM cells at the mentioned concentration. It was concluded that dose-dependent conjugated NPs could play a therapeutic role in cancer therapy.
GRAPHICAL ABSTRACT
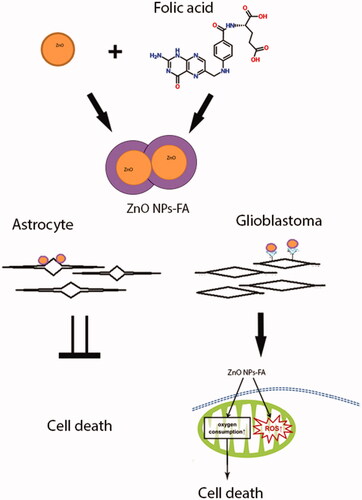
Introduction
Glioblastoma Multiforme (GBM) is a common brain tumour in adults with a high incidence and mortality rate in spite of surgery, radiation and chemotherapy [Citation1,Citation2]. With all the advances made in the treatment of GBM, the survival rate is still very low, estimated below two years since diagnosis. Conventional treatments for GBM include invasive surgery, chemotherapy and radiotherapy, each of which has its own limitations. Therefore, the treatment outcome is still poor [Citation3]. Astrocytes act as the most effective satellite cells in the central nervous system and are involved in several physiological functions [Citation4–6]. Pathological studies have shown the involvement of astrocytes in GBM. Recently, nanotechnology has more focused on the treatment of certain types of diseases, including cancers [Citation7]. The nanoparticles play an important role in the development of new drug delivery methods for cancer treatment [Citation8].
Zinc oxide (ZnO) is a metal oxide with a significantly small size less than many other organic materials. This material is widely used as an additive in numerous materials and products [Citation9]. In addition, ZnO has the photocatalytic ability and photo-oxidizing capacity against prokaryotic and eukaryotic cells [Citation10–13]. By using multifunctional nanoplatforms, ZnO bombards malignant cells from the outside through the external release of reactive oxygen species (ROS) [Citation14]. Therapeutic benefits of the conjugated form of ZnO have been recently of potential medical interest [Citation15]. Folic acid is a member of vitamin B family and plays an important role in health and disease. Among the known conjugation agents, folic acid has attracted more attention since it is essential for metabolism and biosynthesis of cellular pathways (e.g. purine and methionine) as a part of the enzymatic co-system for DNA and amino acids [Citation16]. Moreover, folic acid has a high affinity for cancer cell receptors as a ligand and is very effective in intracellular activity [Citation17,Citation18]. This compound is able to control the size of nanoparticles using its surface density. In addition, it can easily bind to folate receptors [Citation15]. Due to the existence of folate receptors in cancer cells, folic acid is specifically used to detect cancerous cells [Citation19]. Accordingly, it seems that the conjugated form of ZnO might have more therapeutic effects on certain cancers. In order to examine this hypothesis, the present study was conducted to assess the effects of folic acid-ZnO NPs on GBM cell lines.
Materials and methods
Reagents
The following compounds were purchased from the mentioned institutions: folic acid (C19H19N7O6, procured from Sigma), dimethyl sulfoxide (DMSO) from Sigma; ammonia from MERCK; DCF powder (dichlorofluorescein) from Sigma; DMEM from Gibco Co.; foetal bovine serum (FBS) from Gibco Co.; Trypsin-EDTA from Gibco Co.; penicillin-streptomycin antibiotics from Sigma; MTT powder from Sigma; PBS from Gibco Co.; folic acid from Sigma Co.; and acetate zinc from Sigma Company (USA). In addition, 1321N1 and U87MG cell lines were purchased from the Cell Bank of the National Center for Genetic and Biological Diversity of Iran.
Synthesis of ZnO NPs
ZnO nanoparticles were prepared by the sol-gel technique from the zinc acetate (Zn(CH3COO)2.2H2O). Zinc acetate solution (250 mg/10 ml TDW) combined with 10 ml of ammonia solution (Merck, USA) (1:1). The prepared solution added to the folic acid solution (folic acid (C19H19N7O6, is dissolved in mildly alkaline TDW) [Citation16] and the pH of the mixture considered at 7.5. The final solution sonicated for 30 min and 80%, centrifuged for 10 min at 6000 rpm. Finally, the precipitate placed in a glass plate and kept in the room temperature until dried. The sample used for the following techniques [Citation15].
Physical methods of characterization of the ZnO nanoparticles
Transmission electron microscopic (TEM, LEO 906 Zeiss 100KV, Germany) was used to evaluate the morphology of the synthesized product. To this end, the ZnO sample dispersed in TDW through a probe sonicator. A drop of the same compound was placed on a carbon-coated copper grid and dried at room temperature. Furthermore, the selected area electron diffraction (SAED) patterns were recorded to determine the growth orientation of the synthesized ZnO [Citation15].
Spectroscopic measurements
Fourier transmission infrared (FTIR) spectroscopy of the powders (as pellets in KBr, without moisture) was performed using a Fourier transform infrared spectrometer (Perkin Elmer FTIR system (USA); Spectrum GX) in the range of 400–600 cm−1 with a resolution of 0.2 cm−1 [Citation15].
Preparation of treatments
First, we dissolved 20 mg of folic acid-ZnO NPs powder in 20 ml deionized water and made a 20 mg/ml concentration, then prepared serial concentrations (1.25, 2.5, 5 and 10 mg/ml).
Cell culture
After purchasing the cell lines, the thawing cells were cultured in Dulbecco’s modified Eagle’s medium (DMEM) with 5% (v/v) foetal bovine serum, 10% (v/v) horse serum, 100 units/ml penicillin and 100 μg/ml streptomycin, maintained at 37 °C in a 90% humidified atmosphere containing 5% CO2. After that, four subculture cells used for treatments and tests.
Viability assay
After four subcultures, cells seeded at the density of 5000 per well in a 96-well microplate for the MTT assay. Afterwards, the cells divided into three groups of control, sham and various concentrations of 10, 5, 2.5 and 1.25 mg/ml, at 12, 24 and 48 h with three triplications. At the end of the treatment, we added 20 μl of MTT (5 mg/ml in PBS) for four hours. After adding the 200 μL/well DMSO, the absorbance was read at 570 nm by ELISA reader (Bio-Rad) after 10 min of incubation [Citation20].
ROS assay
Twelve hours after adding the folic acid-ZnO NPs to the cellular media, the cells evaluated under invert microscope. Following that, we emptied the environment inside each well and added 1 ml of PBS solution into all wells. After that, 1 ml of DCF solution added to each well, and the plate wrapped with an aluminium foil and incubation for 30 min. After 30 min, 1 ml PBS solution was added and read by ELISA reader in 450–550 nm [Citation21].
Statistical analysis
Data analysis were performed by SPSS 24 using four independent tests, one-way ANOVA, and Bonferroni and Dunnett multiple comparisons tests. In addition, data were presented as mean ± SEM, and p value less than .05 was considered statistically significant.
Results
TEM results
We measured the size of ZnO NPs-FA by using TEM. In this respect, the mean diameter of isolated particles was 20 nm ± 1 ().
FTIR results
FTIR spectral studies of ZnO NPs-FA showed that the infrared spectrum recorded in the range of 4000–400 cm−1. The initial peak was 3473 cm−1, which showed the presence of the OH band. The second peak was 1617 cm−1, which demonstrated the CO band. Finally, the presence of COOH indicated the presence of ZnO NPs-FA. Accordingly, FTIR confirmed the conjugation of FA and ZnO NPs ().
Viability assay results
As represented in , in the GBM cell lines treated by ZnO NPs-FA, all different concentrations significantly reduced viability, with the exception of the 0.31 and 0.62 mg/ml concentrations, compared to the control and sham groups. The effects of ZnO NPs-FA on GBM cell lines considered as dose-dependent. In addition, a high concentration of NPs led to more cell death and better suppression of the inflammation after 12 h (p < .01). Results of MTT assay for astrocyte cell line showed that ZnO NPs-FA concentrations within the range of 0.31–5 mg/ml had no cytotoxicity. The higher concentrations, especially 20 mg/ml, showed significant cytotoxicity for astrocytes, compared to other concentrations, as well as the control and sham groups (p < .01). Accordingly, the ZnO NPs-FA concentrations within the range of 1.25–5 mg/ml considered as safety doses for astrocyte viability ().
Figure 3. Percentage of viability of treated glioblastoma cells at 12 h (Mean ± SEM); * a significant difference with the control and sham groups simultaneously. ‡ a significant difference with the other concentration groups simultaneously.
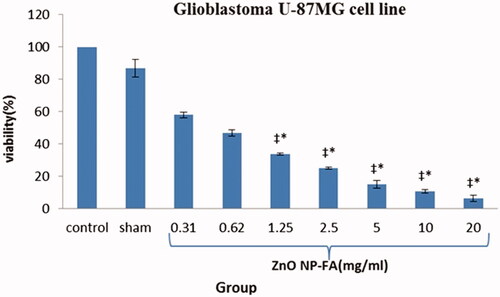
Figure 4. Percentage of viability of treated astrocyte cells after 12 h (Mean ± SEM); * a significant difference with the control and sham groups simultaneously. ‡ a significant difference with the other concentration groups simultaneously.
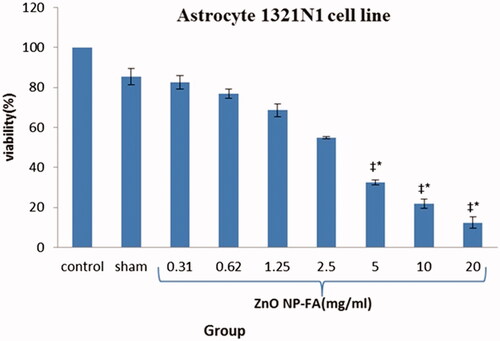
To study the role of the time exposure of ZnO NPs-FA on GBM cell lines, three different exposure times (i.e. 12, 24 and 48 h) were applied for the effective concentration of 1.25 mg/ml (). The most significant cytotoxicity for the GBM cell lines was observed after 48 h of exposure time (p < .01). Moreover, the cytotoxicity effects of ZnO NPs-FA on GBM cell line was time dependent.
ROS assay results
The ROS production following treatment with different concentrations of 1.25, 2.5, 5 and 10 mg/ml ZnO NPs-FA in GBM cell lines shown in . Compared to the control and sham groups, there was a significant increase in ROS production in all the mentioned concentrations. Among these concentrations, the most efficient result was recorded for the of 10 mg/ml concentration of ZnO NPs-FA.
Figure 6. Generation of ROS in glioblastoma U-87MG (a) and astrocyte 1321N1 (b) cell lines treated with different concentrations of folic acid-ZnO NPs after 12 h using the oxidation sensitive dye DCFH-DA.
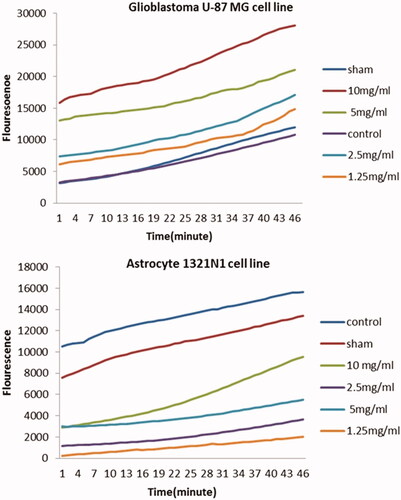
For the astrocyte cell line, our results showed that ROS production in 1.25 and 2.5 mg/ml concentrations was less than the control group and had no significant increase in this regard (p > .05). Meanwhile, there was a higher ROS generation in concentrations of 5 and 10 mg/ml, compared to other concentrations.
Discussion
GBM is a type of brain tumour from glial cells that is fast growing and likely to spread. The use of nanotechnology can be promising since the conventional therapies of the tumours have been inadequate and unsuccessful. Nanoparticles play an important role in developing new drug delivery methods for cancer treatment [Citation8]. Nanoparticles, with their selective targeting capabilities and superior efficacy, are becoming increasingly important in modern cancer therapy and starting to overshadow traditional cancer therapies such as chemotherapy, radiation and surgery (Gunjan Bisht and Sagar Rayamajhi) [Citation22]. Nanoparticles target the tumour cells, and pass the tumour cell membrane easily. ZnO nanoparticles, with their unique properties such as biocompatibility, high selectivity, enhanced cytotoxicity and easy synthesis, may be a promising anticancer agent [Citation22]. In 2009, Ostrovsky et al. reported the cytotoxic effects of ZnO NPs on the U-87MG glioma and astrocyte cell lines. According to them, while there was no cytotoxic activity in astrocyte cells, ROS generation increased in GBM cells. Therefore, ZnO NPs showed a selective cytotoxic effect on the removal of cancer cells [Citation8]. In the mentioned research, the size of ZnO NPs was 60 nm, which was three times larger than the NPs used in the current research. In 2016, Wahab et al reported cytotoxic effects of Zno-Nps on Glioma malignant cell line and the non-malignant neonatal cell line of MRC5 fibroblast [Citation12]. In 2011, Premanathan et al. studied the effect of ZnO nanoparticles on human HL60 cell lines and PBMC (normal blood cell) counts. They showed that the ZnO nanoparticles had the ability to eliminate HL60 cancer cells [Citation23]. There are also other studies regarding the use of different nanoparticles such as gold, platinum and titanium oxide for cancer therapy [Citation11–19].
Hanley et al. in 2008 reported that ZnO nanoparticles have an inherent nature showing selective cytotoxicity against tumour cells in vitro condition compared with other nanoparticles [Citation24]. There are also some reports of combination use of ZnO nanoparticles with other agents and modalities. In 2008, Guo et al. evaluated the cytotoxic effects of ZnO nanoparticles on leukaemia cancer cells using Daunorubicin and ultraviolet radiation. They used ZnO NPs as the carrier agents for cancer cells that induced cellular toxicity. It seems that the combination of ultraviolet radiation with Daunorubicin together with ZnO nanoparticles showed better therapeutic effects on blood cancer cells [Citation11].
There is disparity of the results by using ZnO nanoparticles with or without other agent. For example, Ostrovsky et al. reported cell death in GBM cell lines with 10 mmol/l ZnO NPs after 72 h, whereas our findings showed a significant cell death with 1.25 and 2.5 mg/ml concentrations of ZnO NPs-FA in GBM cell lines after 12 h and an astrocyte cytotoxicity in 5 mg/ml. We assume that this variety in results might come from the size and dose of Zno NPs and using other combining agents with ZnO NPs. The action mechanism of ZnO NPs on tumour cells is a matter of question. Now we know that in addition to inherent nature and selectivity of the ZnO nanoparticles against tumour cells, they easily attach to cell membrane and initiate the intracellular ROS elevation and protein activity disequilibrium, that lead to ROS-induced oxygen species and induction of apoptosis.
Better results were found when we used ZnO NPs conjugated with folic acid as compared to other studies that used ZnO NPs merely. Folic acid has many metabolic effects on different cells and tissues. Folic acid inserts its effects on cells via folate receptors (FRs). The folate receptor binds with folic acid and physiologically active N5-methytetrahydrofolate with great affinity [Citation25]. Folic acid facilitates DNA synthesis that in turn leads to cell division, the phenomenon that is vital to tumour cells. The expression of folate receptor alpha is limited in adult tissue. Different studies showed that FRs expressed in a variety of cancer type. FRs are widely used for tumour targeting with folic acid conjugates of diagnostic and therapeutic [Citation26]. In clinic, anti-folate chemotherapy used for some certain cancers. It seems that anti-folate drugs attach to folate receptors and cause the inhibition of the cell division of cancerous cells; anti-folate agents do not let the cancerous cells using body folic deposit for their proliferation. Although the role of folic acid in cell proliferation for cancerous cells, we used its conjugated form with ZnO NPs in order to ensure of higher affinity for FRs receptors by ZnO. In other words, we assume that FA part of ZNO NPs-FA complex facilitate the attachment of ZnO NPS to cancerous cells. Therefore, ZnO NPs-FA has a higher apoptosis induction potential. In addition to this role of FA, there are some reports that show the protective effect of folic acid on certain cancers such as colorectal. Kim et al in 2013 reported that in individuals with the highest dietary folate intake compared with those with the lowest intake dietary folate intake the risk of colorectal cancer reduced significantly [Citation27]. Thus, FA seems has dual role and its possible protective effect should consider in cancer therapy.
For the results of the effects of ZnO NPs- FA on astrocyte cell line, we showed that ZnO NPs-FA induce apoptosis in astrocyte cell line for all doses used except for the dose of 1.25–2.5 mg/ml that no apoptosis was seen that considered as a safe dose. For the effects of ZnO NPs-FA on astrocyte cell line there might be other mechanisms of effectiveness because we did not find any reports that confirm the existence of FRs on astrocyte cells and we assume that the size and the dose of ZnO NPs-FA might play a role in this regard. However, more studies are needed for understanding the exact mechanisms of nanoparticles with or without conjugated agents on normal and tumour cells.
Conclusions
In conclusion to the results of many researches on the effect of nanoparticles and regarding their unique properties, especially for ZnO NPs, the future of cancer therapy will change dramatically and by using NPs many well promising methods will help the patients suffering from cancer. Among these particles, we believe that the ZnO NPs definitely play an important role especially if used in conjugated form such as FA.
Compliance with ethical standards
This article was approved by the Ethics Committee of Iran University of Medical Sciences.
Acknowledgements
We extend our gratitude to the colleagues and laboratory staff of NRC for their sincere collaboration.
Disclosure statement
There is no conflict of interest regarding the publication of this paper.
Additional information
Funding
References
- Mirjolet C, Papa AL, Créhange G, et al. The radiosensitization effect of titanate nanotubes as a new tool in radiation therapy for glioblastoma: a proof-of-concept. Radiother Oncol. 2013;108:136–142.
- Stupp R, Mason WP, van den Bent MJ, et al. Radiotherapy plus concomitant and adjuvant temozolomide for glioblastoma. N Engl J Med. 2005;352:987–996.
- Scott CB, Scarantino C, Urtasun R, et al. Validation and predictive power of Radiation Therapy Oncology Group (RTOG) recursive partitioning analysis classes for malignant glioma patients: a report using RTOG 90-06. Int J Radiat Oncol Biol Phys. 1998;40:51–55.
- Powell EM, Geller HM. Dissection of astrocyte-mediated cues in neuronal guidance and process extension. GLIA. 1999;26:73–83.
- Fiacco TA, Agulhon C, McCarthy KD. Sorting out astrocyte physiology from pharmacology. Annu Rev Pharmacol Toxicol. 2009;49:151–174.
- Occhipinti R, Somersalo E, Calvetti D. Astrocytes as the glucose shunt for glutamatergic neurons at high activity: an in silico study. J Neurophysiol. 2009;101:2528–2538.
- Roco MC. Broader societal issues of nanotechnology. J Nanopart Res. 2003;5:181–189.
- Ostrovsky S, Kazimirsky G, Gedanken A, et al. Selective cytotoxic effect of ZnO nanoparticles on glioma cells. Nano Res. 2009;2:882–890.
- Urata K, Narahara H, Tanaka Y, et al. Effect of endotoxin-induced reactive oxygen species on sperm motility. Fertil Steril. 2001;76:163–166.
- Rasmussen JW, Martinez E, Louka P, et al. Zinc oxide nanoparticles for selective destruction of tumor cells and potential for drug delivery applications. Expert Opin Drug Deliv. 2010;7:1063–1077.
- Guo D, Wu C, Jiang H, et al. Synergistic cytotoxic effect of different sized ZnO nanoparticles and daunorubicin against leukemia cancer cells under UV irradiation. J Photochem Photobiol B. 2008;93:119–126.
- Wahab R, et al. Self-styled ZnO nanostructures promotes the cancer cell damage and supresses the epithelial phenotype of glioblastoma. Sci Rep. 2016;6:19950.
- Zhang L, Jiang Y, Ding Y, et al. Mechanistic investigation into antibacterial behaviour of suspensions of ZnO nanoparticles against E. coli. J Nanopart Res. 2010;12:1625–1636.
- Yuan Q, Hein S, Misra R. New generation of chitosan-encapsulated ZnO quantum dots loaded with drug: synthesis, characterization and in vitro drug delivery response. Acta Biomater. 2010;6:2732–2739.
- Dutta S, Ganguly BN. Characterization of ZnO nanoparticles grown in presence of folic acid template. J Nanobiotechnol. 2012;10:29.
- Wu Z, et al. Solubility of folic acid in water at pH values between 0 and 7 at temperatures (298.15, 303.15, and 313.15) K. J Chem Eng Data. 2010;55:3958–3961.
- Saikia C, Das MK, Ramteke A, et al. Evaluation of folic acid tagged aminated starch/ZnO coated iron oxide nanoparticles as targeted curcumin delivery system. Carbohydr Polym. 2017;157:391–399.
- Geszke M, Murias M, Balan L, et al. Folic acid-conjugated core/shell ZnS: Mn/ZnS quantum dots as targeted probes for two photon fluorescence imaging of cancer cells. Acta Biomaterialia. 2011;7:1327–1338.
- Zak AK, Abrishami ME, Majid WHA, et al. Effects of annealing temperature on some structural and optical properties of ZnO nanoparticles prepared by a modified sol–gel combustion method. Ceram Int. 2011;37:393–398.
- Moongkarndi P, Kosem N, Kaslungka S, et al. Antiproliferation, antioxidation and induction of apoptosis by Garcinia mangostana (mangosteen) on SKBR3 human breast cancer cell line. J Ethnopharmacol. 2004;90:161–166.
- Held P, Newick K. Using BioTek’s Synergy™ HT Reader to measure reactive oxygen species (ROS) generation in stimulated cells. BioTechniques. 2009;46:61–62.
- Bisht G, Rayamajhi S. ZnO nanoparticles: a promising anticancer agent. Nanobiomedicine (Rij). 2016;3:9–9. (Godište 2016):
- Premanathan M, Karthikeyan K, Jeyasubramanian K, et al. Selective toxicity of ZnO nanoparticles toward Gram-positive bacteria and cancer cells by apoptosis through lipid peroxidation. Nanomedicine. 2011;7:184–192.
- Hanley C, Layne J, Punnoose A, et al. Preferential killing of cancer cells and activated human T cells using ZnO nanoparticles. Nanotechnology. 2008;19:295103.
- Elnakat H, Ratnam M. Distribution, functionality and gene regulation of folate receptor isoforms: implications in targeted therapy. Adv Drug Deliv Rev. 2004;8:1067–1084.
- Siwowska K, Schmid R, Cohrs S, et al. Folate receptor-positive gynecological cancer cells: in vitro and in vivo characterization. Pharmaceuticals. 2017;10:72.
- Kim Y-I. Role of folate in colon cancer development and progression. J Nutr. 2003;133:3731S–3739S.