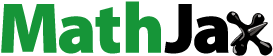
Abstract
The aim of present study was to develop the efficient targeting of Concanavalin-A conjugated nanotransfersomal gel to bind directly to melanocytes gel layer against UVB induced skin carcinoma. Carbopol loaded nanotransfersomal gel have prepared by modified rotary evaporation sonication technique & conjugated synthesized by carbodiimide method and they were characterized the morphology, zeta potential, penetration and cell viability. In vitro release studies & skin permeation have determined using Franz diffusion cell and confocal laser scanning microscope (CLSM). The conjugated formulation showed vesicles size, polydispersity index, zeta potential and % conjugation efficiency of 179.0 ± 0.32 nm, 0.197 ± 0.07, 35.1 ± 0.21 mV and 89.73 ± 1.29% respectively. The surface morphology was confirmed by transmission electron microscopy (TEM) and FTIR to make sure the compatibility among its ingredients. Con-A conjugated nanotransfersomal gel showed toxicity on melanoma (A375) in a concentration range of 0.4–2.0 mg/mL, but less toxicity toward HaCaT cells. The MTT assay has analyzed against two different cell lines, to determine their anti-cancer potentials and their targeting ability. Conjugated formulation were found to decrease the cell viability, higher skin targeting efficacy in in-vitro & in-vivo. Concanavalin conjugated nanotransfersomal gel of apigenin promise an efficient and economic approach for the skin cancer.
Introduction
Natural Drugs targeting specific organs and tissues has become one of the critical endeavours and significant challenges of the current era along with improving therapeutic index and bioavailability at site-specific delivery. The clinically significant impact of targeted drug delivery lies in the ability to specifically target a drug or drug carrier to minimize drug-originated systemic toxic effects. Targeted drug delivery systems have been developed to optimize regenerative techniques [Citation1]. Although conventional chemotherapy has been successful to an extent, the main drawbacks of chemotherapy are its poor bioavailability, high-dose requirements, adverse side effects and low therapeutic indices, which is being faced by the researchers in the anti-cancer formulation. We have exhibited that the enhanced permeability and retention is the basis for using nanobioconjugated active targeting formulation, aiming for a better understanding of tumour biology in the current years and new targeted drug delivery approaches that are being explored for effective dermal delivery at the targeted or specific site is significant. The advantages of the targeted release system are the reduction in the frequency of the dosages taken by the patient, having a more uniform effect of the drug, reduction of drug side-effects, and reduced fluctuation in circulating drug levels [Citation2–4]. Thus, bioconjugates nanocarriers therapy, worldwide, is to be used progressively for targeted delivery and has a high expansion treatment efficacy for melanoma skin cancer.
Lectins, a group of specific glycoproteins present in the animals as well as plant cells, are extracted from jack bean seeds. Although lectins have been widely studied for their potential in skin cancer diagnosis and have also been investigated for significant antiproliferative activities both in-vitro and in-vivo and generation from diverse mechanisms, including apoptosis, autophagy, and inhibition of tumour growth [Citation5]. Con-A is one of the most abundant lectins for a potential anti-neoplastic agent for melanoma cancer therapeutics and to prevent their spread [Citation6]. Several studies have demonstrated that Con-A induces caspase-dependent apoptosis in melanoma A375, have the strength to induce autophagy in hepatoma cell, and inhibits proliferation on B16 melanoma cells and fibroblast 3T3 cells [Citation7–9].
Malignant melanoma skin cancer mainly occurs due to UV-light exposure because a large amount of UV radiation reaches earth’s surface, attributable to the slow depletion of the ozone layer. The increases of UVB (290 to 320 nm) directly damages the cellular DNA leading to the formation of the 6–4 cyclobutane pyrimidine dimmers, DNA base and nucleoprotein crosslinking, and polynucleotide chains disruption [Citation10]. UVA (320 to 400 nm) indirectly damages the DNA by the production of oxygen reaction species. This type of skin cancer is associated with the melanocytes of the epidermal layer. UV radiation can cause the suppression of the immune system that leads to inadequate prevention of cancer formation [Citation11,Citation12].
Despite the recent advancement in therapeutics, significant challenges are yet present in the field of skin cancer. Commonly used chemotherapy has given unsatisfactory results, Many drugs like carmustine, dacarbazine, paclitaxel, cisplatin and carboplatin can add to the side effects as hair loss, loss of appetite, diarrhea, fatigue and increased risk of infection, as the therapy is deleterious to patient health by making patients more susceptible to other diseases and often cause death by weakening the immune system of the patient [Citation13].
Nanocarriers have been demonstrated to increase the solubility and bioavailability of poorly soluble-in-water drugs. Nanocrystals and mixed micelles system, which are not only promising and effective for improving aqueous solubility of apigenin but also have the ability for controlled drug release and precise targeting have been reported previously [Citation14,Citation15]. Nanovesicular carriers have been used to deliver drugs to target sites for cancer therapeutics. These lipid nanocarriers are adsorbed into the skin surface, allowing lipid exchange between the outermost layers of the stratum corneum [Citation16].
Recently, the strength of bioactive medicine to suppress carcinogenesis has attracted the worldwide attention of cancer exploration and treatment, among researchers. Apigenin is a bioflavone compound, which is present in a wide variety of plants and herbs and distributed plentifully in common vegetables and fruits such as grapes, apples, garlic, onions, oranges, chamomile tea and parsley [Citation17]. Apigenin that is significant chemically (4,5,7-trihydroxyflavone) is a promising skin cancer chemotherapeutic agent, both in-vitro and in-vivo, which are serving diverse physiological functions, for example, as strong anti-inflammatory, antioxidant, anti-carcinogenic, antiviral activities, anti-ageing, antibacterial and blood pressure reduction [Citation18]. Apigenin have been previously studied for human cancers in vitro and in vivo by multiple biological effects such as inducing cell cycle arrest, autophagy, triggering cell apoptosis, cell migration, invasion and suppress immune response [Citation19,Citation20]. Apigenin has also been studied to suppress protein kinase cell activity and prevention of UVB-induced skin carcinogenesis and most type of cancers, including melanoma, breast cancer, lung cancer, colorectal cancer, prostate cancer, osteosarcoma and liver cancer [Citation21,Citation22].
More studies have shown that advanced liposomes, niosomes of natural medicine have limited skin benefit, production instability and low bioavailability of compounds exhibiting anticarcinogenic, antioxidants and ant-inflammatory effects [Citation23–25] (reference. Kotla et al. [Citation26] have reported that liposomal drug delivery is unsuccessful due to their inability to permeate the deeper layers of the skin, which showed a lack of prospective availability as a carrier and reservoir for sustaining and degrading permeability within wide layers of the skin. Among them, polyphenol loaded nanotransfersomes have good strength to improve baicalin efficacy in inflammatory skin disorders and also phospholipids vesicles based hydrogels have improved in dermal delivery and formulation stability [Citation27,Citation28]. Transfersomes are self-regulating and self-optimizing ultradeformable lipids nanovesicles and are composed of water, surfactants, and phospholipids for enhanced and significant chemotherapeutic dermal drug delivery and as a drug carrier for non-invasive targeted drug delivery and sustained release of therapeutic agents [Citation29,Citation30].
Presently, the most promising aspect of utilization of various bioconjugates phytonanomedicine has been reported by different researchers and scientists such as Saraf et al.[Citation31], who have reported that PEGylated nanoparticles accumulate in tumours by the enhanced permeation and retention (EPR) effect as a function of cellular adhesion. Developing truly targeted drug delivery systems has to be based on a clear understanding of the problems that must be solved. Folate receptor-fabricated nanogels can serve as a better option for treating various cancers in terms of drug loading and stability, and cellular uptake of anticancer drugs studied and performed by Gupta et al. [Citation32] was significant. Con-A conjugated nanotransfersomal gel was employed as the nanocarrier to enhance cancer cell targeting, Con-A as targeting ligands, carbopol as a linker for prolonging circulation and phospholipids for flexibility on the skin surface. Our objective was to utilize con-A conjugated nanotransfersomal gel of a natural anticancer drug to investigate its improving anticancer efficacy and enhanced targeted delivery of UV induced skin malignant melanoma and reducing the systemic toxicity commonly accompanying chemotherapeutics.
Materials and Methods
Materials
Apigenin was purchased from Sigma Aldrich (Bombay). Tween 80, Rhodamine, [1-Ethyl-3,3-(dimethylaminopropyl) carbodiimide) (EDC)] and Phospholipid were purchased from Himedia Pvt, Ltd. Mumbai, India. Phospholipid (phosphotidylcholine) from soya lecithin containing not less than 98% PC and Flonida cream, were purchased from Shalak Pharmaceutical Ltd, Karampura New Delhi market. Con-A was supplied as a gift sample by Bio-research products, Inc., 323W. Cherry St., North library (IA 5231). N-Hydroxysuccinimide (NHS) was supplied as a gift sample from Shivam Enterprises, Pune, Maharashtra (India). Purified water from Millipore ultrapure water system (Synergy UV water purifier system, India) was used throughout the study. All other chemicals used in the study were of analytical grade.
Chemicals for cell lines and culture
The two cell lines were obtained from the National Center for Cell Science, Pune, India.3-(4,5-Dimethylthiazol-2-yl)-2,5-diphenyltetrazolium bromide (MTT) was purchased from Sigma Aldrich Ltd.(India), HaCaT cells were cultured in Dulbecco’s Modified Eagle’s Medium Nutrient Mixture F-12 HAM (DMEM F-12 HAM) with 2mM L-glutamine supplemented with 10% fetal bovine serum (FBS). Penicillin (100 mg/mL), streptomycin (100 mg/mL) and amphotericin-B (5 mg/mL), and the cells were incubated in CO2 incubator with 5% CO2. The cell suspension was centrifuged at 3000 rpm for 3 min and then re-suspended in the growth medium for further studies.
Methods
Preparation of nanotransfersomes by rotary evaporation sonication technique
Apigenin loaded nanotransfersomes were formulated by a rotary evaporation sonication technique with slight modifications as demonstrated by Cevc et al. [Citation33] and Saraf et al. [Citation32]. Concisely, apigenin drug, tween 80 and soya phosphotidylcholine were dissolved in ethanol. Ethanol was evaporated by rotary evaporation (Buchi rotavapor R-3000 with 14,000 Water bath, Switzerland) under reduced pressure above the lipid transition temperature for the formation of lipid film on the wall of the flask. The film was hydrated at 45 °C using a saline phosphate buffer solution (pH 5.5) by rotation at 60 rpm [Citation29,Citation34]. The transfersomes formed were allowed to swell for 2 h at room temperature. The resulting vesicles were sonicated for 30 min in a probe sonicator to reduce the size of the vesicles uniformly. The prepared transfersomes were stored in an airtight container for further study.
Conjugation of Con-A with apigenin loaded nanotransfersomes
Apigenin-loaded nanotransfersomes were Con-A conjugated using Carbodiimide method with some modifications as reported by Damink et al. [Citation35] and Jangdey et al. [Citation36]. Briefly, activation of carboxyl group of nanotransfersomes (50 mL) was carried out by addition of 1 mL of 0.1 M 1-ethyl-3,3-(dimethylaminopropyl) carbodiimide (EDC) and 1 mL of 0.11 M of N-hydroxysuccinimide (NHS) in phosphate buffer (pH 5.5). After 3 h incubation at room temperature, excess coupling agent and byproducts were removed by washing nanotransfersomes with phosphate buffer (pH 5.5) and filtered. The latter was suspended in 10 mL of Con-A solution in phosphate buffer (pH5.5) and after incubation for overnight. Con-A conjugated nanotransfersomes were obtained by centrifugation (C-24, BC, Cooling centrifuge, REMI, India) for 30 min at 9000 rpm, followed by three to four times washing with Millipore water. The obtained Con-A conjugated nanotransfersomes thus were dried at room temperature.
Preparation of conjugated nanotransfersomal based Gel
Conjugated nanotransfersomal base gel was prepared as previously described by Khan et al. [Citation37], with some modified, increased apigenin loading. Briefly, dispersing the 1 g of the Carbopol 934 in a sufficient quantity of Millipore distilled water. After complete dispersion, the Carbopol 934 solution was kept in the dark for 24 h for complete swelling. Afterwards, the optimized conjugated nanotransfersomes was gradually added to the viscous solution of Carbomer 934 beneath magnetic stirring. The pH values were subsequently regulated to 5–6. Then other ingredients like isopropyl alcohol (5% wt/wt), PEG-400(5% wt/wt), PG (5% wt/wt), and triethanolamine (0.5g) were added to obtain a homogeneous dispersion of gel.
Characterization of Con-A conjugated nanotransfersomes
Morphology, vesicle size and Zeta potential
The surface of conjugated nanovesicle transfersomes was selected and explored by transmission electron microscope (TEM), (Hitachi J 500, H7500 Japan). One drop of sample Con-A conjugated nanotransfersomes was placed on carbon-coated grids followed by negative staining with 2% w/v aqueous solution of phosphor-tungustic acid for viewed visualization of vesicles at 100 kV.
Size of the nanotransfersomes and Con-A conjugated nanotransfersomes was analyzed by dynamic light scattering (DLS) with a Malvern Zetasizer 3000 HAS (Malvern Instruments, Malvern, UK). The formulation (0.1 mL) was dispersed in 50 mL of sufficient amount of ultra-purified water in a volumetric flask and mixed thoroughly with vigorous shaking before the conduct of experiment and light scattering was monitored at 25 °C at a 90° angle between laser and detector.
Measurement of the zeta potential for Con-A conjugated nanotransfersomes and nanotransfersomes was determined using a Zetasizer (Malvern instrument, USA). Samples were placed in clear disposable zeta cells and results were recorded. The sample was diluted by Millipore ultra-purified water prior to the experiment. The measurements were performed in triplicate [Citation12].
X-ray diffraction study
The analysis of the natural crystalline or amorphous of apigenin-loaded nanolipid Con-A conjugated transfersomes was performed by X-ray diffraction (XRD). X-ray powder diffraction studies of pure drug apigenin, phospholipid (soya lecithin), Con-A, physical mixture (apigenin, phospholipid and Con-A) in ratio of (1:1:1) and Con-A conjugated nanotransfersomes were carried out using powder X-ray diffractometer (PANalytical 3kWX’ pert powder, Cambridge, UK). Samples were positioned in the sample stage and scanned from 2° to 60° with an operating voltage of 40 kV and current of 30 mA. A Cu-Ka radiation source was used and the scanning rate 2 h/min was 5 °C/min.
FTIR study
FTIR spectrum (Shimadzu 8400U) was carried out to confirm the conjugation of Con-A with apigenin loaded nanotransfersomes. Drug sample (apigenin, Con-A and Con-A conjugated nanotransfersomes) was analyzed using the KBr pellet technique. ∼1–5 mg of the sample was mixed with dry potassium bromide and the samples were examined at transmission mode over wavenumber range of 4000 to 400 cm−1.
Differential scanning colorimetry study
Differential Scanning Colorimetry (DSC) is one of the thermo-analytical techniques for assessment of the heat energy uptake for providing data on endothermic (heat absorption) and exothermic (heat evolution) processes. DSC analysis was performed using a DSC instrument (Mettler 305, Switzerland). The sample of 4 mg was placed in aluminium pans (Al-Crucibles, 40µl Al) and scanned between 25 to 400 °C under a nitrogen atmosphere and at a heat flow rate of 10 °C/min. The samples were studied for pure drug, phospholipids, Con-A, carbodiimide and Con-A conjugated nanotransfersomes.
Determination of interference of formulation additives in the estimation of apigenin
Stock solution of apigenin (100 µg/ml) in methanol was placed separately in five volumetric flasks. To this solution, 10 mg of each additive was added in separate volumetric flasks. The flasks were manually stirred for 6 h and kept overnight. The solution of each flask was filtered by Whatman filter paper (#41). Each solution was diluted sufficiently to produce the final concentration of 5 µg/ml in separate volumetric flasks. The absorbance of resultant solution was measured at 336 nm using UV-visible spectrophotometer (Systronics, Japan).
Conjugation efficiency of Con-A nanotransfersomes and percent entrapment efficiency
Apigenin loaded nanotransfersomes were bound to Con-A and the amount of Con-A was calculated as the difference between the Con-A added initially and the Con-A recovered after incubation with the nanotransfersomes. The amount of lectin in the supernatant was estimated by using Folin–Ciocalteu phenol reagent. Reagent C (10 mL) was added in a suspension containing 50 mL of conjugated nanotransfersomes in 50 mL of millipore distilled water, mixed thoroughly and allowed to stand for 20 min. then 1 mL of the reagent D was added rapidly with immediate mixing to the above solution. After 30 min, the solution was filtered with Whatman filter paper (#41), volume was measured against blank using a UV-visible spectrophotometer (Perkin Elmer Lambda 25 double beam UV-Visible Spectrophotometer, Singapore) at 750 nm. The experiment was performed in triplicate. Reagent A and reagent B were prepared by dissolving Na2CO3 (2 g) in 0.1 N NaOH and CuSO4.5H2O (0.5 g) in 1% sodium/potassium tartrate to make 100 mL solutions each, respectively. Reagent C was prepared by mixing 50 mL of the reagent A with 1 mL of reagent B. Reagent D was prepared by diluting Folin–Ciocalteu phenol reagent (2.0 N) with distilled water to make it 1.0 N [Citation36].
The quantity of apigenin entrapped within Con-A conjugated nanotransfersomal gel was determined by ultracentrifugation. The unentrapped apigenin in nanotransfersomes dispersion was separated by cooling centrifuge (Remi instruments Ltd., Mumbai, India) at 13,000 rpm, for 20 min at room temperature and the supernatant was decanted. The concentration of apigenin in the supernatant was determined using UV–visible spectrophotometer at λmax 336 nm. Each experiment was performed in triplicate [Citation38]. The % entrapment efficiency was calculated with the following equation:
(1)
(1)
Spreadability
Spreadability measurements of all the developed gels were carried out individually in triplicate using a wooden block and glass slide apparatus. A modified apparatus consisting of two glass slides containing sample in between, with the lower side fixed to a wooden plate and upper one attached to a balance by a hook, was used to determine the Spreadability. Specified amount of gel was taken, and the spreadability was calculated using the following formula
(2)
(2)
Where S = Spreadability in g cm s−1; M = Mass of gel placed between the two slides; L = Length of slide; t = Time taken by the upper slide to detach [Citation39].
In vitro drug release study
In vitro release studies of drug loaded Concanavalin conjugated nanotransfersomes and pure drug suspension and marketed product was carried out for each drug individually using modified Franz diffusion (Mol. Wt. cut of 6000–8000, HI Media Ltd, Mumbai, India). Vertical Franz Diffusion cell was designed and validated prior to the release study. The cellophane membrane was mounted on a diffusion cell assembly having a diffusion area of 2.5 cm. The receptor compartment consisted of a 22.5 mL phosphate buffer at pH 5.5 as the receptor fluid agitated at 100 rpm, and was maintained at 37 ± 0.5 °C throughout the experiments. The prepared formulation was placed in the membrane of the donor compartment. An aliquot of 2 mL sample was withdrawn at suitable time intervals and replaced immediately with an equal volume of fresh diffusion medium. The % drug release was calculated and a graph of % drug release against time was plotted, release studies were performed in triplicate for each formulation [Citation40]. Data were expressed as the cumulative amount of apigenin permeated through the membrane considering the total amount of drug applied of each formulation.
Release kinetics analysis
Release kinetics from the Con-A conjugated nanotransfersomal gel formulation were compared to different kinetic models. The models studied were: Zero order rate kinetics, first order rate kinetics, Higuchi’s kinetics. In this kinetic behaviour, the graph is plotted between the cumulative percent release with respect to time. In Ritger–Peppas exponential kinetics, the graph is between the log of cumulative percent drug release with respect to time [Citation41].
In-vitro skin retention and deposition study
The skin deposition and retention studies of various formulations were conducted in order to analyze the content of apigenin in the skin after 24 h of diffusion. At the end of the experiment, the skin mounted on the diffusion cell was removed safely, and the skin surface samples were washed up with distilling and methanol on both sides and carefully dried. For the assessment of drug deposition and retention in the skin, the cleaned skin was cut into small pieces, mashed completely in a beaker and 10 mL of 50% methanol was added to this mass. Finally, the beaker was mechanically shaken in a water bath shaker at 37 °C for 3 h for the complete isolation of the drug. After suitable dilution, the apigenin content in the filtrate was determined by UV spectroscopy [Citation42].
Ex-vivo skin permeation studies
Goatskin samples
Fresh Goatskin was collected from a local slaughterhouse. After thorough washing, the hair on the skin was removed using a surgical razor and the skin was separated from the underlying cartilage and subcutaneous fat using a scalpel and cut into appropriate sizes. The skin was stored in a formalin solution at 4 °C. The skin was first immersed in ultra-purified water at 60 °C for 2 min and the epidermis was then peeled off. Dried skin samples were kept under −20 °C for later use.
Assessment of permeation studies
The ex-vivo skin permeation analysis was performed to use the full-thickness goat skin through using modified fabricated Franz diffusion cell. The skin was cut with an effective diffusion area of 2.5 cm2 and clamped between the donor and the receptor chamber of diffusion cell. The receptor chamber was filled with freshly prepared phosphate buffer pH 5.5. The diffusion cell was maintained at 37 °C and the solution of the receptor chamber was stirred continuously at 500 rpm by using magnetic stirrer with a hot plate (Remi equipments, Mumbai). The formulation (2 ml) was gently placed in the donor chamber at 1, 2, 3, 4, 5, 6, 12, 18 and 24 h, 5.0 mL of the solution in the receptor compartment was removed and analyzed using UV- spectrophotometer and replaced immediately with an equal volume of fresh buffer. The permeation coefficient of goat skin (Kp) was calculated using the equation Kp = Jss/C, where C is the initial concentration of the drug in the formulation pertain to the donor phase [Citation37].
Histopathological evaluation of skin for photodamage
The animal skin was constantly examined for 6 weeks for the slow progress of photocarcinoma. The evaluation criterion was adopted from Kaur et al. [Citation32] with slight modifications. An evaluatory scale was developed according to the skin conditions from 0–5, with representations of normal skin at zero and severely damaged skin at 5. Further skin biopsies were taken every week from each group of animals and were embedded in paraffin after fixing in 10% buffered formalin. Then the sections were sliced with a semi Automated Rotary Microtome (Leica®, Germany.) and stained accordingly using Hematoxylin and Eosin (H and E) staining dyes. Finally, the stained specimens were observed under a Fluroscence microscope (Nikon®, India), in order to determine the extent of damage caused to the skin components [Citation43].
Myeloperoxidase assay
MPO is an enzyme which generates reactive oxidant species as part of its function in innate most defence mechanisms and critically expressed in UV irradiated skin. In brief, a snap frozen skins biopsy of size about 10–20 mm was harvested and incubated in hexadecyltrimethylammonium bromide containing phosphate buffer at a temperature of −80 °C. After incubation, the tissue samples were subjected to homogenization followed by sonication and centrifugation. Centrifugation helps in removing skin debris. The supernatant liquid left after centrifugation was analyzed spectrophotometrically for myeloperoxidase levels. A comparison of exposed tissue samples readings was done with the control group in order to correlate the results with the release profile of myeloperoxidase levels and their inhibition by the prepared formulations [Citation44].
Confocal cell uptake studies
The slides of skin penetration of Rhodamine 123 were then viewed under confocal laser scanning microscopy (CLSM) to study the internalization of apigenin loaded Con-A conjugated nanotransfersomal gel by the tissues at Osmania University, Hyderabad. After applying Goatskin, in vitro, to systems containing probe and Conjugated nanotransfersomes containing 0.03% rhodamine, they were used for the assessments. The conjugated nanotransfersomal gel was applied homogeneously and nonocclusively to skin tissue. The experiments were run in Franz diffusion cells and the receiver contained phosphate buffer pH 5.5 solution. After 24 h, the skin was removed and washed with phosphate buffer. The skin was then rapidly frozen by liquid nitrogen and a skin surface perpendicular rectangular piece was taken from the site of drug application with the help of a sharp blade. This skin tissue was fixed on the sample holder with the help of a Tissue frozen medium gel. The skin perpendicular sections (dermis to horny layer) of (250 µm) full thickness were cut with the help of cryomicrotome (Gung, Leica, Germany). The treated area was cut out and tested for probe penetration. The full skin thickness was optically scanned at 15–30 nm increments through the Z-axis of a Leica DMIRE2 confocal laser scanning microscope (Germany) attached to a Leica TCS SP2 fluorescence microscope. Optical excitation was carried out with a 488 nm argon laser-beam, and fluorescence emission was detected above 555 nm.
MTT Assay
The cells were seeded at a cell density of 105 cells/well into 96 well plates and human skin melanoma cell line A375 and skin keratinocyte cell line HaCaT cells were incubated for 24 h at 37 °C in an atmosphere of 95% air and 5% CO2. Cytotoxicity of cells to apigenin activity was determined by a standard spectrophotometric [3- (4, 5-dimethylthiazole-2-yl)-2, 5-diphenyl tetrazolium] MTTY assay. Then, 25 μL of apigenin, plain drug loaded gel and apigenin loaded conjugated nanotransfersomal gel, at different concentrations in PBS, was added to culture plates for 48 h. After treatment, cells were rinsed twice with PBS, and the serum-free culture medium without phenol red was replaced in all wells. Cells were then incubated for 4 h with the MTT solution (5 mg/mL). The yellow tetrazolium salt was metabolized by viable cells to purple crystals of formazan. The crystals were solubilized overnight in a mixture consisting of 20% sodium dodecyl sulfate (SDS) in HCl (0.01 M). The product was quantified spectrophotometrically by absorbance measurement at a 336 nm wavelength using a microplate reader. The cellular viability was expressed as the percentage of viable cells compared to the control group [Citation32].
Stability study of con-A conjugated nanotransfersomes
To assess the ability of developed formulations, sealed bottles of the freshly prepared Con-A conjugated nanotransfersomes were placed in a stability chamber and stored at room temperature and accelerated temperature and humidity (25 ± 2 °C, 60 ± 5% RH) and (40 ± 2 °C, 75 ± 5% RH) for up to 6 months. After 6 months of storage, the samples were evaluated for structural integrity for vesicles size, zeta potential and % entrapment efficiency [Citation32].
Statistical analysis
Data were presented as mean ± standard deviation and all experiments in this study were repeated at least three times. The results were statistically analyzed by analysis of variance ANOVA test; P values < .05 were considered as significant.
Results and discussion
Synthesis of conjugates
The successful synthesis of the conjugates was confirmed by IR and DSC study. The conjugation of Con-A to the nanotransfersomes was done through the crosslinking of EDC-NHS on the surface of transfersome. Con-A coupling to the nanotransfersomes was done by the carbodiimide technique. 1-Ethyl-3, 3-(dimethylaminopropyl) carbodiimide (EDC) is a water-soluble derivative of carbodiimide. N-Hydroxysuccinimide (NHS) is often used to assist the carbodiimide coupling in the presence of EDC. The reaction includes the formation of the intermediate active ester (the product of condensation of the carboxylic group and NHS) that further reacts with the amine function to yield finally the amide bond. IR studies confirmed the attachment of Con-A with nanotransfersomes, because the coupling of Con-A and transfersomes was dependent on the amide bond between NH2 group of Con-A and OH group of apigenin.
Morphology, vesicle size and zeta potential
TEM images showed in Figure1(A,B) exhibit that nanotransfersomes were smaller unilamellar with a well-identified spherical vesicle confirming the vesicular characteristics indicating Con-A conjugated did not affect the vesicles structural integrity. The vesicle size of Con-A conjugated nanotransfersomal gel was found to be increased as compared to nanotransfersomes which may be due to the presence of lectin coating on the formulation. The average vesicle size of nanotransfersomes and Con-A conjugated nanotransfersomal gel was 158.5 ± 0.25 nm and 179.0 ± 0.32 nm and polydispersity index was found to be 0.199 ± 0.03 and 0.197 ± 0.07, respectively and is graphically shown in . The zeta potential of Con-A conjugated nanotransfersomal gel was found to be increased positively as compared to nanotransfersomes, which is a property beneficial for interaction with the dermal tissues and bypass aggregation of vesicles and induced repulsive interaction between the vesicles [Citation12]. Zeta potential of nanotransfersomes and Con-A conjugated nanotransfersomal gel was 31.06 ± 0.33 mV and 35.1 ± 0.21 mV, respectively and results are reported in and graphically shown in .
Figure 1. (A) Transmission electron microscope images of apigenin loaded nanotransfersome and (B) Con-A conjugated nanotransfersomal gel, (C) Mean vesicle size of nanotransfersomes, (D) Mean vesicle size of Con-A conjugated nanotransfersomal gel, (E) Zeta potential of nanotransfersomes and (F) Zeta potential of Con-A conjugated nanotransfersomal gel.
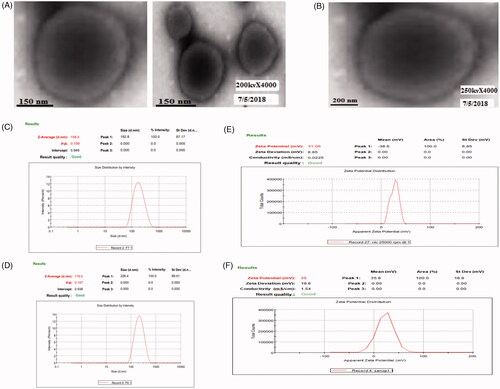
Table 1. Zeta potential, vesicles size and PDI of optimized formulations.
X-ray diffraction study
The X-ray diffraction, studied of pure drug apigenin, indicated an existence of sharp diffraction peak at 2θ value of 13.2°, 15.5°, 16.6°, 18° and 23°. Phospholipids showed characteristics peak 13.2° and 25.08° and Con-A displayed characteristics peak at 2.79° and 2.95°. A physical mixture of apigenin, phospholipid and Con-A in equal proportions (1:1:1) showed the appearance of newer peaks with low intensity. X-ray diffraction analysis of Con-A conjugated nanotransfersomes structure confirmed an amorphous nature which indicates the relatively low intensity observed in the nanotransfersomes. Possibly they are easy to be complexed and are graphically presented in .
Figure 2. (A) X-ray diffractogram of (a) Apigenin, (b) Con-A, (c) Phospholipids, (d) physical mixture, (e) Con-A conjugated nanotransfersomal gel. (B) FTIR spectra of (a) Apigenin, (b) Con-A, (c) Con-A conjugated nanotransfersomal gel. (C) DSC Thermogram of (a) Apigenin (b) Con-A conjugated nanotransfersomes (c) Con-A (d) Phospholipids (E) Carbodiimide. (D) In vitro drug release profile of Con-A conjugated nanotransfersomal gel, marketed product and pure drug suspension in skin pH 5.5.
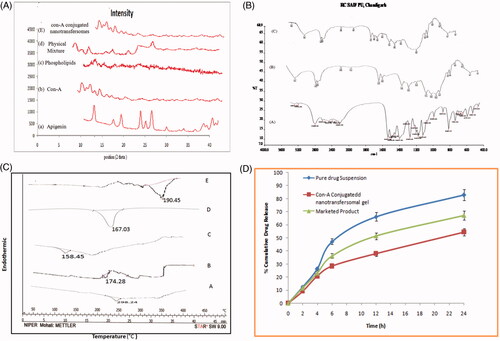
FTIR Spectroscopy Study
FTIR studies confirmed the conjugate of Con-A with apigenin loaded nanotransfersomes because coupling Con-A and nanotransfersomes depended on the amide bond between NH2 group of Con-A and OH group of apigenin. The IR spectrum of Con-A conjugated nanotransfersomal gel clearly indicated peaks (3471.1, 1735, 1690, 800–600 etc.) for amide revealing the presence of amide group in the formulation, while peaks for amide groups were absent in the IR spectrum of apigenin loaded nanotransfersomes. The FTIR spectra of apigenin showed characteristic peaks at 3550–3200 cm−1(O–H stretching), and the C–H stretching occurs above 3000 cm−1 with the aliphatic C–H stretching. The very strong bands in FTIR at 3287 cm−1 (C–H stretching) of aromatic group of apigenin and the C = O stretching bands are observed at in the region 1750–1735 cm−1 (C = O is stretching). The observed bands at 1651, 1500, 1354 and 1245 cm−1 in IR corresponds to ring modes, which are localized on the benzene part of the molecule but disappeared in the conjugated nanotransfersomal gel formulation, while the new peaks at 1559 cm−1 C–C stretching and 1607 cm−1 aromatic C–H bending) were appeared. Data are shown in .
DSC study
Thermodynamic data from DSC thermogram is shown a sharp melting for the pure drug apigenin, Con-A conjugated nanotransfersomes, Con-A, phospholipids and carbodiimide in . A sharp melting transition of the pure drug was observed at 226.98 °C and Con-A conjugated nanotransfersomes showed an endothermic peak at 174.28 °C. Con-A, phospholipids and carbodiimide showed an endothermic peak at 158.45 °C, 167.03 °C and 190.45 °C, respectively. DSC thermogram showed that the Peak intensity of heat enthalpy was reduced and slightly shifting in the melting point of drug which may be due to its solubility in the nanotransfersomes.
Con-A conjugation efficiency and percent entrapment efficiency
The quantity of Con-A bound to the apigenin loaded nanotransfersomes was determined and the amount of Con-A was estimated by using Folin–Ciocalteu reagent. The % conjugation efficiency of Con-A conjugated nanotransfersomal gel containing apigenin was calculated as 86.8 ± 1.06%. The quantity of apigenin entrapped within con-A conjugated nanotransfersomal gel was determined by ultracentrifugation. The unentrapped apigenin in nanotransfersomes dispersion was separated by a cooling centrifuge (Remi instruments Ltd., Mumbai, India) at 13,000 rpm, for 20 min at room temperature and the supernatant was decanted. The concentration of apigenin in the supernatant was determined using UV–visible spectrophotometer at λmax 336 nm (Perkin Elmer Lambda 25 double beam UV-Visible Spectrophotometer, Singapore). Each experiment was performed in triplicate [Citation38]. The entrapment efficiency of apigenin inside phospholipids to form Con-A conjugated nanotransfersomal was found to be 89.05 ± 0.62%. Entrapment efficiency was higher due to the higher concentration of phospholipids and lower concentration of surfactant. This may be accredited to an increase in density of transfersomes with a decrease in surfactant concentration and also transfersomes containing Tween-80 have higher entrapment than other vesicles containing Span-80 which may be agreed by other studies [Citation37].
Spreadability study
The spreadability value of con-A conjugated nanotransfersomal and marketed product was found to be 9.01 ± 0.32 g.cm sec−1 and 7.3 ± 0.33 g.cm sec−1, respectively, whereas the spreadability value of developed conventional gel formulations was found to be 6.5 ± 0.17 g.cm sec−1. All the developed formulations exhibited almost similar spreadability values. This observation revealed that the incorporation of drug loaded Con-A conjugated nanotransfersomal gel into the gel base did not affect the spreadability of novel gel formulations. This might be due to the small vesicles size range of drug loaded gel giving low spreadability values indicating easy spreading on the skin.
In-vitro drug release studies
The in-vitro release studies of the drug from the apigenin loaded Concanavalin conjugated nanotransfersomal gel, pure drug suspension and marketed product were investigated in vitro over a period of 24 h using a cellophane membrane and which followed a biphasic release. The finding of our results of % cumulative drug release curve of Concanavalin conjugated nanotransfersomal gel had the lowest amount of drug release, almost 54.46 ± 0.25, observed after 24 h as compared to other pure drug suspension and marketed product dispersion was 82.80 ± 0.34 to 67.16 ± 1.22, respectively. The outcomes exhibit that the formulation represents initial burst release of the surface-adsorbed drug, was observed about 10–15% within 2 h and which followed by slow diffusion. But after 2 h release of the drug from the conjugated nanotransfersomal gel, there was towards that showed the sustained release manner. They represent the in-vitro release suggesting the burst release of drug was due to availability of the free apigenin in the outer surface of the conjugated nanotransfersomes [Citation45]. The results of the drug release are reported in and .
Table 2. In vitro drug release profile of Con-A conjugated nanotransfersomal gel, marketed product and pure drug suspension in skin pH 5.5.
Determination of drug excipients interaction study
Absorbance data of drug excipients results are presented in for the interference of additives in the estimation of apigenin. Results observed showed that the excipients, polymer and other materials used in the preparation and conjugation of transfersomal gel did not show any interference in the estimation of the drug.
Table 3. Absorbance data for the interference of additives in the estimation of apigenin.
Release kinetics
The % drug release data from the apigenin loaded Con-A conjugated nanotransfersomal gel formulation was compared to pure drug suspension and marketed product from different kinetic models reported in . The results followed that the model was best fitted with data in the Higuchian equation (R2 = 0.992). The R2 value of first order for Con-A conjugated nanotransfersomal gel was near to one, thus; release of drug apigenin from nanotransfersomal gel formulations followed zero order release kinetic with R2 = 0.965 in Ritger–Peppas equation. The drug release from the Con-A conjugated nanotransfersomal gel is mostly by the diffusion and is best described by Fickian diffusion. But in case of a possible swelling in the system, other processes in addition to diffusion play an important role in exploring the drug release mechanisms. The observed drug release mechanism by diffusion from this system is described by Fick’s second law of diffusion [Citation41].
Table 4. Release behaviour of apigenin from Con-A conjugated nanotransfersomal gel formulations.
Ex-vivo skin permeation and skin deposition study
The ex-vivo skin permeation study was carried out for Con-A conjugated nanotransfersomal gel and the results of drug permeated release are reported in and values are represented as mean ± SD (n = 3). The cumulative amount of apigenin penetrated through the abdominal goatskin in all vesicle formulations was significantly (P < .05) higher than the nanotransfersomes and marketed product. The amount of apigenin permeated through the skin from the Con-A conjugated nanotransfersomal gel was significantly (P < .05) higher than that from a pure drug suspension and marketed product. Flux of Con-A conjugated nanotransfersomal through goatskin was 8.42 ± 0.73 and % drug retention was 0.91 ± 0.02, while pure drug suspension and marketed product were significantly obtained lower transdermal flux and percent drug retention. The nanosize, as well as the influence of Con-A conjugated lectins, were able to better penetrate to the entire depth of skin and also surfactant swells the stratum corneum and the intact vesicle can penetrate into and through the intact skin cells. So this permeation is required for the fabricated formulation for the efficacy of skin cancer [Citation42].
Table 5. Permeation and % drug retention data for apigenin loaded Con-A conjugated nanotransfersomal gel, marketed and pure drug suspension across abdominal goat skin.
Table 6. Effect of apigenin, unloaded and apigenin-loaded Con-A conjugated nanotransfersomal gel on cellular viability (as % of control) of MTT assay on (a) HaCaT cells and (b) A375 cells.
Histopathological studies
The main objective of the Histopathological evaluation was to determine the targeting ability of Mice skin of the control group (group-I), UV-Irradiated mice skin of control group (group-II), UV-Irradiated mice skin of PDS group (Group-III) in comparison with conjugation-NTG (group-IV) and Marketed product (group-V) dermally to evaluate its topical characteristics and their effect on skin. The macroscopic effects of UV radiation on the animal’s skin were highly distinguishing for evaluation shown in (). The non-irradiated skin was free from any type of lesion formation as shown while the irradiated groups developed lesion from the fourth week onwards in nearly 50% of the test animals as shown in . At the end of 4 weeks about 85% of animals developed photocarcinoma lesions. (extensive degradation). The cellular components like collagen, elastin, matrix proteins network were found damaged. The animals treated with free apigenin gel developed lesser skin lesions in comparison to the irradiated group as shown in . The animals treated with market formulation (Flonida cream, Shalak Pharmaceutical Ltd, Karampura New delhi), as shown in , showed minimal lesion formation at the last week of the irradiation. , shows the skin section after 4 weeks treatment with the Con-A conjugated nanotransfersomal gel showed on discontinuation of the UV irradiations the formulation showed healing effect. The test samples showed nominal skin degradation and negligible lesion formation. So it showed that no changes such as skin irritation or toxicity in case of edema and erythema of the treating skin.
Figure 3. (A) (a) Showing the photomicrograph of the Mice skin of control group, (b) UV-Irradiated Mice skin of control group, (c) UV-Irradiated Mice skin of PDS group, (d) UV-Irradiated Mice skin of marketed formulation (Flonida, Shalak Pharmaceutical India) group, (e) UV- Irradiated Mice skin of Con-A Conjugated NTG group. (B) Showing the effect of all prepared formulations on the level of increase of MPO. (C) CLSM image showing the depth of skin penetration of Rhodamine red (florescence marker) after 24 h into the goat skin surface at 250 µm (a) (PDS), (b) MP, (c) CCNTG. (D) effect of apigenin, unloaded and apigenin-loaded Con-A conjugated nanotransfersomal gel on cellular viability (as % of control) of MTT assay on HaCaT Cells (a) and A375 cells (b).
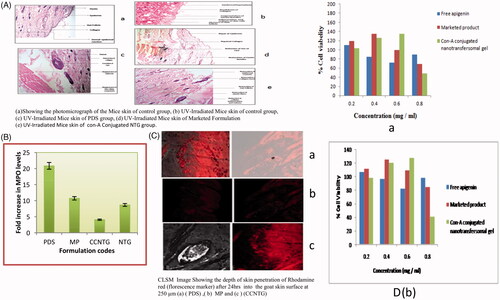
Myeloperoxidase assay
The application of MP reduced neutrophil activation did not reduce neutrophil infiltration. But, the application of CCNTG reduced neutrophil activation as well as neutrophil infiltration also. While there were no such effects observed when pure drug suspension was applied topically to the animals. It may be due to the poor penetration ability of formulation. Although topical application of PDS, MP reduced edema, they failed to decrease the dermal neutrophil numbers, when compared with the control and standard groups. Topical treatment of PDS MP did not reduce neutrophil activation as measured by myeloperoxidase levels, while there was a significant decrease in the neutrophil activation in case of CCNTG (P = 0.04 − 0.056) as compared with standard (P = .05) and control (P = .01). The capability of CCNTG to reduce myeloperoxidase without disturbing neutrophil homing implies that CCNTG are selective in inhibiting the neutrophil activation and the data are shown in .
Confocal laser scanning microscopy analysis
The localization of a vesicular penetration measured by CLSM study was carried out for three formulation, Con-A conjugated nanotransfersomal gel, PDS and marked product (Flonida cream, Shalak Pharmaceutical Ltd, Karampura New delhi) and graphs reported in . The images of the Con-A conjugated nanotransfersomal gel have penetrated across the depth into the cell observed the internalization of vesicles well within the skin cell. Showed that the lesser extent interlunation within marketed product and a pure drug suspension. However, it is apparent that the intact con-A conjugated nanotransfersomal gel was retained in the treated skin leading to comparatively higher penetration into deeper tissues than marketed product probably due to the rigid nature of nanotransfersomal gel. Moreover, low fluorescence intensity was recorded with the marketed product and pure drug suspension as compared to Con-A conjugated nanotransfersomal gel, which reveals that higher the cumulative amount of drug was permeated from Con-A conjugated nanotransfersomal [Citation46,Citation47].
Cytotoxicity studies
Cytotoxicity experiments were carried out on HaCaT cells and A375 cells lines to examine the effect on cell proliferation of apigenin as well as the marketed product and apigenin-loaded conjugated nanotransfersomal gel. Cytotoxicity assay showed that apigenin-loaded conjugated nanotransfersomal gel has significant toxicity achievements A375 compared to its low toxicity towards HaCaT as shown in and images are shown in . The incubation with apigenin for 48 h induced a concentration-dependent inhibition of cell proliferation as determined by MTT assay. For this study, the amount of apigenin present in apigenin loaded conjugated nanotransfersomal gel was equivalent to 0.4892/5 mg. The inhibition was 18.28, 23.34 and 32.50% at 100, 200 and 400 µg/mL of apigenin, respectively. The evaluation of unloaded and apigenin-loaded conjugated nanotransfersomal gel cytotoxicity demonstrated that, until the concentration corresponded to 200 µg/mL of apigenin, the formulations protected against apigenin cytotoxicity since no inhibition of cell proliferation was observed. Nevertheless, for the highest concentration studied, the reduction in cell viability was about 60% for drug-loaded nanoemulsion gel, due to the sum of the cytotoxic effects of both apigenin and marketed product. MTT Results showed that conjugated nanotransfersomal gel has retained the specificity of apigenin towards cancer cells without affecting the normal cells.
Stability studies
Stability of the formulated Con-A conjugated nanotransfersomes was found out of certain physicochemical properties like vesicles size, zeta potential, PDI and % entrapment efficiency and analyzed for up to 6 months. The stability studies showed that there was negligible increase in the vesicle size from 179.0 ± 0.32 nm to 179.9 ± 0.74 nm and PDI was found in slightly changes from 0.197 ± 0.07 and 0.198 ± 0.24 during the storage at room temperature and accelerated temperature and humidity. The initial % EE of the conjugated nanotransfersomes was found to be 89.05 ± 0.62%. After 6 months of storage at the same temperature conditions, it was found to be 89.05 ± 0.62% to 90.27 ± 0.21%. In contrast, there were no significant changes in the % EE during storage of formulation for 6 months. Zeta potential with slight reduction from 35.1 ± 0.21 mV to 34.69 ± 0.85 mV after 6 months storage at same temperature conditions. Thus, Con-A conjugated nanotransfersomal gel was found to be stable at room temperature and accelerated temperature and humidity for up to 6 months and results are represented in .
Table 7. Storage stability studies of the Con-A conjugated nanotransfersomal gel at different time interval and temperature conditions.
Conclusion
In this research study, Con-A conjugated nanotransfersomal gel was successfully synthesized by carbodiimide method with having targeted ability for better entrapment efficiency and evaluated against skin cancer by using two different cell lines. The characteristic bands of FTIR spectra certainly indicate the physico-chemical stability of the drug inside the formulation and apigenin can be attached to Con-A conjugated nanotransfersomes without altering their structural integrity. The conjugated nanotransfersomal gel was able to improve both in in-vitro and in-vivo permeation and skin deposition of apigenin compared to the marketed formulations and retentive in the entire depth of skin for a sustainable action. These conjugates significantly improved the therapeutic efficacy and safety and possessed small vesicles sizes, and in vitro drug release study indicated the prolongation sustained release. In vivo imaging and pharmacokinetic studies revealed that conjugates had varying degrees of prolonged circulation and exhibited the lowest toxicity. Con-A conjugated nanotransfersomal gel showed reduced cytotoxicity on A375 cells as compared to HaCaT cell lines and the histological studies confirm that this approach could be used for combating the deleterious effects of ultraviolet radiations. It is concluded that developed natural drug conjugated delivery system could possibly potential effective for skin malignant melanoma.
Acknowledgement
The authors acknowledge University Grant Commission-SAP, New Delhi, India, for the instrumental facility. One of the authors extend their gratitude towards the head of the cosmetic lab, Institute of Pharmacy, Pt. Ravishankar Shukla University, Raipur (C.G.) for providing facilities to carry out research work. The author also wants to thank library of Pt. Ravishankar Shukla University for providing e-resources available through UGC-INFLIBNET.
Disclosure statement
The authors have reported no conflict of interest.
References
- Kwona K, Hanb B, Parka K et al. Analysis on the current status of targeted drug delivery to tumours. J Control Release. 2012;164(2):1–18.
- Kamboj S, Saini V, Bala S, et al. Vesicular drug delivery systems: a novel approach for drug targeting. Int J Drug Deliv. 2013;5(2):121–130.
- Li J, Wang Y, Tao J et al. Recent advances in targeted nanoparticles drug delivery to melamona. Nanomedicine. 2015;11:769–794.
- Naves LB, Dhand C, Reddy J, et al. Nanotechnology for the treatment of melanoma skin cancer. Prog Biomater. 2017;6:13–26.
- Fohona SC, Bi-Botti CY. Current status of lectin-based cancer diagnosis and therapy. AIMS Molecular Sci. 2017;4(1):1–27.
- Sina A, Roy A, Annabi B. The lectin Concanavalin-A signals MT1-MMP catalytic independent induction of COX-2 through an IKKy/NF-kB- dependent pathway. J Cell Commun Signal. 2010;4:31–38.
- Lei HY, Chang CP. Induction of autophagy by Concanavalin–A and its application in antitumour therapy. Autophagy. 2007;4:402–404.
- Liu B, min MW, Bao JK. Induction of apoptosis by Concanavalin-A and its molecular mechanisms in cancer cells. Autophagy. 2009;5:432–4334.
- Zheng SH, Jie C, Chun-yang L. Antitumour effects of Concanavalin-A and Sophora flavescens lectin in vitro and in vivo. Acta Pharmacol Sinica. 2014;35:248–256.
- Fonseca JV, Bentley LB, Iyomasa MM, et al. Evaluation of protective effect of a water-in-oil microemulsion incorporating quercetin against UVB-induced damage in hairless mice skin. J Pharm Pharmaceut Sci. 2010;13(2):274–285.
- Rai S, Pandey V, Rai G. Transfersomes as versatile and flexible nanovesicular carriers in skin cancer therapy: the state of the art. Drug Deliv. 2017;8:1–18.
- Avadhani KS, Manikkathi J, Tiwari M, et al. Skin delivery of epigallocatechin-3-gallate (EGCG) and hyaluronic acid loaded nanotransfersomes for antioxidant and anti-aging effects in UV radiation induced skin damage. Drug Deliv. 2017;24(1):61–74.
- Saraf S, Deep C. Herbal photoprotective formulations and their evaluation. Open Nat Prod J. 2009;2:71–76.
- Saraf S, Jangdey MS, Gupta A, et al. Development and optimization of Apigenin-loaded transfersomal system for skin cancer delivery: in vitro evaluation. Artif Cells Nanomed Biotechnol. 2017;45(7):1452–1462.
- Zhang Z, Cui C, Wei F et al. Improved solubility and oral bioavailability of apigenin via Soluplus/pluronic F127 binary mixed micelles system. Drug Dev Ind Pharm. 2017;43(8):1276–1282.
- Saraf S, Gupta A, Alexander A, et al. Advancements and avenues in nanophytomedicines for better pharmacological responses. J Nanosci Nanotechnol. 2015;15:1–10.
- Yan X, Zhan Y, Shao H, et al. Apigenin in cancer therapy: anti-cancer effects and mechanisms of action. Cell Biosci. 2017;7(50):1–16.
- Sung B, Chang HY, Kim ND. Role of apigenin in cancer prevention via the induction of apoptosis and autophagy. J Cancer Prev. 2016;4:216–226.
- Zhao G, Han X, Cheng W et al. Apigenin inhibits proliferation and invasion and induces apoptosis and cell cycle arrest in human melanoma cells. Oncol Rep. 2016;37:2277–2285.
- Lee YM, Lee G, Kim BM et al. Inhibition of glutamine utilization sensitizes lung cancer cells to apigenin-induced apoptosis resulting from metabolic and oxidative stress. Int J Oncol. 2016;48:399–408.
- Angulo P, Kaushik G, Subramaniam D, et al. Natural compounds targeting major cell signalling pathways: a novel paradigm for osteosarcoma therapy. J Hematol Oncol. 2017;10(1):1–10.
- Xu M, Wang S, Song YU et al. Apigenin suppresses colorectal cancer cell proliferation migration and invasion via inhibition of the Wnt/beta-catenin signaling pathway. Oncol Let. 2016;11:3075–3080.
- Bilia AR, Guccione C, Manconi M, et al. Vesicles and micelles: two versatile vectors for the delivery of natural products. J Drug Deliv Sci Technol. 2016;32:241–255.
- Manconi M, Cencetti C, Nacher A et al. Nanodesign of new self-assembling core-shell gellan transfersomes loading baicalin and in vivo evaluation of repair response in skin. Nanomedicine: Nanotechnol, Biol Med. 2018;14(2):569–579.
- Menezes AC, Campos PM, Euleterio C et al. Development and characterization of novel 1-(1-Naphthyl) Piperazine loaded lipid vesicles for prevention of UV-induced skin inflammation. Eur J Pharm Biopharm. 2016;104:101–109.
- Kotla NG, Rooney P, Pandit A, et al. Biomimetic lipid-based nanosystems for enhanced dermal delivery of drugs and bioactive agents. ACS Biomater Sci Eng. 2017;3:1262–1272.
- Cevc G, Vierl U. Nanotechnology and transdermal route: a state of the art review and critical appraisal. J Control Release. 2010;141(3):277–299.
- Mirpalomo S, Nacher A, Dies-sales O, et al. Inhibition of skin inflammation by baicalin ultradeformable vesicles. Int J Pharm. 2016;511(1):23–29.
- Jain S, Jain P, Umamaheshwari RB, et al. Transfersomes a novel vesicular carrier for enhanced transdermal delivery: development, characterization and performance evaluation. Drug Dev Ind Pharm. 2003;29:1013–1026.
- Saraf S, Jangdey MS, Gupta A. Fabrication, in-vitro characterization and enhanced in-vivo evaluation of carbopol based nanoemulsion gel of apigenin for UV induced skin carcinoma. Drug Deliv. 2017;24(1):1026–1036.
- Grantab R, Sivananthan S, Tannock IF. The penetration of anticancer drugs through tumour tissue as a function of cellular adhesion and packing density of tumour cells. Cancer Res. 2016;66:1033–1039.
- Gupta A, Kaur CD, Saraf S. Formulation, characterization, and evaluation of ligand-conjugated biodegradable quercetin nanoparticles for active targeting. Artif Cells Nanomed Biotechnol. 2016;44(3):1–11.
- Cevc G, Grbauer D, Schatzlein A, et al. Ultraflexible vesicles, transfersomes, have an extremely low pore penetration resistance and transport therapeutic amounts of insulin across the intact mammalian skin. Biochim Biophys Acta. 1998;1368:201–215.
- Abdallah MH Transfersomes as a transdermal drug delivery system for enhancement the antifungal activity of Nystatin. Int J Pharm Sci. 2013;5(4):560–567.
- Damink O, Dijikstra PJ, Feijen J, et al. Cross-linking of dermal sheep collagen using a water-soluble carbodiimide. Biomaterials. 1996;17(8):765–773.
- Jain SK, Jangdey MS. Lectin conjugated gastro-retentive multiparticulate delivery system of clarithromycin for the effective treatment of Helicobacter pylori. Int J Mol Pharmaceut. 2009;6:295–304.
- Khan MA, Pandit J, Sultana Y, et al. Novel carbopol-based transfersomal gel of 5-fluorouracil for skin cancer treatment: in vitro characterization and in vivo study. Drug Deliv. 2014;22:795–802.
- Saraf S, Paliwal S, Saraf S. Sphingosomes a novel approach to vesicular drug delivery. Int J Cur Sci Res. 2011;1:63–68.
- Aiyalu R, Govindarjan A, Ramasamy A. Formulation and evaluation of topical herbal gel for the treatment of arthritis in animal model. Braz J Pharm Sci. 2016;52(3):493–507.
- Garg T, Goyal AK, Marwah A, et al. Permeation enhancer strategies in transdermal drug delivery. Drug Deliv. 2015;23(2):564–578.
- Siepmann J, Peppas NA, Modelling of drug release from delivery systems based on hydroxypropyl methylcellulose (HPMC). Adv Drug Deliv. 2001;48:139–157.
- Shen LN, Zhang YT, Wang Q, Feng NP. Enhanced in vitro and in vivo skin deposition of apigenin delivered using ethosomes. Int J Pharm. 2014;460:280–288.
- Das S, Das J, Paul A, et al. Efficacy of PLGA-loaded apigenin nanoparticles in Benzopyrene and ultraviolet-B induced skin cancer of mice: mitochondria mediated apoptotic signalling cascades. Food Chem Toxicol. 2013;62:670–680.
- He S, Cen B, Zhang Z. A tumour-targeting cRGD-EGFR siRNA conjugate and its anti-tumour effect on glioblastoma in vitro and in vivo. Drug deliv. 2017;24(1):471–481.
- Mukerje A, Vishwanatha JK. Characterization and evaluation of curcumin-loaded PLGA nanospheres for cancer therapy. Anticancer Res. 2009;29:3867–3876.
- Ahad A, Mohammed A, Kohli K, et al. Formulation and optimization of nanotransfersomes using experimental design technique for accentuated transdermal delivery of valsartan. Nanomedicine. 2012;8:237–249.
- Sarwa KK, Rudrapal M, Mazumder B. Topical ethosomal capsaicin attenuates edema and nociception in arthritic rats. Drug Deliv. 2013;22:1–10.