Abstract
In this study, an attempt has been made to evaluate the effect of products of β-galactosidase (βGS) catalyzed reaction i.e. glucose and galactose and their structurally related compound vitamin C (VC) on the catalytic activity of native and PANI-CS-NC and PANI-CS-Ag-NC adsorbed βGS. Results indicated a decline in catalytic activity of soluble enzyme in the presence of all investigated compounds. The order of inhibition was found to be VC < glucose < galactose. However, the immobilized preparations were found more resistant to inactivation caused by the added compounds. About 48% activity was retained by PANI-CS-Ag-NC-βGS in the presence of galactose (5%, w/v), while the native enzyme exhibited only 18% of its original activity. A significant decrease in absorbance and fluorescence intensity was evaluated in soluble enzyme incubated in the presence of all investigated compounds. Three-dimensional fluorescence graphs, CD and FT-IR spectroscopic studies illustrated noteworthy conformational changes in the secondary structure and microenvironment of the soluble enzyme in the presence of VC and tested sugars. These results suggest that both PANI-CS-NC and PANI-CS-Ag-NC bound βGS are more resistant to the exposure caused by the higher concentration of added glucose, galactose, and VC and, therefore, can be effectively utilized for the production of a hassle-free lactose nano-biosensor.
GRAPHICAL ABSTRACT
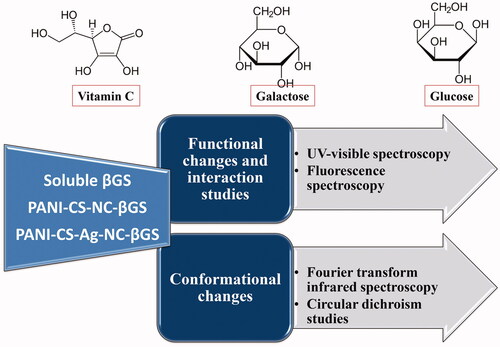
Introduction
β-galactosidase, βGS (β-d-galactoside galactohydrolase, E.C. 3.2.1.23) is an important hydrolytic enzyme that presents numerous interesting applications in the food industry. Its major application is to hydrolyze lactose in milk or derived products [Citation1,Citation2]. Lactose is the chief sugar (4–5%) present in milk and its hydrolysis makes milk and its products fit for consumption to lactose intolerant people [Citation3]. βGS has been obtained from a variety of microorganisms and sources including fungi, bacteria, yeasts, plants, etc. The microbial sources represent the major group because the enzymes derived from other sources are of high cost and of low-production yield [Citation4]. The hydrolyzed lactose-free products thus formed have enhanced sweetness, solubility and flavour [Citation5,Citation6].
However, free enzymes cannot be used directly in biotechnological industries due to the long processing time, difficult recovery and non-reusability [Citation7]. Considerable research endeavours have been made to circumvent the aforementioned problems associated with the utilization of free enzymes. Immobilization of enzymes is a widely used technique that provides a number of advantages over the use of soluble enzymes. It allows continuous and automated processing, easier separation of reaction products, easy recovery and reutilization of the enzyme, making enzyme-based processes cost-effective and industrially viable [Citation8–10]. Various methods for immobilization include physical adsorption, covalent attachment, entrapment etc.
Recently, with the rapid development of nanotechnology, NM has attracted enzymologists as a versatile enzyme immobilization support due to their large specific surface area, tailor-made size at the nanometer scale and similar size with enzyme molecules [Citation11–13]. Nano-immobilization can provide a solution to the demerits possessed by the conventional supports used for immobilization like the poor yield of immobilization and steric hindrances caused by the substrate [Citation14,Citation15]. Until now, various nanostructures, such as nanoparticles [Citation16,Citation17], nanofiber [Citation18], nanotube [Citation19], nanosheet [Citation20] and most recently, nanoflowers [Citation21] have been explored as support for the immobilization of industrially important enzymes [Citation22]. Among them, polymer-based NCs have attracted considerable attention in the last few years due to their exceptional stability against chemical, electrical and mechanical exposure [Citation23,Citation24].
Galactose is one of the products of βGS catalyzed reaction and also a well known competitive inhibitor (and rarely mixed inhibitor) for the majority of β-galactosidases. Several studies have been conducted showing the inhibition of βGS by the reaction products galactose and glucose [Citation25,Citation26]. For the first time, Park and Oh [Citation27] have shown that both galactose and glucose exhibit an uncompetitive inhibition. Geven et al. [Citation28] purified this enzyme from thermoacidophilic Alicyclobacillus acidocaldarius subsp. Rittmannii isolated from Antarctica. The enzyme was greatly inhibited by the reaction product galactose using substrates, o-nitrophenyl β-galactopyranoside (ONPG) and lactose. Lineweaver-Burk kinetics showed that galactose is a linear mixed-type inhibitor.
In this study, an effort has been made to systematically investigate the effect of substrate, products and VC on the activity of free and polyaniline chitosan nanocomposite (PANI-CS-NC) and polyaniline chitosan silver nanocomposite (PANI-CS-Ag-NC) bound βGS. This work mainly focuses on the effect of ascorbic acid, glucose, and galactose on the structural and functional integrity of soluble and immobilized βGS preparations. The interactions between the enzymes and the added compounds along with conformational changes in the protein were examined with the help of UV-visible, fluorescence, Fourier-transform infrared (FT-IR) and circular dichroism (CD) spectroscopic studies.
Materials and methods
Materials
Aspergillus oryzae βGS (8.4 U mg−1 solid) was obtained from Sigma-Aldrich Chemicals Co. St. Louis, Missouri, USA. ONPG, sodium carbonate, glucose, galactose and ascorbic acid were purchased from Sisco Research Laboratories (SRL), Mumbai, India. Chitosan, silver nitrate, hydrazinium hydrate, hexadecyl-trimethyl ammonium bromide surfactant, ammonium persulphate and hydrochloric acid were obtained from Thermo Fisher Scientific, Waltham, Massachussets, USA. All solvents and other reagents were of analytical grade and supplied by SRL.
Methodology
Synthesis of PANI-CS-NC and PANI-CS-Ag-NC
The synthesis of PANI-CS-NC and PANI-CS-Ag-NC has already been described earlier [Citation24].
Immobilization of β-galactosidase on PANI-CS-NC and PANI-CS-Ag-NC
The immobilization of βGS on these NCs has been previously mentioned [Citation24].
Effect of glucose, galactose and VC on the activities of soluble and immobilized β-galactosidase preparations
Soluble and immobilized βGS preparations (0.12 U) were independently incubated with various concentrations of sugars (1.0–5.0%, w/v) and ascorbic acid (0.01 mg mL−1– 0.1 mg mL−1) in 0.1 M sodium acetate buffer, pH 4.5 for 1 h at 37 °C. The effect of these effectors on the activity of soluble and immobilized enzyme preparations was monitored by conducting routine βGS assay at 37 °C for 15 min. The activity of the enzyme without these added effectors was taken as control (100%) for the calculation of residual percent activity. The enzyme activity was estimated using UV-1800 spectrophotometer, Shimadzu, Japan at 405 nm.
Spectroscopic studies
UV-visible spectroscopy
The spectral changes in the free and immobilized βGS preparations were monitored using the instrument (Shimadzu, Kyoto, Japan, UV-visible spectrophotometer 1800). The spectra of soluble, PANI-CS and PANI-Ag-CS-NC bound enzyme were compared in the presence of different investigated compounds. βGS was mixed with varying concentrations of VC, glucose and galactose in order to examine their interaction with the free and immobilized enzyme. Concentrations of all effectors have been mentioned in the above section. Detailed parameters have been previously described [Citation29].
Fluorescence measurements
Fluorescence measurements were recorded on a Shimadzu RF6000 spectrofluorophotometer, Japan. For fluorescence intensity measurements, 1 mg mL−1 βGS solution was mixed with varying concentrations of VC, glucose and galactose. The concentrations of all modulators have been previously described [Citation29].
Three-dimensional fluorescence spectroscopy analysis
These studies were performed to visualize the structural changes taking place in βGS in the presence of all the effectors before and after immobilization [Citation29].
The changes in the molecular structure of soluble and immobilized βGS were also analyzed with the help of FT-IR studies, using Perkin Elmer instrument, Waltham, Massachusetts, USA, Spectrum- II, wave number 4000–400. For FT-IR, defined amounts of treated and untreated soluble and immobilized enzymes (liquid samples) were incubated with added sugars and VC. The concentrations of enzymes and added compounds used have already been described in Section “Activity assay of β-galactosidase” [Citation29].
Circular dichroism studies
The changes in the secondary structure of the native and immobilized enzyme in the presence of added VC, glucose and galactose were evaluated by CD spectroscopy (Jasco J-815 CD spectropolarimeter, Jasco, Tokyo, Japan). For soluble, PANI-CS and PANI-Ag-CS-NC immobilized βGSs, 0.012 U were taken for all comparative measurements. The α-helical and β-sheet contents in the respective protein have been calculated by the K2D3 software [Citation29].
Activity assay of β-galactosidase
The activity of βGS was determined using o-nitrophenyl-D-galactopyranoside (ONPG) as a substrate and by measuring the release of o-nitrophenol from the substrate at 405 nm [Citation26].
Protein estimation
Protein concentration was calculated according to the method described by Lowry et al. [Citation30]. Bovine serum albumin was employed as standard.
Statistical analysis
Each value represents the mean for three independent experiments performed in duplicates, with average standard deviations, ≤5%. The statistical analysis for comparison between free and both immobilized enzyme preparations was performed by One Way ANOVA test. The data expressed in various experimental studies were plotted using origin 6.1 and p values ≤.05 were considered as statistically significant.
Results and discussion
Effect of glucose, galactose and VC on the activities of soluble, PANI-CS-NC and PANI-CS-Ag-NC bound β-galactosidase preparations
The two sugars, glucose and galactose are the products of βGS catalyzed reaction. Therefore, it is essential to portray the effect of high concentrations of these sugars on the catalytic activity of native and immobilized βGS. UV-visible spectrophotometry was performed to examine the effect of such compounds on the activity of βGS. Galactose competes with substrate for active sites and its competitive inhibitory role has already been described earlier [Citation31]. Table S1 depicts the relative activity of soluble and immobilized βGS in the presence of equimolar concentrations (1–5%, w/v) of both sugars. The suppression of βGS activity was found to be dose dependent and in order of glucose < galactose. PANI-CS-NC-βGS and PANI-CS-Ag-NC-βGS retained 28 and 48% of their original activity in presence of 5% (w/v) galactose, while the free enzyme could maintain only 18% of its activity under similar experimental conditions.
The soluble enzyme exhibited 57% of its activity in the presence of 5% (w/v) of glucose. Immobilization had a positive effect on the activity of PANI-CS-NC-βGS and PANI-CS-Ag-NC-βGS. PANI-CS-NC-enzyme retained 69% activity, while PANI-CS-Ag-NC-βGS was found more resistant to inhibition mediated by added glucose and reserved 83% of activity under identical incubation conditions. The presence of silver ions in PANI-CS-Ag-NC-βGS somehow protects the active site of enzyme by preventing alterations in conformation. Free enzyme undergoes unfavourable conformational changes under denaturing conditions which is responsible for the decreased activity of the enzyme [Citation32]. The immobilized enzyme showed marked resistance in inhibition caused by the tested compounds which might be due to conformational changes occurring outside the active site of the enzyme after binding to NC. Silver-containing NC bound βGS appeared more rigid to inhibition caused by higher concentrations of such compounds and retained a greater fraction of catalytic activity.
Table S2 demonstrates inhibition constants (Ki) for soluble and immobilized βGS incubated in the presence of higher concentrations of glucose and galactose. Ki values for βGS bound to NC containing Ag in the presence of glucose and galactose were found to be on the higher side which infers that this enzyme preparation is significantly more resistant to the added compounds. VC is one of the compounds found in milk and it is structurally related to glucose and galactose. Therefore, we have evaluated the effect of VC on the activity of free and immobilized βGS (Table S3). The soluble enzyme lost about 28% of its initial activity in the presence of 0.1 mg mL−1 of VC whereas it had a little effect on the activity of PANI-CS-NC-βGS and did not influence the activity of PANI-CS-Ag-NC-βGS.
Spectroscopic studies
UV-visible spectroscopy
To further develop this study, UV-visible spectra were recorded in order to determine the interaction of these products of the enzyme-catalyzed reaction and VC with native and immobilized βGS. depicts UV-visible spectra for soluble and PANI-CS-NC-βGS and PANI-CS-Ag-NC-βGS in presence of glucose, galactose and VC. βGS exhibited λmax at 280 nm after exposure to a maximum concentration of the investigated compounds. Native and immobilized enzyme displayed a hypochromic effect. A peak shift was observed only in case of PANI-CS-Ag-NC-βGS in the presence of VC. The order of hypochromicity was similar to that observed for the inhibitory effect, i.e. VC < glucose < galactose. The most prominent changes in absorbance spectra were recorded for free enzyme in the presence of added compounds with respect to control. The recorded spectra for PANI-CS-Ag-NC-βGS showed least hypochromic effect. It indicates stronger binding of such compounds with the soluble enzyme as compared to immobilized βGS. This corresponds to tabulated data of activity retention, where the free enzyme was found to be most affected by the addition of compounds. Since humans are increasingly exposed to various food colorants like carmoisine, the binding of carmoisine and its inhibitory effect on amyloid fibrillation in lysozyme have been recently investigated. The results exhibited a decrease in the absorbance of lysozyme on addition of carmoisine. However, there was no shift in the position of maximum wavelength, λmax [Citation33].
Fluorescence emission spectroscopy
Fluorescence spectroscopy is one of the most excellent techniques to investigate the binding of bioactive molecules to proteins as it is more sensitive compared to other optical methods [Citation34,Citation35]. Three amino acid residues; viz, tryptophan (Trp), tyrosine (Tyr) and phenylalanine (Phe) are primarily responsible for the intrinsic fluorescence of βGS. In fact, the intrinsic fluorescence of βGS is mainly contributed by tryptophan, because Phe has a very low quantum yield and the fluorescence of Tyr is almost totally quenched if it is ionized, or near an amino group, a carboxyl group, or a Trp. This viewpoint has been supported by the experimental observations made by other researchers [Citation36,Citation37].
Fluorescence measurement studies were carried out to understand the interactions between hydrolyzed products and native and immobilized βGS. For this purpose, the intrinsic fluorescence spectra of βGS in the presence of added compounds were recorded. When βGS was excited at 380 nm, it exhibited a strong emission peak at 436 nm. Upon the addition of VC, glucose and galactose to βGS solution, decreased fluorescence intensity was observed with no significant shift in its emission maxima which was observed at 436 nm (. However, in immobilized βGS preparations, the quenching was apparently reduced inferring less interaction taking place between βGS bound to PANI-CS-NC and the added compounds. Again, Ag containing NC bound βGS demonstrated the least decrease in fluorescent intensity in the presence of all investigated compounds. Tryptophan fluorescence is known to be more sensitive to the polarity of its local environment [Citation38]. Quenching in fluorescence has been previously reported by various researchers to validate the interactions between protein and added compounds [Citation33,Citation39].
Three-dimensional fluorescence analysis
Three-dimensional fluorescence spectroscopy is an informative tool to study conformational changes in biomolecules, especially proteins. The decrease in peak intensities or change in the appearance of peaks in the 3 D graph indicates the presence of effectors causing conformational changes in the protein [Citation40]. The 3 D spectra and corresponding contour diagrams for free and immobilized βGS in presence of VC, glucose and galactose have been shown in . As illustrated in figures, 3 D spectra of βGS exhibited three typical peaks, Rayleigh scattering peak (peak A) and two fluorescence peaks (peak B and C). Out of these two peaks, peak B indicates spectral characteristics of Trp and Tyr residues of protein whereas, peak C reveals polypeptide backbone structure of βGS and the intensity of this peak is correlated with βGS secondary structure [Citation41].
Figure 3. 3D fluorescence spectra and corresponding contour map of soluble and immobilized βGS in presence of VC. The spectra illustrate (a), (b) and (c) for controls of soluble, PANI-CS-NC-βGS, and PANI-CS-Ag-NC- βGS and (a′), (b′) and (c′) for native, PANI-CS-NC-βGS, and PANI-CS-Ag-NC-βGS treated with VC.
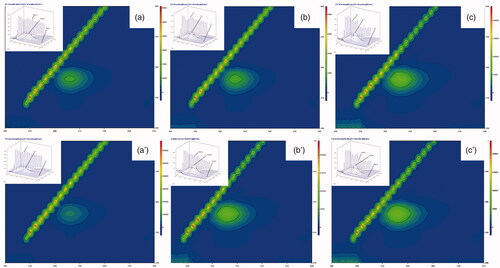
Figure 4. 3D fluorescence spectra and corresponding contour map of soluble and immobilized βGS in presence of glucose. The spectra show (a), (b) and (c) for controls of soluble, PANI/CS-NC-βGS and PANI-CS-Ag-NC-βGS and (a′), (b′) and (c′) for native, PANI/CS-NC-βGS, and PANI-CS-Ag-NC-βGS.
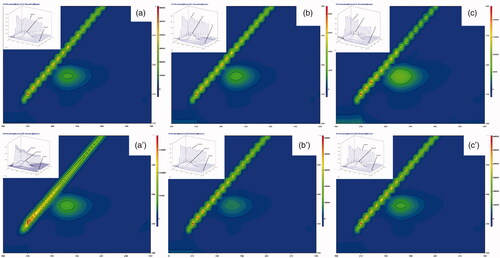
Figure 5. 3D fluorescence spectra and corresponding contour map for soluble and immobilized βGS in the presence of galactose. The spectra demonstrate (a), (b) and (c) as controls of soluble, PANI-CS-NC-βGS and PANI-CS/Ag-NC-βGS and (a′), (b′) and (c′) for native, PANI-CS-NC-βGS, and PANI-CS/Ag-NC-βGS.
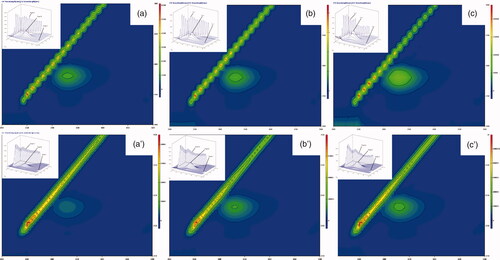
The consequences of added compounds namely VC, glucose and galactose on conformational properties of βGS were studied by recording 3 D fluorescence spectra for free and NC bound βGS in presence of specified compounds. The fluorescence intensities of both peaks A and B in free βGS declined upon addition of all three effectors. The order of inhibition was found to be VC < glucose < galactose (). These spectral changes revealed that interaction between added compounds and βGS resulted in micro-environmental and conformational changes in the soluble protein. On the contrary, PANI-CS-NC-βGS and PANI-CS-Ag-NC-βGS showed a less inhibitory effect in the presence of tested compounds. Alterations were mostly observed in peaks A and B. Changes in peak A are suggestive of an increase in the diameter of βGS which translates into an enhanced Rayleigh scattering effect, thus signifying complex formation, while alteration in peak B is attributed to alterations in the microenvironment of Tyr and Trp residues [Citation42]. PANI-CS-Ag-NC-βGS was found to be least affected in the presence of all examined compounds exhibiting minor conformational changes. The alternations in peak C related to the conformational changes in peptide backbone associated with helix–coil were mostly observed in galactose mediated inhibition.
Fourier-transform infrared spectroscopy
FT-IR spectral results provide valuable information about the content of protein secondary structure. The IR spectrum of protein exhibits two characteristic absorption bands, viz., amide I and amide II. These are prominent vibrational band of protein backbone. The amide I band appears in a region of 1700–1600 cm−1 due to C = O stretching vibrations of peptide linkages, while the amide II band is attributable to C–N stretch coupled with N–H bending mode appearing at 1600–1500 cm−1. Amide I band is known to be more sensitive to protein secondary structural changes than the amide II band [Citation43]. The conformational changes in soluble and immobilized βGS upon binding to VC and different sugars were evaluated by recording FT-IR spectra of free and NC bound βGS incubated in the presence of all studied compounds (. The FT-IR spectrum of free enzyme exhibited the only amide I band at 1629 cm−1. After additions of investigated compounds, a shift in the position of amide I bands was visualized. The amide I band was shifted from 1629 cm−1 to 1647 cm−1 and an additional amide II band appeared at 1557 cm−1. A sudden decrease in transmittance intensity with respect to untreated βGS was also witnessed as it is evident from the FT-IR spectra of the native enzyme. The band shift, additional band and decreased intensity were indicative of changes in protein secondary structure upon its interaction with VC and added sugars.
Figure 6. FT-IR spectra of soluble and NC immobilized βGS in the presence of VC and sugars. The spectra depict (a) free (b) PANI-CS-NC-βGS and (c) PANI-CS-Ag-NC-βGS.
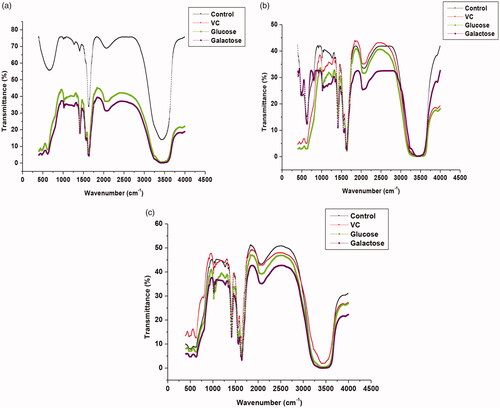
However, immobilized βGS preparations in the presence of VC, glucose, and galactose exhibited spectra identical to control. Both amide bands I and II were observed at same wave number which infer less conformational changes in immobilized βGS preparations in presence of all tested compounds. Galactose, a competitive inhibitor of βGS showed a maximum change in IR spectra of PANI-CS-NC-βGS. These results are in agreement to our previous findings which described that these compounds at higher concentrations were successful in suppressing the activity of soluble βGS, while the PANI-CS-NC-βGS showed remarkably higher resistance to inhibition mediated by such compounds. Pawar et al. [Citation39] investigated the binding of proton pump inhibitors to human serum albumin by means of spectroscopic and molecular modelling approaches. They observed shifts in protein amide bands due to conformational changes in the protein upon its interaction with omeprazole and esomeprazol.
Circular dichroism analysis
demonstrates CD spectroscopic measurements of soluble, PANI-CS-NC and PANI-CS-Ag-NC adsorbed βGS in the presence of investigated compounds. Among all the investigated compounds the highest changes in ellipticity were observed in the presence of galactose in all enzyme preparations with respect to control. Significant conformational changes were noticed in a soluble enzyme in the presence of galactose which led to a loss in its enzymatic activity. Table S4 summarizes α-helical and β-sheet content in all preparations in the presence of VC and sugars. α-Helical content was abruptly increased to 4 fold in a free enzyme in the presence of added galactose. On the other hand, insignificant changes were observed in the secondary structure of the immobilized βGS in presence of both sugars and VC with respect to control. These results corroborate well with those obtained through UV-visible, fluorescence-based spectroscopic experiments and FT-IR studies. Other researchers have previously employed CD technique to substantiate secondary structural changes taking place upon immobilization in proteins. He et al. [Citation44] evaluated conformational changes in the secondary structure of trypsin and chymotrypsin in the presence of Bowman-Birk inhibitor by CD measurements.
Figure 7. CD spectra of native and immobilized βGS in the presence of added compounds. The spectra illustrate (a) free (b) PANI/CS-NC-βGS and (c) PANI-CS-Ag-NC-βGS.
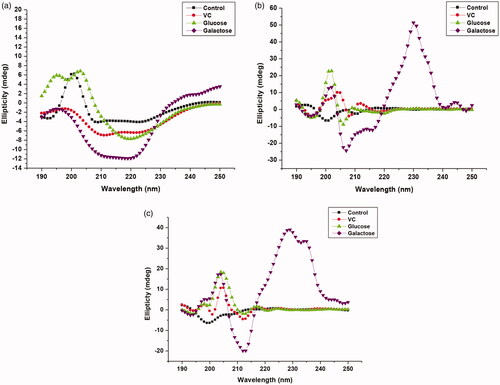
This study is an extension to our previously published work [Citation24,Citation29] where we have demonstrated the immobilization strategy and stability enhancement of the immobilized enzyme over the soluble counterpart. Furthermore, in one of the studies, we obtained a remarkable increase in the catalytic activity of NC bound enzymes in the presence of essential ions found in milk [Citation29].
Conclusion
To summarize, this study demonstrates the effect of products of lactose-hydrolyzed reaction, glucose and galactose and their structurally related compound, VC on the functional and structural properties of βGS in free and bound form in a comprehensive manner. Herein, a systematic effort has been done to measure changes in the structure of free and immobilized βGS in the presence of VC glucose and galactose. The soluble βGS showed a major loss in its catalytic activity in the presence of investigated compounds. PANI-CS-Ag-NC-βGS was found to be the most resistant against the effect of VC and products of βGS catalyzed reaction. Major secondary structural changes were noted in native enzyme incubated in the presence of galactose. This is an indication of unfavourable changes in the secondary structure of the protein which caused destabilization that leads to a loss in enzyme activity. Both immobilized NBC retained significantly higher catalytic activity and exhibited minor conformational changes in the presence of VC, glucose and galactose. Thereby, the immobilized βGS preparations can be effectively employed for the production of hindrance-free nano-biosensor for lactose detection in milk and its products and to produce lactose-free dairy products to be fed upon by lactose intolerant people.
Supplemental Material
Download ()Acknowledgements
QH designed the experiments and contributed in finalizing the manuscript; MK performed the experiments and analyzed the experimental data. Authors are extremely grateful to the instrumentation facility of Department of Chemistry, AMU Aligarh for conducting FT-IR and CD experiments.
Disclosure statement
The authors declare that they have no competing interests.
References
- Husain Q. Beta galactosidases and their potential applications: a review. Crit Rev Biotechnol. 2010;30:41–62.
- Gheczy N, Kuchler A, Walde P. Proteinase K activity determination with β-galactosidase as sensitive macromolecular substrate. Anal Biochem. 2016;513:54–60.
- Adam AC, Rubio-Texeira M, Polaina J. Lactose: the milk sugar from a biotechnological perspective. Crit Rev Food Sci Nutr. 2004;44:553–557.
- Cardoso B, Silverio SC, Luis A, et al. βGS from Aspergillus lacticoffeatus: a promising biocatalyst for the synthesis of novel prebiotics. Int J Food Microbio. 2017;257:67–74.
- Grosova Z, Rosenberg M, Rebros M. Perspectives and applications of immobilised β-galactosidase in food industry: a review. Czech J Food Sci. 2008;26:1–14.
- Khan M, Husain Q, Naqvi AH. Graphene based magnetic nanocomposites as versatile carriers for high yield immobilization and stabilization of β-galactosidase. RSC Adv. 2016;6:53493–53503.
- Klein MP, Hackenhaar CR, Lorenzoni ASG, et al. Chitosan crosslinked with genipin as support matrix for application in food process: support characterization and β-D-galactosidase immobilization. Carbohyd Polym. 2016;137:184–190.
- Husain Q, Ulber R. Immobilized peroxidase as a valuable tool in the remediation of aromatic pollutants and xenobiotic compounds: a review. Crit Rev Env Sci Tech. 2011;41:770–804.
- Khan M, Husain Q, Bushra R. Immobilization of β-galactosidase on surface modified cobalt/multiwalled carbon nanotube nanocomposite improves enzyme stability and resistance to inhibitor. Int J Biol Macromol. 2017;105:693–701.
- Husain Q. Remediation of phenolic compounds from polluted water by immobilized peroxidases. In: Bharagava R., Chowdhary P, editors. Emerging and eco-friendly approaches for waste management. Singapore: Springer; 2019.
- Husain Q. Magnetic nanoparticles as a tool for the immobilization/stabilization of hydrolases and their applications: an overview. Biointerface Res Appl Chem. 2016;6:1585–1606.
- Husain Q. Nanomaterials as novel support for the immobilization of amylolytic enzymes and their application: A review. Biocatalysis. 2017a;3:31–53.
- Husain Q. Nanomaterials immobilized cellulytic enzymes and their industrial applications: a literature review. JSM Biochem Mol Biol. 2017b;41029–1041.
- Husain Q. Carbon nanotubes mediated immobilized glucose oxidase biosensors as an effective and sensitive analytical tool. Biointerface Res Appl Chem. 2018a;8:3060–3074.
- Husain Q. Nanocarriers immobilized proteases and their industrial applications: an overview. J Nanosci Nanotechnol. 2018b;18:486–499.
- Ansari SA, Husain Q. Immobilization of Kluyveromyces lactis β galactosidase on concanavalin-A layered aluminium oxide nanoparticles-It’s future aspects in biosensor applications. J Mol Catal B: Enzym. 2011;70:119–126.
- Khan MJ, Khan FH, Husain Q. Application of immobilized β-amylase in the saccharification of starch. J Appl Biol Sci. 2011;5:33–39.
- El-Aassar MR. Functionalized electrospun nanofibers from poly (AN-co-MMA) for enzyme immobilization. J Mol Catal B: Enzym. 2013;85–86:140–148.
- Yu G, Wu W, Zhao Q, et al. Efficient immobilization of acetylcholinesterase onto amino functionalized carbon nanotubes for the fabrication of high sensitive organophosphorus pesticides biosensors. Biosens Bioelectron. 2015;68:288–294.
- Royvaran M, Taheri-Kafrani A, Isfahani AL, et al. Functionalized superparamagnetic graphene oxide nanosheet in enzyme engineering: a highly dispersive, stable and robust biocatalyst. Chem Eng J. 2016;288:414–422.
- Zhang B, Li P, Zhang H, et al. Preparation of lipase/Zn (PO4)2 hybrid nanoflower and its catalytic performance as an immobilized enzyme. Chem Eng J. 2016;291:287–297.
- Misson M, Jin B, Zhang H. Immobilized β-Galactosidase on functionalized nanoparticles and nanofibers: a comparative study. Int Proceed Chem Biol Environ Eng. 2015;90:7763.
- Khan M, Husain Q, Asmat S. Synthesis, characterization and applications of nano graphene armored enzymes. In: Kumar, CV, editors. Methods Enzymology. Vol. 609. Academic press, USA; 2018a, pp 83–142
- Khan M, Husain Q, Ahmad N. Elucidating the binding efficacy of β-galactosidase on polyaniline chitosan nanocomposite and polyaniline chitosan silver nanocomposite: activity and molecular model insights. J Chem Technol Biotechnol. 2019;94:837–849.
- Fontes EAF, Passos FML, Passos F. A mechanistical mathematical model to predict lactose hydrolysis by β-galactosidase in a permeabilized cell mass of Kluyveromyces lactis: validity and sensitivity analysis. Process Biochem. 2001;37:267–274.
- Haider T, Husain Q. Immobilization of β galactosidase from Aspergillus oryzae via immuonoaffinity support. Biochem Eng J. 2009;43:307–314.
- Park AR, Oh DK. Effects of galactose and glucose on the hydrolysis reaction of a thermostable beta-galactosidase from Caldicellulosiruptor saccharolyticus. Appl Microbiol Biotechnol. 2010;85:1427–1435.
- Gul-Guven R, Kaplan A, Guven K, et al. Effect of various inhibitors on β-galactosidase purified from the thermoacidophilic Alicyclobacillus acidocaldarius subsp. Rittmannii isolated from Antartica. Biotechnol Bioproc E. 2011;16:114–119.
- Khan M, Husain Q. Effect of metal ions present in milk on the functional and structural integrity of native and polyaniline chitosan nanocomposites bound β-galactosidase: a multi-spectroscopic approach. Food Chem. 2019;279:312–320.
- LOWRY OH, ROSEBROUGH NJ, FARR AL, et al. Protein measurement with the folin phenol reagent. J Biol Chem. 1951;193:265–275.
- Portaccio M, Stellato S, Rossi S, et al. Galactose competitive inhibition of β-galactosidase (Aspergillus oryzae) immobilized on chitosan and nylon supports. Enzyme Microb Technol. 1998;23:101–106.
- Secundo F. Conformational changes of enzymes upon immobilisation. Chem Soc Rev. 2013;42:6250–6261.
- Basu A, Kumar GS. Interaction and inhibitory influence of the azo dye carmoisine on lysozyme amyloid fibrillogenesis. Mol Biosyst. 2017;13:1552–1564.
- MacManus-Spencer LA, Tse ML, Hebert PC, et al. Binding of Perfluorocarboxylates to serum albumin: a comparison of analytical methods. Anal Chem. 2010;82:974–981.
- Bozoglan BK, Tunc S, Duman O. Investigation of neohesperidin dihydrochalcone binding to human serum albumin by spectroscopic methods. J Lumin. 2014;155:198–204.
- Sulkowska A. Interaction of drugs with bovine and human serum albumin. J Mol Struct 2002;614:227–232.
- Huang BX, Kim HY, Dass C. Probing three-dimensional structure of bovine serum albumin by chemical cross-linking and mass spectrometry. J Am Soc Mass Spectrom. 2004;15:1237–1247.
- Ghisaidoobe ABT, Chung SJ. Intrinsic tryptophan fluorescence in the detection and analysis of proteins: a focus on forster resonance energy transfer techniques. IJMS. 2014;15:22518–22538.
- Pawar SK, Punith R, Naik RS, et al. Spectroscopic and molecular modelling approaches to investigate the binding of proton pump inhibitors to human serum albumin. J Biomol Structure Dynamics. 2017;35:3205–3220.
- Cheng Z, Liu R, Jiang X. Spectroscopic studies on the interaction between tetrandrine and two serum albumins by chemometrics methods. Spectrochim Acta A. 2013;115:92–105.
- Paul BK, Guchhait N. A spectral deciphering of the binding interaction of an intramolecular charge transfer fluorescence probe with a cationic protein: thermodynamic analysis of the binding phenomenon combined with blind docking study. Photochem Photobiol Sci. 2011;10:980–991.
- Li D, Wang Y, Chen J, et al. Characterization of the interaction between farrerol and bovine serum albumin by fluorescence and circular dichroism. Spectrochim Acta A. 2011;79:680–686.
- Grewal MK, Chandrapala J, Donkor O, et al. Fourier transform infrared spectroscopy analysis of physicochemical changes in UHT milk during accelerated storage. Int Dairy J. 2017;66:99–107.
- He H, Li X, Kong X, et al. Effects of disulfide bond reduction on the conformation and trypsin/chymotrypsin inhibitor activity of soybean Bowman-Birk inhibitor. J Agric Food Chem. 2017;65:2461–2467.