Abstract
Mitochondrial dysfunction is a major contributory factor for myocardial ischemia-reperfusion (I/R) injury. It has been reported that Pink1-Parkin-mediated mitochondrial autophagy could effectively remove damaged mitochondria and excess ROS to ensure the stability of intracellular mitochondria. The present study was designed to evaluate whether the polymerized porcine haemoglobin (pPolyHb), a novel type of haemoglobin oxygen carrier, has an effect on I/R injury via regulating the Pink1-Parkin mediated mitochondrial autophagy pathway in myocardial H9C2 cells. The results revealed that pPolyHb could effectively reduce apoptosis and improve the survival rates of H9C2 cells. In addition, Pink1 and Parkin levels gradually decreased with pPolyHb reoxygenation. The inhibition of mitochondrial autophagy through mitochondrial-division inhibitor-1(mdivi-1) resulted in a decrease in anti-apoptotic protein Bcl-2 and an increase in pro-apoptotic protein Bax and CytC. In conclusion, pPolyHb has a protective effect on myocardial ischemia-reperfusion injury by regulating moderate mitochondrial autophagy.
Introduction
Acute ischemic cardiovascular disease is a serious threat to human health due to its increasing morbidity and mortality and is characterized by acute organ circulation disorder, damage of tissues, varying degrees of angina, myocardial infarction and so on [Citation1,Citation2]. The following ischemia-reperfusion (I/R) caused by the sudden recovery of oxygen in ischemic tissues will make things worse. The definite pathogenesis of ischemia-reperfusion (I/R) remains unclear, but considerable evidence have implicated that mitochondrial dysfunction is a major contributory factor for I/R injury via the abnormal production of reactive oxygen species (ROS) and calcium overload in cells. Pink1-Parkin-mediated mitochondrial autophagy has been reported in cardiovascular disease through the effective removal of damaged mitochondria and excess ROS, in order to ensure the stability of intracellular mitochondria [Citation3,Citation4]. At present, although traditional clinical methods have been adopted for the thrombolysis of the cardiovascular system, such as thrombolytic agents and stent interventional therapy [Citation5], merely a small part of patients could be diagnosed and receive treatment, such as thrombolytic therapy, within 3 h, or in time. Therefore, it is critical to develop therapeutics that aim to supplement oxygen to hypoxic tissues within a short period of time, and further avoid the (I/R) injury in hypoxia tissues induced by the sudden increase in blood and oxygen.
Some promising haemoglobin-based oxygen carriers (HBOCs) have been developed in an attempt to treat acute ischemic cardiovascular disease and decrease reperfusion injury. HBOCs are chemically modified haemoglobin solutions that have several advantages, when compared with packed red blood cells, including prolonged shelf-life, lack of a cross-matching requirement and minimal infectious risks or concerns on immunogenicity [Citation6]. HBOC-201 (Biopure; Cambridge, MA) is a glutaraldehyde-polymerized bovine haemoglobin (Hb) solution. Caswell et al. demonstrated that treatment with HBOC-201 before myocardial I/R improved organ oxygenation by increasing plasma and total haemoglobin concentration, and increasing oxygen unloading in the microcirculation, thereby also reducing the extent of myocardial inflammation and I/R injury in the canine myocardium [Citation7]. HBOC-201 has been evaluated in a phase II study in patients undergoing cardiopulmonary bypass surgery [Citation8]. Previous studies have revealed that liver reperfusion with a perfusate contains polymerized bovine haemoglobin molecules (HbG, Oxyglobin) that could effectively reduce reperfusion injury after cold liver preservation [Citation9]. The positive effect of HbG on reperfusion injury most likely depends on the improved organ oxygenation, preventing tissue necrosis and the induction of HO-1, with a subsequent increase in heme cleavage. A third product (Hemolink) was evaluated in a phase III study in patients undergoing coronary artery bypass graft surgery. However, the trial was suspended [Citation10]. In addition, several other haemoglobin-based oxygen carriers were in the clinical stages. Oxygen therapeutics have several potential clinical applications in the management of perioperative blood loss, trauma, acute normovolemic hemodilution, traumatic brain injury and blood requirements in patients who refuse or have contraindications to transfusions of red blood cells.
Polymerized porcine haemoglobin (pPolyHb) is novel type of haemoglobin-based oxygen carrier that uses glutaraldehyde for intramolecular and intermolecular crosslinking to form glutaraldehyde pPolyHb. It has been demonstrated that 1.5 g/kg of pPolyHb recovery can effectively restore blood pressure, maintain hemodynamic stability and effectively supply oxygen to tissues, as well as in the rat hemorrhagic shock model [Citation11]. In the isovolumic hemodilution model, pPolyHb could effectively increase animal haemoglobin, restore blood pressure, heart rate and blood oxygen content and correct hypoxia-induced metabolic acidosis [Citation12]. In addition, pPolyHb reoxygenation can significantly reduce the area of myocardial infarction in rats and improve oxygen supply in ischemic tissues in the rat model of myocardial I/R injury and in middle cerebral artery occlusion (MCAO) cerebral (I/R) injury [Citation13].
The present study was designed to evaluate whether pPolyHb, which acts as a novel type of haemoglobin oxygen carrier, has an effect on I/R injury via the regulation of the Pink1-Parkin-mediated mitophagy pathway in myocardial H9C2 cells.
Methods
Preparation of pPolyHb
The porcine Hb cross-linked by glutaraldehyde (Lifegen 100) was prepared as described previously, with certain modifications [Citation14,Citation15] and was a kind gift from Lifegen Co. Ltd. (Xi’an, Shaanxi, China) Briefly, Hb from fresh porcine blood was purified through specific steps and then cross-linked by glutaraldehyde. Small molecules, including excess glutaraldehyde and tetrameric haemoglobin, were removed by ultrafiltration. The polymerization technique allowed the retention of SOD and CAT activities. pPolyHb (10.5 ± 0.5 g/dL, methaemoglobin 5%, endotoxin 1.0 EU/mL, osmolality 300–330 mOsm, PH 7.4 ± 0.05, average molecular weight of pPolyHb 600 ± 50 kD, 64 kD tetramer 2%) was formulated in a buffer consisting of Na+ 135–155 mmol/L, K+ 3.0–5.0 mmol/L, Ca2+ 1–3 mmol/L, Cl− 140–160 mmol/L and stored at 4 °C until use. Additional details regarding pPolyHb are currently being withheld because of pending patents.
Establishment of the I/R injury model in H9C2 myocardial cells
H9C2 (Wuhan Punuosai Technology Company, China) was cultured in DMEM high-glucose medium and supplemented with fetal calf serum (10%), 2 mM of L-glutamine, 100 U/mL of penicillin and 100 g/mL of streptomycin at 37 °C in 5% CO2. The fusion degree of cells used for the experiment was more than 70%. These cells were randomly divided into two groups: control group and experimental group. Cells in the control group were routinely cultured while cells in the experimental group were further divided into different groups, according to the concentration of pPolyHb reperfusion (5, 10, 20, 40, 80, 100, 150, and 200 μM) within 24 h (cells were incubated in serum-free medium after removing the complete medium, followed by exposure in an hypoxia incubator for 3 h and normal reperfusion for 24 h via pPolyHb). Then, cells were harvested after a series of processes for further analysis.
MTT assay
Cell viability was measured using MTT assay. Briefly, cells were seeded into 96-well plates at a density of 1 × 104 cells/well. After incubation with different drugs for the indicated time periods, 10 μL of MTT solution (final concentration, 0.5 mg/mL) was added, and the incubation was continued for 4 h at 37 °C. Then, the culture medium was carefully removed, 100 μL of DMSO was added to dissolve the formazan crystals, and absorbance was measured using a microtiter plate reader (SpectraMax 190; Molecular Devices, USA) at a wavelength of 490 nm. Cell viability was expressed as an optical density (OD) value. In addition, cell morphology was observed using an inverted/phase contrast microscope, and the images were obtained (Olympus BX61, Japan).
Lactate dehydrogenase (LDH) assay
LDH, an indicator of cell injury, was detected after reoxygenation with pPolyHb using an assay kit (Institute of Jiancheng Biotechnology, China), according to manufacturer’s protocol. Enzyme activity was measured according to an absorbance of 440 nm.
Creatine kinase (CK) assay
Creatine kinase is usually used as a common marker for the clinical detection of myocardial infarction, rhabdomyolysis, muscle atrophy, and other diseases. It is mainly distributed in the cytoplasm and mitochondria of the heart, bones, brain and other tissues. Clinical studies have confirmed that the occurrence of myocardial infarction and other series of diseases, such as myocardial ischemia or myocarditis can significantly improve serum creatine kinase levels. Accordingly, in order to explore the effect of pPolyHb reoxygenation on I/R injury after cardiomyocyte hypoxia, the culture supernatant was collected to detect CK activity.
Cellular apoptosis assay
Cellular apoptosis was analyzed using an Annexin V-FITC apoptosis detection kit (Beyotime Institute of Biotechnology, China) according to manufacturer’s instructions. H9C2 cells were incubated in different concentrations of pPolyHb for 24 h followed by the washing and collecting of cells in PBS and centrifugation (800 g, 10 min). Annexin V-FITC binding buffer (195 μL), Annexin V-FITC(5 μL) and propidium iodide staining solution(10 μL) were used (10–20 min at room temperature) for phosphatidylserine (PS) staining on the cell membrane, and these cells were detected by flow cytometry(Nikon Corporation, Tokyo, Japan). The apoptotic rate was expressed as the number of positively stained apoptotic H9C2 divided by the total number of H9C2 counted and multiplied by 100%.
Western blot assay
H2O2, as a common active oxygen molecules, can freely pass through the mitochondria. A physiological dose of H2O2 can be used as signal transduction molecules to regulate various signalling pathways. Therefore, these cells were treated with 400 M of H2O2 for 2 h to induce mitochondrial autophagy, and these were assigned as the positive control group. Other groups were established, including the control group, I/R group, I/R + 10 μM pPolyHb and I/R + 20 μM pPolyHb. After processing, these cells were washed and collected in ice-cold PBS and centrifuged (800 g, 10 min). Then, the cell pellet was resuspended in ice-cold lysis buffer and incubated on ice for 30 min. After centrifugation at 12,000 rpm, the supernatant was transferred, and the remaining proteins were stored at 80 °C.
The protein concentrations were determined using a Bradford protein assay kit (Beyotime Institute of Biotechnology, China). The proteins were separated using SDS-PAGE electrophoresis and transferred onto PVDF membranes. The membranes were blocked for 2 h in Tris-buffered saline and Tween 20 (TBST, pH 7.6), which contain 5% non-fat dry milk powder. Then, the membranes were incubated overnight at 4 °C, with antibodies against Pink1, Parkin and the apoptotic protein of Bcl-2, Bax, cytochrome c and β-actin, followed by washing in TBST. Afterwards, the membranes were probed with different secondary antibodies (dilution at 1:5000) at room temperature for 90 min, followed by washing in TBST. The protein bands were detected using chemiluminescence and quantified using the Quantity One software package (Bio-Rad Laboratories, UK). The results for the control group were defined as 100%.
Results
Effect of pPolyHb on H9C2 cells viability
The effect of pPolyHb on H9C2 cells viability is presented in . Cells that were not pretreated with pPolyHb were assigned as the control group, and the survival rate was set to 100%. Compared with the control group, different concentrations of pPolyHb pretreatment continued to maintain the cellular activity. The survival rates for groups with 5, 10, 20 and 40 μM of pPolyHb were 108.77%, 120.72%, 113.70% and 109.99%, respectively, and these were significantly higher than those in the normal group (p < .05). This indicates that pPolyHb is not poisonous to cells and can effectively maintain the cell growth status.
Figure 1. pPolyHb can effectively maintain the cell growth state. Cell viability was tested by MTT assay. After treatment with different concentrations of pPolyHb, cells were cultured for 24 h. When compared with the control group, the 5 μM pPolyHb, 10 μM pPolyHb, 20 μM pPolyHb and 40 μM pPolyHb groups was *p < .05. No significant differences were found in the other groups.
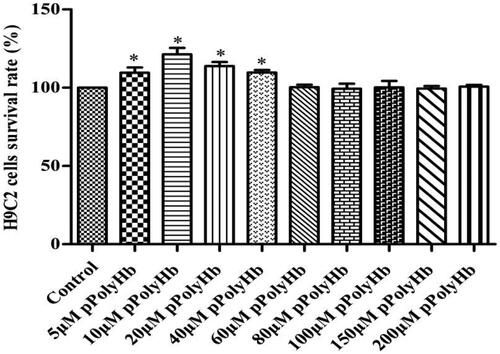
Effect of pPolyHb on hypoxia – reoxygenation cells viability
The effect of pPolyHb on hypoxia-reoxygenation cell activity is presented in . In the control group, the survival rate of cells was set to 100%. The I/R group was a complete hypoxia-reoxygenation group via DMEM medium reoxygenation, resulting in cell viability (63%) while cell viability in the different concentrations of pPolyHb reoxygenation (5, 10, 20, 40, 60, 80, 100, 150 and 200 μM) were increased to 82.93%, 89.41%, 87.63%, 76.51%, 73.07%, 72.06%, 72.52%, 65.47% and 67.53%, respectively. In particular, 10 μM and 20 μM of pPolyHb could significantly improve cell viability, which suggests that 10 μM and 20 μM of pPolyHb can reduce I/R injury to some content and protect cells. Therefore, these two concentrations of pPolyHb were selected for the subsequent experiments.
Figure 2. After hypoxia/reoxygenation, the appropriate concentration of pPolyHb was able to effectively increase the viability of H9C2 cardiomyocytes. Cell viability was determined by MTT assay after hypoxia/reoxygenation. *p < .05, compared with the control group; #p < .05 compared with the I/R group.
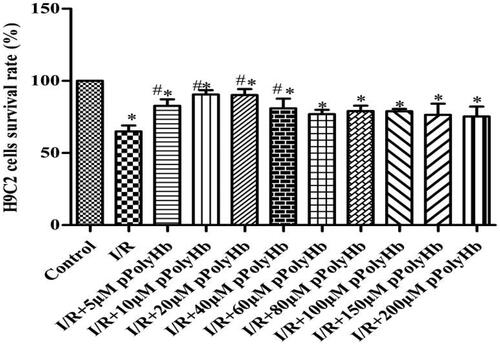
LDH and CK test results
The concentrations of LDH and CK in the different groups are presented in . In apoptotic or necrotic cells, intracellular LDH was released into the cell culture medium via the damaged cell membrane structure, and the concentration could be indirectly analyzed. CK is usually used as a common marker for the clinical detection of myocardial infarction. In order to rule out the similar effects of other macromolecules, the same concentration of BSA vs pPolyHb was deoxygenated to detect LDH and CK activities. The results revealed that the LDH activity was 342.56 U/L in the I/R group, which was significantly different from that in the control group (175.43 U/L), indicating that these cells were damaged after hypoxia-reoxygenation. There was no difference in LDH activity observed between the BSA and I/R groups. However, pPolyHb could effectively reduce the release of LDH. The CK activity of each group was similar to LDH, which suggests that pPolyHb has a protective effect on myocardial I/R injury.
Figure 3. A certain concentration of pPolyHb can reduce the activity levels of creatine kinase (CK) and lactate dehydrogenase (LDH), after hypoxia/reoxygenation. The activity of LDH and CK in the supernatant of H9C2 cells was determined by ELISA. **p < .01, compared with the control group; ##p < .01, compared with the I/R group.
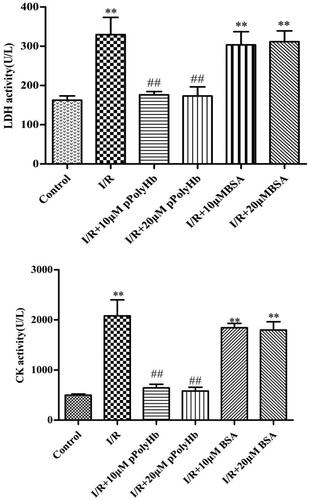
Effects of pPolyHb on the apoptosis of H9C2 cells
The effects of pPolyHb on the apoptosis of H9C2 cells during I/R injury are presented in . These results reveal that there was a significant difference in apoptosis rate between the control group (10%) and I/R group (36%). In addition, cell apoptosis improved after the reoxygenation with pPolyHb and the apoptotic rates decreased to 18% and 16%, respectively. These revealed that pPolyHb could alleviate apoptosis under the status of I/R injury.
Effects of reoxygenation with pPolyHb on the expression of Pink1 and Parkin
In order to investigate the effect of pPolyHb on mitochondrial autophagy during I/R injury in cardiomyocytes, the expression of signal-associated proteins Pink1 and Parkin were detected, which play an important role in mitochondrial autophagy. As shown in in the H2O2 group, the content of Pink1 significantly increased, when compared with the control group, but there was no significant difference between the H2O2 and I/R groups at the reoxygenation time of 12 and 24 h. After treatment with different concentrations of pPolyHb, Pink1 became significantly higher, when compared to the control group, but there was no significant difference when compared with the H2O2 group. Similarly, pPolyHb could also induce Parkin expression, when compared with the control group, indicating the promoting effect of the mitochondrial autophagy of pPolyHb in the I/R injury of cardiomyocytes.
Figure 5. The upregulation of pPolyHb in mitochondrial autophagy induced by hypoxia is closely correlated to the Pink1-Parkin signalling pathway. The western blot analysis of the expression of Pink1 and Parkin is shown. A: Control; B: H2O2; C: I/R; D: I/R + 10 μM pPolyHb; E: I/R + 20 μM pPolyHb; (a) The expression of Pink1 among groups at the reoxygenation times of 12 and 24 h. (b) The expression of Parkin among groups at the reoxygenation times of 12 and 24 h. *p < .05, compared with control group; #p < .05, compared with the I/R group.
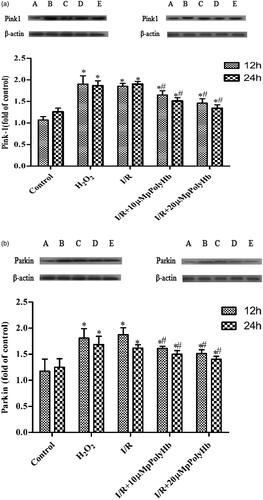
The expression of bax, bcl-2 and CytC after mitochondrial autophagy inhibition
The results for the apoptotic protein are presented in . In order to investigate the association between the possible role of mitochondrial autophagy and cell apoptosis, cells were treated with 5 μM of mitochondrial division inhibitor-1 (Mdivi-1) for 1 h, and the hypoxia-reoxygenation model was subsequently established. The expression of Bcl-2/β-actin, Bax/β-actin, CytC/β-actin, and Bcl-2/Bax were detected by immunohistochemistry, which are key indicators in the process of apoptosis. The results revealed that the expression of Bcl-2 in each group was lower than that in the control group, indicating that the mitochondrial division inhibitor could inhibit the expression of anti-apoptotic protein Bcl-2. The ratios of Bax/β-actin in the H2O2, I/R group, 10 μM pPolyHb group and 20 μM pPolyHb group were higher than those in the control group. The trend of the CytC content was consistent with that of Bax, and the ratio of Bcl-2/Bax in each group was also significantly lower than that in the control group.
Figure 6. Mitochondrial autophagy induced by pPolyHb can inhibit the apoptosis of cells to some extent, and play a protective role on myocardial cells. A: Control; B: H2O2; C: I/R; D: I/R + 10 μM pPolyHb; E: I/R + 20 μM pPolyHb; Cells were treated with 5 μM of mitochondrial division inhibitor-1 (Mdivi-1) for 1 h, and the expression of apoptotic proteins Bax, Bcl-2 and CytC was detected by western blot under the condition of mitochondrial autophagy inhibition. *p < .05, **p < .01, compared with the control group; #p < .05, ##p < .01, compared with the I/R group.
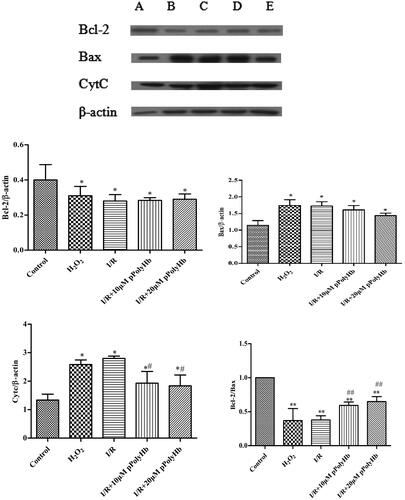
Discussion
Acute ischemic cardiovascular disease is a serious threat to human health, and the aetiology is the thrombosis or narrow gap formation in the inner vessel [Citation16]. Therefore, the immediate supply of blood and oxygen is the key to the treatment of ischemic cardiovascular diseases. I/R injury is caused by the sudden recovery of oxygen in ischemic tissues. Mitochondrial dysfunction has been considered to be a major contributing factor for I/R injury. The present study was designed to evaluate whether the pPolyHb as a novel type of haemoglobin oxygen carrier has effects on I/R injury via the regulation of the Pink1-Parkin-mediated mitophagy pathway in myocardial H9C2 cells. pPolyHb has excellent oxygen-carrying/oxygen-releasing capacities and nanometer molecular diameter characteristics, and its diameter is merely approximately 20 nm, which is much smaller than that of red blood cells (7–8 μm), allowing it to easily pass through the narrow gap within the vascular cavity to supply oxygen to the ischemic cells or tissues, thereby alleviating I/R injury. The results revealed that pPolyHb could effectively reduce the apoptosis and improve the survival rates of H9C2 cells, and provide a protective effect on myocardial I/R injury by regulating moderate mitochondrial autophagy.
According to the MTT experimental results, different concentrations of pPolyHb had no toxic effect on H9C2 cells. Meanwhile, 10 μM and 20 μM of pPolyHb could effectively restore the viability of cells after ischemia. These findings are consistent with the superior oxygen-carrying and releasing capabilities of pPolyHb, which can help improve cell viability.
The detection of cell culture medium LDH and CK concentration is an indirect analysis to evaluate cell damage. In apoptotic or necrotic cells, intracellular LDH is released into the cell culture medium via the damaged cell membrane structure and its concentration can be indirectly analyzed. Furthermore, pPolyHb can effectively reduce the release of LDH and CK, causing the values to return to near baseline. Meanwhile, the apoptosis of cells after pPolyHb reoxygenation was detected. These results reveal that pPolyHb alleviated the apoptosis of cells, confirming the protective effect of pPolyHb for I/R injury.
Myocardial contraction and the operation of the heart are mainly dependent on mitochondria to provide energy, which may induce myocardial I/R injury. It has been reported that a large number of damaged or dysfunctional mitochondria accumulate within cells, resulting in increased intracellular ROS, and leading to cardiac dysfunction. Therefore, it is particularly critical to maintain the normal function of mitochondria. Mitochondrial autophagy can protect cells by removing damaged, senescent and dysfunctional mitochondria [Citation17]. During the process of mitochondrial autophagy, multiple pathways are activated to function, and the typical Pink1-Parkin-mediated pathway has been widely evaluated for improving myocardial homeostasis [Citation18,Citation19].
In order to determine whether pPolyHb reoxygenation can play a cytoprotective effect by activating the mitochondrial autophagy Pink1-Parkin pathway, the expression of Pink1 and Parkin protein was detected. The results revealed that Pink1 and Parkin protein content gradually decreased and that this content was lower than that in the H2O2 treatment group and I/R group, indicating that pPolyHb can activate moderate mitochondrial autophagy. The association between the possible role of mitochondrial autophagy and cell apoptosis was analyzed by detecting the expression of Bax, CytC and Bcl-2 after mitochondrial autophagy inhibition. These results revealed that when mitochondrial autophagy was inhibited, anti-apoptotic protein Bcl-2 content decreased and pro-apoptotic protein Bax and CytC content increased, indicating that moderate mitochondrial autophagy can reduce cell apoptosis and play a cytoprotective effect.
In summary, pPolyHb can reduce myocardial I/R injury and play a protective effect on cells. In the present study, the molecular mechanism of the protective effect of pPolyHb was explored from the cellular level. However, further studies related to its specific signal pathway needs to be conducted to verify the protective mechanism of pPolyHb on myocardial I/R injury in vivo, which may provide theoretical support and useful information for the improvement of the pPolyHb oxygen carrier.
Acknowledgements
We would like to thank LetPub (www.letpub.com) for providing linguistic assistance during the preparation of this manuscript.
Disclosure statement
No potential conflict of interest was reported by the authors.
Additional information
Funding
References
- Budaj A, Brieger D, Steg PG, et al. Global patterns of use of antithrombotic and antiplatelet therapies in patients with acute coronary syndromes: insights from the Global Registry of Acute Coronary Events (GRACE). Am Heart J. 2003;146:999–1006.
- Doll JA, Tang F, Cresci S, et al. Change in angina symptom status after acute myocardial infarction and its association with readmission risk: an analysis of the Translational Research Investigating Underlying Disparities in Acute Myocardial Infarction Patients' Health Status (TRIUMPH) Registry. J Am Heart Assoc. 2016;5:e003205.
- Li Y, Qiu L, Liu X, et al. PINK1 alleviates myocardial hypoxia-reoxygenation injury by ameliorating mitochondrial dysfunction. Biochem Biophys Res Commun. 2017;484:118–124.
- Dai H, Deng Y, Zhang J, et al. PINK1/Parkin-mediated mitophagy alleviates chlorpyrifos-induced apoptosis in SH-SY5Y cells. Toxicology. 2015;334:72–80.
- Baskin JL, Reiss U, Wilimas JA, et al. Thrombolytic therapy for central venous catheter occlusion. Haematologica. 2012;97:641–650.
- Eastman AL, Minei JP. Comparison of Hemoglobin-based oxygen carriers to stored human red blood cells. Crit Care Clin. 2009;25:303–310. Table of Contents.
- Caswell JE, Strange MB, Rimmer DM, 3rd, et al. A novel hemoglobin-based blood substitute protects against myocardial reperfusion injury. Am J Physiol Heart Circ Physiol. 2005;288:H1796–H1801.
- Serruys PW, Vranckx P, Slagboom T, et al. Haemodynamic effects, safety, and tolerability of haemoglobin-based oxygen carrier-201 in patients undergoing PCI for CAD. EuroIntervention. 2008;3:600–609.
- Topp SA, Krieg A, Koch A, et al. Hemoglobin-glutamer 200 reduces reperfusion injury of the cold preserved rat liver by induction of heme oxygenase-1. J Surg Res. 2008;150:243–254.
- Stollings JL, Oyen LJ. Oxygen therapeutics: oxygen delivery without blood. Pharmacotherapy. 2006;26:1453–1464.
- Wang L, Liu F, Yan K, et al. Effects of resuscitation with polymerized porcine hemoglobin (pPolyHb) on hemodynamic stability and oxygen delivery in a rat model of hemorrhagic shock. Artif Cells Nanomed Biotechnol. 2017;45:51–57.
- Zhu H, Dang X, Yan K, et al. Pharmacodynamic study of polymerized porcine hemoglobin (pPolyHb) in a rat model of exchange transfusion. Artif Cells Blood Substit Immobil Biotechnol. 2011;39:119–126.
- Xie Z, Liu L, Zhu W, et al. The protective effect of polymerized porcine hemoglobin (pPolyHb) on transient focal cerebral ischemia/reperfusion injury. Artif Cells Nanomed Biotechnol. 2015;43:180–185.
- Gu J, Chang TM. Extraction of erythrocyte enzymes for the preparation of polyhemoglobin-catalase-superoxide dismutase. Artif Cells Blood Substit Immobil Biotechnol. 2009;37:69–77.
- Zhu H, Du Q, Chen C, et al. The immunological properties of stroma-free polyhemolysate containing catalase and superoxide dismutase activities prepared by polymerized bovine stroma-free hemolysate. Artif Cells Blood Substit Immobil Biotechnol. 2010;38:57–63.
- Dzupa V, Waldauf P, Motovska Z, et al. Risk comparison of bleeding and ischemic perioperative complications after acute and elective orthopedic surgery in patients with cardiovascular disease. Arch Orthop Trauma Surg. 2016;136:907–911.
- Andres AM, Hernandez G, Lee P, et al. Mitophagy is required for acute cardioprotection by simvastatin. Antioxid Redox Signal. 2014;21:1960–1973.
- Zha Z, Wang J, Wang X, et al. Involvement of PINK1/Parkin-mediated mitophagy in AGE-induced cardiomyocyte aging. Int J Cardiol. 2017;227:201–208.
- Barodia SK, Creed RB, Goldberg MS. Parkin and PINK1 functions in oxidative stress and neurodegeneration. Brain Res Bull. 2017;133:51–59.