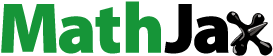
Abstract
Hypericum perforatum oil loaded electrospun polymeric wound dressing material was produced in order to be used in wound therapy. H. perforatum oil is known to have curative effect on wound-healing process. Wound dressing material was produced in two layers, upper layer of which was made of electrospun PCL nanofibres in order to maintain membrane integrity and mechanical strength, and bottom layer that is designed to be in contact with the wound was formed by electrospraying and electrospining of PEG/H. perforatum oil and PCL polymer solutions from opposite directions (concurrently). Methods such as FTIR, optical and electron microscopy, tensile tests, gas permeability tests, contact angle and swelling tests, in vitro release tests were utilized for material characterization. Encapsulation of H. perforatum oil in PEG capsules which were hold by PEG fibres among PCL fibres was confirmed. H. perforatum oil was released in controlled manner. Antimicrobial activity tests on Staphylococcus aureus and Escherichia coli revealed that H. perforatum content exhibited antimicrobial activity on both. Material was found to be biocompatible and suitable for use as wound dressing according to the results of in vitro tests, in which L929 mouse fibroblast cell line incubated with materials for investigation of biocompatibility (WST-1) and cell–material interactions (proliferation, apoptosis/necrosis).
Introduction
Wound can be defined as the injury or opening on the skin surface by chemical, mechanical and/or thermal damage. There are two major wound types of wounds, acute (burn, trauma, etc.) and chronic (diabetes, tumour, physiological contaminations, etc.). Since wounds of acute injuries heal generally in 8–12 weeks, healing process of chronic wounds takes longer [Citation1]. Wound healing is a biological process that is directly related with physiological parameters (haemostasis, inflammation, migration and maturation) [Citation1,Citation2]. In such circumstances, it is mandatory to keep the wound moist and protected from external factors (microorganisms, foreign bodies, etc.) by covering the wound surface with a convenient wound dressing [Citation3].
There are different commercial and registered polymeric wound dressing materials. Talymed® is such product that consists of poly-N asetyl glucosamine fibres (pGlcNAc) isolated from microalgae and stimulates the cell migration by interacting with fibroblasts and endothelial cells [Citation4]. Hyalosafe® is a transparent wound dressing material that is designed for maintaining a moist wound environment for superficial wounds [Citation5]. A microenvironment rich in hyaluronic acid (HA) is provided while the degradation of the wound dressing material which is formed from benzyl esters of HA [Citation5]. Thus, inherent wound healing is supported [Citation5]. Non-adherent porous nylon wound dressing material Actisorb™ Plus consists of active carbon and silver [Citation6]. When a microorganism infiltrates within the material, it is adsorbed on the active carbon and exterminated by the antimicrobial activity of silver [Citation6].
Healing may exhibit versatility depending on both wound and patient’s conditions. Therefore, one type of wound dressing material cannot maintain suitable conditions for wound healing. It is aimed to converge the ideal wound dressing material by new design and production techniques. There are various modern wound dressing films, in which different natural, synthetic polymers and composites of these polymers were used. Incorporation of bioactive molecules (growth factors, vitamins, minerals, antimicrobial agents, etc.) with the wound dressing material, to provide controlled release in the wound environment, also maintains a faster and better progress in healing process [Citation2]. Herbal agents (volatile oils, flavonoids, alkaloids, terpenoids, saponins, tannins, phenolic compounds, etc.) exhibiting antimicrobial activity, support coagulation, inflammation, collagen synthesis, epithelisation stages during the healing process [Citation7–9] Hypericum perforatum plant containing active agents such as hypericin and hyperforin, has the therapeutic effect on wound healing [Citation10]. H. perforatum oil exhibits antimicrobial activity on various Gram-positive and Gram-negative bacteria species [Citation11]. It is determined that ointments made with various herbal oils including H. perforatum oil, positively effects wound healing [Citation7]. Furthermore, it is noted that H. perforatum extracts supported wound healing by increasing the activation of fibroblasts and stimulation of collagen synthesis [Citation12,Citation13]. Direct application of H. perforatum oil on the wound, as commonly used in alternative medicine for burn-wound healing, decreases the sense of inflammation and speeds up the wound-healing process. However, oil layer above the wound prevents the gas transfer by ceasing the air contact of the wound and obstructs the patient compatibility.
In this study, integrated polymeric wound dressing materials were produced by using modern wound dressing material together with alternative medicine (herbal oil therapies) with the aim of speeding up the healing process and maintaining all the requirements (protecting the wound from external factors such as exogenous bacteria etc., avoiding infection and antimicrobial activity, maintaining moist control, providing gas permeability, no adhesion to the wound, etc. [Citation2]) for wound healing. For this purpose, H. perforatum oil was integrated with the modern wound dressing material. The thin upper layer of the double layer polymeric membranes was made of electrospun PCL nanofibres in order to maintain membrane integrity and mechanical strength. Bottom layer, in contact with the wound, was produced by concurrently electrospraying and electrospining of PEG/H. perforatum oil and PCL polymer solutions from opposite directions. Electrospun nanofibre dressing materials provide big advantages by mimicking the extracellular matrix (ECM) together with providing high porosity and low pore size, high surface/volume ratio, lightweight and strong mechanical properties [Citation14,Citation15]. Water-soluble polyethylene glycol (PEG) and mechanically strong poly(ɛ-caprolactone) (PCL) are biocompatible polymers and used as wound dressing materials [Citation16–20]. Bottom layer consist H. perforatum oil encapsulated in PEG between electrospun PCL fibres. Encapsulation of the H. perforatum oil was achieved by addition into PEG solution followed by electrospraying. Controlled release of H. perforatum oil was assured by dissolution of PEG microcapsules while in contact with the wound exuda.
Materials and methods
Materials
Polyethyleneglycol (PEG) with 10000 Da molecular weight purchased from Merck, Germany and polycaprolactone (PCL) with 80000 Da molecular weight purchased from Sigma-Aldrich, UK. 100% pure H. perforatum oil (Awe Cemre Laboratories, Turkey) was added to membrane structure as active agent. Chloroform (Merck, Germany) and methanol (Merck, Germany) mixture (5:1) were used as a solvent.
Esherichia coli (ATCC 25922) and Staphylococcus aureus (ATCC 29213) bacterial strains (were supplied by Gaziosmanpaşa University, Faculty of Medicine, Biochemistry Laboratories) and Mueller Hinton Bouillon (MHB), Luria-Bertani broth (LB), Mueller Hinton Agar (MHA), Eosine Methylene Blue (EMB) Agar mediums (Merck, Germany) were used for antimicrobial activity tests.
L929 fibroblast cell line obtained from Sigma-Aldrich and Dulbecco's modified Eagle's medium (DMEM)-low glucose (%10 foetal bovine serum (FBS), %1 l-glutamine, %1 antibiotic (penicillin/streptomycin), trypsin-EDTA, Hoechst 33342, propidium iodide (PI) and ribonuclease A (RNase A) purchased from Biological Industries Ltd. Kibbutz Beit Haemek, Israel. WST-1 (2–(4-iodophenyl)-5–(2,4-disulphophenyl)-2 h-tetrazolium purchased from Roche, Germany for biocompatibility studies.
Methods
Wound dressing material with double layer design was produced by electrospinning and electrospraying technique with the aim of lessening scar formation while supporting wound healing. The thin upper layer was produced from electrospun PCL nanofibres in order to maintain membrane integrity and mechanical strength. Lower layer that would be in contact with the wound was produced simultaneously by electrospinning of PCL solution and electrospraying of H. perforatum oil containing PEG solution from opposite directions. Lower layer was formed of H. perforatum oil encapsulated with PEG that is incorporated with PCL nanofibres. H. perforatum oil was released in a controlled manner by interaction of PEG capsules with the wound exudate.
Membrane production by co-electospinning and H. perforatum oil loading by electrospraying
Bottom layer of the polymeric membranes that would be in contact with the wound were produced by co-spinning of PEG and PCL. The H. perforatum oil loaded capsules in this layer were formed by electrospinning of centaury oil-PEG solution. For this purpose, 0.04 g/ml of PEG/chloroform solution was prepared. H. perforatum oil was added to the solution dropwise. Since chloroform is solution that does not disrupt ester bonds, it dissolved certain amount of centaury oil, and this resulted a homogeneous electrospinning solution in which no phase separation was observed. The homogeneous solution was placed in a syringe with a 20 G needle and it was spun onto a rotating collector by using a syringe pump with a rate of 3.0 ml/h. The distance between the needle tip and the collector was 7 cm, and the applied voltage was 14 kV. Electrospinning of PCL and electrospraying were implemented simultaneously from opposite directions. PCL was dissolved in 20% (v/v) methanol/chloroform mixture. PCL solution was placed in a syringe with 20 G needle and electrospun with a rate of 1.5 ml/h at the beginning. The distance between the needle tip and the collector was 13 cm, and the applied voltage was 14 kV. Certain amount of PCL solution was electrospun with a rate of 2.0 ml/h about the end of the bottom layer by co-spinning. This is due to the creation of a transition zone in order to maintain the integrity between bottom and top layers. The top layer of the membrane was formed by only PCL at a rate of 3.0 ml/h without changing the other electrospinning conditions. Thus, no-oil containing PEG/PCL membrane (membrane 0) and PEG/PCL membranes with different oil contents (7% (v/v) - 17% (v/v) - 34% (v/v), respectively membrane 1–2–3). Membranes were placed in a desiccator under vacuum after production. Dried membranes were then packed and kept at +4 °C until use.
Chemical structure and morphology of the membranes
FTIR spectra of the electrospun membranes were obtained in order to check if any change has occurred in the chemical structure during the interaction of PEG-PCL polymer with the solvent under high voltage electro-spinning conditions. Attenuated total reflectance Fourier Transform Infrared Spectroscopy (ATR-FTIR) analysis was performed using an ATR-equipped spectrophotometer (Perkin Elmer, Spectrum 100, USA) in the range of 4000–500 cm−1 [Citation21]. The surface morphologies of the top and bottom layers of the membranes were determined by scanning electron microscope (SEM) (JEOL JSM-5600, UK). Sections that were previously dried under vacuum, having a diameter of 7.5 mm were fixed on SEM sample platform. Surfaces were coated by a conductive layer for 15 min under vacuum (Polaron SC500 Sputter Coater, UK), and then, SEM images were obtained.
Mechanical properties
A wound dressing shall exhibit properties such as flexibility, mechanical strength and elasticity in order to provide ease of application and use. In order to determine the mechanical properties of the membranes, test specimens were cut out from the membranes in 5 mm width and 10 cm length in accordance with standard (ASTM ASTM D 882–02, 2002). Tensile test measurements were carried out with a constant head speed of 500 mm/min and 500 N load at room temperature using a tensile test instrument (Devotrans, BP D NU, Turkey) in accordance with related standard (EN ISO 134934–1: 2013). Average of three measurements were taken for each of four different membranes (Membrane 0, 1, 2, 3).
Density and porosity
Membranes were cut into 1.0 × 1.0 cm dimensions and weighed. Thickness of the membranes were measured and volume, membrane density (g/cm3) and specific volume (Vm) were calculated [Citation22]. Then, porosity (%) of the membranes were calculated using the equation below.
(1)
(1)
where Vo and Vm are the specific volume of the pure film (prepared by polymer blend) and electrospun membrane, respectively. Measurements were done for 10 samples of membranes. Calculated values are summarized on .
Table 1. Density and porosity in membranes.
Gas permeability
Air permeability was measured with the air permeation device (SDL Atlas/M021A, ABD) in accordance with the related ISO standard (TS 391 EN ISO 9237.1999) using the following test parameters; sample area of 20 cm2, applied pressure of 100 Pa and conditioning parameters of 20 2
65
%RH. Average of 6–8 measurements of permeation rate (l/m2/s) from one side to the other side of membranes were calculated for each membrane.
Hydrophilicity and wettability
Hydrophilicity of the membranes were determined with contact angle measurement device (Attention, Biolin Scientific, Sweden) according to the related standard (ASTM D 7334, 2013). Measurements were carried out under air atmosphere. 4 μl of deionized water was dropped on the membrane and the image of the drop was shot with a camera.
The angle between the water drop and the membrane was measured from the image with the help of the computer software. Average of three measurements from each surface were calculated.
Swelling studies performed in phosphate buffered saline (PBS at ∼ pH 7,4) for determination of wettability of membranes. Circular membranes with 7,5 mm radius was put into PBS and incubated at 37 °C. Membranes were taken out from the solution at different time periods (3, 6, 9, 12, 15, 20, 25, 30, 45, 60 min) and weighed. Swelling ratio (SR) was calculated using the equation below.
(2)
(2)
where W0 and Wt are initial and final weight of membranes, respectively [Citation16].
Mass loss in time (weight remaining %)
Membranes were cut in 2 cm × 2 cm dimensions and pre-weighed samples were immersed in phosphate buffer solution (PBS at ∼ pH 7,4 and 37 °C) for predetermined time intervals (2,4,6,8,10,12,14 days). After these time intervals, membranes were taken out from PBS and dried for two days in an incubator. Then, 10 dried samples of each membrane (with and without oil) were weighed. Weight remaining (%) of the membranes was calculated using the following equation.
(3)
(3)
where Wafter and Wbefore dry weights of the membranes after and before mass loss, respectively.
Controlled release of H. perforatum oil
In vitro H. perforatum oil release study was performed in phosphate-buffered saline (PBS at ∼ pH 7,4). H. perforatum oil containing circular membranes (membrane 1) with 7,5 mm radius was put into PBS and incubated at 37 °C. Membranes was removed from the solution at predetermined time intervals and released amount of oil was determined with a spectrophotometer (BOECO S-30, Germany) by optical density measurements of solutions (by taking absorbance at 225 nm [Citation23].
Antimicrobial activity
The antimicrobial activity of the H. perforatum oil-loaded electrospun membranes was investigated by using disc diffusion method on S. aureus (ATCC 29213) and E. coli (ATCC 25922) which are Gram-positive and Gram-negative bacteria, respectively. Circular sections of 0.75 cm in diameter were taken out from four different membranes (0, 1, 2, 3) with different amounts of H. perforatum oil content and these samples were sterilized under UV radiation for 15 min before their use in solid culture mediums of microorganisms. For this purpose, S. aureus and E. coli strains in solid culture medium were inoculated to MHB and LB liquid mediums, respectively. It was observed that liquid culture showed absorbance values between 0.08 and 0.13 at 625 nm after 2 h of incubation. This interval was accepted to match with 0.5 McFarland standard that is used for cultures to be used in antimicrobial activity test with disc diffusion method. Samples from the liquid cultures of S. aureus and E. coli having a turbidity value matching with this standard were taken with the help of swab and then cultivated on MHA and EMB agar mediums. Microorganisms treated with membranes were incubated at 37 °C for 16–20 h. After 16 h of incubation, antimicrobial activity of the membranes on S. aureus and E. coli strains were observed/evaluated.
Interaction of membranes with cells
Cell adhesion and proliferation of L929 fibroblast cells on the sides containing H. perforatum oil loaded PEG capsules in four different membranes (1, 2, 3) with different amounts of H. perforatum oil content were investigated. For this purpose, L929 cells were incubated one day before cell culture studies. Cells were cultured in the medium containing 1% penicillin (antibiotic), 10% foetal bovine serum (FBS) and DMEM-L glucose (low glucose) at 37 °C and 5% CO2 conditions. Two membranes (sections with diameter of 0.75 cm) were selected from each group and sterilized for 15 min under UV. Cells which were aligned on the culture plate as confluent single layer in the 1 day culture, was detached from the surface by treating with Trypsin-EDTA (0.25% trypsin, 0.02% EDTA) solution. Suspended cells were counted with a counting equipment (Invitrogen Countess Automated Cell Counter, Korea). Membranes were place in 24 well plates by their PEG capsule containing sides up. 35 μl of cell suspension containing 1 × 105 cells were added onto each membrane. After cultivation, they were incubated for 30 min (37 °C’ta 5% CO2) in order to maintain cell attachment before adding cell culture medium. After this incubation period, 200 μl of cell culture medium was added into each well. Cells cultivated on the membranes were incubated for two days at 37 °C and 5% CO2 conditions. After two days of incubation, membranes were transferred to another plate and dried for one day in order to get SEM images. Dried membranes were then fixed on the SEM platform by the side to be analysed on top. These surfaces were coated with carbon by a Polaron SC500 Sputter Coater equipment for 15 min. The coated surfaces were investigated by using an scanning electron microscope (JEOL JSM-5600 SEM, United Kingdom). Different regions of the membrane were photographed with different magnifications.
Cytotoxicity
L929 fibroblast cells were cultivated on the 24-well plates as 1 × 104 cells in each wells. Cells were cultivated on four different membranes (0, 1, 2, 3) having different amounts of centaury oil content as well as direct interaction with different amounts of H. perforatum oil and incubated for one night at 37 °C and 5% CO2. Following one night of incubation, cells were incubated in dark for three hours by adding freshly prepared colourless (phenol resist) medium and WST-1 solution to each well after removal of mediums in the wells. After the completion of the incubation period, samples were analysed with the use of an ELISA plate reader (BioTek PowerWave XS2, USA) at 440 nm wavelength. Cell viability (%) of membranes was calculated using the following equation [Citation24].
(4)
(4)
where OD is optic density
Apoptosis and necrosis
The apoptotic and necrotic effect of the produced membranes on L929 fibroblast cells were investigated by using double staining technique. Double stain is a mixture of stains that contains 1% and 5% of propidium iodide (PI) and Hoechst stains, respectively. Since Hoechst stains the nucleus of both alive and apoptotic cells to blue, PI stains only the nucleus of the necrotic cells to red, while both bonds to DNA and it can be observed with fluorescent microscope at different wavelengths (Hoechst: 350 nm, PI: 535 nm). Besides, the stain solution contains 1% of ribonuclease A (RA). RA is used to avoid non-specific bonding by destroying cytoplasmic RNA. L929 fibroblast cells were cultivated in 24-well plate with 1 × 104 cells in each well. Then, these cells were treated with four different membranes (0, 1, 2, 3) having different amounts of centaury oil content and incubated one night at 37 °C and 5% CO2 conditions. Following one night incubation, medium in each well was removed, and 150 μl double stain solution was carefully added to each well, and incubated for 15 min in dark for interaction with stain. Apoptotic and necrotic cells were then investigated with a fluorescent microscope (Inverted Microscope, Leica DM6000B, Germany).
Results and discussion
Chemical structure (FTIR) of the membranes
FTIR spectra () were analysed, stretching bands of C-H and C-O in PEG structure were observed at 2882 cm−1 and 1094 cm−1 respectively. Peaks observed in the range between 1467 and 1341 cm−1 were associated with the C-H bending. CH2 bonding specific to PCL were observed at 2865 cm−1 wavenumbers. Similarly, CH2 bonding specific to PEG was observed nearby wavenumbers. Peaks associated with CH2 bonding in the membranes formed with PEG-PCL copolymer, were observed between the peaks associated with PEG and PCL. Also, relatively small peak observed at 2942 cm−1 that is found in the region associated with C-H stretching, is specific to PCL and was observed in the structures of PCL containing membranes. Peak observed at 1721 cm−1 shows the presence of the carbonyl group (C=O) in PCL structure. Peak at this wavenumber was also observed for membranes made of PEG-PCL. Peaks associated with C-H stretching band specific to H. perforatum oil was observed at 2854 an 2923 cm−1 [Citation23,Citation25]. Addition of oil to PEG-PCL membranes resulted with a shift in the C-O stretching peaks. Peak observed at 1744 cm−1 was associated with the carbonyl groups present in the ester bonds. Same peak was evident in both ester bond containing PCL and PCL membranes loaded with H. perforatum oil. Results obtained from FTIR-ATR analyses revealed that H. perforatum oil was successfully loaded to the membranes and compounds integrated with the structure did not resulted with any change in the chemical structure due to the interactions with the solvent.
Morphology (SEM)
Non-woven PCL nanofibres on the top layer of the membranes (), PCL fibres and thin PEG fibres carrying the PEG capsules with H. perforatum oil on the bottom layer of the membranes in contact with the wound () can clearly be seen on the SEM images.
Figure 2. SEM images of top and bottom layers of 7% (v/v) H. perforatum oil containing PEG-PCL membrane: top layer of the membrane made of PCL fibres, (A) x200 magnification, (B) x400 magnification; bottom layer of the membranes in contact with the wound (PCL fibres and thin PEG fibres carrying the PEG capsules with H. perforatum oil), (C) x400 magnification, (D) x1000 magnification.
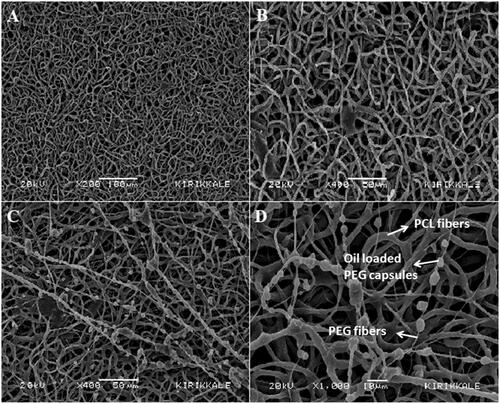
Mechanical properties (tensile tests)
Young's Modulus values of the membranes were found to be between 0.90 and 3.17 MPa (). Young's Modulus values for the membranes 1, 2 and 3 were higher than the value for membrane 0. And therefore H. perforatum oil addition to the membranes within PEG capsules resulted with an increase in stiffness. Wound dressings shall maintain adequate tensile strength, elasticity and flexibility in order to cover the wound area. It is concluded that the membranes were elastic enough to be used for dressing the wound. The results of tensile properties of the membranes were comparable with the other results [Citation19,Citation26] and also human skin [Citation27].
Table 2. Tensile test results for the membranes.
Table 3. Contact angle values of the membrane layers with/without oil.
Density and porosity
Density and the specific volume (Vm) were found to be 0.3 g/cm3 and 3.333 cm3/g respectively for the membrane 0, and 0.477 g/cm3 and 2.096 cm3/g for the membrane 1. Use of oil with the membranes resulted with an increase in density and decrease in specific volume as expected. Hence, porosity of the membranes were decreased significantly by oil use. Porosity was calculated as 73% for membrane 0 and 56% for membrane 1.
Gas permeability
Gas permeability of the wound dressing materials is an important property in terms of maintaining the oxygenation of the wound area beside the systemic blood stream, and removing the carbon dioxide present at the wound area and excess of the water vapour originated with exudate. Water vapour permeability of the wound dressing materials should be between 90–2890 g/m2/day [Citation28]. Air permeation values for membranes 1and 0 were measured as 672 g/m2/day and 1128 g/m2/day, respectively. These values were determined to be in the limits defined increase in density together with the decrease in porosity of the membranes resulted with significant decrease in gas permeability. But it can be concluded that gas permeability of both membranes were good.
Hydrophilicity (contact angle measurements)
Contact angle measurements were conducted for determination of hydrophilic/hydrophobic character of the membranes (). It can be said that then liquid wets the surface if θ < 90°, or does not wet the surface if θ > 90°. θ < 10° shows stronger wetting (super-hydrophilic), θ > 150° shows strong non-wetting (super-hydrophobic) character [Citation29]. The average of the contact angle measurements for upper layer of the membranes (electrospun PCL layer) was 97.18°. This upper was found to be slightly hydrophobic. Since the average value of the contact angle measurements for bottom layer that is in contact with the wound (co-electrospun PEG-PCL layer) was 69.94°, this value increased to 86.13° when oil was used loaded in this layer (co-electrospun PEG-PCL layer with oil). Oil decreased the hydrophilicity of the membrane significantly due to its hydrophobic structure. Absorption of the exuda by the membrane is important for moisture control [Citation1]. Upper layer that was formed with non-woven PCL nanofibres showed hydrophobic character and kept the moisture in the bottom layer. Using PEG polymer with hydrophilic character gave the bottom layer of the membrane the ability to absorb moisture. However, oil with hydrophilic character balanced the hydrophilicity of the bottom layer. Therefore, the risk of cell attachment was minimized. Extremely hydrophobic wound dressing membranes may cause irritation at the wound area. Extreme hydrophilicity may lead cell adhesion and then cell proliferation on the membrane surface. On the occasion of sticking of the wound tissue to membrane, serious problems may occur at the healing wound area upon removal of the wound dressing. Because of these, it is undesired for the wound dressing material to have extremely hydrophilic or extremely hydrophobic characters. It is concluded that the membrane exhibited a balanced hydrophilicity, and therefore, it is suitable within this context for use as wound dressing material.
Wettability (swelling ratio%)
Control of moisture at the wound area is very important [Citation1]. Extreme moisture or the dryness of the wound delays healing [Citation1]. Capacity of exudate absorption (wettability) of the wound dressing is important in terms of maintaining moisture control of the wound. Degree of swelling ratios (%) of the membranes with/without oil (Membrane 0 & 1) sketched in . Membranes exhibited fast and linear swelling ratios (%) within the first three minutes. At third minute, swelling ratios of the membrane 0 (around 90%) was higher than membrane 1 (around 170%). Swelling was balanced at 12th minute for membrane 1 (around 120%) and at 25th minute for membrane 0 (around 290%). Comparing the swelling ratios of the membranes at balance, swelling capacity decreased by half in the case of oil loading. It can be concluded that decreasing porosity together with hydrophilicity by oil loading resulted with a decrease in the capacity to absorb moisture. Wettability of the membrane 0 was greater than wettability of the membrane 1; however, both membranes have satisfactory capacities to absorb moisture [Citation28,Citation30].
Mass loss in time (weight remaining %)
Membranes were incubated in PBS and dry weight of the membranes was recorded after incubation of predetermined time intervals (2, 4, 6, 8, 10, 12, 14 days) then remaining weight (%) values were calculated (). Membrane 0 exhibited a faster weight loss compared to membrane 1 within the first 4 days. Total weight loss for each membrane was similar around 20% on sixth day. Membrane 1 exhibited faster weight loss between eight and 12 days. Total weight loss for membrane 0 and membrane 1 after 14 days was around 25% and 32%, respectively.
Controlled release of H. perforatum oil
Controlled release of H. perforatum oil from the polymeric membrane occurred by dissolution of PEG in aqueous medium. Thus, release rate was controlled by dissolution rate of PEG. H. perforatum oil release was investigated by spectrophotometric analyses of samples that were taken every two hours within first two days and every 12 hours within following days. H. perforatum oil release from membrane 1 was fast until day six. After day six, controlled release of H. perforatum oil was slightly decreased. At 11th day release was significantly decreased due to dissolution of great majority of the PEG capsules. Release graphs ( inset graph) confirmed continuous and controlled release of H. perforatum oil from the membranes to the medium occurred during 14 days.
Antibacterial activity tests
Antimicrobial activity of the H. perforatum oil loaded electrospun PEG-PCL membranes were investigated on Staphylococcus aureus and Escherichia coli which are Gram-positive and Gram negative bacteria, respectively. There were no inhibition zones observed on antimicrobial activity images () for membrane 0. Therefore, membrane 0 did not exhibit antimicrobial activity on S. aureus and E. coli. Inhibition zones were evident for membranes loaded with H. perforatum oil (membranes 1, 2, 3). Inhibition zone diameters (mm) were measured and summarized on . Oil loaded membranes exhibited antimicrobial activity on E. coli irrelevantly to the amount of the oil loaded. However, antimicrobial activity on S. aureus increased significantly with the increasing amount of oil loaded. The reason for observing different zones for these two organisms is that S. aureus is Gram positive, and E. coli is Gram negative. The wall surrounding the cell membrane of S. aureus forms a diffusion barrier for penetration of oil. And therefore it made the membrane difficult to show antimicrobial activity. Thus, the membranes showed better antimicrobial activity on S. aureus with increasing amount of oil content. Controlled release of the oil, entrapped in the membrane structure by encapsulation with PEG polymer, from the membrane showed antimicrobial activity on both gram positive and gram negative bacteria. Therefore antimicrobial activity property was brought in the polymeric membranes, that had no antimicrobial activity, by integration in the structure with H. perforatum oil that's known to have antimicrobial activity [Citation12].
Table 4. Inhibition zone diameters of membranes (membrane 0, 1, 2, 3) on E. coli and S. aureus.
Interaction of membranes with cells
SEM images of the membrane surfaces after two days of incubation were given in . Since PEG capsules/fibres present on the surface of the membrane were dissolved in the medium, surface images exhibited significant difference compared to surface image on . This confirms the dissolution of PEG capsules and release of the oil to the medium. There were no cells observed to be attached or proliferated on the surface of the membrane after incubation with cells. This situation confirms that wound dressing material did not adhere on the wound. It is important that wound dressing materials shall not adhere on the wound [Citation2]. Thus, it would be possible to remove the wound dressing material without causing any deformation on the wound surface after healing.
Biocompatibility (cytotoxicity/WST-1)
Cell viability values of the groups were summarized in and . A slight decrease was observed in cell viability value for the membrane 0 compared to the negative control but it was not toxic. Since cell viability increased for membrane 1, a more significant increase was observed for membrane 2 and membrane 3. Depending on this, it was concluded that increasing oil content in the membrane resulted with increase in cell viability. Cytotoxicity of H. perforatum oil in different volumes (1, 2, 3 μl) was investigated under same conditions and it was observed that cell viability increased compared to negative control. Both H. perforatum oil and membranes loaded with H. perforatum oil increased cell viability by increasing the metabolic activity. Hence, it can be concluded that both membranes and the oil were biocompatible.
Figure 8. Apoptosis/necrosis (%) and cell viability (%) of control groups (positive/negative) and membrabes (0, 1, 2, 3).
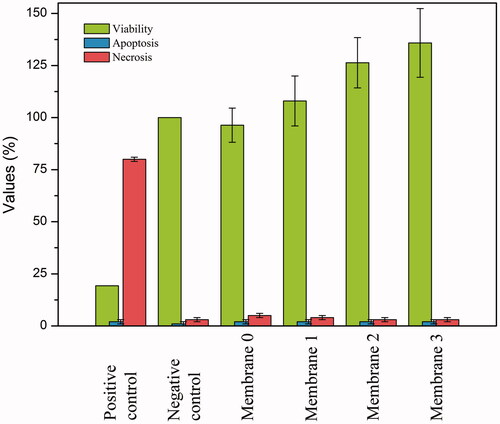
Table 5. Cytotoxicity/WST-1 test results and apoptosis/necrosis (%) values.
Apoptosis and necrosis
The apoptotic and necrotic effect of the produced membranes onto L929 fibroblast cells were investigated by using Hoechst/PI double staining technique. Apoptosis/necrosis assay work was carried out for both negative control with cells only (A,B) and positive control treated with DMSO (C,D). Fluorescence microscopy images of apoptosis/necrosis assay were presented for membranes in and for control groups in . Images on the left column show the predominantly viable cells in blue colour due to Hoechst staining, and images on the right column show the non-viable necrotic cells in red colour due to PI staining. Bright spots on the images showing the apoptotic cells indicates the necrotic cells, which are stained in red colour on the opposite column. It is evident that polymeric membranes did not cause significant increase in the tendency to apoptosis and necrosis. Apoptotic and necrotic indexes were obtained as 2 ± 1 and between 3 ± 1 – 5 ± 1 respectively ( and ). Apoptotic/necrotic effect of the membranes were found to very low. Ratios for both apoptotic and the necrotic cells were decreased by increasing H. perforatum oil content. This is also in consistent with the cytotoxicity results. Therefore, biocompatibility of the membranes were enhanced by increasing H. perforatum oil content, and apoptotic/necrotic effect decreased.
Conclusion
There are many different types of wounds classified as acute and chronic. Wound healing consists of complex processes that differ from patient to patient and wound type. Generally modern wound dressing materials are used to protect the wound from external environment and control moisture around the wound area. However, curative oils are also known to be used in wound healing. But direct application of the oil to wound avoids air contact and makes patient compliance difficult. In this study, H. perforatum oil, known to have curative effect, was integrated to polymeric membranes that holds the properties of an ideal wound dressing material such as gas permeability, antimicrobial activity, non-adherence to wound, absorption of moisture, elasticity, biocompatibility, etc.
Double layered polymeric membranes were produced by using electrospinning and electrospraying techniques. The upper layer made of electrospun PCL fibres was designed to maintain the membrane integrity and elasticity during healing process. Bottom layer to be in contact with the wound was made of concurrently electrospun PCL and PEG fibres. There are oil droplets on the PEG fibres, which were encapsulated by electrospraying. The release of H. perforatum oil encapsulated within PEG polymer was controlled by dissolution of PEG polymer in aqueous medium. Polymeric PCL and PEG fibres and also H. perforatum oil encapsulated in PEG capsules, could be produced as desired in the wound dressing material design. Membranes had sufficient elasticity in order to be used as wound dressing material. H. perforatum oil content have led to increase in density, and decrease in porosity and gas permeability. Also hydrophobic character of the H. perforatum oil decreased the hydrophilicity of the membranes. Wettability of the membranes decreased by decreasing porosity and hydrophilicity. Porosity, gas permeability, hydrophilicity and wettability values were decent when compared with literature. It is observed that membranes exhibited weight loss and release of H. perforatum oil to the medium in a controlled manner, by dissolution of PEG fibres and capsules in aqueous medium. H. perforatum oil-loaded membranes showed antimicrobial activity on S. aureus and E. coli. Cell culture studies revealed that, the membranes do not have the risk of adhesion to wound, they do not have apoptotic/necrotic effects, both the membranes and H. perforatum oil are biocompatible; moreover, they have proliferative effect on cells.
Successful integration of curative oils to modern wound dressing materials was proved to be possible by this study. Also, it suggests an approach that enables the integration of curative oils with electrospun polymeric membranes having different designs, by encapsulation using electrospraying technique. It was concluded that the membranes produced within the context of this study has the potential to be used as wound dressing material.
Disclosure statement
No potential conflict of interest was reported by the authors.
Additional information
Funding
References
- Zahedi P, Rezaeian I, Ranaei‐Siadat SO, et al. A review on wound dressings with an emphasis on electrospun nanofibrous polymeric bandages. Polym Adv Technol. 2010;21:77–95.
- Boateng JS, Matthews KH, Stevens HN, et al. Wound healing dressings and drug delivery systems: a review. J Pharm Sci. 2008;97:2892–2923.
- Geronemus RG, Robins P. The effect of two new dressings on epidermal wound healing. J Dermatol Surg Oncol. 1982;8:850–852.
- Dickinson LE, Gerecht S. Engineered biopolymeric scaffolds for chronic wound healing. Front Physiol. 2016;7:341.
- anikatherapeutics.com [Internet]. USA: Anika Therapeutics; [cited 2018 Sept 12]. Available from: https://www.anikatherapeutics.com/products/dermal/hyalosafe/
- systagenix.com [Internet]. US: Systagenix; [cited 2018 Sept 12]. Available from: http://www.systagenix.ae/our-products/manage-odour/actisorbandtrade-plus-25-dressing-274
- Süntar I, Akkol EK, Keleş H, et al. A novel wound healing ointment: a formulation of Hypericum perforatum oil and sage and oregano essential oils based on traditional Turkish knowledge. J Ethnopharmacol. 2011;134:89–96.
- Thakur R, Jain N, Pathak R, et al. Practices in wound healing studies of plants. Evid Based Complementary Altern Med. 2011;2011:1.
- Özkorkmaz EG, Yusuf ÖZAY. Yara iyileşmesi ve yara iyileşmesinde kullanılan bazı bitkiler. Türk Bilimsel Derlemeler Dergisi. 2009;2:63–67.
- Wölfle U, Seelinger G, Schempp CM. Topical application of St. John's wort (Hypericum perforatum). Planta Med. 2014;80:109–120.
- Rančić A, Soković M, Vukojević J, et al. Chemical composition and antimicrobial activities of essential oils of Myrrhis odorata (L.) Scop, Hypericum perforatum L and Helichrysum arenarium (L.) Moench. J Essential Oil Res. 2005;17:341–345.
- Saddiqe Z, Naeem I, Maimoona A. A review of the antibacterial activity of Hypericum perforatum L. J Ethnopharmacol. 2010;131:511–521.
- Prisăcaru AI, Andriţoiu CV, Andriescu C, et al. Evaluation of the wound-healing effect of a novel Hypericum perforatum ointment in skin injury. Rom J Morphol Embryol. 2013;54:1053–1059.
- Kim GH, Yoon H. A direct-electrospinning process by combined electric field and air-blowing system for nanofibrous wound-dressings. Appl Phys A. 2008;90:389–394.
- Han D, Gouma PI. Electrospun bioscaffolds that mimic the topology of extracellular matrix. Nanomedicine. 2006;2:37–41.
- Sinha M, Banik RM, Haldar C, et al. Development of ciprofloxacin hydrochloride loaded poly (ethylene glycol)/chitosan scaffold as wound dressing. J Porous Mater. 2013;20:799–807.
- Shingel KI, Di Stabile L, Marty JP, et al. Inflammatory inert poly (ethylene glycol)–protein wound dressing improves healing responses in partial‐and full‐thickness wounds. Int Wound J. 2006;3:332–342.
- Bader RA, Herzog KT, Kao WJ. A study of diffusion in poly (ethyleneglycol)-gelatin based semi-interpenetrating networks for use in wound healing. Polym Bull. 2009;62:381–389.
- Augustine R, Kalarikkal N, Thomas S. Electrospun PCL membranes incorporated with biosynthesized silver nanoparticles as antibacterial wound dressings. Appl Nanosci. 2016;6:337–344.
- Kil’deeva NR, Vikhoreva GA, Gal’braikh LS, et al. Preparation of biodegradable porous films for use as wound coverings. Appl Biochem Microbiol. 2006;42:631–635.
- Rabiller-Baudry M, Gouttefangeas F, Lannic J, et al. Coupling of SEM-EDX and FTIR-ATR to (quantitatively) investigate organic fouling on porous organic composite membranes. In: Mendez-Vilas A, editor. Current Microscopy Contributions to Advances in Science and Technology. 2012. p. 1–11. Formatex Research Center, Badajoz, Spain.
- Gupta B, Saxena S. Chitosan-polyethylene glycol coated cotton membranes for wound dressing. Indian J Fibre Text Res. 2011;36:272–280.
- Zavoi S, Fetea F, Ranga F, et al. Comparative fingerprint and extraction yield of medicinal herb phenolics with hepatoprotective potential, as determined by UV-Vis and FT-MIR spectroscopy. Not Bot Horti Agrobo. 2011;39:82–89.
- Hussien NA, Işıklan N, Türk M. (Aptamer-functionalized magnetic graphene oxide nanocarrier for targeted drug delivery of paclitaxel. Mater Chem Phys. 2018;211:479–488.
- Huck-Pezzei VA, Pallua JD, Pezzei C, et al. Fourier transform infrared imaging analysis in discrimination studies of St. John's wort (Hypericum perforatum). Anal Bioanal Chem. 2012;404:1771–1778.
- Johnson J, Niehaus A, Nichols S, et al. Electrospun PCL in vitro: a microstructural basis for mechanical property changes. J Biomater Sci Polym Ed. 2009;20:467–481.
- Daly CH, Odland GF. Age-related changes in the mechanical properties of human skin. J Invest Dermatol. 1979;73:84–87.
- Kweon H, Ha HC, Um IC, et al. Physical properties of silk fibroin/chitosan blend films. J Appl Polym Sci. 2001;80:928–934.
- Zhao T, Jiang L. Contact angle measurement of natural materials. Colloids Surf B Biointerfaces. 2018;161:324–330.
- Patil BS, Mastiholimath VS, Kulkarni AR. Development and evaluation of psyllium seed husk polysaccharide based wound dressing films. Orient Pharm Exp Med. 2011;11:123.