Abstract
Tumour drug delivery using nanocarriers is attracting ample attentions due to their high drug-loading capacity. Regarding specific tumour microenvironment properties as acidic pH, smart nanocarriers with the ability of responding to the microenvironment, can have a profound effect on the level of drug release and subsequent tumour treatment. In this study, by combining the advantages of multiwall carbon nanotube and pH-sensitive nanogels, multifunctional magneto/pH-responsive nano-hybrid system is developed to deliver the doxorubicin as a general cancer chemotherapeutic drug. The chemical and physical properties of the nanocarrier, as well as drug-loading efficiency and drug releasing characteristics were analysed. It was showed functionalized CNT has low pH-responsiveness in acidic environment, whereas chitosan-coated magnetic nanocomposite can result in greater pH-responsiveness and subsequently higher drug release over a week compared to nanocomposite system without chitosan. This behaviour was proved in Live/Dead assay of the U-87 glioblastoma cell lines exposed to DOX release supernatant at different time intervals so that significant effect of DOX supernatant on cancer cell proliferation suppression was showed.
Introduction
Carbon nanotube (CNT) as a one-dimensional nanomaterial is attracting great attention in different filed of nanomedicine. It has also been revealed that stable form of CNT like functionalized ones enable the field of nanotechnology to potentially emerge as a powerful drug delivery system for both proposes of targeted or localized therapeutic agents’ delivery [Citation1,Citation2]. Although f-CNTs have great drug-loading capacity, they cannot be used solely in urgent field of targeted drug delivery and hence composite forming of CNTs with magnetic nanostructures make them as a promising carrier for magnetic-based drug delivery. Regarding cancer treatment, using pH-sensitive materials for responding to the decreased pH of the tumour microenvironment is considered as a promising approach for intracellular or peri-tumoural release of cancer therapeutics [Citation3,Citation4]. So, CNT-based nano-hybrids with stimuli-responsive polymeric materials can be used as a suitable candidate for either controlled or on-demand drug release [Citation5,Citation6].
Regarding using, smart pH-sensitive microgels for tumour cell drug release, Zhang et al used chitosan-derived microgels loaded with methotrexate disodium for target and localized delivery to HeLa cancer cells [Citation7]. Moreover, due to using CNT-based pH-sensitive nanostructures for tumour cell drug release, CNT-hyaluronic conjugates were used. It was reported that DOX was complexed with CNT using hydrophobic interactions and subsequently released in the low pH condition under intracellular endolysosomal conditions [Citation8].
Due to developing a new pH-responsive material for anticancer drug delivery agent, chitosan nanogel was coated on the surface of the MnFe2O4 magnetic nanoparticle. Subsequently, they were deposited on the surface of the functionalized CNTs to form a covalent-bonded pH-/magneto-responsive CNT. To the best of our knowledge, it is the first report of CNT decoration with MnFe2O4 contained pH-responsive nanogel as a magnetic guidance drug-targeting carrier which is sensitive to acidic pH of tumour microenviroenment.
Materials and method
Chitosan-coated MnFe2O4 nanogel synthesis
MnFe2O4 nanoparticles were synthesized through chemical co-precipitation method using Fe(NO3)3.6H2O and MnCl2.4H2O precursors. Chitosan coating was also conducted as followed. 10 ml of MnFe2O4 nanoparticle suspension with concentration of 1.5%wt was prepared in deionized water and subsequently the same volume of the 20 mg/mL CTAB solution was added. The final mixture was sonicated in the ice bath for 30 min. The procedure was followed by dropwise adding 100 ml chitosan solution (0.02 g chitosan powders dissolved in 100 ml of 1% (w/v) in acetic acid solution) and the whole solution was mechanically stirred at 1000 rpm for 2 h. Prepared chitosan coated nanoparticles were magnetically decanted and purified by several washing steps in deionized water.
Magnetic nanogel decoration on the CNT
Multiwall carbon nanotube (MWCNT) were first functionalized through surface etching in the solution of H2SO4 (96%), HNO3 (60%) and KMnO4 with ratio of (3:1:4) to MWCNT. 1 g MWCNT was added to the above solution followed by refluxing in boiling temperature for 24 h. The filtered functionalized CNT was subsequently used for making nanocomposite with magnetic chitosan nanogel. This procedure was conducted by adding 15 mg/mL chitosan-coated magnetic nanoparticles to the certain concentration of CNT in DI water followed by adding 2 ml of EDC and NHS with concentrations of 5 mg/mL 6 mg/mL respectively. Through this reaction, activated carboxyl groups of the functionalized CNTs are prone to covalently bind to NH3+ groups of the chitosan. The mixed solution was sonicated for 2 h with low amplitude in the ice bath.
Physical and chemical characterization
Phase identification of the hybrid sample was studied by Philips X’pert Pro prefix powder X-ray diffractometer using monochromatic CuKα radiation (λ = 1.5405 A). The morphology of CNT as well as magnetic decorated hybrid CNT were observed using field-emission scanning electron microscope (Hitachi S-4800 FESEM). Vibrating sample magnetometer (VSM, Meghnatis Daghigh Kavir Co., Iran) was used to measure the magnetic properties of the samples at room temperature. The mean size and size distribution of the functionalized CNTs before and after magnetic decoration as well as zeta potential variation were measured by dynamic light scattering (DLS) and zeta sizer respectively (Malvern, Autoszer 4700).
Anticancer drug loading, release and cell viability study
Both f-CNT and CNT nanocomposite were mixed with DOX-HCl solution (1 mg/mL) at 4 °C for 24 h. Finally, loaded particles were magnetically separated and washed three times with PBS to eliminate the un-bonded surface DOX molecules. For drug release study, 1 mg/mL suspensions of DOX-loaded f-CNTs and nanocomposite prepared in 2 ml PBS with adjusted pH 5.3 (representing tumor microenvironment pH) or pH 7.4 (the physiological pH). The solution was kept at 37 °C and CNT nanocomposites were magnetically separated at different time intervals. The concentration of DOX in the supernatant was quantified using UV–Vis spectroscopy at the wavelength of 480 nm. To visualize the effect of DOX release concentration at each pH and time intervals, Live-dead assay was conducted using 1 µM calcein AM and 4 µM ethidium homodimer. The whole experiment was illustrated in so that the schematic view of the loading and releasing behaviour of the CNT-hybrid nanogels at acid pH of the tumour microenvironment is depicted. To quantify the amount of drug toxicity in each time intervals, PrestoBlue assay was used. It can measure the metabolic activity of the live cells with the fluorescent excitation and emission wavelengths of 560 nm and 590 nm respectively. In this regard, 10% PrestoBlue reagent of the total culture medium, was added directly to each well and incubated for 20 min.
Results and discussion
As illustrated in , B there is significant difference in the CNT morphology after magnetic nanogel decoration so that dispersed naogels with diameter of around 50 nm are somewhat aggregated along the spaghetti CNT fibers to form 200 nm clusters. As could be seen in , XRD pattern of the synthesized hybrid magnetic CNT is representative of both CNT and MnFe2O4 phases in the structure. According to JCPDS card no. 26–1079, the diffraction peaks at 2Ɵ =26.08° and 43.08° are related to the (002) and (100) planes of the f-CNT, while according to JCPDS card of 74–2403, characteristic peaks of the MnFe2O4 present at 2Ɵ of 35.2°, 56.6° and 62.1° for (311), (511) and (440) planes, respectively.
Figure 2. SEM images of the (A) f-CNT and (B) hybrid Chi-MnFe2O4/CNT, Scale bare is 100 nm. C) XRD analysis of the hybrid Chi-MnFe2O4/CNT sample.
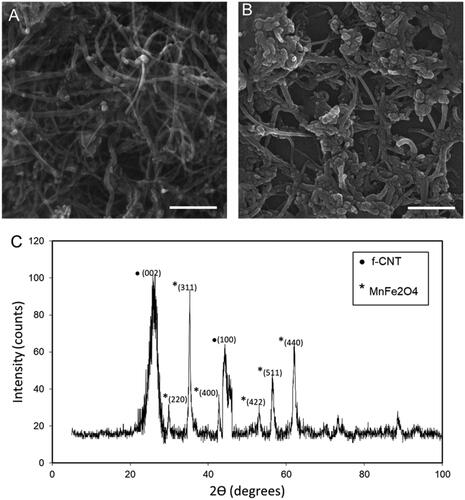
Regarding FTIR analysis (), absorption bands around 3436 cm−1 are characteristic of stretching vibration of O-H groups on the surface of CNTs. Moreover, according to FTIR spectra of the hybrid material, absorption bands at 3450 cm−1 is indication of O-H vibration positioned on the amine bands in chitosan structure [Citation9]. Absorption peak at 2921 and 2855 cm−1 corresponds to vibration of C-H groups in CNT structure. Two sharp peaks of N-H vibration group and C-N vibration of the chitosan amine groups at 1640 cm−1 and 1419 cm−1, respectively, show the presence of the chitosan in the structure of the hybrid nanotube [Citation10]. In addition, characteristic peaks of the MnFe2O4 is appeared at the range of 600 cm−1 to 900 cm−1 showing the Fe-O stretching bond frequency in the spectra of the hybrid nanotube. These peaks are absent in the spectra of the pure functionalized nanotube. According to , magnetization behaviour of the magnetic nanoparticle, chitosan-coated nanoparticles and hybrid carbon nanotube was assessed through using VSM for dry powder in the field of 12 kOe at room temperature. The value of the saturation magnetization of the experimental samples are obtained to be around 89 emu/g for MnFe2O4 nanoparticles while this value is dropped considerably to 68 emu/g and 48 emu/g after surface treatment and conjugation to carbon nanotube, respectively. This value are comparable to the value reported for chitosan-coated magnetic nanoparticles (53 emu/g) and shows its feasibility for target drug delivery systems [Citation11]. Magnetic saturation decrement of the composite nanoparticles compared to pure MnFe2O4ones is assumed to be due to chemical surface bonding of the magnetic nanoparticle's atoms to the CNT. Through this reaction, orientation of surface atom spins and therefore and the net magnetic moment of the atoms is altered [Citation12]. None of the pure magnetic nanoparticles neither surface treated ones do not show any corercivity at room temperature which is indication of single domain nanoparticle presence and their superparamagnetic properties. Absence of the remanence magnetic field after magnetic field removing also indicates the capability of this hybrid/nanotube system for efficient magnetic-based drug delivery of chemotherapeutic drugs to the tumour microenvironment.
Figure 3. (A) FTIR spectra of the (I) f-CNT and (II) Chi- MnFe2O4/CNT samples. (B) VSM plot of the MnFe2O4, Chitosan coated MnFe2O4 and the hybrid Chi- MnFe2O4/CNT samples.
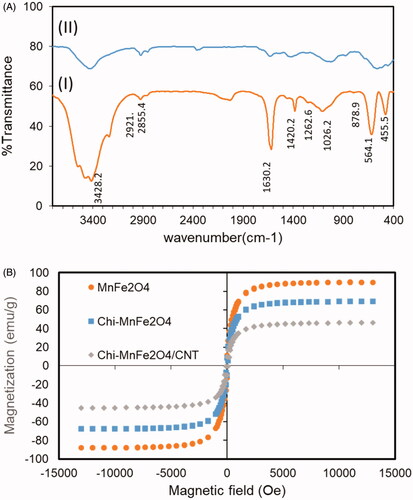
As shown in the , zeta potential of the hybrid/nanotube decreased from −18 mv for f-CNT to −9.09 mv. Abundance of the carboxylic functional group on the surface of the chemically modified nanotubes with net negative zeta potential of around −18 mv make it potent to electrostatically adsorb positive charge chitosan nanogels with NH3+ groups. The primary physical absorption is changed to the covalent bonding through EDC-NHS linking mechanism. According to , hydrodynamic particle diameter was increased through magnetic nanogel decoration on the surface of the CNTs so that diameter of 90 and 190 nm are reported for f-CNT and Chi- MnFe2O4/CNT samples, respectively. It is clear that both CNT-based samples present two particle size distribution peaks which indicates the morphology of the samples with the aspect ratio more than 1 [Citation13].
Figure 4. (A) Zeta potential analysis of the samples (B) before magnetic decoration, f-CNT and (C) after magnetic decoration, Chi-MnFe2O4/CNT. (E) Effect of magnetic decoration on the hydrodynamic diameter of the f-CNT.
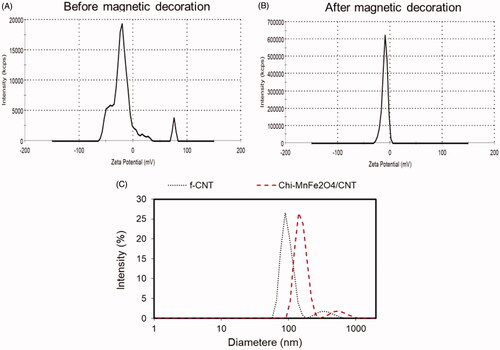
Drug-loading capacity of the both f-CNT and hybrid CNTs calculated as (weight per cent of DOX loaded on CNT/weight per cent of DOX initially added) × 100 and reported to be 71% and 92%, respectively. Greater loading efficiency of the hybrid nanotube is likely to be reason of DOX diffusion in the chitosan nanogel structure and electrostatic interaction as well as π–π interaction of carbon atoms with DOX molecule [Citation7,Citation14,Citation15]. As shown in , the release of the DOX from hybrid CNTs is more pH-triggered compared to f-CNT. The drug release curve shows the primary burst release for Chi- MnFe2O4/CNT sample for both pH which is related to the release of surface un-bonded DOX molecules. This trend is followed by slow DOX release to the extent of 48% and 82% for pH 5.3 and 7, respectively, while we cannot observe any significant difference between cumulative release profiles of the f-CNT at two different pH of 5.3 and 7. The main reason of the greater pH-dependency release of the hybrid nanotube is dedicated to two mechanisms. First, the competing role of the H+ ions on hydrogen bonding weakening between DOX and CNT atoms [Citation15] and second the electrostatic repulsion between protonated amino groups of the chitosan structure which enables more drug release form swollen nanogel network at reduced pH [Citation7,Citation16].
Figure 5. Cumulative release profile of the (A) Chi- MnFe2O4/CNT and (B) f-CNT samples in two experimental pH of 5.3 and 7.
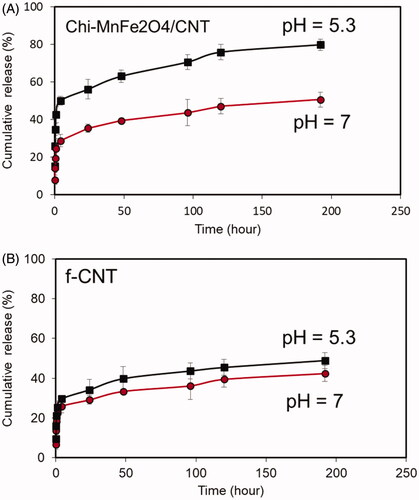
shows the live-dead imaging of the U-87 cells incubated with DOX supernatant from the Chi-MnFe2O4/CNT sample at the specific time intervals. It is blatant that by time progressing form Day 0 to Day 7 the number of dead cells stained with red fluorescent dye is increased and the level of cell attachment on the bottom of the culture plate is decreased. Moreover, metabolic activity of the monolayer-cultured cells was analysed using presto-blue assay in different time intervals of the experiment and was shown in . The results of presto-blue confirm the live-dead assay data, so that, cell viability decreased over time from day 1 to day 7 compared to each control samples. It is shown that accumulative concentration of the released drug has positive effect on cancer killing so that, Doxorubicin slows or stops cancer cells by blocking intracellular topoisomerase II — enzyme [Citation17]. Generally, it is obvious that amount of released drug at each time point can have a significant effect on cell growth and division. Therefore, the controlled released concentration of the DOX obtained from the release profile is effectual in cancer cell proliferation suppressing which could be finally considered as a potential choice for targeted, smart and controlled drug delivery to tumours.
Conclusion
In this research, a novel hybrid/carbon nanotube with magnetic properties was firstly developed to be considered as a potential nanocarrier for the smart drug release at the local of the tumour microenvironment. It was showed that this hybrid material has more drug-loading capacity and more smart release response to acidic environment of the tumour in comparison to just functionalized CNT. Further works are focused on the target ability of these hybrid/CNTs based on antibody conjugation as well as cancer cell killing efficiency in three-dimensional (3D) tumour models to be considered as an effective drug carrier with the emulated tumour conditions.
Disclosure statement
No potential conflict of interest was reported by the authors.
References
- Zhang W, Zhang Z, Zhang Z. The application of carbon nanotubes in target drug delivery systems for cancer therapies. Nanoscale Research Lett. 2011;6:555.
- Prato M, Kostarelos K, Bianco A. Functionalized carbon nanotubes in drug design and discovery. Acc Chem Res. 2008;41:60–68.
- Spizzirri UG, Hampel S, Cirillo G, et al. Spherical gelatin/CNTs hybrid microgels as electro-responsive drug delivery systems. Int J Pharm. 2013;448:115–122.
- Prakash S, Malhotra M, Shao W, et al. Polymeric nanohybrids and functionalized carbon nanotubes as drug delivery carriers for cancer therapy. Adv Drug Deliv Rev. 2011;63:1340–1351.
- Molina M, Asadian-Birjand M, Balach J, et al. Stimuli-responsive nanogel composites and their application in nanomedicine. Chem Soc Rev. 2015;44:6161–6186.
- Seyfoori A, Koshkaki MR, Majidzadeh-A K, 26 – nanohybrid stimuli-responsive microgels: a new approach in cancer therapy. In: Holban AM, Grumezescu AM, editors. Nanoarchitectonics for smart delivery and drug targeting. William Andrew Publishing; Norwich, NY. 2016. p. 715–742.
- Zhang H, Mardyani S, Chan WC, et al. Design of biocompatible chitosan microgels for targeted pH-mediated intracellular release of cancer therapeutics. Biomacromolecules. 2006;7:1568–1572.
- Datir SR, Das M, Singh RP, et al. Hyaluronate tethered, “smart” multiwalled carbon nanotubes for tumor-targeted delivery of doxorubicin. Bioconjug Chem. 2012;23:2201–2213.
- Carson L, Kelly-Brown C, Stewart M, et al. Synthesis and characterization of chitosan-carbon nanotube composites. Mater Lett. 2009;63:617–620.
- López-Cruz A, Barrera C, Calero-DdelC VL, et al. Water dispersible iron oxide nanoparticles coated with covalently linked chitosan. J Mater Chem. 2009;19:6870–6876.
- Ge Y, Zhang Y, He S, et al. Fluorescence modified chitosan-coated magnetic nanoparticles for high-efficient cellular imaging. Nanoscale Res Lett. 2009;4:287.
- Gui R, Jin H. Temperature-regulated polymerization and swelling/collapsing/flocculation properties of hybrid nanospheres with magnetic cores and thermo/pH-sensitive nanogel shells. RSC Adv. 2014;4:2797–2806.
- Liu H, Pierre-Pierre N, Huo Q. Dynamic light scattering for gold nanorod size characterization and study of nanorod–protein interactions. Gold Bull. 2012;45:187–195.
- Lu YJ, Wei KC, Ma CCM, et al. Dual targeted delivery of doxorubicin to cancer cells using folate-conjugated magnetic multi-walled carbon nanotubes. Colloids Surf B Biointerfaces. 2012;89:1–9.
- Ali-Boucetta H, Al-Jamal KT, McCarthy D, et al. Multiwalled carbon nanotube–doxorubicin supramolecular complexes for cancer therapeutics. Chem Commun. 2008; 28 (4): 459–461.
- Raman C, Berkland C, Kim K, et al. Modeling small-molecule release from PLG microspheres: effects of polymer degradation and nonuniform drug distribution. J Control Release. 2005;103:149–158.
- Tacar O, Sriamornsak P, Dass CR. Doxorubicin: an update on anticancer molecular action, toxicity and novel drug delivery systems. J Pharm Pharmacol. 2013;65:157–170.