Abstract
Retinal endothelial cells (RECs) are involved in many ocular diseases such as age-related macular degeneration (AMD) and diabetic retinopathy. Salicin is the major ingredient of willow bark extract, and it has been shown to be a potent anti-inflammatory agent. We aim to explore whether salicin has a vascular protective effect in RECs. Our data indicate that the presence of salicin in RECs culture media ameliorates interleukin-1β (IL-1β)-induced cellular reactive oxygen species (ROS) production and NADPH oxidase 4 (NOX-4) expression. At the cellular level, salicin attenuates IL-1β-induced mitochondrial injury as revealed by its preservation on mitochondrial membrane potential (MMP). Furthermore, salicin inhibits IL-1β-induced production of pro-inflammatory cytokines such as tumor necrosis factor-α (TNF-α), interleukin-6 (IL-6), and monocyte chemoattractant protein-1 (MCP-1), vascular adhesion molecules such as intercellular cell adhesion molecule-1 (iCAM-1) and vascular cell adhesion molecule 1 (VCAM-1), and high-mobility group protein 1 (HMGB-1). On the other hand, salicin recovers IL-1β-induced reduction of endothelial nitric oxide synthase (eNOS) and nitric oxide (NO) release. The presence of salicin significantly reduces the IL-1β-induced release of lactate dehydrogenase (LDH), indicating that it mitigates cytokine caused cytotoxicity. Mechanistically, we show that salicin suppresses IL-1β-induced activation of the nuclear factor-kappa B (NF-κB) signaling as revealed by its suppression on nuclear p65 protein and transfected NF-κB promoter. Collectively, our study demonstrates by multiple facets of its mechanisms that salicin is a protective agent in retinal endothelial cells. These results imply its potential use in therapeutic usage of retinal disease.
Introduction
Retinal endothelial cells (RECs) are one of the major cell types which are involved in many ocular diseases. Retinal microvasculature contributes to the blood-retinal barrier which is essential to normal visual function [Citation1]. Alterations of the retinal endothelium play a crucial role in the development of retinal diseases. Among the retinal diseases, age-related macular degeneration (AMD) and diabetic retinopathy are two major eye disorders that affect millions of people worldwide [Citation2]. AMD is viewed as the most common cause of blindness in older adults in western countries. Diabetic retinopathy is one of the most prevalent microvascular diseases impairing the vision of patients. Treatment of these retinal diseases remains a challenge as there are currently no effective treatments approved for AMD and diabetic retinopathy [Citation3,Citation4]. AMD features the death of choroidal endothelial cells, retinal pigment epithelium (RPE), and photoreceptor cells, and the fate of these cell types are interdependent, the loss of one type often results in the death of others [Citation5,Citation6]. A decline in RECs and altered vascular permeability are the early signs of these retinal diseases. Diabetic retinopathy can result from the damage diabetes causes to the microvasculature in the retina, and this vascular damage can cause vision loss. Increased levels of pro-inflammatory cytokines, including TNF-α, IL-1β, and chemokines, have been reported in patients with diabetic retinopathy. The inflammatory retinal environment could increase local glucose consumption and ROS production which eventually cause the apoptosis of RECs [Citation7]. Recent studies have explored the inhibition of inflammation in diabetic retinopathy as a potential treatment target.
Willow bark is a commonly used traditional medicine. Known for its alleviating effect on fever, pain, and inflammation, willow bark has been referred to as “nature’s Aspirin.” In preclinical and clinical studies, willow bark has been reported to be effective in pain management and anti-inflammation [Citation8,Citation9]. Salicin is the major component of willow bark extract. Salicin is metabolized to salicylic acid in vivo and then plays a major role in anti-inflammatory and anti-analgesic effects. For example, it has been recently reported that salicin displays an anti-inflammatory effect against lipopolysaccharides (LPS) in RAW264.7 cells in mouse models by suppressing the expression of pro-inflammatory cytokines such as TNF-α, IL-1β, IL-6, and IL-10 and the activation of NF-κB [Citation10]. Another study reported that administration of salicin could ameliorate inflammatory response, suppress edema and mucosal damage in a dextran sulfate sodium (DSS)-induced colitis in mouse models [Citation11]. We have been motivated by willow bark’s healing effects and previous studies to investigate whether salicin plays a beneficial role in retinal vascular cells. In this study, we applied salicin to cultured retinal endothelial cells and revealed its protective role and the molecular mechanism involved.
Materials and methods
Cell culture, treatment, and adhesion assay
Primary human retina endothelial cells (RECS, ACBRI 181) were purchased from Cell Systems (Kirkland, WA). HAECs were purchased from Lonza (Basel, Switzerland). Salicin was provided by Sigma-Aldrich and dissolved in dimethyl sulfoxide (DMSO). RECS were grown in 2% low serum growth media (EGM2) in low passage numbers (less than 10). All the cells were maintained at 5% CO2 in an incubator at 37 °C. For the IL-1β and salicin experiment, 10 ng/mL freshly prepared IL-1β containing media was added to confluent RECs cell media in the presence or absence of salicin (50 and 100 μM) for 24–72 h.
Quantitative real-time PCR analysis
The total RNA from RECs were extracted according to the manufacturer’s instruction with the micro RNeasy Micro Kit from Qiagen (Hilden, Germany). The RNA concentrations were quantified by a Nanodrop spectrophotometer from Cole-Parmer (Chicago, IL). A total of 1 μg of RNA was used to synthesize the cDNA by iScript™ Reverse Transcription Supermix for RT-qPCR from Invitrogen (Carlsbad, CA). A SYBR based real-time PCR experiment was performed to detect the total transcripts of mRNA of NOX4, TNF-α, IL-6, and MCP-1, HMGB-1, ICAM-1 VCAM-1, and eNOS by the ABI 7500 platform. The relative expression levels of these genes were normalized to the glyceraldehyde-3-phosphate dehydrogenase (GAPDH) level by 2–ΔΔCT method [Citation12]. The following primers were used in this study: GAPDH (For: 5′-GGAGCGAGATCCCTCCAAAAT-3′, Rev: 5′-GGCTGTTGTCATACTTCTCATGG-3′); TNF-α (For: 5′-GTCACTCATTGCTGAGCCTCT-3′; Rev: 5′-AGCTTCTTCCCACCCACAAG-3′);
IL-6 (For: 5′-GGTACATCCTCGACGGCATCT-3′, Rev: 5′-GTGCCTCTTTGCTGCTTTCAC-3′); MCP-1 (For: 5′-ATGCAATCAATGCCCCAGTC-3′, Rev: 5′-TGCAGATTCTTGGGTTGTGG-3′); VCAM-1 (For: 5′-TGT TTG CAG CTT CTC AAG CTT TT-3′, Rev: 5′-GAT GTG GTC CCC TCA TT CGT-3′); ICAM-1 (For: 5′-GGC CTC AGT CAG TGT GA-3′; Rev: 5′-AAC CCC ATT CAG CGT CA-3′); eNOS (For: 5′-GTGATGGCGAAGCGAGTGAAG-3′; Rev, 5′-CCGAGCCCGAACACACAGAAG-3′); NOX-4 (For, 5′-AATGCACCAACAAATGGGGC-3′; Rev, 5′-ACCTATTCGACACTGGAGATG-3′).
Western blot analysis
RECs at varying conditions were lysed by radioimmunoprecipitation assay (RIPA) buffer with protease inhibitors. A total of 20 μg cell lysates were loaded to 4 to 20% precast polyacrylamide gel electrophoresis (PAGE) gel to separate the proteins according to their size. The separated protein mix was transferred to polyvinylidene fluoride (PVDF) membranes to detect the corresponding protein levels by specific antibodies. Blots were developed using enhanced chemiluminescence (ECL) [Citation13]. The following antibodies were used in this study: NOX-4 (1:2000, #ab133303, Abcam); eNOS (1:1000, #ab76198, Abcam); p65 (1:2000, #9460, Cell Signaling Technology); lamin B1 (1:5000, #13435, Cell Signaling Technology); β-actin (1:10,000, #3700, Cell Signaling Technology); anti-rabbit IgG, HRP-linked Antibody (1:2000, #7074, Cell Signaling Technology); anti-mouse IgG, HRP-linked Antibody (1:2000, #7072, Cell Signaling Technology).
Enzyme-linked immunosorbent assay (ELISA)
To measure the secreted levels of pro-inflammatory cytokines, RECs culture media were collected for the analyses by ELISA with the following commercial ELISA kits purchased from R&D systems: TNF-α (#DTA00D), IL-6 (#D6050), and MCP-1 (#DCP00). To measure the expression of cell adhesion molecules, RECs were lysed and used for ELISA analysis with the following ELISA kits from R&D systems: ICAM-1 (DCD540) and VCAM-1 (DY809). The experiments were performed following the manufacturer’s instructions. The data were collected by a 96-well plate reader spectrometry. The absolute values were obtained from a standardized 4-PL curve. The relative levels of TNF-α, IL-6, and MCP-1 were presented by normalized to total protein amounts in each condition.
Determination of intracellular ROS
Cellular reactive oxygen (ROS) production was measured by quick staining the cells with the probe dihydroethidium (DHE). Cells were treated with IL-1β (10 ng/mL) in the presence or absence of salicin (50 and 100 μM) for 48 h. Cells were then probed with DHE (5 μmol/L) for 30 min at 37 °C in darkness. After three washes, fluorescent images were captured by a fluorescent microscope. The fluorescent density of images was quantified by Image J software.
Intracellular nitric oxide (NO) measurement
We measured intracellular NO level based on the method of cell-permeable fluorescent probe 4-amino-5-methylamino-2’,7’-difluorofluorescein diacetate (DAF-FM DA). The dye was purchased from Sigma-Aldrich (254109-22-3, St. Louis, MO). Briefly, 5 μM of DAF-FM DA was added to the RECs cell culture media and permeated into living cells for 30 min. Upon entry into the cells, DAF-FM DA was deacetylated and reacted with intracellular NO to form green-fluorescence. The fluorescence intensity of the DAF-FM-NO complex was quantified as the percentage change from the initial value.
Mitochondrial membrane potential assay
Mitochondrial membrane potential (MMP) assay was based on the measurement of tetramethylrhodamine methyl and ethyl esters (TMRM) staining [Citation14]. Based on the concentration of TMRM accumulation in the mitochondria, the aggregated fluorescent counts were measured to determine the depolarization of mitochondria and cell health status. We purchased the TMRM kit (Thermo Fisher Scientific) and MMP activity was measured with fluorescent density and quantified to present the data.
NF-κB promoter assay
The 3× NF-κB binding sites containing the luciferase vector were purchased from Thermo Fisher Scientific. Cells were co-transfected with the NF-κB promoter and a firefly luciferase promoter by Lipofectamine 2000 reagent from Invitrogen (Carlsbad, CA). At 24 h post-transfection, the cells were then switched to 10 ng/mL IL-1β media in the presence or absence of salicin at the concentrations of 5 and 10 μM for an additional 48 h. The total cell lysates were collected to measure the dual luciferase activity of the renilla and firefly luciferases. The relative luciferase was calculated by normalizing the activity of firefly luciferase to the renilla luciferase activity.
Statistical analysis
Experimental data are shown as mean ± standard derivation (SD). Statistical analysis between different comparisons was performed by a one-way analysis of variance (ANOVA) test, followed by the Bonferroni post-hoc test. p values of less than .05 were determined statistically significant.
Results
Salicin ameliorates IL-1β-induced oxidative stress in RECs
To examine the effect of salicin in retinal endothelial cells, we treated RECs with 10 ng/mL of IL-1β which caused the cellular release of pro-inflammatory cytokine and reactive oxidative species (ROS). We then examined the cellular ROS status in the presence or absence of salicin by directly staining the ROS signals and determining the NADPH oxidase key subunit NOX-4. As shown in , the cellular permeable DHE levels from the treatment groups were compared to the non-treated RECs. There was an increase of about threefold when RECs were treated with IL-1β, but this increase was significantly attenuated when two doses of salicin (50 and 100 uM) were added to the cells, with the higher dose being more potent than the lower (). NADPH oxidase is one of the major sources of the generation of cellular ROS, so we then assessed the expression of its catalytic subunit NOX-4. At mRNA level, IL-1β treatment resulted in a roughly fourfold induction of NOX-4, but this induction was deterred when two doses of salicin were presented at the same time, with the higher dose of the two being more effective (). At the protein level, we got a very similar result and thus confirmed the inhibitory effect of salicin on IL-1β mediated induction of NOX-4 at the protein level ().
Figure 1. Salicin ameliorates IL-1β-induced oxidative stress in human primary retinal endothelial cells (RECs). (A) The cellular reactive oxygen species (ROS) was stained by dihydroethidium (DHE); Scale bars, 100 μm. (B) The expression of NOX-4 at the mRNA levels was determined by real-time PCR. (C) The expression of NOX-4 at the protein levels was determined by western blot analysis. Cells were treated with IL-1β (10 ng/mL) in the presence or absence of salicin (50 and 100 μM) for 48 h (*, #, $, p < .01 vs. previous column group, n = 5–6).
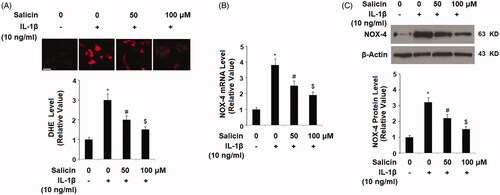
Salicin mitigates IL-1β-induced mitochondrial dysfunction
Next, we examined the influence of salicin on retinal endothelial mitochondrial function by determining the mitochondrial membrane potential (MMP) with TRRM staining. When compared to the nontreated ECs, IL-1β reduced more than 70% of the MMP level, but the presence of two doses of salicin gradually recovered the reduced MMP, with the higher dose proving more effective (). The ameliorative effect of salicin on MMP indicates that it has a protective role in endothelial mitochondrial function.
Figure 2. Salicin mitigates IL-1β-induced mitochondrial dysfunction in RECs. Cells were treated with IL-1β (10 ng/mL) in the presence or absence of salicin (50 and 100 μM) for 48 h. The levels of mitochondrial membrane potential (MMP) were determined by tetramethyl rhodamine methyl ester (TMRM) staining. Scale bars, 100 μm (*, #, $, p < .01 vs. previous column group, n = 6).
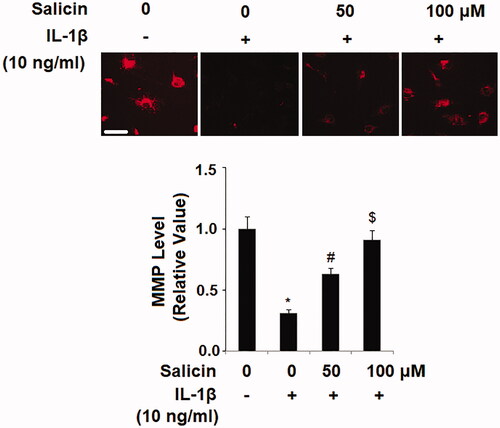
Salicin suppresses IL-1β-induced production of pro-inflammatory cytokines
Since IL-1β induces various pro-inflammatory factors, including cytokines, we measured the levels of these cytokines in the presence of salicin. We performed quantitative PCR and ELISA experimentation to determine both mRNA transcription and their secretion in the culture media respectively, and the expression change of three major cytokines including TNF-α, IL-6, and MCP-1. At the mRNA level and compared to non-treated cells, IL-1β increased the mRNA transcription of these cytokines by roughly 3- to 4-fold, but two doses of salicin showed significant suppression on their effects, with the higher dose being more effective (). At the protein level, salicin displayed a similar suppressive role against IL-1β-induced induction of these cytokines (). Thus, we conclude that salicin has an inhibitory role on IL-1β triggered pro-inflammatory cytokine release.
Figure 3. Salicin inhibits IL-1β-induced secretions of pro-inflammatory cytokines in RECs. (A) Expressions of TNF-α, IL-6, and MCP-1 at the mRNA levels were determined by real-time PCR analysis. (B) Secretions of TNF-α, IL-6, and MCP-1 were determined by ELISA respectively. Cells were treated with IL-1β (10 ng/mL) in the absence or presence of salicin (50 and 100 μM) for 48 h (*, #, $, p < .01 vs. previous column group, n = 5–6).
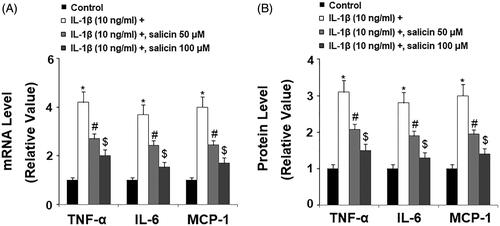
Salicin reduces IL-1β-induced production of high-mobility group protein 1 (HMGB-1)
High mobility group box 1 protein (HMGB-1) is a conserved stress-responsive nuclear protein that is released into the extracellular space by endothelial cells. We measured the releases of endothelial-derived HMGB-1 by ELISA. Compared to control cells, IL-1β induced more than threefold HMGB-1 release. However, the presence of two doses of salicin significantly attenuated this induction, with the higher dose being more effective than the lower (). Thus, the HMGB-1 experiment indicated that salicin suppresses IL-1β-induced endothelial stress.
Figure 4. Salicin reduces IL-1β-induced secretions of high-mobility group protein 1 (HMGB-1) in RECs. Cells were treated with IL-1β (10 ng/mL) in the absence or presence of salicin (50 and 100 μM) for 48 h. Secretions of HMGB-1 were determined by ELISA (*, #, $, p < .01 vs. previous column group, n = 6).
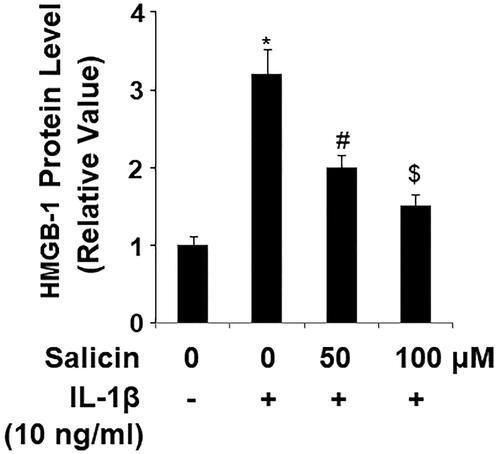
Salicin inhibits IL-1β-induced release of lactate dehydrogenase (LDH)
The consequences of cellular stress by IL-1β often cause endothelial cytotoxicity which can threaten the survival of cells. We hypothesized that salicin would possess certain protective properties against cellular stress. Indeed, in our experiment assessing LDH release, IL-1β caused a roughly eightfold release of LDH, but the presence of the two doses of salicin greatly reduced the increase in LDH, with the 100 μM dose nearly reducing the released LDH level to the control level ().
Figure 5. Salicin inhibits IL-1β-induced release of lactate dehydrogenase (LDH) in RECs. Cells were treated with IL-1β (10 ng/mL) in the absence or presence of salicin (50 and 100 μM) for 48 h. The release of LDH was determined using a commercial kit (*, #, $, p < .01 vs. previous column group, n = 6).
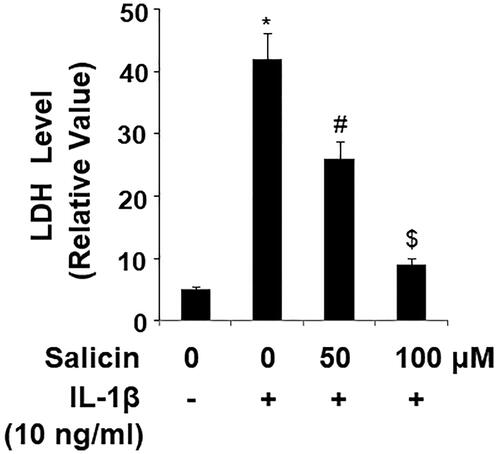
Salicin inhibits IL-1β-induced expression of vascular adhesion molecules
Cytokine-induced endothelial dysfunction leads to the production of vascular adhesion molecules which attract immune cells. We thus measured major vascular adhesion molecules including ICAM-1 and VCAM-1. At the mRNA level, RECs treated with IL-1β express nearly six to sevenfold increase in ICAM-1 and VCAM-1, respectively. But the presence of the two doses of salicin dramatically reverted this increase, with the higher dose being more effective (). At the protein level, the nontreated cells produced a very low level of these proteins, while IL-1β induced a large amount of ICAM-1 and VCAM-1 expression. The addition of the two doses of salicin greatly reduced their levels, with the high dose being more potent ().
Figure 6. Salicin inhibits IL-1β-induced expression of ICAM-1 and VCAM-1 in RECs. (A) Expressions of ICAM-1 and VCAM-1 at the mRNA levels were determined by real-time PCR. (B) Expressions of ICAM-1 and VCAM-1 at the protein levels were determined by ELISA. Cells were treated with IL-1β (10 ng/mL) in the presence or absence of salicin (50 and 100 μM) for 48 h (*, #, $, p < .01 vs. previous column group, n = 5–6).
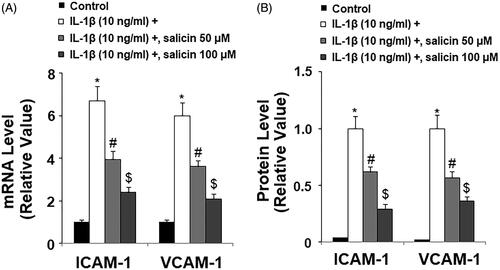
Salicin inhibits IL-1β-induced reduction of endothelial nitric oxide synthase (eNOS) and nitric oxide (NO)
Next, we aimed to investigate whether salicin had an influence on retinal endothelial function. We assessed NO production, eNOS protein, and the intracellular NO level. At the mRNA level and compared to the control, IL-1β treatment reduced more than half of the eNOS transcript, but the two doses of salicin gradually recovered normal eNOS expression, with the high dose being more effective (). Using western blot analysis, we recapitulated this effect and confirmed its protection of the eNOS level (). Measurements of the level of intracellular NO showed that, compared to the control, IL-1β treatment reduced cellular NO by more than half. However, the presence of the two doses of salicin gradually recovered NO levels to normal, with higher dose being more effective (). Thus, we concluded that salicin protects endothelial function by ameliorating stress-caused reduction of eNOS expression and NO level.
Figure 7. Salicin inhibits IL-1β-induced reduction of endothelial nitric oxide synthase (eNOS) and nitric oxide (NO). (A) The expression of eNOS at the mRNA levels was determined by real-time PCR. (B) The expression of eNOS at the protein levels was determined by western blot analysis. (C) The level of intracellular NO was determined by DAF-FM DA staining. Scale bars, 100 μm. Cells were treated with IL-1β (10 ng/mL) in the presence or absence of salicin (50 and 100 μM) for 48 h (*, #, $, p < .01 vs. previous column group, n = 5–6).
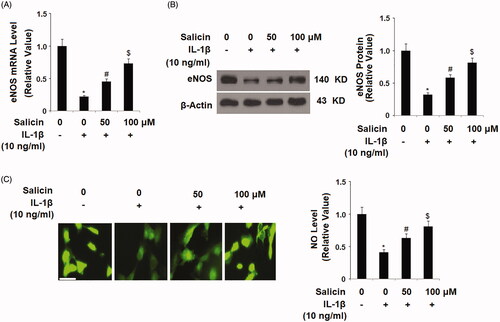
Salicin suppresses cellular NF-κB activity
Finally, we explored the molecular pathway through which salicin operates. Based on its antagonism of IL-1β-induced induction of ROS and pro-inflammatory factors, we hypothesized that salicin might interact through the NF-κB pathway. We then tested the effect of salicin on nuclear NF-κB and its promoters. By blotting the retention of the nuclear NF-κB subunit p65, we compared the levels of nuclear NF-κB under the different treatment conditions. Compared to the non-treated control, IL-1β treatment resulted in a roughly threefold increase in p65 accumulation in the nuclei. But the addition of two doses of salicin gradually mitigated this increase, with the high dose being more effective (). When the NF-κB luciferase vector was transfected into the cells, IL-1β caused a nearly 49-fold increase in promoter activity. But this induction was lessened with two doses of salicin added into the culture (). We concluded that salicin indeed inhibited cytokine-caused endothelial NF-κB activity.
Figure 8. Salicin inhibits IL-1β-induced activation of the NF-κB signaling. (A) Nuclear levels of p65 were determined by western blot analysis. (B) NF-κB luciferase activities were measured. Cells were treated with IL-1β (10 ng/mL) in the absence or presence of salicin (50 and 100 μM) for 48 h (*, #, $, p < .01 vs. previous column group, n = 5–6).
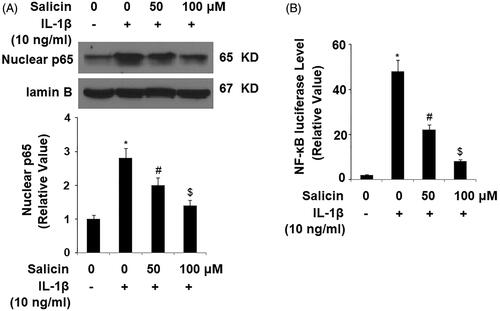
Discussion
Retinal diseases affect a large number of people and have a significant influence on the quality of a patient’s life, and the treatment of retinal disease is an area of active research. The cost of developing new agents for retinal diseases is high, and we have been searching for an alternative treatment by sifting active constituents of traditional medicine. The pain relieving capabilities of willow bark extract have been recognized throughout history, and it has been widely used to relieve joint pain, muscle pain, headache, and other inflammatory complications. Recently, studies have revealed that the major active ingredient in willow bark, salicin, shows certain curative abilities in vascular endothelial cells [Citation15–17]. Two studies separately demonstrated that salicin or willow bark extract reduces reactive oxygen species production in cultured endothelial cells by increasing antioxidant enzymes [Citation18,Citation19]. Salicin even inhibited the angiogenic activity of endothelial cells, and furthermore inhibited tumor growth and angiogenesis in a mouse tumor model [Citation19]. Inspired by these studies and the facts about salicin’s curative properties, we initiated our study to investigate how salicin negates harmful effects from local inflammation in cultured RECs.
Our data suggest that salicin is an effective agent which exhibits potent vascular protection in retinal endothelial cells. Our data support that salicin is a pro-survival, anti-inflammatory, anti-ROS agent that protects RECs from IL-1β-induced injury. In the present study, we show that the presence of salicin mitigates much of the cytokine IL-1β-caused endothelial toxicity as revealed by LDH release assay. Salicin protects from IL-1β-induced endothelial NO reduction and eNOS decrease, suggesting that salicin acts to protect endothelial cells from the cytokine IL-1β. Our data show that salicin protects against IL-1β-induced mitochondrial dysfunction of RECs as revealed by the ability of salicin to ameliorate the collapse of MMP and HMGB-1 release. Disruption in mitochondrial functioning is an early signature of apoptosis. These protective effects suggest that mitochondrial aid is one of the mechanisms by which salicin protects RECs from apoptosis caused by cellular stress. HMGB-1 is a stress-responsive factor released from the nucleus, which can lead to endothelial mitochondrial dysfunction [Citation20]. All this evidence implies that salicin acts as a pro-survival vector in RECs. Our findings demonstrate that salicin significantly reduced IL-1β-caused production of vascular cytokines (TNF-α, IL-6, and MCP-1) and adhesion molecules (ICAM-1 and VCAM-1). The wide range of suppression capabilities on these key pro-inflammatory factors suggests that salicin possesses anti-inflammatory properties in RECs. We furthermore demonstrated that salicin suppresses IL-1β-induced total cellular ROS level and expression of NOX-4. NOX-4 is the dominant catalytic unit of NAPDH oxidase in endothelial cells, which attributes to a great extent to ROS generation in endothelial cells [Citation21]. The effect of the suppression of ROS production is in accordance with the fact that it can protect the mitochondrial function of the retinal endothelial cells. Our data indicate that salicin possesses robust inhibition capabilities on IL-1β-induced NF-ƙB activation as revealed by its suppression on nuclear p65 accumulation and NF-ƙB promoter activity, which highlights the mechanism of the molecular pathway it regulates.
Taken together, our study reveals the insights of the vascular protection of salicin in RECs. The exact manner in which salicin promotes endothelial protection remains to be answered. We are still exploring whether or not any cell surface receptor or other cellular mediators other than NF-ƙe is involved in the abilities of salicin. RECs are highly differentiated and energy demanding endothelial cells, which convert light to chemical signals to sense light, and the normal function of retinal EC requires high production of ATP. The excellent regulation of its integrity against harmful local stimuli is critical to preserving the visual function in the process of aging or in diabetic conditions. Our data support the fact that salicin possesses unique retinal vascular protection, and its application could be a potential candidate to prevent these retinal diseases.
Disclosure statement
No potential conflict of interest was reported by the authors.
References
- Cunha-Vaz J, Bernardes R, Lobo C. Blood-retinal barrier. Eur J Ophthalmol. 2011;21:S3–S9.
- Philips BE, Antonetti DA. Blood-retinal barrier. In: Joussen AM, Gardner TW, Kirchhof B, et al. editors. Retinal vascular disease. Berlin (Germany): Springer-Verlag; 2007. p. 139–153.
- Mitchell P, Liew G, Gopinath B, et al. Age-related macular degeneration. Lancet 2018;392:1147–1159.
- Relhan N, Flynn HW, Jr. The early treatment diabetic retinopathy study historical review and relevance to today's management of diabetic macular edema. Curr Opin Ophthalmol. 2017;28(3):205–212.
- Whitmore SS, Braun TA, Skeie JM, et al. Altered gene expression in dry age-related macular degeneration suggests early loss of choroidal endothelial cells. Mol Vis. 2013;19:2274–2297.
- Miller JW, Bagheri S, Vavvas DG. Advances in age-related macular degeneration understanding and therapy. US Ophthalmic Rev. 2017;10:119–130.
- Rübsam A, Parikh S, Fort PE. Role of inflammation in diabetic retinopathy. Int J Mol Sci. 2018;19:E942.
- Vlachojannis JE, Cameron M, Chrubasik S. A systematic review on the effectiveness of willow bark for musculoskeletal pain. Phytother Res. 2009;23:897–900.
- Fiebich BL, Appel K. Anti-inflammatory effects of willow bark extract. Clin Pharmacol Ther. 2003;74:96–97.
- Li Y, Wu Q, Deng Y, et al. D(-)-Salicin inhibits the LPS-induced inflammation in RAW264.7 cells and mouse models. Int Immunopharmacol. 2015;26:286–294.
- Verma N, Verma R, Kumari R, et al. Effect of salicin on gut inflammation and on selected groups of gut microbiota in dextran sodium sulfate-induced mouse model of colitis. Inflamm Res. 2014;63:161–169.
- Hormozi M, Ghoreishi S, Baharvand P. Astaxanthin induces apoptosis and increases activity of antioxidant enzymes in LS-180 cells. Artif Cells Nanomed Biotechnol. 2019;47:891–895.
- Zhang J, Liu M, Liu W, et al. Ras-ERK1/2 signalling promotes the development of osteosarcoma through regulation of H4K12ac through HAT1. Artif Cells Nanomed Biotechnol. 2019;47:1207–1215.
- Lin Z, Guichun Z, Lifeng L, et al. Protective effect of α-lipoic acid against antimycin A cytotoxicity in MC3T3-E1 osteoblastic cells. Cell Stress Chaperones 2017;22:5–13.
- Kaufeld AM, Pertz HH, Kolodziej H. A chemically defined 2,3-trans procyanidin fraction from willow bark causes redox-sensitive endothelium-dependent relaxation in porcine coronary arteries. J Nat Prod. 2014;77:1607–1614.
- Freischmidt A, Jürgenliemk G, Kraus B, et al. Contribution of flavonoids and catechol to the reduction of ICAM-1 expression in endothelial cells by a standardised Willow bark extract. Phytomedicine 2012;19:245–252.
- Knuth S, Schübel H, Hellemann M, et al. Catechol, a bioactive degradation product of salicortin, reduces TNF-α induced ICAM-1 expression in human endothelial cells. Planta Med. 2011;77:1024–1026.
- Kong CS, Kim KH, Choi JS, et al. Salicin, an extract from white willow bark, inhibits angiogenesis by blocking the ROS-ERK pathways. Phytother Res. 2014;28:1246–1251.
- Ishikado A, Sono Y, Matsumoto M, et al. Willow bark extract increases antioxidant enzymes and reduces oxidative stress through activation of Nrf2 in vascular endothelial cells and Caenorhabditis elegans. Free Radic Biol Med. 2013;65:1506–1515.
- Ago T, Kitazono T, Ooboshi H, et al. Nox4 as the major catalytic component of an endothelial NAD(P)H oxidase. Circulation 2004;109:227–233.
- Tang D, Kang R, Van Houten B, et al. High mobility group box 1 (HMGB-1) phenotypic role reveal,.ed with stress. Mol Med. 2014;20:359–362.