Abstract
Salidroside (Sal) exerted widely pharmacological effects in multitudinous diseases had been certified. The actual study clarified the protective activity of Sal in H2O2-injured human trabecular meshwork (HTM) cells. HTM cells were disposed with H2O2 to construct an oxidative damage model in vitro. Then, Sal was utilized to administrate HTM cells, and cell viability, apoptosis, apoptosis-interrelated proteins and ROS production were appraised using CCK-8, flow cytometry, western blot and DCFH-DA staining. MiR-27a inhibitor and its control were transfected into HTM cells, and the influences of miR-27a inhibition in HTM cells stimulated with H2O2 and Sal were detected. PI3K/AKT and Wnt/β-catenin pathways were ultimately investigated to uncover the underlying mechanism. We found that H2O2 evoked HTM cells oxidative damage, as evidenced by repressing cell viability, inducing apoptosis, activating cleaved-caspase-3/-9 expression and increasing ROS production. Sal significantly lightened H2O2-evoked oxidative damage in HTM cells. Additionally, miR-27a was up-regulated by Sal, and miR-27a suppression significantly reversed the protective effect of Sal on H2O2-injured HTM cells. Finally, Sal activated PI3K/AKT and Wnt/β-catenin pathways through enhancement of miR-27a in H2O2-injured HTM cells. In conclusion, these discoveries suggested that Sal could protect HTM cells against H2O2-evoked oxidative damage by activating PI3K/AKT and Wnt/β-catenin pathways through enhancement of miR-27a.
H2O2 evokes HTM cells oxidative damage;
Sal relieves H2O2-induced oxidative damage in HTM cells;
Sal enhances miR-27a expression in H2O2-injured HTM cells;
Repressed miR-27a reverses the protective impacts of Sal on H2O2-injured HTM cells;
Sal activates PI3K/AKT and Wnt/β-catenin pathways by increasing miR-27a.
Highlights
Introduction
Glaucoma is a complex neurodegenerative disease, which can result in the optic nerve damage and even blindness [Citation1]. Glaucoma is predominantly asymptomatic in the beginning but could have symptoms such as eye pain, photophobia, redness of the eye, blurred vision and sometimes maybe appear nausea [Citation2,Citation3]. The increased intraocular pressure and lack of blood supply of the optic nerve are the primary risk factors of glaucoma [Citation4,Citation5]. Evidence have proven that high intraocular pressure can cause the death of optic nerve and retinal ganglion cells, thereby impairment of visual function [Citation6,Citation7]. Currently, the goals of glaucoma remedy are to avoid glaucomatous damage and nerve damage, and the key therapeutic strategies of glaucoma include medicines, laser and conventional surgery [Citation8,Citation9]. Although these methods may save remaining eyesight, they cannot improve the visual loss caused by glaucoma. Increasing researches demonstrated that trabecular meshwork (TM) cells play a vital role in aqueous humour circulation, and TM cell injury is closely associated with the increased intraocular pressure [Citation10,Citation11]. Therefore, it is of great importance to search for neoteric drugs to relieve TM cell injury in glaucoma patients.
Salidroside (Sal, molecular formula: C14H20O7, molecular weight: 300.3044) is a familiar traditional Chinese medicine (TCM), which is extracted from the dried roots, rhizomes and whole grass of Rhodiola rosea L. [Citation12]. Studies have shown that Sal possesses widely pharmacological activities, such as anti-tumour, anti-fatigue, anti-ageing, anti-hypoxia, enhancement of immune function, bidirectional regulation of the central nervous system, as well as repair and protection of the body [Citation13–15]. Interestingly, it has been reported that Sal exerted protective impact against hydrogen peroxide (H2O2)-induced cell apoptosis. Evidence from Chen et al. proven that Sal could protect primary cultured rat hippocampal neurons against H2O2-evoked cell apoptosis [Citation16]. Wang et al. stated that Sal could prohibit H2O2-induced apoptosis in PC12 cells through preventing cytochrome c release and inactivating of caspase cascade [Citation17]. Nonetheless, whether Sal could protect human trabecular meshwork (HTM) cells against H2O2-induced damage remain uninvestigated.
MicroRNA-27a (miR-27a) has been reported to play a crucial role in regulating cancer cell growth, colony formation and migration, which also functions as a tumour suppressor in acute leukaemia [Citation18,Citation19]. One study from Cai et al. testified the protective mechanisms of miR-27a against oxygen-glucose deprivation-induced injury in hippocampal neurons [Citation20]. Additionally, an interesting study disclosed that miR-27a was down-regulated in mouse macrophage cells (RAW264.7) under H2O2 administration, moreover, miR-27b has confirmed to be an oxidative stress-responsive miRNA modulates nuclear factor-κB (NF-κB) pathway in RAW264.7 cells [Citation21]. However, whether miR-27a serves as a relevant miRNA in oxidative stress in HTM cells is still unclear. Hence, in our study, we explored the above issues.
In the actual study, we constructed a H2O2-induce oxidative damage model in HTM cells and probed the functions of Sal in H2O2-injured HTM cells. Moreover, miR-27a inhibitor and its control were transfected into HTM cells to study the mediatory effect of miR-27a on H2O2-injured HTM cells. Besides, PI3K/AKT and Wnt/β-catenin pathways were investigated to uncover the underlying mechanism. These explorations might provide more evidence for supporting the glaucoma clinical research and might present a neoteric idea for remedying glaucoma.
Materials and methods
Cell culture and treatment
HTM cells were obtained from cadaver eyes agreed with the local ethics committee. The detached HTM cells were cultured in non-essential amino acid high glucose Dulbecco’s modified Eagle’s medium (DMEM) comprising 10% fetal bovine serum (FBS), 100 units/mL penicillin, 100 μg/mL streptomycin sulfate, 2 mM L-glutamine, 110 mg/mL sodium pyruvate. (All these reagents were obtained from Invitrogen, Carlsbad, CA, USA.) Cells were maintained in the incubator at 37 °C encompassing 95% air and 5% CO2. These cells were administrated with disparate concentrations of H2O2 (0–400 μM) to construct an oxidative cell damage model in vitro. Non-disposed cells served as a blank (normal) control. Sal was purchased from Sigma-Aldrich (SMB00072, St. Louis, MO, USA) and was diluted in phosphate buffered saline (PBS, Sigma-Aldrich). Then, cells were disposed with diverse concentrations of Sal (0–5 μM) for 12 h before administration with H2O2.
Cell counting kit-8 (CCK-8) assay
After stimulation, HTM cells were seeded in 96-well plate with 5.0 × 103 cells/well, CCK-8 kit (Dojindo Molecular Technologies, Gaithersburg, MD) was employed to detect the ability of HTM cells. In brief, 10 μL CCK-8 solution was replenished into the culture medium after disposition with H2O2 or Sal, and the culture plate was placed in the incubator for 1 h at 37 °C in humidified 95% air and 5% CO2. The absorbance was measured at 450 nm using a Microplate Reader (Bio-Rad, Hercules, CA, USA).
Apoptosis assay
Annexin V-fluorescein isothiocynate (FITC)/propidium iodide (PI) Dead kit (Invitrogen) was utilized to analyze the percentage of apoptotic cells. After administration, cells were rinsed twice in pre-cold PBS. Then, cells were re-suspended with 1 × Binging Buffer (Sigma-Aldrich), and the concentration of cells was adjusted to 1 × 106 cells/mL. The 500 μL cell suspension was supplemented to the bottom of the flow tube, meanwhile added 5 μL Annexin V-FITC and 10 μL PI to stain cells for 15 min at room temperature in the dark. Afterwards, the stained cells were subjected into a FACS can (Beckman Coulter, Fullerton, CA, USA) for flow cytometry analysis. The data were analyzed using FlowJo software (Tree Star, San Carlos, CA, USA).
Reactive oxygen species (ROS) assay
ROS level was measured by flow cytometry using 2,7-dichlorofluorescein diacetate (DCFH-DA, Nanjing Jiancheng, Nanjing, China). Briefly, HTM cells were cultivated in a 6-well plate, after administration with H2O2 or Sal, which were rinsed twice with PBS and co-incubated in serum-free culture medium containing 10 μM DCFH-DA for 20 min at 37 °C in the dark. After incubation, these cells were rinsed with PBS and digested by 0.25% trypsin-EDTA for sample collection. After centrifugation for 1 min, cells were rinsed with PBS again, and 500 μL PBS was replenished to re-suspend cells. Finally, the fluorescent intensities were measured utilizing a flow cytometer (488 nm excitation, 521 nm emission).
Cell transfection
Expression vectors of miR-27a inhibitor and the relevant control (NC) were synthesized by GenePharma Co. (Shanghai, China). Above vectors were transfected into HTM cells by utilizing Lipofectamine 3000 reagent (Invitrogen) following the manufacturer’s direction.
Reverse transcription-quantitative PCR (RT-qPCR)
Total RNA was extracted from above-transfected cells by exploiting Trizol reagent (Life Technologies Corporation, Carlsbad, CA, USA) on the basis of the supplier’s specification. cDNA was synthesized by employing a PrimeScript™ 1st Strand cDNA Synthesis kit (TaKaRa, Dalian, China). RT-qPCR was executed to analyze miR-27a expression by utilizing Taqman Universal Master Mix II with the TaqMan MicroRNA Assay (Applied Biosystems, Foster City, CA, USA). U6 acted as the internal control. The data were detected using the 2−ΔΔCt method [Citation22].
Western blot assay
The protein sample from disposed cells was extracted exploiting radio immunoprecipitation assay (RIPA) lysis buffer (Beyotime, Shanghai, China). The proteins in supernatant were quantified by employing the BCA™ Protein Assay Kit (Pierce, Appleton, WI, USA). The protein samples were then electrophoresed using SDS-PAGE. After electrophoresis, proteins were transferred to polyvinylidene fluoride (PVDF) membranes, and these membranes were sealed in 5% non-fat milk for 1 h at indoor temperature. Subsequently, these membranes were co-cultivated with primary antibodies of pro-caspase-3 (ab32150), cleaved-caspase-3 (ab2302), pro-caspase-9 (ab32068), cleaved-caspase-9 (ab2324), phospho (p)-PI3K (ab182651), t-PI3K (ab133595), phospho (p)-AKT (ab81283), t-AKT (ab18785), β-catenin (ab16051), and β-actin (ab8227, all from Abcam, Cambridge, UK) at 4 °C overnight. After this, a horseradish peroxidase (HRP)-conjugated goat anti-rabbit IgG (ab205718, 1:2000, Abcam) was replenished, and co-incubated for 1 h at indoor temperature. The protein blots were visualized by exploiting the ECL Western blotting detection reagent (GE Healthcare, Braunschweig, Germany), and the intensity of the bands was quantified using ImageJ software (Bio-Rad).
Statistical analysis
All experiments were carried out three repeats. The data from multiple experiments are explained as the mean ± standard deviation (SD). Statistical analyses were executed by exploiting Graphpad statistical software (GraphPad, San Diego, CA, USA). The p values were reckoned utilizing a one-way analysis of variance (ANOVA). The statistically significant difference results were indicated as p < .05.
Results
H2O2 induced HTM cells oxidative damage
We initially constructed the H2O2-induce oxidative damage model in HTM cells. The diverse concentrations of H2O2 (0, 50, 100, 200, 300 and 400 μM) were employed to dispose HTM cells. Then, cell viability, apoptosis and ROS production were examined. In , we observed that the percentage of cell viability was repressed by H2O2 at the concentrations of 100 μM (p < .05), 200 μM (p < .01), 300 μM (p < .01) and 400 μM (p < .001). Due to the IC50 of viability was presented at the concentration of 200 μM, hence, we selected 200 μM H2O2 to stimulate HTM cells in the following experiments. Then, flow cytometry assay revealed that H2O2 evoked cell apoptosis in HTM cells as relative to control group (p < .001, ). Meanwhile, cleaved-caspase-3 and cleaved-caspase-9 protein levels were obviously enhanced by H2O2 in HTM cells (). Further, the results in showed that the ROS production was elevated by H2O2 administration (p < .001). All the above results indicated that the model of H2O2-induce oxidative damage in HTM cells was successfully constructed.
Figure 1. H2O2 evoked HTM cells oxidative damage. (A) After disposition with disparate concentrations of H2O2 (0-400 μM), cell viability was estimated via CCK-8. HTM cells were stimulated with 200 μM H2O2, (B) cell apoptosis, (C) pro-caspase-3, cleaved-caspase-3, pro-caspase-9 and cleaved-caspase-9, and (D) ROS production were assessed by flow cytometry, western blot and DCFH-DA staining. CCK-8: Cell Counting Kit-8; ROS: reactive oxygen species; DCFH-DA: 2,7-dichlorofluorescein diacetate; *p < .05; **p < .01; ***p < .001.
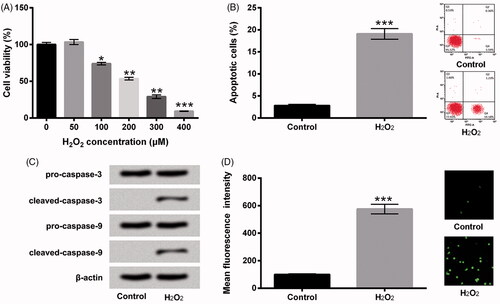
Sal relieved H2O2-induced oxidative damage in HTM cells
Subsequently, the diverse concentrations of Sal (0, 1, 2, 3, 4, 5 μM) were utilized to dispose HTM cells, cell viability was estimated using CCK-8 assay. As shown in , we discovered that cell viability was prohibited by Sal at the concentration of 5 μM (p < .05). However, there was no definite effect of other concentrations of Sal (1, 2, 3, 4 μM) on cell viability in HTM cells. To further probe the impacts of Sal on H2O2-induced oxidative damage in HTM cells, we selected the concentrations of Sal (2, 3, 4 μM) to dispose HTM cells, and cell viability was determined again. In , the results showed that Sal accelerated cell viability in H2O2-injured HTM cells at the concentrations of 3 and 4 μM (p < .05). Then, 3 μM Sal was employed to stimulate HTM cells in the subsequent experiments. Additionally, Sal significantly repressed cell apoptosis (p < .05, ), meanwhile down-regulated cleaved-caspase-3 and cleaved-caspase-9 in H2O2-injured HTM cells (). Besides, the ROS generation was markedly repressed by Sal in H2O2-injured HTM cells (p < .05, ). Based on these results, we concluded that Sal could alleviate H2O2-induced oxidative damage in HTM cells.
Figure 2. Sal relieved H2O2-evoked oxidative damage in HTM cells. (A) After administration with diverse concentrations of Sal (0–5 μM), cell viability was examined via CCK-8. (B) After disposition with 200 μM H2O2 and Sal (2, 3 and 4 μM), cell viability was appraised using CCK-8 again. HTM cells were stimulated with 200 μM H2O2 alone or 200 μM H2O2 + 3 μM Sal, then (C) the percentage of apoptotic cells, (D) pro-caspase-3/-9 and cleaved-caspase-3/-9, and (E) ROS production were estimated by flow cytometry, western blot and DCFH-DA staining. Sal: salidroside; CCK-8: Cell Counting Kit-8; ROS: reactive oxygen species; DCFH-DA: 2,7-dichlorofluorescein diacetate; *p < .05; **p < .01.
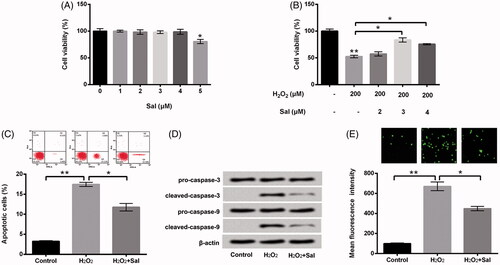
Sal enhanced miR-27a expression in H2O2-injured HTM cells
Next, miR-27a expression in Sal, H2O2 or H2O2 together with Sal was evaluated using RT-qPCR. As shown in , we discovered that miR-27a was up-regulated in Sal alone-disposed HTM cells (p < .001), but down-regulated in H2O2-stimulated HTM cells as compared with control group (p < .05). Nevertheless, H2O2 together with Sal significantly enhanced miR-27a expression in HTM cells as compared with only H2O2 administration (p < .001). These data indicated that miR-27a might be a momentous controller in the process of Sal protected HTM cells against H2O2-evoked oxidative damage.
Figure 3. Sal enhanced miR-27a expression in H2O2-injured HTM cells. HTM cells were disposed with 3 μM Sal alone, 200 μM H2O2 alone or 200 μM H2O2 + 3 μM Sal, miR-27a expression was appraised via RT-qPCR. Sal: salidroside; miR-27a: microRNA-27a; RT-qPCR: reverse transcription-quantitative PCR; *p < .05; ***p < .001.
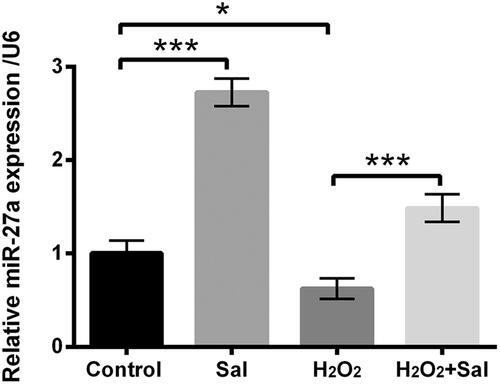
Sal mitigated H2O2-induced oxidative damage through elevation of miR-27a in HTM cells
To further clarify the functions of miR-27a in H2O2-injured HTM cells, miR-27a inhibitor and its correlative control were transfected into HTM cells, and the transfection efficiency was assessed using RT-qPCR. As results displayed in , miR-27a was significantly repressed in miR-27a inhibitor transfected cells as compared with that in NC transfected cells (p < .01), stating that miR-27a was successfully prohibited after transfection. Then, the impacts of repressed miR-27a on cell viability, apoptosis and ROS level were appraised. We disclosed that repressed miR-27a prohibited cell viability (p < .01, ), evoked apoptosis (p < .001, ), increased cleaved-caspase-3 and cleaved-caspase-9 expression (), meanwhile elevated ROS generation (p < .001, ). Afterwards, transfected cells or un-transfected cells were disposed by H2O2 or H2O2 together with Sal, cell viability, apoptosis and ROS production were examined again. We discovered that the regulatory impacts of Sal on H2O2-injured HTM cells were all reversed by repression of miR-27a, as hindered cell viability (p < .05), evoked apoptosis (p < .05), enhanced cleaved-caspase-3 and cleaved-caspase-9, meanwhile elevated ROS production (p < .05) (). These results indicated that Sal possibly protected HTM cells against H2O2-induced oxidative damage through enhancement of miR-27a.
Figure 4. Repressed miR-27a hindered cell growth and elevated ROS level in HTM cells. After miR-27a inhibitor and NC transfections, (A) miR-27a expression was estimated via RT-qPCR. (B) Cell viability, (C) the percentage of apoptotic cells, (D) pro-caspase-3/-9 and cleaved-caspase-3/-9, and (E) ROS production were appraised using CCK-8, flow cytometry, western blot and DCFH-DA staining. MiR-27a: microRNA-27a; RT-qPCR: reverse transcription-quantitative PCR; CCK-8: Cell Counting Kit-8; ROS: reactive oxygen species; DCFH-DA: 2,7-dichlorofluorescein diacetate; **p < .01; ***p < .001.
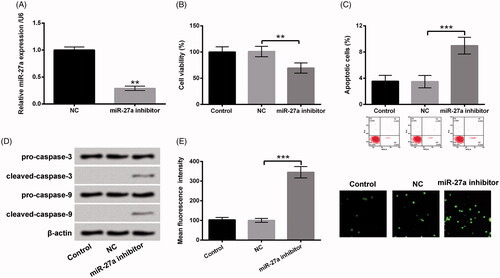
Figure 5. Sal eased H2O2-evoked oxidative damage through elevation of miR-27a in HTM cells. Transfected miR-27a inhibitor cells or NC cells were disposed with 200 μM H2O2 alone or 200 μM H2O2 + 3 μM Sal, (A) cell viability, (B) apoptosis, (C) pro-caspase-3/-9, cleaved-caspase-3/-9, and (D) ROS production were detected using CCK-8, flow cytometry, western blot and DCFH-DA staining. Sal: salidroside; miR-27a: microRNA-27a; NC: negative control; CCK-8: Cell Counting Kit-8; ROS: reactive oxygen species; DCFH-DA: 2,7-dichlorofluorescein diacetate; *p < .05; **p < .01.
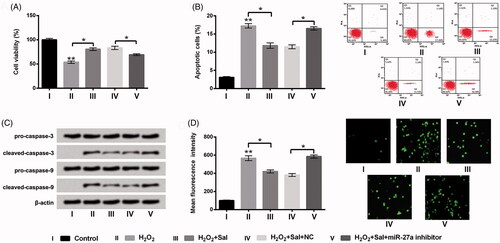
Sal activated PI3K/AKT and Wnt/β-catenin pathways through enhancement of miR-27a
To further find out the underlying mechanism of Sal protected H2O2-injured HTM cells, the impacts of PI3K/AKT and Wnt/β-catenin pathways on H2O2 and Sal disposed HTM cells after miR-27a inhibitor transfection were estimated. We observed that suppressed miR-27a restrained p-PI3K, p-AKT and β-catenin protein levels in HTM cells (p < .05 or p < .01, ). Additionally, we observed that H2O2 administration also hindered p-PI3K, p-AKT and β-catenin protein levels (p < .05 or p < .001, ). Nonetheless, Sal notably up-regulated p-PI3K, p-AKT and β-catenin in H2O2-disposed HTM cells (p < .05 or p < .001, ). When HTM cells were transfected with miR-27a inhibitor, the accelerative impacts of Sal on these two signalling pathways was overturned by repressed miR-27a (p < .05 or p < .001, ). The above-mentioned observations suggested that Sal activated PI3K/AKT and Wnt/β-catenin pathway through enhancement of miR-27a, thereby mitigating H2O2-evoked oxidative damage in HTM cells.
Figure 6. Repressed miR-27a blocked PI3K/AKT and Wnt/β-catenin pathways in HTM cells. HTM cells were transfected with miR-27a inhibitor and NC, (A and B) p/t-PI3K and p/t-AKT, and (C and D) β-catenin protein levels were appraised using western blot. MiR-27a: microRNA-27a; PI3K: phosphatidylinositol 3-kinase; AKT: protein kinase B; *p < .05; **p < .01.
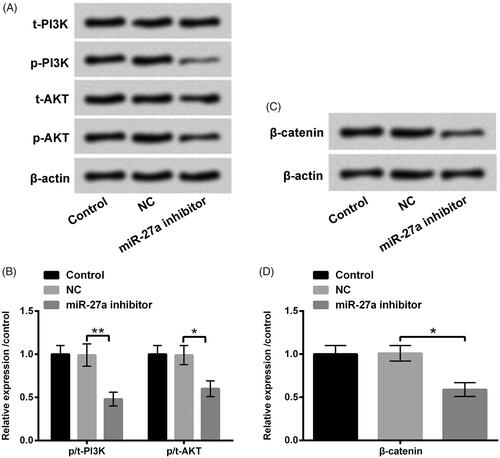
Figure 7. Sal activated PI3K/AKT and Wnt/β-catenin pathways through enhancement of miR-27a in H2O2-disposed HTM cells. After miR-27a and NC transfection, cells were disposed with 200 μM H2O2 alone or 200 μM H2O2 + 3 μM Sal, (A and B) p/t-PI3K and p/t-AKT, and (C and D) β-catenin protein levels were estimated by western blot assay. Sal: salidroside; miR-27a: microRNA-27a; PI3K: phosphatidylinositol 3-kinase; AKT: protein kinase B; *p < .05; ***p < .001.
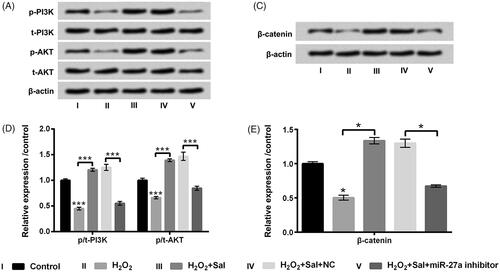
Discussion
In the actual study, we constructed a H2O2-evoked oxidative damage model in vitro and probed whether Sal exerted the protective activity in H2O2-injured HTM cells. We disclosed that Sal evidently relieved H2O2-induced oxidative damage in HTM cells, as facilitating cell viability, restraining apoptosis, repressing cleaved-caspase-3/-9 and prohibiting ROS production. Additionally, we found that miR-27a expression was up-regulated by Sal, and inhibition of miR-27a obviously overturned the protective activity of Sal in H2O2-injured HTM cells. Furthermore, PI3K/AKT and Wnt/β-catenin pathways were activated by Sal disposition through enhancing miR-27a in H2O2-injured HTM cells.
It is reported that high intraocular pressure has become a pivotal risk factor for causing glaucoma [Citation23]. TM is momentous component of the aqueous humour system, and abnormality of TM could affect the intraocular pressure [Citation24]. Recent study has testified that oxidative stress can evoke TM cells endoplasmic reticulum stress (ERS), thereby affecting the remodelling of the extracellular matrix of TM [Citation25]. Oxidative stress is caused by an imbalance between ROS production and antioxidants level [Citation26]. As a major ROS, H2O2 can alter the intracellular redox state and induce oxidative damage through changing into a highly reactive hydroxyl radical [Citation27]. Several studies have proven that the level of H2O2 in mitochondria was significantly enhanced in the pathogenesis of neurodegenerative diseases [Citation28,Citation29]. Therefore, H2O2 has been extensively used to construct a cell model of oxidative stress for studying the neuro-protective action. Based on these previous studies, we constructed a H2O2-evoked oxidative damage model in HTM cells. Results disclosed that H2O2 administration repressed cell viability, accelerated apoptosis, elevated cleaved-caspase-3/-9 protein level, and enhanced ROS generation, indicating that the oxidative cell damage model was successfully constructed.
Increasing evidence testified that TCM administration exerted anti-oxidative activity in diversified diseases [Citation30,Citation31]. For example, Lv et al. disclosed that Crocin could protect retinal ganglion cells against H2O2-evoked oxidative damage via mediating mitochondrial pathway and activation of NF-κB pathway [Citation32]. Luna et al. found that Resveratrol could effectively prevent the expression of glaucoma markers (intracellular ROS and inflammatory markers) induced by chronic oxidative stress in TM cells [Citation11]. Sal has been reported to be a strong anti-oxidative supplement in TCM [Citation33]. Study from Shi et al. discovered that Sal could protect retinal endothelial cells against H2O2-evoked injury through adjusting oxidative status and apoptosis [Citation34]. Nonetheless, whether Sal could protect HTM cells against H2O2-evoked oxidative damage remain vague. In the passing study, we discovered that Sal obviously relieved H2O2-evoked oxidative damage in HTM cells. These data indicated that Sal might be a protector in H2O2-injured HTM cells.
Studies have been proven the closely relevance between miRNAs and oxidative stress [Citation35,Citation36]. One study displayed that miR-184 could prevent chronic oxidative stress evoked HTM cells apoptosis and cytotoxicity via targeting hypoxia-inducible factor-1α (HIF-1α) [Citation37]. MiR-27a is a popular miRNA in the resent studies and has been reported to be linked to the development of cancers [Citation38]. Nevertheless, to our best knowledge, there is no evidence to uncover the role of miR-27a in H2O2-evoked oxidative damage. In the actual study, we testified the enhancement of miR-27a in Sal and H2O2 co-stimulated HTM cells. Therefore, we supposed that miR-27a might be a momentous regulator for the protective role of Sal in H2O2-injured cells. To verify the hypothesis, miR-27a inhibitor was transfected into HTM cells to repress miR-27a expression in the disposed HTM cells. As expected, inhibition of miR-27a significantly overturned the protective effect of Sal on H2O2-injured cells. These data suggested that Sal weakened H2O2-evoked oxidative damage might through elevation of miR-27a in HTM cells
PI3K/AKT and Wnt/β-catenin have been proven to be linked to oxidative stress in diverse diseases [Citation39,Citation40]. Study from Kang et al. found that Myricetin could protect cells against H2O2-evoked apoptosis through mediating PI3K/AKT pathway [Citation41]. Qi et al. recently stated that Wnt/β-catenin pathway played a crucial role in the protective impacts of FDP-Sr against oxidative stress evoked apoptosis in MC3T3-E1 cell [Citation42]. Wu et al. demonstrated that Sal could protect PC12 cells against oxidative stress injury via regulation of Wnt/β-catenin pathway [Citation43]. Nonetheless, the mediatory impacts PI3K/AKT and Wnt/β-catenin on H2O2-injured HTM cells have not been reported. Our study discovered that Sal activated PI3K/AKT and Wnt/β-catenin pathways via enhancement of miR-27a in H2O2-injured HTM cells.
Taken together, these investigations testified that Sal could protect HTM cells against H2O2-evoked oxidative damage via activating PI3K/AKT and Wnt/β-catenin pathways through enhancement of miR-27a. These data might provide a neoteric therapeutic strategy for the remedy of glaucoma. Further studies are still necessary for probing other impacts of Sal on glaucoma.
Disclosure statement
No potential conflict of interest was reported by the authors.
References
- Danesh-Meyer HV, Levin LA. Glaucoma as a neurodegenerative disease. J Neuroophthalmol. 2015;35:S22–S28.
- Mantravadi AV, Vadhar N. Glaucoma. Primary care: clinics in office practice. 2015;42:437–449.
- Loewen RT, Roy P, Parikh HA, et al. Impact of a glaucoma severity index on results of trabectome surgery: larger pressure reduction in more severe glaucoma. PLoS One. 2016;11:e0151926.
- Mwanza JC, Hochberg JT, Banitt MR, et al. Lack of association between glaucoma and macular choroidal thickness measured with enhanced depth-imaging optical coherence tomography. Invest Ophthalmol Vis Sci. 2011;52:3430–3435.
- Pandey A, Singh P, Kaul A, et al. Glaucoma: role of neuroprotective agents. Int J Basic Clin Pharmacol. 2014;3:755–760.
- Zhang SW, Huang Y, Li Q, et al. Changes of anterior scleral thickness after surgery in acute angle-closure glaucom a patients with high intraocular pressure. Int Eye Sci. 2016;16:695–697.
- Jonas JB, Nagaoka N, Fang YX, et al. Intraocular pressure and glaucomatous optic neuropathy in high myopia. Invest Ophthalmol Vis Sci. 2017;58:5897–5906.
- Noecker RJ. The management of glaucoma and intraocular hypertension: current approaches and recent advances. Ther Clin Risk Manag. 2006;2:193–206.
- Frezzotti P, Mittica V, Martone G, et al. Longterm follow-up of diode laser transscleral cyclophotocoagulation in the treatment of refractory glaucoma. Acta Ophthalmol. 2010;88:150–155.
- Banerjee J, Leung C-T, Li A, et al. Regulatory roles of anoctamin-6 in human trabecular meshwork cells. Invest Ophthalmol Vis Sci. 2017;58:492–501.
- Luna C, Li G, Liton PB, et al. Resveratrol prevents the expression of glaucoma markers induced by chronic oxidative stress in trabecular meshwork cells. Food Chem Toxicol. 2009;47:198–204.
- Guo Y, Zhao Y, Zheng C, et al. Synthesis, biological activity of salidroside and its analogues. Chem Pharm Bull. 2010;58:1627–1629.
- Ma C, Hu L, Tao G, et al. An UPLC-MS-based metabolomics investigation on the anti-fatigue effect of salidroside in mice. J Pharm Biomed Anal. 2015;105:84–90.
- Hu X, Lin S, Yu D, et al. A preliminary study: the anti-proliferation effect of salidroside on different human cancer cell lines. Cell Biol Toxicol. 2010;26:499–507.
- Kong YJ, Shi XF, Jun MA, et al. Role of rhodiola active ingredient on central nervous system. J Liaoning Univ Tradit Chinese Med. 2012;14:67–68.
- Chen X, Zhang Q, Cheng Q, et al. Protective effect of salidroside against H2O2-induced cell apoptosis in primary culture of rat hippocampal neurons. Mol Cell Biochem. 2009;332:85–93.
- Cai L, Wang H, Li Q, et al. Salidroside inhibits H2O2-induced apoptosis in PC12 cells by preventing cytochrome c release and inactivating of caspase cascade. Acta Biochim Biophys Sin. 2008;40:796–802.
- Ma Y, Yu S, Zhao W, et al. miR-27a regulates the growth, colony formation and migration of pancreatic cancer cells by targeting Sprouty2. Cancer Lett. 2010;298:150–158.
- Scheibner KA, Teaboldt B, Hauer MC, et al. MiR-27a functions as a tumor suppressor in acute leukemia by regulating 14-3-3theta. PLoS One. 2012;7:e50895.
- Cai Q, Wang T, Yang W-J, et al. Protective mechanisms of microRNA-27a against oxygen-glucose deprivation-induced injuries in hippocampal neurons. Neural Regen Res. 2016;11:1285–1292.
- Thulasingam S, Massilamany C, Gangaplara A, et al. miR-27b*, an oxidative stress-responsive microRNA modulates nuclear factor-kB pathway in RAW 264.7 cells. Mol Cell Biochem. 2011;352:181–188.
- Livak KJ, Schmittgen TD. Analysis of relative gene expression data using real-time quantitative PCR and the 2(-delta delta C(T)) method. Methods. 2001;25:402–408.
- Kim SH, Lee EJ, Han JC, et al. The effect of diurnal fluctuation in intraocular pressure on the evaluation of risk factors of progression in normal tension glaucoma. PLoS One. 2016;11:e0164876.
- Kim JW. Effect of nitric oxide on the expression of matrix metalloproteinase and its association with migration of cultured trabecular meshwork cells. Korean J Ophthalmol. 2016;30:66–75.
- Zhao J, Wang S, Zhong W, et al. Oxidative stress in the trabecular meshwork (Review). Int J Mol Med. 2016;38:995–1002.
- Choi JY, Kang JT, Park SJ, et al. Effect of 7,8-dihydroxyflavone as an antioxidant on in vitro maturation of oocytes and development of parthenogenetic embryos in pigs. J Reprod Dev. 2013;59:450–456.
- Virdis A, Duranti E, Taddei S. Oxidative stress and vascular damage in hypertension: role of angiotensin II. Int J Hypertens. 2011;2011:1.
- Roediger B, Armati PJ. Oxidative stress induces axonal beading in cultured human brain tissue. Neurobiol Dis. 2003;13:222–229.
- Lv R, Du L, Lu C, et al. Allicin protects against H(2)O(2)-induced apoptosis of PC12 cells via the mitochondrial pathway. Exp Ther Med. 2017;14:2053–2059.
- Zhao Y, Zhou G, Wang J, et al. Paeoniflorin protects against ANIT-induced cholestasis by ameliorating oxidative stress in rats. Food Chem Toxicol. 2013;58:242–248.
- Gim GT, Kim HM, Kim J, et al. Antioxidant effect of tianwang buxin pills a traditional Chinese medicine formula: double-blind, randomized controlled trial. Am J Chin Med. 2009;37:227–239.
- Lv B, Chen T, Xu Z, et al. Crocin protects retinal ganglion cells against H2O2-induced damage through the mitochondrial pathway and activation of NF-kappaB. Int J Mol Med. 2016;37:225–232.
- Xu MC, Shi HM, Wang H, et al. Salidroside protects against hydrogen peroxide-induced injury in HUVECs via the regulation of REDD1 and mTOR activation. Mol Med Rep. 2013;8:147–153.
- Shi K, Wang X, Zhu J, et al. Salidroside protects retinal endothelial cells against hydrogen peroxide-induced injury via modulating oxidative status and apoptosis. Biosci Biotechnol Biochem. 2015;79:1406–1413.
- Ayaz L, Dinc E. Evaluation of microRNA responses in ARPE-19 cells against the oxidative stress. Cutan Ocul Toxicol. 2018;37:121–126.
- Yildirim SS, Akman D, Catalucci D, et al. Relationship between downregulation of miRNAs and increase of oxidative stress in the development of diabetic cardiac dysfunction: junctin as a target protein of miR-1. Cell Biochem Biophys. 2013;67:1397–1408.
- Wang D, Wang H, Wu X, et al. MiR-184 prevents chronic oxidative stress induced human trabecular meshwork cells apoptosis and cytotoxicity in vitro by targeting hypoxia-inducible factor 1α. Int J Clin Exp Pathol. 2017;10:2754–2763.
- Wang YL, Gong WG, Yuan QL. Effects of miR-27a upregulation on thyroid cancer cells migration, invasion, and angiogenesis. Genet Mol Res. 2016;15:gmr15049070.
- Zhang Y, Yang JH. Activation of the PI3K/Akt pathway by oxidative stress mediates high glucose-induced increase of adipogenic differentiation in primary rat osteoblasts. J Cell Biochem. 2013;114:2595–2602.
- Tao GZ, Lehwald N, Jang KY, et al. Wnt/β-catenin signaling protects mouse liver against oxidative stress-induced apoptosis through the inhibition of forkhead transcription factor FoxO3. J Biol Chem. 2013;288:17214–17224.
- Kang KA, Wang ZH, Zhang R, et al. Myricetin protects cells against oxidative stress-induced apoptosis via regulation of PI3K/Akt and MAPK signaling pathways. IJMS. 2010;11:4348–4360.
- Qi HH, Bao J, Zhang Q, et al. Wnt/β-catenin signaling plays an important role in the protective effects of FDP-Sr against oxidative stress induced apoptosis in MC3T3-E1 cell. Bioorg Med Chem Lett. 2016;26:4720–4723.
- Wu DM, Han XR, Wen X, et al. Salidroside protection against oxidative stress injury through the Wnt/beta-catenin signaling pathway in rats with parkinson's disease. Cell Physiol Biochem. 2018;46:1793–1806.